1. Introduction
The middle-infrared band between 1.5 and 4 μm is a crucial atmospheric window which contains the characteristic curves of various gases, such as CO2, CO, H2O, HF, etc. Such gases have a strong absorption peak, and the dispersion of the laser is weak in this band[1–4]. Thus, the optoelectronic devices working in this condition play an important role in the regions of optical and environmental monitoring, free space communication, infrared test, infrared radar, biotechnology, etc[5–7]. Therefore, this field has become the scientific research focus and a hotspot in many countries, and its progress will promote the improvement of the regions mentioned above as well.
The band gap of antimony is exactly located in the middle-infrared band between 1.5–4.5 μm, especially InAs, AlSb and GaSb whose lattice constant is about 6.1 ?. The similar lattice constant leads to favourable lattice and strain matching among them, which makes it prone to form the compounds with low defect density. The band gap of InGaAsSb and AlGaAsSb which matches the substrate can be regulated in a wide range, and its emission wavelength covers 1.5–4.5 μm, so the GaSb-based laser should be a top priority when fabricating the mid-infrared semiconductor lasers in this wavelength range.
Traditional antimony lasers, such as lasers with the Fabry–Perot (F–P) resonator, always have a large linewidth, fast changing working current and spectra varying with temperature[8]. Most of the mid-infrared semiconductor lasers should be required to work in a single longitudinal mode and have a single lasing wavelength as well as the narrow linewidth, so that there are no big broadenings after long distance transmission. In the field of III–V semiconductor lasers, the main ways to achieve a single longitudinal mode are utilizing the distributed feedback (DFB) cavity and DBR cavity[9]. Being subject to the existing conditions, especially the lithography condition, it is difficult to fabricate low order DBR graphics in the hundreds nanometers level[10, 11]. Only by using high-accuracy lithography, such as holographic lithography, electron beam lithography and nanoimprint lithography, can meet the demand mentioned above[12, 13]. Compared with these methods which possess high cost and long development period, conventional UV lithography can only fabricate graphics above 2 μm accurately, but it is enough for the high order DBR with relatively long grating period to guarantee the precision. Meanwhile, conventional UV lithography has a short development period and its parameter can be flexibly adjusted to improve the efficiency of development[14]. GaSb-based DBR lasers with narrow linewidth have rarely been reported recently, so we compared the devices with other GaSb-based diode lasers with narrow linewidth, we obtain almost the same SMSR with the devices they reported (around 24 dB), but the lithography method we used was more economical, compared with the electron beam lithography and holographic lithography they applied. In addition, what we focused on most was the optimization of the current available process, however, the papers mentioned above paid little attention to this aspect.
In this letter, the growth and fabrication process of GaSb-based type-I quantum well semiconductor lasers were investigated in detail. The 23rd-order distributed Bragg reflection grating devices were fabricated by using conventional UV lithography. In order to improve the quality of gratings, three main problems were solved with optimized condition. We optimized the etching condition to reduce the lateral undercut and regulate the etching depth as well as obtain smooth and vertical side walls. We also discussed the influence of different duty ratio and etching depth on the mode selection, which provide empirical data for further study on DBR gratings. These devices have been shown to produce single-mode emission near 2 μm and excellent SMSR of more than 22.5 dB.
2.
Design and fabrication
2.1
Material growth
The lasers were fabricated out of a separate confinement heterostructure grown by molecular beam epitaxy (MBE) on an n-GaSb (100) substrate[15, 16]. Three In0.42Ga0.58As0.16Sb0.84 quantum-wells (10 nm) with Al0.27Ga0.73As0.02Sb0.98 barrier layer (20 nm) embedded in Al0.27Ga0.73As0.02Sb0.98 waveguide layer (630 nm) acted as an active region. The active region was surrounded by lattice matched Al0.55Ga0.45 As0.05Sb0.95 cladding layers (2000 nm) and then capped with highly doped p-GaSb cap layer (250 nm)[17]. The typical epitaxial structure of the devices is shown in Fig. 1.

class="figure_img" id="Figure1"/>
Download
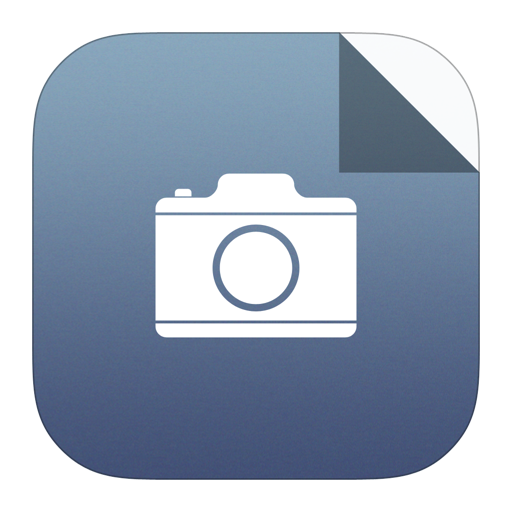
Larger image
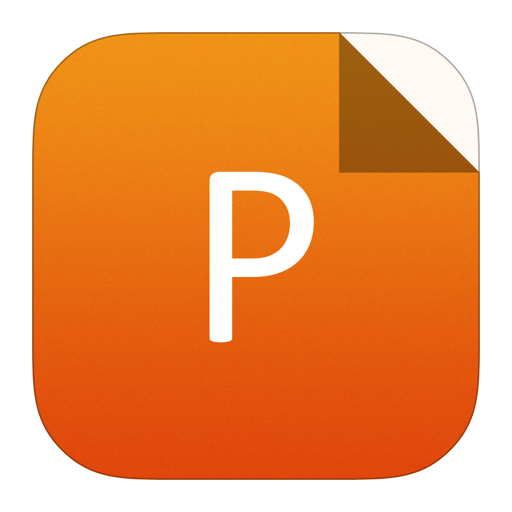
PowerPoint slide
Figure1.
(Color?online) Epitaxial structure used to fabricate the DBR laser.
2.2
Optimization of gratings
The etching depth can affect the effective index of refraction (EIOR) of the gratings and then change the coupling coefficient. As for the etching near the waveguide layer, it is more sensitive to the feedback disturbance of the optical field, so the etching depth needs to be rigidly controlled. Another issue that must be of concern is the lateral undercut, in other words, the etching width. Because ICP etching is nonselective, it is mainly reflected in the etching of the side wall. The etching width influences the duty ratio of the gratings and then affects the coupling coefficient[18]. The third issue mentioned above is the steepness of the side wall, by increasing which can enhance the feedback effect of gratings to the optical field. In the case of high-order gratings with low coupling coefficient, this is an effective way to improve the mode selection ability[19].
In order to achieve the optimized condition considering the problems mentioned above, we optimized the key process parameters of ICP etching at first. Ar usually worked as the carrier gas in the ICP etching process of GaSb and AlGaAsSb, inert gas Ar can improve the generation efficiency of plasma and strengthen the physical bombardment. The reaction gases include SiCl4, BCl3 and Cl2, which can be dissociated into plasma, and then Cl ions react with III–V materials to form volatile compounds. Therefore, the type and ratio of reaction gases can change the etching effect of GaSb-based materials. SiCl4 and BCl3 have different characteristics when working as an etching gas. Using SiCl4 as the etching gas produces a high etching but better lateral morphology if the etching rate is under control. As for BCl3, its etching rate is low and easy to regulate, but the broadening is wider. Cl2 typically produces a high etch rate,but results in a rougher surface[20]. We designed five kinds of gas ratio schemes according to the previous etching experience and the characteristics of the etching gases, which were applied on an epi-wafer. Five kinds of gas ratio schemes are shown in Table 1.
No. 1 | No. 2 | No. 3 | No. 4 | No. 5 |
Cl2:1 | Cl2:0 | Cl2:0 | Cl2:4 | Cl2:5 |
SiCl4:3 | SiCl4:3 | SiCl4:2 | BCl3:10 | BCl3:10 |
Ar:5 | Ar:5 | Ar:5 | Ar:3 | Ar:3 |
Table1.
Different ICP etching gases and flowrate ratios.
Table options
-->

Download as CSV
No. 1 | No. 2 | No. 3 | No. 4 | No. 5 |
Cl2:1 | Cl2:0 | Cl2:0 | Cl2:4 | Cl2:5 |
SiCl4:3 | SiCl4:3 | SiCl4:2 | BCl3:10 | BCl3:10 |
Ar:5 | Ar:5 | Ar:5 | Ar:3 | Ar:3 |
The etching results under five kinds of etching ratio are shown in Fig. 2. The etching methods No. 1–3 compared with methods 4–5 have better steepness, which can be kept within 95°. Although their etching morphologies are analogous, etching rates differ greatly and are 27, 20, and 16 nm/s, respectively. We can figure out that the addition of Cl2 increases the rate of chemical etching, and the increase of SiCl4 flow improves the etching rate by boosting the plasma concentration. For the purpose of accurate control over etching depth, the depth rate should be as small as possible, that is why we consider No. 3 is the relatively suitable scheme. Its favourable steepness of 91° and etch width broadening of 440 nm, which are the best among five designs, is also a vital reason to choose No. 3.

class="figure_img" id="Figure2"/>
Download
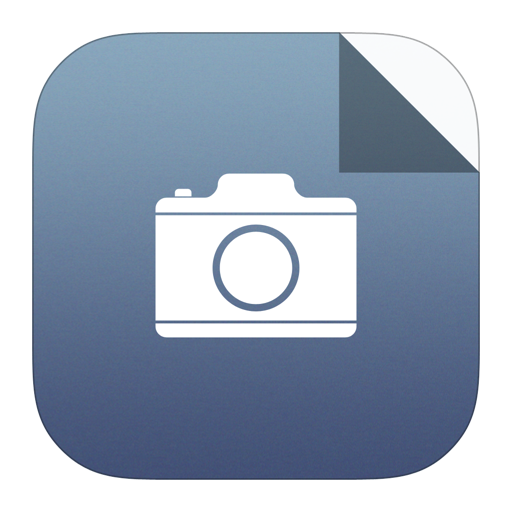
Larger image
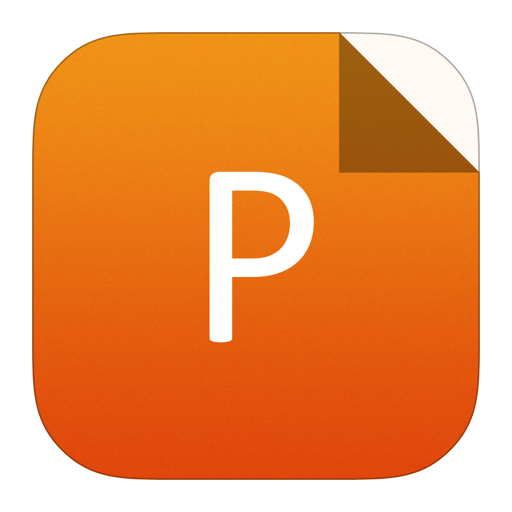
PowerPoint slide
Figure2.
SEM photos of etching morphology under different etching conditions.
The reason why we replaced the photoresist with SiO2 + photoresist as the mask layer is to improve the etching steepness and narrow the etching width expansion[21]. The differences are shown in Fig. 3. Only using the soft mask (photoresist) could worsen the etching steepness and expand the etching width due to the shrinkage and lateral consumption of the photoresist, which makes a big difference in the designed duty ratio. Combining hard mask (SiO2) with the remaining soft mask reduce the consumption of hard mask during etching the epitaxial structure, thus limiting the etching width expansion.

class="figure_img" id="Figure3"/>
Download
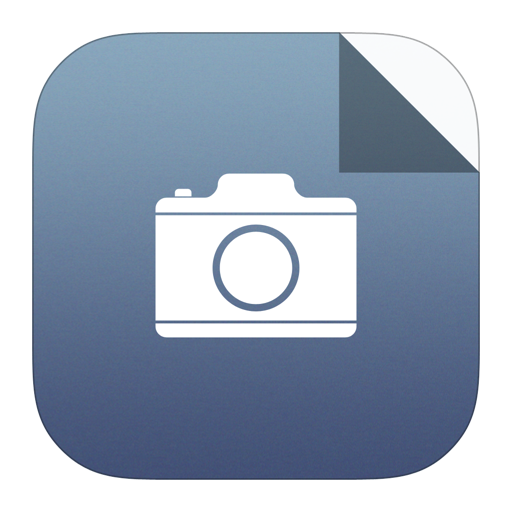
Larger image
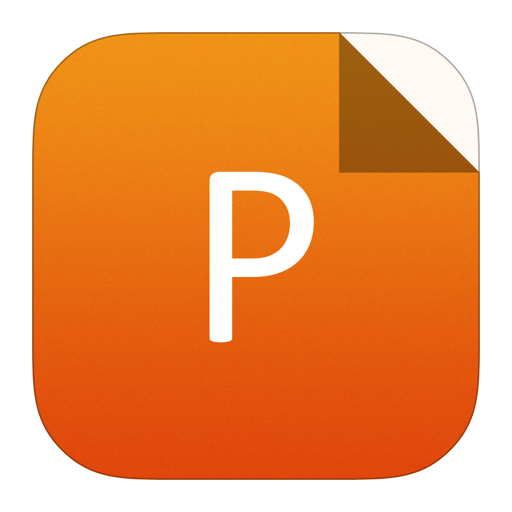
PowerPoint slide
Figure3.
The morphology of different mask layers.
Taking advantage of the optimized etching conditions mentioned above, we fabricated 2 μm GaSb-based 23rd-order DBR lasers with a ridge width of 100 μm, cavity length of 3000 μm and etching depth of 1.7 μm as well as the duty ratio of 57%, then achieve the maximum SMSR of 15.2 dB. Comparing the DBR device and F–P cavity device on the same epitaxial structure as shown in Fig. 4, the high-order DBR device possesses stronger ability for selecting longitudinal mode, whether at the same current (Fig. 4(a)) or output optical power (Fig. 4(b)).

class="figure_img" id="Figure4"/>
Download
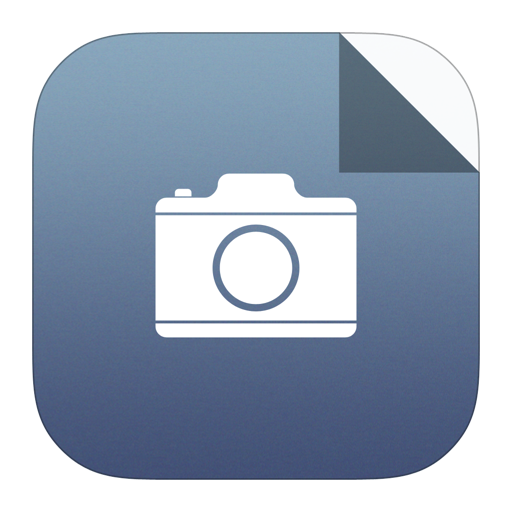
Larger image
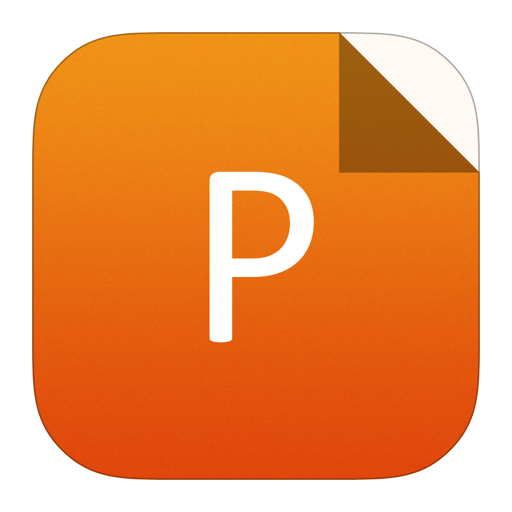
PowerPoint slide
Figure4.
(Color?online) Comparison of the lasing spectrum under the same current and output power.
2.3
Device fabrication
The traditional fabrication methods of DBR gratings were used to fabricate 23rd-order gratings. At first, a 300 nm-thick SiO2 mask layer was deposited by plasma enhanced chemical vapor deposition (PECVD). Secondly, graphics of the ridge waveguide and DBR gratings were transferred to the epi-wafer by conventional UV lithography. Then we used the reactive ion etching (RIE) to etch the SiO2 exposed. After that, the photoresist remained coupled with the SiO2 layer were acting as the mask layer for ICP etching. After removed the remaining photoresist, a 200 nm-thick SiO2 layer was deposited again by PECVD. Conventional UV lithography and ICP dry etching were used to open a contact window on the SiO2 layer. The Ohm contact on the p-side was achieved by using the magnetron sputtering system to deposit 50/50/1000 nm of Ti/Pt/Au layer. The bottom Ohm contacts were formed by AuGeNi/Au of 50/300 nm, deposited by high vacuum thermal evaporation equipment. Then the n-side electrode of the device was annealed rapidly for 2 min and 10 s at 330 °C. Finally, the sample was mounted p-side up on a copper heat sink. The micrograph of the fabricated device and its lasing spectrum are shown in Figs. 5(a) and 5(b), separately.

class="figure_img" id="Figure5"/>
Download
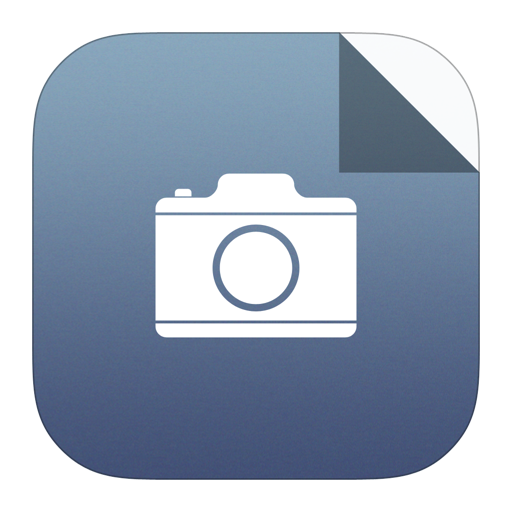
Larger image
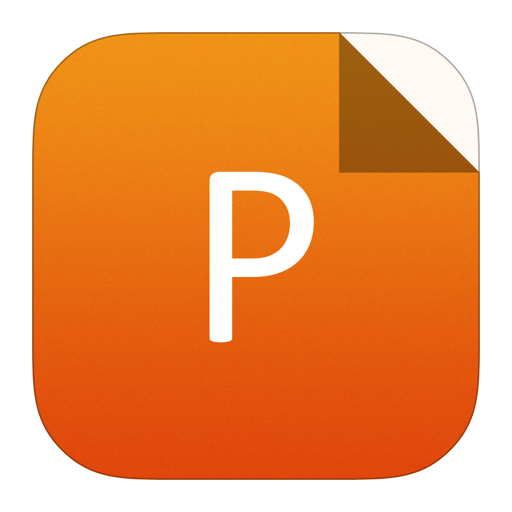
PowerPoint slide
Figure5.
(Color?online) 3D sketch of the fabricated device and its lasing spectrum.
2.4
Research on the relationship between trench depth-duty ratio with SMSR
The mode selectivity of DBR gratings is determined by the reflection spectrum of DBR, which means we can acquire the best effect of mode selection when the reflection spectrum of the DBR can be well matched with the lasing wavelength and possesses narrow peaks. An important parameter to characterize the mode selectivity of DBR lasers is SMSR, while the reflection spectrum of DBR is determined by the duty ratio and trench depth of the DBR gratings. In order to further improve the mode selectivity, we need to figure out the relationship among them. We designed a series of high-order DBR devices with different duty rations and trench depths, then measure the maximum SMSR of them to characterize their effect of mode selection.
According to the dimension precision of UV lithography, we fabricated high-order DBR devices with a gratings period of 13 μm and three separate kinds of trench depth which correspond with the etching depths of 1.55, 1.75, and 1.85 μm respectively, then applied six different duty ration ranges from 57% to 85% on each device. All devices are built on the same epitaxial structure with a ridge width of 100 μm and cavity length of 2000 μm. The relationship between etching depth, duty ratio and SMSR based on the measurement results of the maximum SMSR of devices mentioned above is shown in Fig 6. We also measured the P–I–V curves of the three typical devices, which are shown in Fig. 7. Within the range of the parameters we designed, we can figure out that the peak wavelength of DBR reflectivity decreases with the decrease of the duty ratio and increase of etching depth, according to this we can change these two parameters to adjust the peak wavelength of DBR reflectance spectrum to the lasing wavelength. We got the best effect of mode selection on devices with an etching depth of 1.55 μm and duty ratio of 85% (Fig. 7(c)). Thus, the reflection spectrum of gratings best matches the lasing wavelength.

class="figure_img" id="Figure7"/>
Download
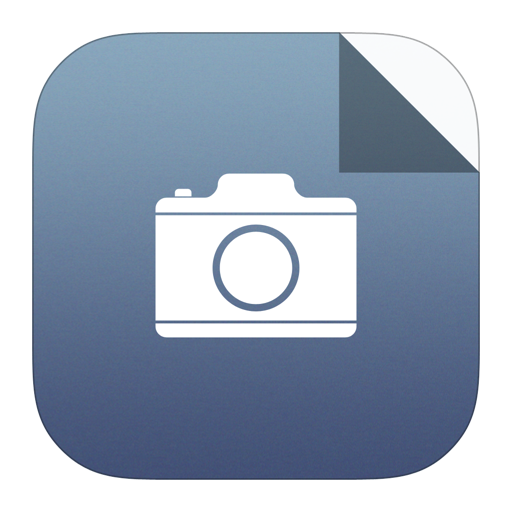
Larger image
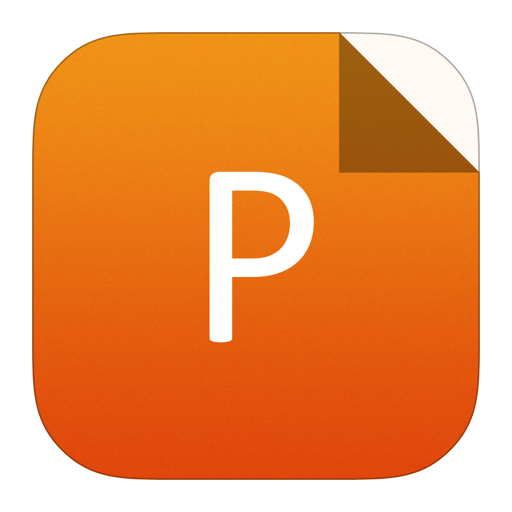
PowerPoint slide
Figure7.
(Color?online) P–I–V curves of three typical devices.

class="figure_img" id="Figure6"/>
Download
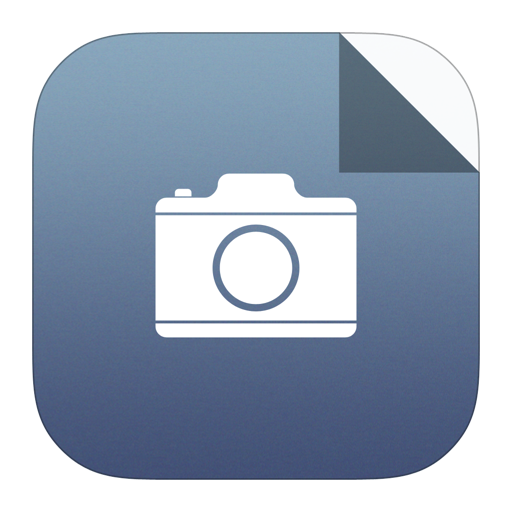
Larger image
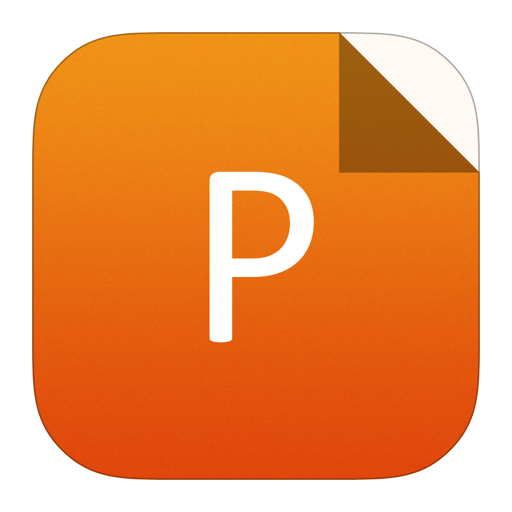
PowerPoint slide
Figure6.
(Color?online) Relationship between duty ratio, etching depth and SMSR.
3.
Measurement
Synthesizing the optimized etching conditions and device parameter mentioned in Section 2, the fabricated devices achieve single mode emission at a wavelength of 2037 nm under the current ranging from 515 to 570 mA and a side mode suppression ratio of more than 20 dB. All the devices were tested without facet coating. Optical spectra were measured with Vertex70 Fourier infrared spectrometer and infrared fluorescence test system. All measurements were done for a component with as-cleaved facets. The relationship between current and wavelength is presented in Fig. 7. The drift velocity of wavelength with current is 0.0057 nm/mA and is less than the value of 0.008 nm/mA when the wavelength is not matched.
The maximum SMSR is 22.52 dB at an operating temperature of 20 °C with the injection current of 535 mA and output power of 13 mW, which is shown in Figs. 8 and 7. The threshold current and slope efficiency are also shown in Fig. 7(c). The fabricated device has a relatively more obvious effect of mode selection than F–P cavity devices and high-order DFB devices, but it is possible to improve the SMSR by changing some parameters in further research, such as the ridge width. The high-order transverse mode of wide-ridge DBR lasers can produce multiple longitudinal modes, which restrict their performance of a single longitudinal mode. Compared with the low order DBR gratings, high order DBR lasers possess higher cavity loss which will reduce the outpower and conversion efficiency[22], and this is verified by the PIV curve of the optimized device in Fig. 7(c). The threshold current is higher than in the low order devices, and the outpower and conversion efficiency is lower. What we are concerned with most is the increase of SMSR, although the output power is low, it is still strong enough for the application of gas detection.

class="figure_img" id="Figure8"/>
Download
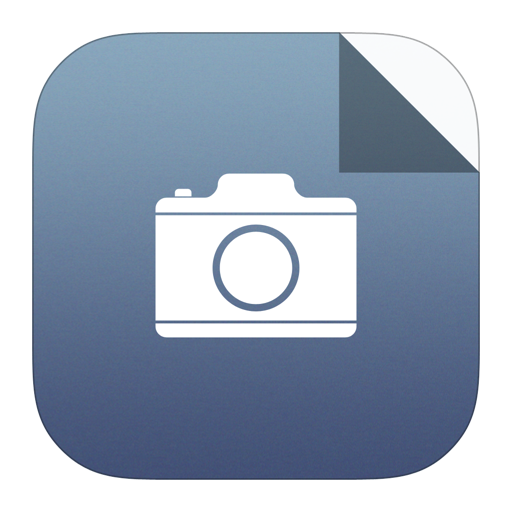
Larger image
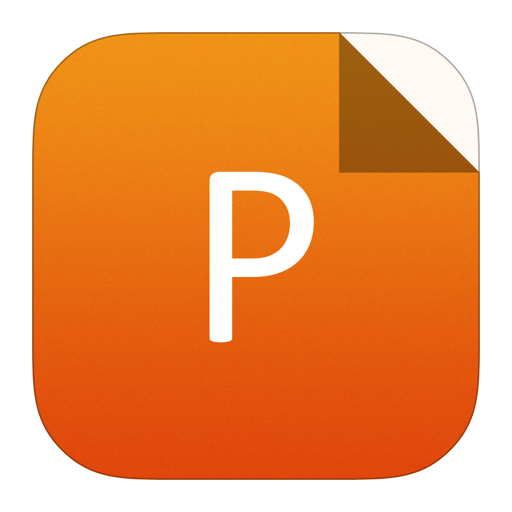
PowerPoint slide
Figure8.
(Color?online) Relationship between current and wavelength.
By reducing the ridge width we can obtain better performance of a single longitudinal mode of high-order DBR devices in further research.

class="figure_img" id="Figure9"/>
Download
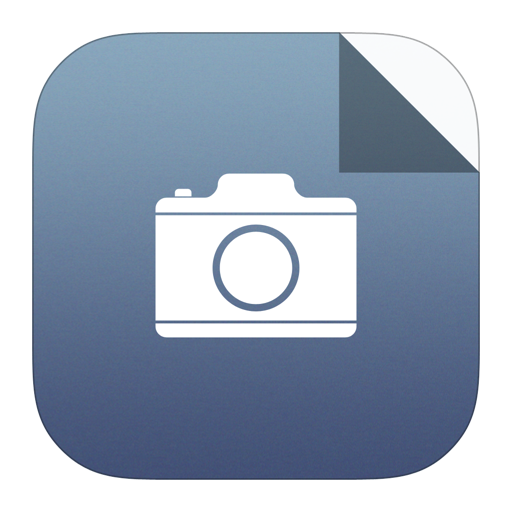
Larger image
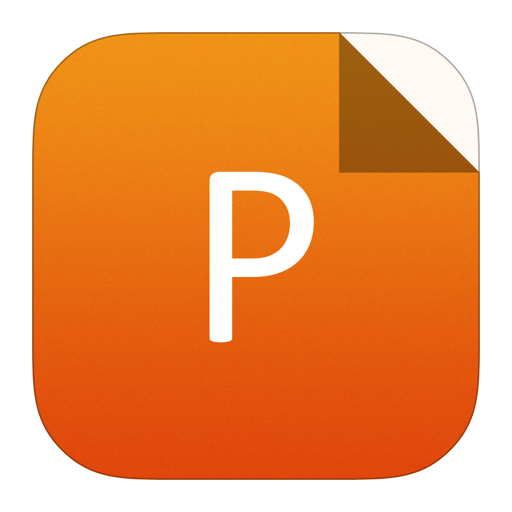
PowerPoint slide
Figure9.
The spectrum of the high-order DBR device with max SMSR.
4.
Conclusion
GaSb-based 2 μm DBR lasers with 23rd-order gratings were fabricated through conventional UV lithography. The ICP etching conditions were optimized with the etching gas ratio of Cl2 : SiCl4 : Ar turning into 0 : 2 : 5, then better morphology of gratings with controllable etching depth, narrow etching width and steep side wall of gratings were obtained. The relationship among duty ratio, etching depth and SMSR have been studied, the max SMSR of 22.52 dB can be achieved with the etching depth of 1.55 μm and duty ratio of 85%. The CW laser was emitted in single longitude mode at room temperature with a wavelength of 2037 nm, which was applicable with gas sensing in the infrared wave band.