1.
Introduction
With the increasing demand for energy, and the limited availability of fossil fuels, there is a need for renewable and sustainable energy sources. One of the sources that is always available is the energy of the sun[1]. Excitonic solar cells (SCs), such as dye-sensitized (DSSC) and organic solar cells appear to have good potential as a cost effective alternative to inorganic solar cells[2]. Nanostructured ZnO has received a great deal of attention during the last few years, due to its unique combination of optical and electrical properties[3]. Among nanostructured materials, ZnO nanowire is one of the most important nanomaterials because it has a large exciton binding energy of 60 meV and at room temperature, it has a direct band gap of 3.37 eV[4]. ZnO is commercially available with advantages such as it is a comparatively non-toxic material, environment-friendly, low cost, easy wet chemical processes, high thermal and chemical stability and high resistance to radiation damage[5].
The application of ZnO in organic, dye sensitized and hybrid solar cells, has been rising over the last few years due to its similarities to TiO2, which is the most studied semiconductor oxide. ZnO shows comparable conduction band position, band gap values and high electron mobility. It can be grown in different nanoforms based on the preparation techniques[6–10]. Particularly, the application of ZnO nanowires, especially well aligned wires, will increase the total surface area of ZnO material compared to ZnO thin film, nanorods and most of the other nanoforms, and consequently will help in improving the contact between the donor and acceptor material in organic solar cells (OSCs), or improve electron injection in dye sensitized solar cells (DSSCs)[11].
Many synthetic methodologies have been employed to achieve ZnO nanowires, including ball-milling[12], chemical bath deposition[13, 14], physical vapor deposition (PVD)[5], hydrothermal process or two step method[15–17], electrochemical deposition[18], sonochemical[19], pulsed-laser deposition[20], vapor phase deposition[21], radio frequency magnetron sputtering[22], sol–gel[23], spray pyrolysis[24], microwave irradiation[25] and chemical vapor deposition[26, 27]. ZnO nanostructures such as nanowires have been extensively studied for many applications including photo-detectors[28, 29], solar cells[30–38], sensors[39–40] light-emitting diodes[41] and photo catalysis[42–44].
In this work, we have synthesized ZnO NWs using a cost effective, simple and rapid low-temperature two-step solution method at different immersing time onto ITO-coated glass substrate. The structure, morphological and optical properties of the ZnO NWs prepared at different immersing times have been characterized. MEH-PPV polymer as an active layer was spin-coated on the ZnO nanowires to constitute the structure of three MEH-PPV/ZnO solar cell samples based on different immersing times. Current density–voltage (I–V) curves of these cells were measured and their photovoltaic parameters were calculated.
2.
Experimental
ZnO nanowire layers were prepared by using the two-step chemical solution method. Firstly, 0.4 M zinc acetate dihydrate was dissolved in isopropanol where an equal molar amount of diethanolamine was added slowly to the solution. The resultant solution was stirred at 60 °C for 30 min to yield a clear transparent homogeneous solution. A ZnO seed layer was deposited by the sol–gel spin coating method onto 15 Ω/□ ITO coated glass substrates. At first, ITO glass substrate was pre-cleaned with detergent, ethanol, acetone and finally with distilled water for 10 min in ultrasonic cleaner, and then dried. For ZnO seed layer formation, the sol was dropped onto ITO glass substrate rotating at 2000 rpm using the spin coater for 60 s. The spin-coating deposition was performed at room temperature. The ZnO seed layer was dried at 100 °C for 10 min in order to remove the solvent. This procedure was repeated twice to obtain our seed layer. The completed seed layers were calcined in air atmosphere at 450 °C for 1 h in a tubular furnace. Secondly, ZnO nanowires were grown on the ZnO seeded-substrates by immersing them in a closed teflon container, inside a stainless steel closed autoclave, containing 50 mM zinc nitrate hexahydrate and 50 mM hexamethylenetetramine (HMT) in deionized water at 60 °C. These seeding substrates were suspended inverted in the nucleation solution for 3, 6, and 8 h. Subsequently, the substrate with the ZnO NWs layer was removed after cooling in the autoclave, then rinsed thoroughly with distilled water to remove any residual reactants and dried in air at 300 °C for 1 h.
The ZnO NWs layer crystalline phases were identified by a grazing angled Philips diffractometer model (PW3040), using a Cu Kα radiation source with λ = 1.54178 ? at operating conditions of 40 kV and 40 mA. Diffraction patterns were recorded in the 2θ range from 10° to 80° at a scanning rate of 0.048°/s. The surface morphology was investigated by field emission scanning electron microscopy (FESEM). Optical properties were recorded by a UV–VIS–NIR spectrophotometer Jasco 760. PL properties were measured using a spectrofluorometer, model Jasco FP-6500, with a Xenon-Arc lamp150 watt light source with 266 nm as the excitation source. The deposited MEH-PPV thin film on glass substrate was investigated by UV–VIS–NIR to measure its optical properties.
The poly [2-methoxy-5(2′-ethyl-hexyloxy)-1,4-phenylenevinylene] with molecular weight 40 000–70 000 g/mol (MEH-PPV) (Aldrich), without further purification, was dissolved in the chloroform for 20 mg/mL then stirred for 2 h at 45 °C in a dark environment. For optical measurement for the active layer, 300 μL from the MEH-PPV solution was dropped on the glass substrate and was spun at 1000 rpm for 60 s and annealed at 100 °C for 5 min. For solar cell preparation, also 300 μL from the MEH-PPV solution was dropped on each ZnO NWs/ZnO seeds/ITO glass and was spin-coated at 1000 rpm for 60 s to construct three different cells based on ZnO NWs layers synthesized at different immersing times, and annealed at 100 °C for 5 min. A 12 nm thick MoO3 layer, as the hole transport layer, was deposited by a NANO-VAK thermal evaporator Model NVTH-350 before evaporation of the 100 nm thick aluminum electrode. The I–V characteristics were measured by a solar cell tester, fabricated by photoemission Tech. USA, including the Keithley 2400 source, I–V tracer where its broad band light source is a 150 W Xenon lamp with intensity of incident light 100 mW/cm2.
3.
ZnO NWs layer characterization
3.1
Structure characteristics
XRD patterns of our synthesized ZnO nanowires layers are depicted in Fig. 1. The investigation of these patterns reflects the structure properties of the ZnO NWs which are well indexed to the standard wurtzite structure zinc oxide (JCPDS Card File No. 89-1397[20], indicating that the investigated ZnO nanowires samples have good crystallinity, preferentially oriented along the c-axis (002), which means that the growth of ZnO NWs are oriented perpendicular to the substrate. It is also observed that all XRD patterns look similar irrespective of the variation of immersing time except for the intensities of the peaks, which increase with increasing the immersing time. The intensity of other diffraction peaks is much weaker than that of the (002) peak. No diffraction peaks from metallic Zn or other phases were observed in these samples, which confirm that all the precursors had been completely decomposed after the deposition process in addition to the high purity of the elaborated ZnO nanowires. The crystallite size of the prepared ZnO nanowires layers was calculated by using Scherer’s formula[2]:
$$D = frac{{0.94lambda }}{{beta cos theta }},$$ ![]() | (1) |
where D is the crystallite size, λ is the used X-ray wavelength where λ =1.540 59 ?, β is the broadening of the diffraction peak measured at half maximum (FWHM) in radians and θ is the angle of diffraction. The calculated average crystallite sizes of the (002) peak are 28.98 nm, 37.8 nm and 47.5 nm for 3, 6, and 8 h samples respectively.
3.2
Surface morphology
From FESEM micrographs (Fig. 2), one can observe that the ZnO layer obtained at lower immersing time shows the initiation of ZnO NWs grown with lower density. On the other hand, the nanowires synthesized at a higher immersing time results in dense growth and are close to being vertically aligned with a bent end. Also, it is remarked that the length of the wires increased with increasing the immersing time where the width of the prepared NWs was found in the range of nano scale, which will be useful in organic solar cell application. These results are comparable with the reported work by Mejía-García et al.[45].

class="figure_img" id="Figure2"/>
Download
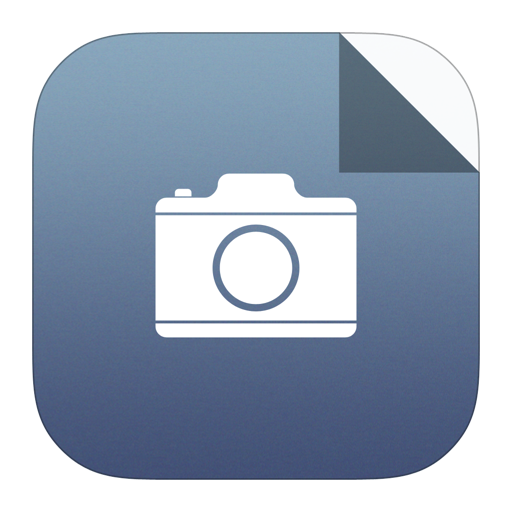
Larger image
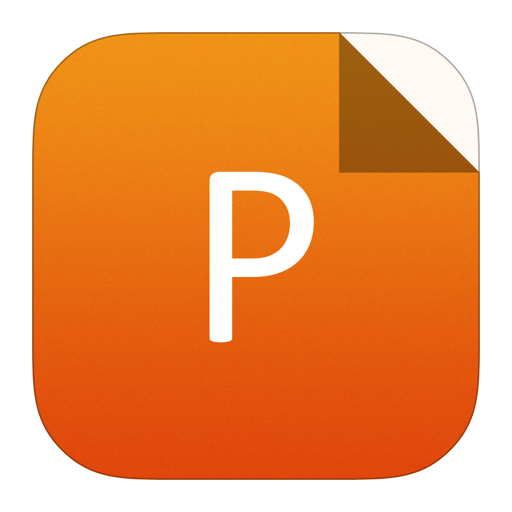
PowerPoint slide
Figure2.
FESEM micrographs of ZnO nanowires prepared at different immersing times.
3.3
Optical properties
Optical transmittance spectra of the ZnO nanowires prepared at different immersing times in the wavelength range of 200–2500 nm are shown in Fig. 3. It is observed that, by increasing the immersing time the transmission in visible and NIR ranges decreased. This behavior can be attributed to the increase in the thickness of ZnO NWs with increasing immersing time, which led to significant blocking of the incident light due to the strong scattering or reflection within ZnO layer or light absorption by the ZnO layer with dimensions comparable to the wavelength of incident light. This result is in agreement with the result obtained by Alaie et al.[46].

class="figure_img" id="Figure3"/>
Download
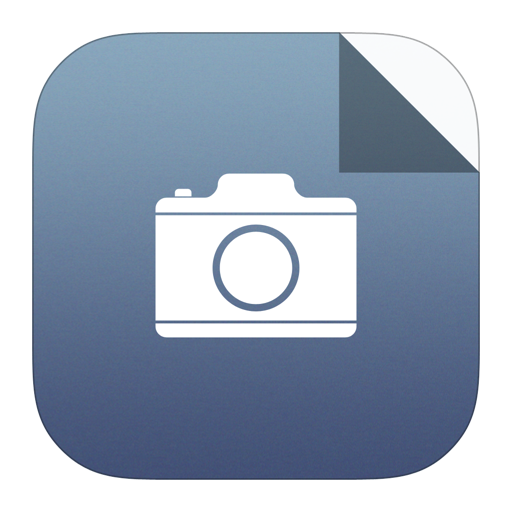
Larger image
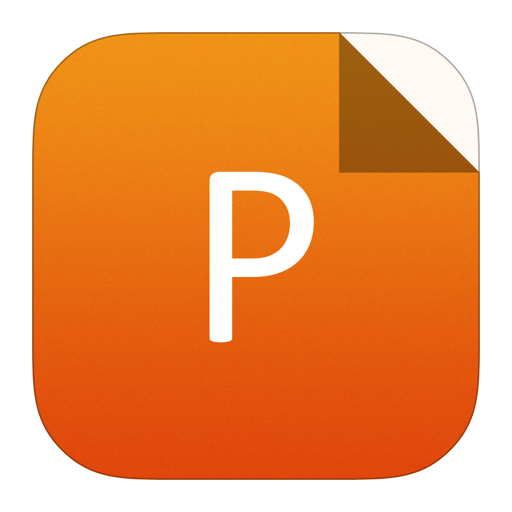
PowerPoint slide
Figure3.
(Color online) Optical transmission of ZnO nanowires prepared at different immersing times.
From the transmission spectra, the absorption coefficient (α) was calculated using the following formula[20]:
$$T = exp [ - alpha (lambda )d],$$ ![]() | (2) |
where T is the optical transmittance and d is thickness of the ZnO NWs layer. By using the absorption coefficient (α), and for the direct transitions, the optical band gaps (Eg) of ZnO NWs layer can be calculated by using the conventional Tauc equation:
$${left( {alpha hnu } ight)^2} = Aleft( {hnu - {E_{ m{g}}}} ight),$$ ![]() | (3) |
where A is a constant, Eg is the direct optical band gap energy and hν is the incident photon energy. Fig. 4 shows the plot of the variation of (αhν)2 versus hν. The energy gap Eg of the samples was estimated from the intercept of the linear portion with hν on the x-axis. The optical band gap of the three samples was found to be 3.2, 3, and 2.9 eV for 3, 6, and 8 h respectively. This decrease in the band gap by increasing the immersing time can be attributed to the enhancement of crystallite size. This behavior can be interpreted with the effective mass model, which can be written approximately as

class="figure_img" id="Figure4"/>
Download
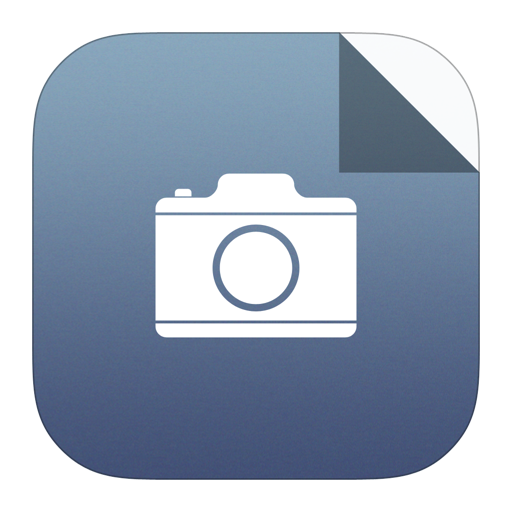
Larger image
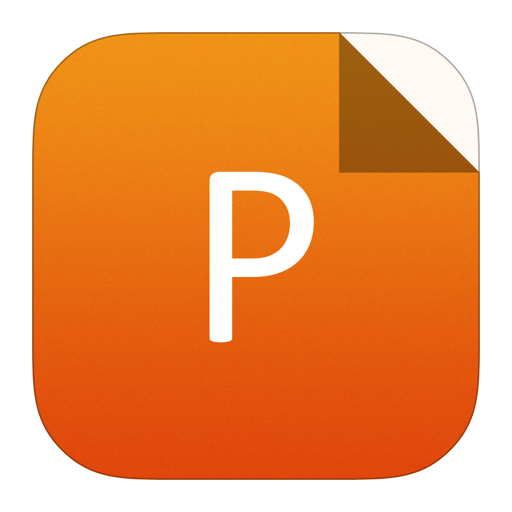
PowerPoint slide
Figure4.
(Color online)
ight)^2$
$${E_{ m{g}}} = {E_{ m{g}}}left( {{ m{bulk}}} ight) + frac{{{hbar ^2}{pi ^2}}}{{2e{r^2}}}left( {frac{1}{{{m_{ m{e}}}}} + frac{1}{{{m_{ m{h}}}}}} ight) - frac{{1.8{e^2}}}{{4pi {varepsilon _{ m{r}}}{varepsilon _0}r}},$$ ![]() | (4) |
where Eg (bulk) is the bulk energy gap, r is the particle radius, me is the electron effective mass, mh is the hole effective mass, εr is the relative permittivity, ε0 is the free space permittivity, ? is Plank's constant divided by 2π, and e is the electron charge. From the previous equation we can see the inverse relation between the crystallite size and the energy gap. Also, it is shown that there is a linear relation between energy band gap and crystallite size, which is in agreement with the reported work of Alaie et al.[46]. The decrease in the band gap of ZnO by increasing crystallite size has also been reported by Sagar et al.[47].
3.4
ZnO NWs layer photoluminescence
The study of photoluminescence is an easy way to evaluate both optical properties and structural defects of ZnO. Normally, ZnO nanostructures can exhibit UV emission and visible emission resulting from the recombination of free excitons and due to some structural defects respectively[4, 48]. Fig. 5 shows the room-temperature photoluminescence spectra of ZnO nanowires growing by the two-step chemical solution method for 3, 6, and 8 h, in which the ultraviolet emission peak is centered at about 375 nm, and another broader emission is situated in the blue–green part of the visible spectrum. This result can be attributed to the degree of crystallization and stoichiometry of the ZnO nanowires. Also one can see that the ultraviolet emission intensity of the nanowires layer increased with increasing the immersing time, which mean the increase in the recombination of free excitons. This may be due to the increasing of the crystal quality of ZnO nanowires layer as confirmed by the XRD shown inFig. 1. However, the intensity of the blue–green emissions becomes weak as immersing time goes on.

class="figure_img" id="Figure5"/>
Download
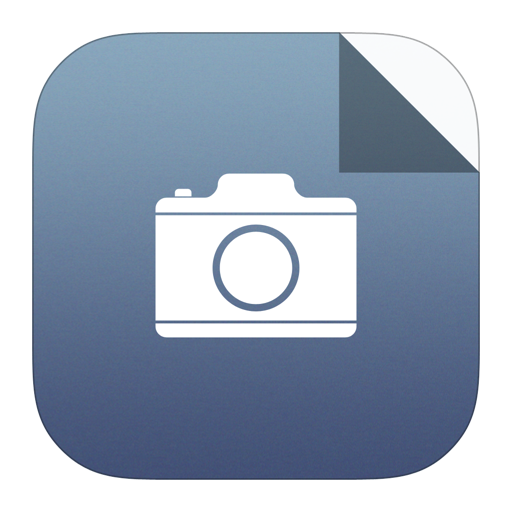
Larger image
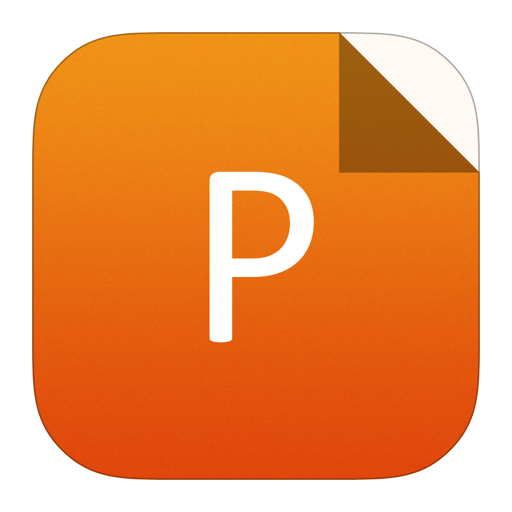
PowerPoint slide
Figure5.
(Color online) Photoluminescence (PL) spectra of the ZnO NWs prepared at different immersing times.

class="figure_img" id="Figure1"/>
Download
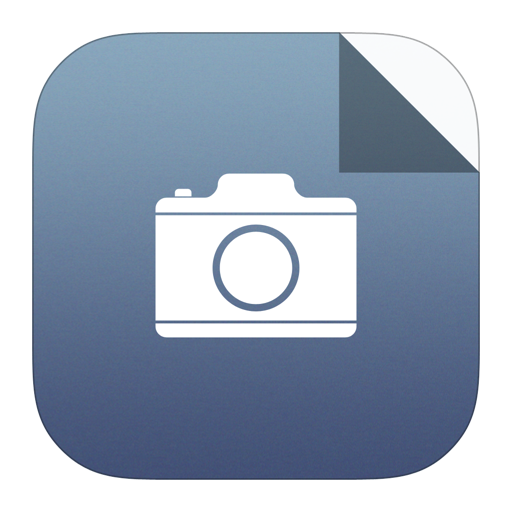
Larger image
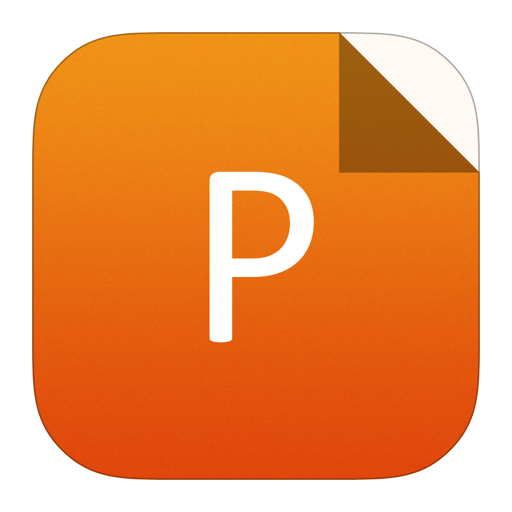
PowerPoint slide
Figure1.
(Color online) XRD of ZnO nanowires prepared at different immersing times.
The visible PL spectra could be deconvoluted with two peaks centered at about 470 and 520 nm that are related to oxygen vacancies as reported by Refs. [42, 49, 50]. This means that the reduction in blue–green PL intensity, by increasing the immersing time, leads to a decrease in the oxygen vacancies, which means decreasing the donor centers in ZnO nanowires and consequently decreasing its carrier concentration.
3.5
Optical properties of MEH-PPV thin film
Fig. 6 shows the spectra of the absorption coefficient for the spin coated MEH-PPV film, deposited on glass substrate, extracted from the transmission of the film using Eq. (2). This figure indicates that, the strong absorption of MEH-PPV extends from 400 to 600 nm, which meets the needs of the solar cell. The maximum absorption coefficient of the MEH-PPV film is 510 nm and can be ascribed to the π–π* transition of the MEH-PPV polymer where the absorption coefficient is in the order of 104 cm?1. The MEH-PPV film optical band gap was calculated by extrapolating the linear portions of (αhν)2 versus hν plot using the relation of Eq. (3) as shown in Fig. 7. The band gap of our MEH-PPV film is about 2.05 eV, which is smaller than the 2.1 eV value reported by Omer and Aziz et al.[48, 51]. The obtained data for both absorption coefficient and band gap of MEH-PPV film confirmed that our polymer is a good candidate for solar cell application.

class="figure_img" id="Figure6"/>
Download
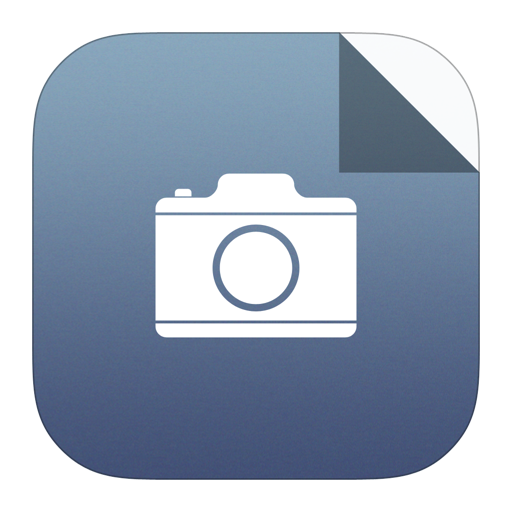
Larger image
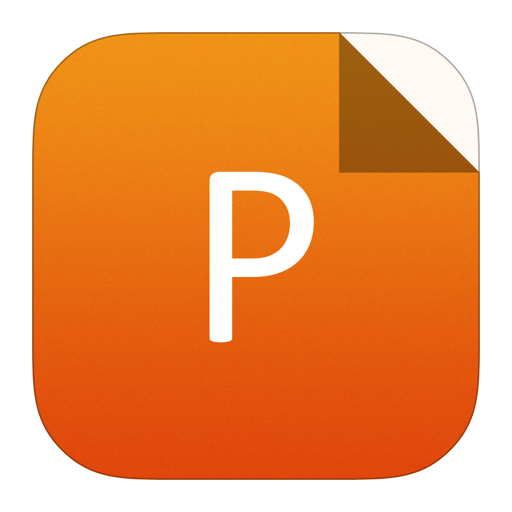
PowerPoint slide
Figure6.
Absorption coefficient of a MEH-PPV thin film prepared on glass substrate as a function of wavelength.

class="figure_img" id="Figure7"/>
Download
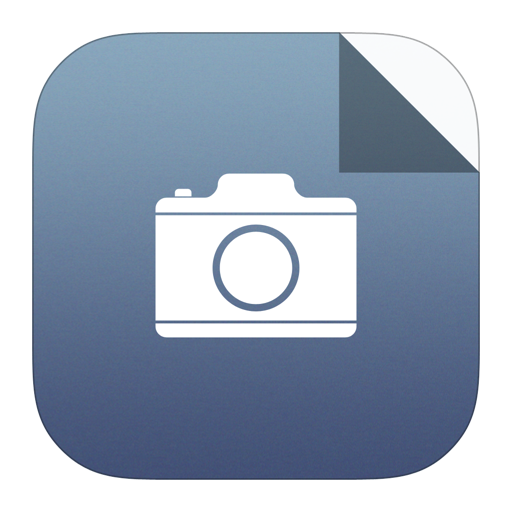
Larger image
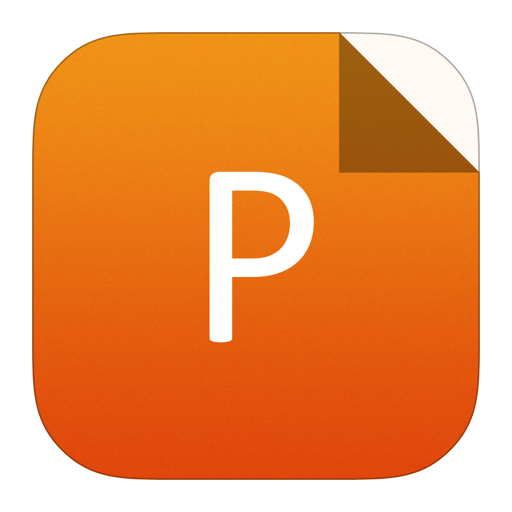
PowerPoint slide
Figure7.
ight)^2 $
3.6
MEH-PPV/ZnO NWs solar cell characterization
Fig. 8 shows the J–V curves of three Al/MoO3/MEH-PPV/ZnO NWs/ZnO seed/ITO glass solar cells based on ZnO nanowires prepared at different immersing times. Shunt resistance (Rsh) and series resistance (RS) can be extracted from the J–V characteristics of Al/MoO3/MEH-PPV/ZnO NWs/ZnO seed/ITO glass solar cells. It has been known that the tangent slope of the vertical part (near Voc) is proportional to the reciprocal of the series resistance (1/Rs) and the tangent slope of the transverse part (near Jsc) is proportional to the reciprocal of the shunt resistance (1/Rsh)[52]. Detailed photovoltaic parameters of the MEH-PPV/ZnO solar cells are extracted from the J–V characteristics; short-circuit current density (Jsc), open circuit voltage (Voc), fill factor (FF), power conversion efficiency (η) and the extracted values of Rs and Rsh are shown in Table 1. It is found that Rs and Rsh increase with increasing immersing time. Voc and η increased with increasing immersing time whereas the Jsc exhibited opposite behavior.
Immersing time | JSC (mA/cm2) | VOC (V) | FF | Rs (Ω) | Rsh (Ω) | η (%) |
3 h | 3.39 | 0.358 | 0.34 | 51.46 | 211 | 0.411 |
6 h | 3.10 | 0.633 | 0.27 | 100 | 235 | 0.523 |
8 h | 2.14 | 0.835 | 0.46 | 166 | 847 | 0.812 |
Table1.
Photovoltaic parameters of Al/MoO3/MEH-PPV/ZnO NWs/ ZnO seed/ITO solar cells sensitized at different immersing times.
Table options
-->

Download as CSV
Immersing time | JSC (mA/cm2) | VOC (V) | FF | Rs (Ω) | Rsh (Ω) | η (%) |
3 h | 3.39 | 0.358 | 0.34 | 51.46 | 211 | 0.411 |
6 h | 3.10 | 0.633 | 0.27 | 100 | 235 | 0.523 |
8 h | 2.14 | 0.835 | 0.46 | 166 | 847 | 0.812 |

class="figure_img" id="Figure8"/>
Download
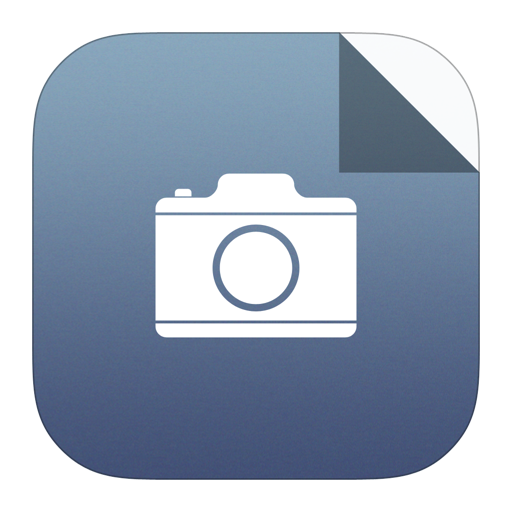
Larger image
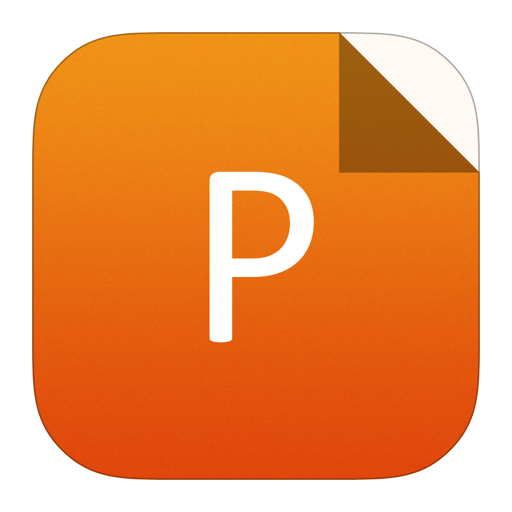
PowerPoint slide
Figure8.
(Color online) J–V curves of MEH-PPV/ZnO solar cells prepared at different immersing times.
The Al/MoO3/MEH-PPV/ZnO NWs/ZnO seed/ITO glass solar cell constructed using ZnO NWs prepared at 8 h showed the best performance where the crystallite size of ZnO is higher than the other samples. This result is in agreement with the reported work by Xue et al. and Hua et al.[53, 54]. Also, by increasing the immersing time, Jsc showed decreasing from 3.388 to 2.14 mA/cm2 and Voc exhibited as increasing and its maximum value of 0.834 V obtained at 8 h. As the immersing time increased, the density of ZnO NWs increased, which leads to preventing MEH-PPV from penetrating through the entire ZnO NWs film, that led to a decrease of the total surface area of ZnO inside the MEH-PPV active layer. This leads to a decrease in the polymer/ZnO contact area, a decrease in the quantity of photons that interact with the active layer that consequently decreased the produced excitons. However, the charge recombination rate also increases with increasing the ZnO NWs layer thickness as confirmed by the UV part of photoluminescence that increased with increasing the immersing time[55] in addition to the effect of the large series resistance of solar cell, resulting in a reduction of JSC. The solar cell synthesized using ZnO NWs layer prepared at 3 h shows low shunt resistance. The low shunt resistance causes power loses in the solar cell by providing an alternate current path for the light generated current. Such a diversion reduces the amount of current flowing through the solar cell junction and reduces Voc and consequently FF of the solar cell. With increasing the immersing time, Voc increases which could be due to increasing of Rsh and consequently suppression of leakage current. With increasing the immersing time the series resistance significantly increases from 51.46 to 100 Ω while Rsh reveals minimal increase from 211 to 235 Ω. This led to reducing the FF from 0.33 to 0.26. At 8 h the higher increase of Rsh to 847 Ω with respect to the increment of Rs results in an increase of the FF to 0.46. The increase in both Voc and FF was great enough to compensate the reduction of Jsc, which in turn improved the overall cell performance[36, 55].
4.
Conclusion
Elaboration and characterization of the ZnO NWs on ITO glass substrate by a two-step solution method was performed. The prepared ZnO nanowires are an obvious hexangular wurtzite structure and are preferentially oriented along the c-axis (002), which means that the growth of ZnO NWs were oriented perpendicular to the substrate. The FESEM micrograph showed that the ZnO NWs, prepared at higher immersing time, are close to being vertically grown and more densely. A maximum power conversion efficiency of 0.812% was achieved for MEH-PPV/ZnO solar cell based on ZnO NWs prepared at the higher immersing time. The improved efficiency is attributed to the increase of Rsh and consequently enhancement of the open-circuit voltage.