1.
Introduction
GaN-based light-emitting diode (LED) as a light source device as well as the front of the optocoupler is widely used in military and aerospace fields[1, 2]. Therefore, the stability of the device working in the conditions of space ray irradiation has received a great deal of attention. Currently, most of the work is concentrated in the high-energy electrons and protons[2–7]. Jin et al. studied the Gamma irradiation effect of GaN multi- quantum well blue LEDs under the forward current[8]. Sawyer et al. studied low frequency noise and reliability characterization of GaN-based LEDs in the forward current region[9]. Masashi Ishii et al. analyzed noise under the forward current of LEDs, and identified the rate-limiting process of a recently developed Eu-doped GaN (GaN:Eu) red LED[10]. While Veleschuk et al. found the reverse microplasmas avalanche breakdown of the InGaN/GaN high-power LEDs as a reliability diagnostic tool[11]. Park et al. reported random telegraph noise in the reverse avalanche breakdown region of GaN-based LED[12]. They studied the reverse leakage caused by increased defects in irradiation. However, the low-frequency noise effects after Gamma irradiation in the reverse avalanche breakdown region of GaN-based LED have not been reported. In this paper, the Gamma irradiation effect of GaN-based LED which has an unstable reverse breakdown current is studied. The micro-plasma noise theory in the reverse avalanche breakdown region was used to analyze its instability, in order to get the sensitive parameters to characterize reverse avalanche breakdown unstable GaN-based LED after gamma irradiation.
2.
Correlation theory between micro plasma noise and γ irradiation
Micro-plasma noise was found in the 1960s in the PN junction reverse avalanche breakdown, since then there have been a lot of experimental and theoretical studies[13–15]. Micro-plasma is a spot of conductivity in the depleted region of the reverse biased PN junction. The micro-plasma itself is a thin conductive tube with a cross-section, surrounded by the non-conductive depletion layer. Micro-plasma occurs in a local imperfection, and it has a submicron diameter. The micro-plasma current is unstable, and the amplitude of micro-plasma current pulses is approximately constant while the DC current IR is the average of the pulses. In recent years, Abdullah et al. researched light-emitting diode micro-plasma, however they found that it is not correlated with noise[7], while Marinov found it in GaAsP light-emitting diodes, and used a randomized switching model to describe the micro-plasma noise[15].
In Compton scattering produced by γ-ray interaction with matter, energetic Compton electrons continue to interact with atoms, introducing lattice damage in the material or the formation of a variety of defects, which produce the ionization effect or displacement effect[17]. For wide bandgap GaN-based materials and devices, the total dose 2 Mrad irradiation (mainly the ionizing radiation effect), does not produce a significant displacement effect[16]. Ionizing radiation effects and new interface state traps will be introduced in the LED active region of the space charge; these defects trap the carrier, resulting in a non-radiative recombination that increases dark current. The Marinov et al.[17] research on the relation between these defects and the switching threshold found that the switching threshold is
$${I_{{ m{th}}}} = q{V_{ m{s}}} N {A_{mu}},$$ ![]() | (1) |
where Aμ is the junction area, Vs is the saturation carrier velocity at high electric field and N is the impurity concentration of the lower doped region in the PN junction, including original and new product defects (introduced by the ionizing radiation effects of γ irradiation). Once the micro-plasma is on, the bias voltage injects free carriers from both sides of the micro-plasmas region, the micro-plasma current is determined by the charge neutrality condition in semiconductors, and is
$${I_{mu}} = 2q{V_{ m{s}}} N {A_{mu}} = 2{I_{{ m{th}}}}.$$ ![]() | (2) |
A very high avalanche multiplication M0 = Ith/IG is required in the micro-plasmas region in order to turn on the micro-plasmas, because the thermally generated current IG is low and the impact ionization is the only supplier of free carriers in the depleted PN junction. In contrast, a low multiplication is sufficient when the micro-plasma is on, because only a compensation loss due to diffusion of carriers from the micro-plasma into the depleted region is required. Different micro-plasmas are consecutively activated when the reverse current is increased; as the reverse current increases, a larger number of conducting micro-plasmas appears, while the number of fluctuating micro-plasmas remains low. In this way, the micro-plasma noise disappears at some higher current. Micro-plasma noise is induced by N random switch parallel combination. When the switches are all closed, the switches are all turned off, and the current is zero. Within the time interval, dt, assumes that the connect probability of the switch being off is dt/τ1, the disconnect probability of switching on is dt/τ2. Where τ1 is the total time when the micro plasma switch is in an ON state, τ2 is the total time when the micro plasma switch is in the OFF state. Provided at a particular instant, disconnect switch N1 and closed switch N2,


$$frac{{{ m d}left( {overline {{N_1}} + Delta {N_1}} ight)}}{{{ m d}t}} = left( {overline {{N_1}} + Delta {N_1}} ight)frac{1}{{{tau _1}}} + left( {overline {{N_2}} + Delta {N_2}} ight)frac{1}{{{tau _2}}} + H(t),$$ ![]() | (3) |
where H(t) is a function of random source. In steady-state, ?N1 is the number of carriers opened, ?N2 is the number of carriers closed. ?N1 = ??N2,
m{d}}overline {{N_1}} }}{{{
m d}t}} = 0$

$$frac{{overline {{N_1}} }}{{{tau _1}}} = frac{{overline {{N_2}} }}{{{tau _2}}},$$ ![]() | (4) |
$$overline {{N_1}} = Nfrac{{{tau _1}}}{{{tau _1} + {tau _2}}},$$ ![]() | (5) |
$$overline {{N_2}} = Nfrac{{{tau _2}}}{{{tau _1} + {tau _2}}},$$ ![]() | (6) |
$$overline {Delta N_1^2} = Nfrac{{{tau _1}{tau _2}}}{{{{left( {{tau _1} + {tau _2}} ight)}^2}}},$$ ![]() | (7) |
$$frac{{{ m d}Delta {N_1}}}{{{ m d}t}} = - frac{{Delta {N_1}}}{tau } + Hleft( t ight),$$ ![]() | (8) |
where
ight)$

$${S_{Delta {N_t}}}left( f ight) = frac{{overline {4Delta N_1^2tau } }}{{1 + {omega ^2}{tau ^2}}} = 4Nfrac{{{tau _1}{tau _2}}}{{({tau _1} + {tau _{2)}}^2}}frac{tau }{{1 + {omega ^2}{tau ^2}}}.$$ ![]() | (9) |
Because the current ripple value IM is proportional to ΔN1, the power spectral density of the micro-plasma noise random switching model can be written as,
$${S_I}(f) = 4I_{ m M}^2frac{{{tau _1}{tau _2}}}{{{{({tau _1} + {tau _2})}^2}}}frac{tau }{{1 + {omega ^2}{tau ^2}}},$$ ![]() | (10) |
where IM is the micro-plasma pulse current, which has the same height and different widths in the time domain. From the above correlation theory, we concluded that the irradiation induced defects increased and accelerated the instability of the threshold current, which caused the micro plasma generation of noise.
3.
Experiment
The experimental samples GaN-based blue light-emitting diodes are produced by an electronics company in Taiwan. Epitaxial materials by MOCVD on the sapphire (Al2O3) substrate, from bottom to top for the low temperature grown GaN buffer layer, followed by the n-GaN layer, an n-AlGaN layer and the InGaN quantum well active region, where the top is a Mg-doped p-AlGaN layer and a p-GaN layer. Firstly, the growth of good LED epitaxial wafers by high-temperature annealing in a N2 environment for 10–40 min, with Mg in an active role. Then photo-resist protection technology using inductively coupled plasma etching (ICP) was used by etching at the stepped graphic, in order to produce the upper and lower electrodes. Thereafter, a p-electrode and an n-electrode metal was deposited in the up-and-down on the table, the p-electrode material is Ni/Au, and the n-electrode material is of Ti/Al/Ti/Au. Then grown in the vicinity of the PN junction layer of SiO2 as protection, and finally by grinding, dicing lobes, the die bonding was made into devices and tested.
The irradiation experiment was conducted at the Northwest Institute of Nuclear Technology in China, using Co60 as the generation of gamma-ray source. The cumulative total dose of gamma irradiation was 30, 60, 200, 500, 1000, and 2500 krad (SiO2). To reduce the annealing effect after the completion of the irradiation, its electrical properties and noise characteristics must be tested within 12 h. I–V characteristics and low-frequency noise of the devices are tested by an automatic test system hardware platform, which is developed by our laboratory based virtual instrument.
4.
Results
4.1
Reverse current test results
As is clearly shown in Fig. 1, sample 1 breaks down after 30 krad irradiation. Before irradiation, its reverse current increases from 0 to 8 × 10?8 A. However, after 30 krad irradiation, its reverse current indicates nearly a straight line at 5 × 10?8 A.

class="figure_img" id="Figure1"/>
Download
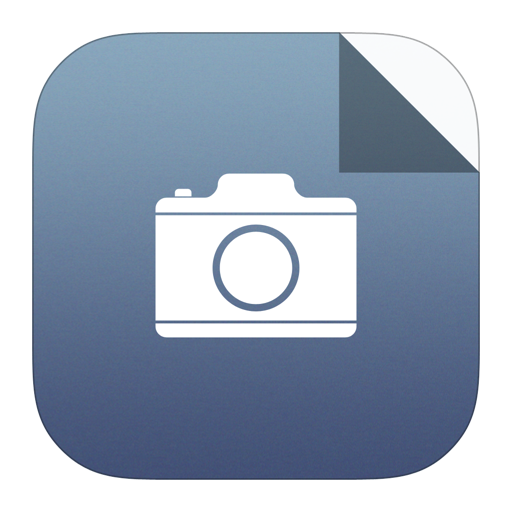
Larger image
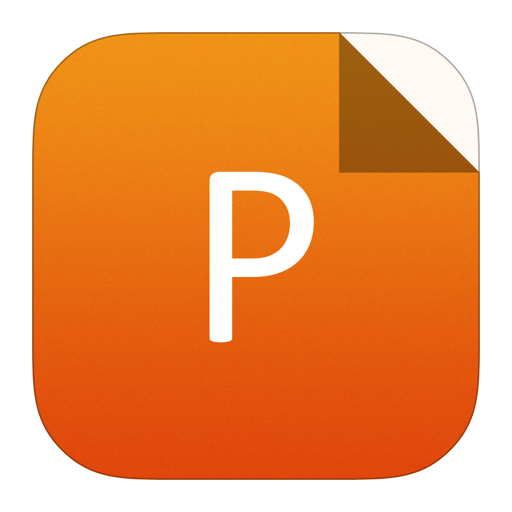
PowerPoint slide
Figure1.
Reverse current of sample1 before and after 30 krad irradiation.
According to Eqs. (1) and (2), before irradiation, the number of device defects is small and the switching threshold is large. Therefore, micro-plasma defects are closed, and the avalanche multiplication factor is small. So, the device is not easy to break down. After irradiation, the number of device defects is large and the switching threshold is small. Therefore, micro-dust defects are opened, and the avalanche multiplication factor is big. So, the device is easy to break down.
The reverse current of sample 2 before and after 2500 krad irradiation is shown in Fig. 2. Before irradiation, its reverse current is increased from ?1.8 × 10?8 A to 0. After 2500 krad irradiation, its reverse current is increased from ?4.4 × 10?8 A to 0. These results indicate that it does not breakdown after 2500 krad irradiation.

class="figure_img" id="Figure2"/>
Download
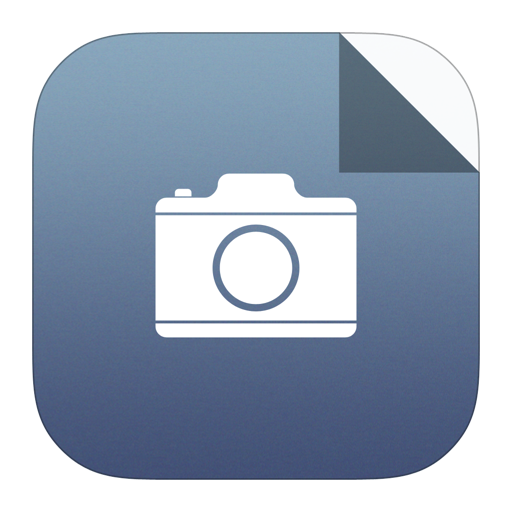
Larger image
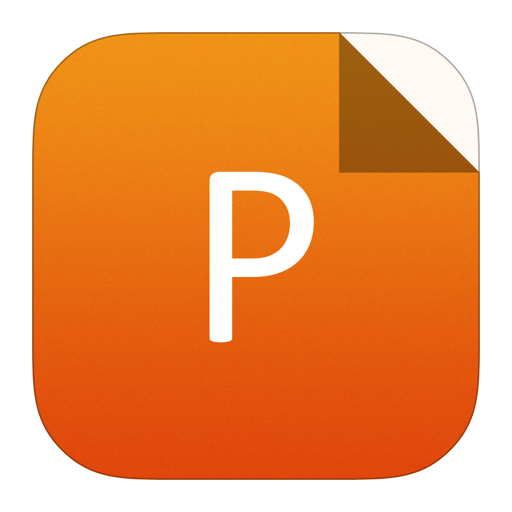
PowerPoint slide
Figure2.
(Color online) Reverse current of sample 2 before and after 2500 krad irradiation.
This is because before irradiation, there are fewer defects in the devices. After high-dose irradiation (2500 krad), even though defects are increasing, there are no changes about the avalanche multiplication factor, therefore, the device does not break down.
4.2
Noise tests results
For sample 1, it breaks down after 30 krad irradiation because of micro-plasma defects. According to the micro-plasma theory[15], micro-plasma has a spot conductivity in the depleted region of the reverse biased PN junction. micro-plasma occurs in a local imperfection. The micro-plasma current is unstable, (see the ellipse circled section in Fig. 3) and the power spectral density of the micro-plasma noise has random switching. Random two-level or multiple-level current impulses occur in electronic devices containing reverse biased P–N junctions in a certain operating mode. These impulses are usually rectangular, featuring constant amplitude, random pulse width and pulse origin time points (see the inserted picture in Fig. 3). This result is similar to the one in Ref. [12].

class="figure_img" id="Figure3"/>
Download
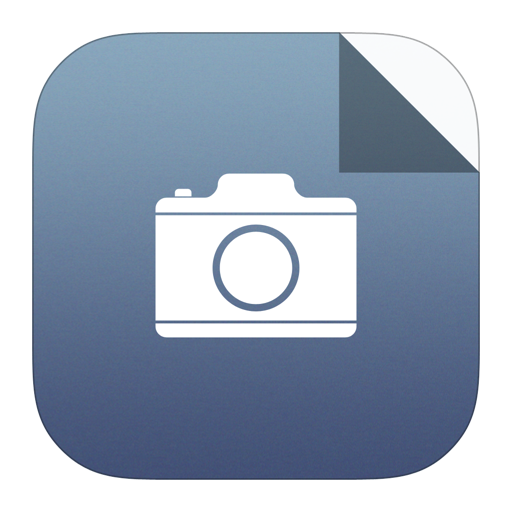
Larger image
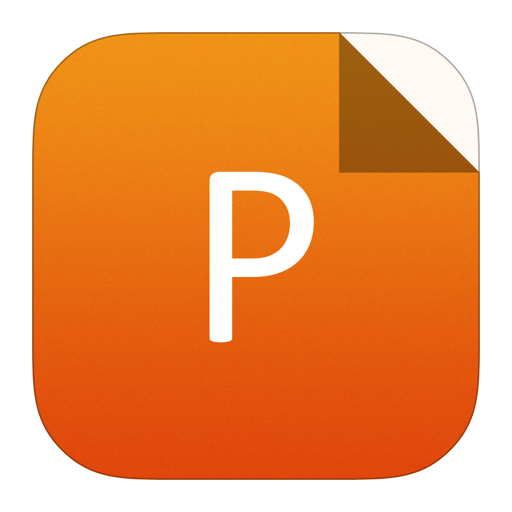
PowerPoint slide
Figure3.
Reverse current characteristics and noise characteristics before irradiation.
5.
Conclusion
Through testing the reverse bias I–V characteristics and low frequency noise of GaN-based blue light-emitting diode (LED) before and after γ irradiation, it is found that after 30 krad γ irradiation, the GaN-based LED is a failure. Reverse soft breakdown region current local instability of this device before irradiation is analyzed by the micro-plasma noise method. The result is obtained that if the GaN-based LEDs contain the micro-plasma defects, they will fail after low doses of γ irradiation. The frequency-domain and time-domain analysis of micro-plasma noise in GaN-based LED before irradiation have been carried out. This result clearly reflects the physical nature, that the micro-plasma defects induce carriers fluctuation noise and the local instability of GaN-based LED reverse bias current.