1.
Introduction
The GaN-based high-electron-mobility transistor (HEMT) is one of the promising candidates for next generation high-frequency and high-power electronic devices, and excellent successes in the improvement of GaN materials and GaN-based devices have been achieved in the past few years[1]. However, some tremendous obstacles must be overcome to improve the performance of these devices further, such as the radio frequency (RF) drain current collapse and other reliability problems[2, 3]. The parasitic effects are considered to be mainly caused by the trapping centers due to the defects or impurities in the device materials, and a lot of energy and efforts have been devoted into the trapping phenomena research[4–17]. By carrying out the turn-on pulse transient tests or simulation, the surface, interface or bulk traps have been investigated, respectively[3–10].
Although the surface- and buffer-related current collapse in GaN HEMT could be distinguished by pulsed I?V measurements[18], it is difficult to study the behavior and mechanism of each trapping effect separately. Transient simulation is a very useful method for the identification of traps in GaN-based HEMTs. Previously, it is reported that the surface- and buffer-induced current collapses in GaN HEMTs show different temperature dependences[19]. Therefore, it is necessary to study the mechanisms of each single-type trapping effect and the coeffect of surface- and bulk-trapping behaviors.
In this paper, the coeffect of surface- and bulk-trapping behaviors on the performance of AlGaN/GaN HEMTs is investigated based on the two-dimensional transient simulation and the mechanism of trapping effects is analyzed from the aspect of device physics. First, the simulation model is calibrated. Second, the gate-lag transient current of AlGaN/GaN HEMT is obtained and the corresponding current collapse is extracted as a function of gate length (Lg). For comparison, two models with different types of traps are used in the simulation. The results show that small-scale devices suffer from larger current collapse due to a stronger electric field. Additionally, it is found that the final steady-state current decreases as expected when both the surface and bulk traps are taken into account in the simulation model. However, the total current collapse is dramatically reduced (e.g. from 18% to 4% for the 90 nm gate-length device). The mitigated current collapse is mainly due to the coeffect of surface- and bulk-trapping behaviors, which is consistent with the experimental measured data. It is indicated that the surface-related current collapse of GaN-based HEMTs may be partially eliminated by the bulk trapping effect with a trade-off of the steady-state current.
This paper is arranged as follows. In Section 2, the simulation model is described and calibrated. The simulated and experimental transient results of AlGaN/GaN HEMTs are analyzed and discussed in Section 3, and in Section 4 we conclude the paper.
2.
Device structure and model
The schematic cross-section structure of the device used in this work is shown in Fig. 1(a). The simulation model was calibrated by comparing with the measured direct current (DC) data (gate length Lg = 0.125 μm), and the comparison results are illustrated in Fig. 1(b). It can be seen that the simulated results agree with the experimental data very well.

class="figure_img" id="Figure1"/>
Download
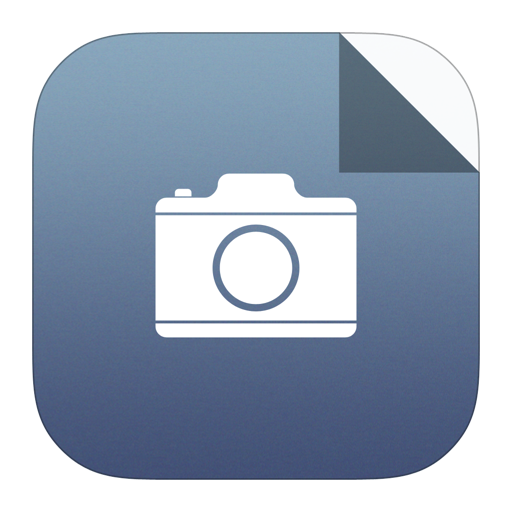
Larger image
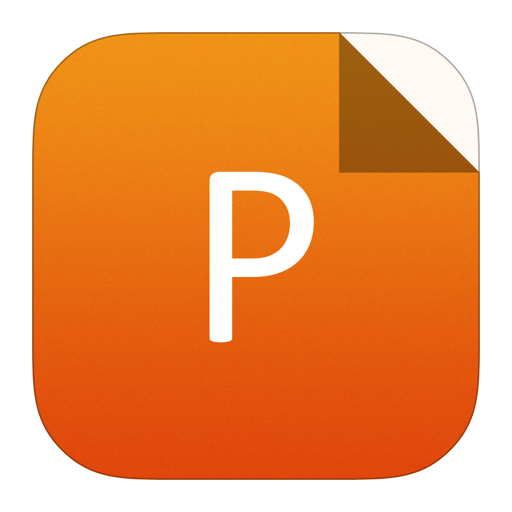
PowerPoint slide
Figure1.
(Color online) Calibration of the simulation model: (a) schematic cross-section structure of the device, (b) comparison results with the measured data.
To study the coeffect of surface- and bulk-trapping behaviors on the performance of GaN-based devices, the gate-lag pulse transient simulations for AlGaN/GaN HEMTs with various gate lengths were conducted. The thickness of Al0.3Ga0.7N barrier layer and GaN buffer layer are 16 nm and 2 μm, respectively. A 2 nm-thick GaN cap layer is also adopted in this work. The Schottky gate is in the middle of the source–drain spacing with the gate–drain distanceLgd = 1 μm. Furthermore, the SiC substrate is eliminated for computational efficiency. Unless specified otherwise, the parameters for the transient simulation model are defined as follows and default values are used for other unspecified parameters. The Schottky barrier of the gate contact is set to be 0.9 eV. Considering the role of hot electrons in the bulk trapping[8], the hydrodynamic transport equations are adopted instead of the drift–diffusion model. The thermodynamic model is included for the thermal effect with a thermal contact at the bottom of the device structure, where the ambient temperature is set to be 300 K. Moreover, the electron mobility degradation model (i.e. high field saturation and doping dependence) and recombination mechanism (i.e. Shockley-Read-Hall, Auger and Radiative) are considered. Following the work in Refs. [5, 20, 21], the polarization effect is equivalent to the effect of fixed positive charges at the AlGaN barrier/GaN buffer interface and negative charges at the GaN cap layer/AlGaN barrier interface with a concentration of 1.13 × 1013 cm?2, respectively. According to the work in Refs. [5, 7, 8, 16], in our simulation, the surface donor traps are supposed to be at the energy level Ed = 0.8 eV and bulk acceptor traps at the energy level Ea = 0.5 eV below the conduction band (Ec). The concentration of surface donor traps located in the interface of passivation/GaN cap layer is Nd = 1.13 × 1013 cm?2, while the bulk traps uniformly distributed in the GaN buffer have a concentration of Na = 1.2 × 1016 cm?3. It is assumed that the electron and hole capture cross sections of the traps are σn, p = 1 × 10?15 cm2.
3.
Results and discussion
In this section, the simulated and experimental transient results of AlGaN/GaN HEMTs with various gate lengths are analyzed and discussed in detail.
First, the simulated gate-lag transient currents of 90 nm gate-length AlGaN/GaN HEMT are plotted in Fig. 2, where a transient voltage applied to the gate terminal is stepped from ?8 V (pinch-off situation for trap filling) to 0 with the drain bias fixed at Vds = 10 V. For comparison, two different models are used in the simulation (numbered as case A and case B). Case A is that AlGaN/GaN HEMT is simulated with only the surface traps considered in the model, while case B is with both the surface traps and bulk traps in the buffer taken into account. As shown in Fig. 2, the two current curves correspond to case A (diamonds) and case B (triangles), respectively. For case A, one rising current trend induced by the surface donor trapping effect is observed after the device is turned on at 1 ms, i.e. the transient current rises with the increasing time until achieving the steady-state current, showing gate-lag current collapse. On the other hand, there are two rising current trends (numbered as region I and region II) for case B.

class="figure_img" id="Figure2"/>
Download
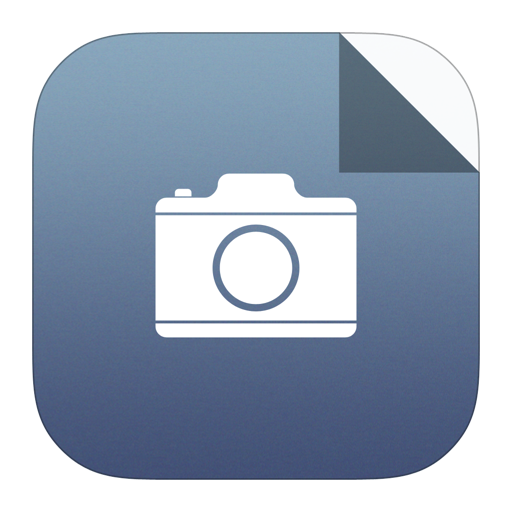
Larger image
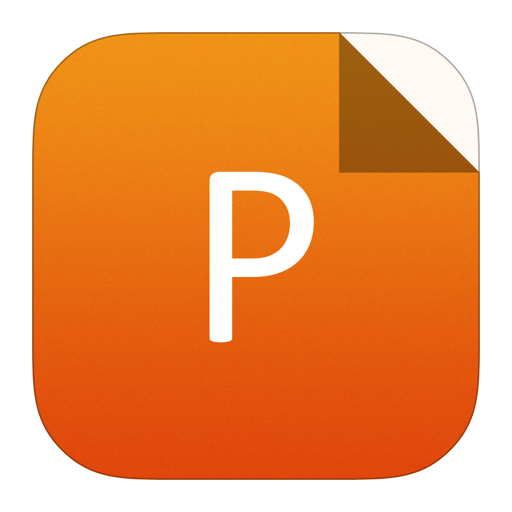
PowerPoint slide
Figure2.
(Color online) The simulated transient currents of AlGaN/GaN HEMT (Lg = 90 nm) with different models.
For clarity, the transient drain currents in Fig. 2 are normalized to the final steady-state current, as shown in Fig. 3, where the measured data from Ref. [19] is also plotted in the inset. With the consideration of device dimensions and operating conditions as well as other non-ideal factors, the accordance of values between the experimental and transient simulation data has not been pursued on purpose. The transient simulation result for case B reproduced the experimental data in Ref. [19] with similar current variation trends. The transient currents can be divided into two regions (I and II), corresponding to the bulk- and surface-trapping effect, respectively. In region I the increased current is induced by the bulk trapping effect in the buffer layer, which has a short time constant. The rising current in region II results from the surface trapping effect with a long time constant.
It can also be noted that the final steady-state current of case B is smaller than case A as expected (see Fig. 2). However, the current collapse (here, the current collapse is defined as the difference between the final steady-state current and the initial current) of case B is dramatically reduced compared with case A (see Fig. 3), which is contrary to the expectation. This is because the current collapse is compensated by the coeffect of surface and bulk traps, and the phenomenon exhibits more obviously as the temperature increases. As is known, the current collapse caused by the surface trapping effect can be explained by the theory of virtual gate[3]. However, if the bulk traps are considered, the bulk acceptor traps will capture electrons when Vgs < Vth (Vth denotes the threshold voltage) and emit the captured electrons when Vgs > Vth. The emitted electrons by the bulk traps can help the transient current recover to the steady state current faster than that of case A, resulting in smaller current collapse. It is suggested that the bulk-trapping effect may not be a bad thing in a certain situation. On the contrary, the surface-related current collapse may be partially eliminated by the bulk trapping effect with optimized design of buffer layers, especially for high temperature operating situation.

class="figure_img" id="Figure3"/>
Download
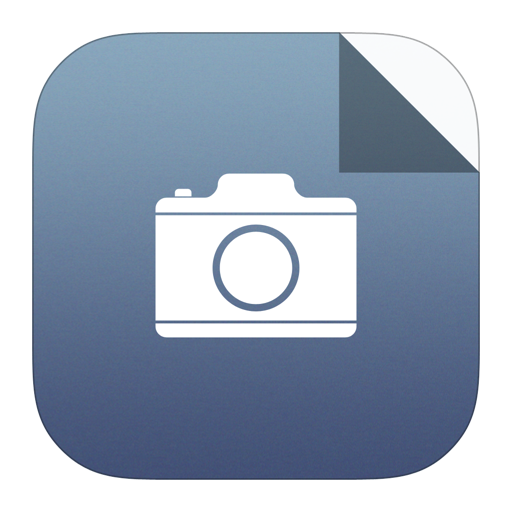
Larger image
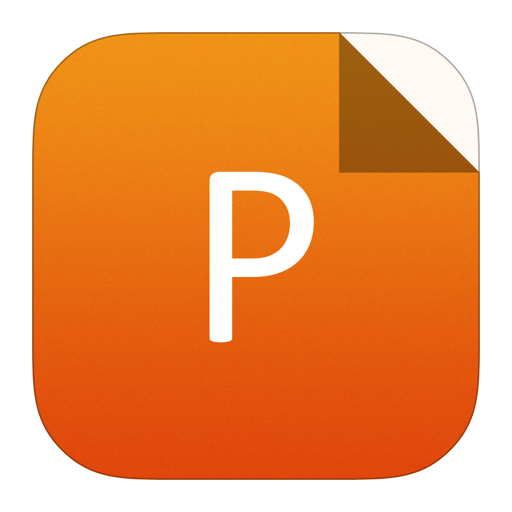
PowerPoint slide
Figure3.
(Color online) The normalized transient currents of AlGaN/GaN HEMT (Lg = 90 nm) with different models, where the measured data from Ref. [19] is plotted in the inset.
In addition, the transient currents of AlGaN/GaN HEMTs with various gate lengths are shown in Fig. 4 to study the dependence of current collapse on the device dimension. Fig. 4(a) and Fig. 4(b) are the simulation results of the two cases with different models, respectively. With the gate length decreasing, the drain current increases, which is expected with the scaling rule. However, the current collapse increases simultaneously with the gate length decreasing, which is unexpected for the device design. This is because the electric field in the small-scale device is stronger, enhancing the trapping effect and resulting in larger current collapse. This means that the trapping effects impact the short-gate device more severely. Moreover, compared with the results of case A in Fig. 4(a) where only surface traps are taken into account in the model, we can see that the current collapses of case B are much smaller [see Fig. 4(b)].

class="figure_img" id="Figure4"/>
Download
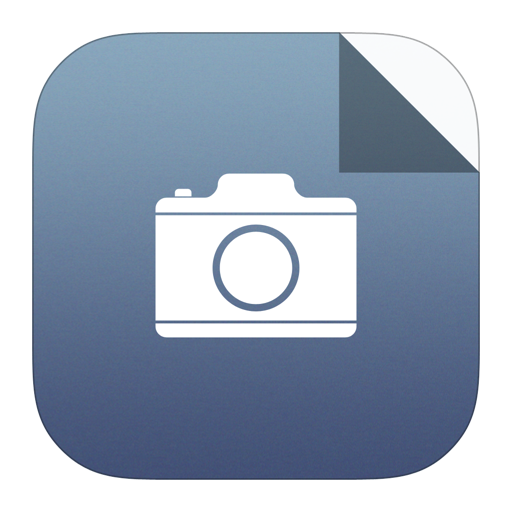
Larger image
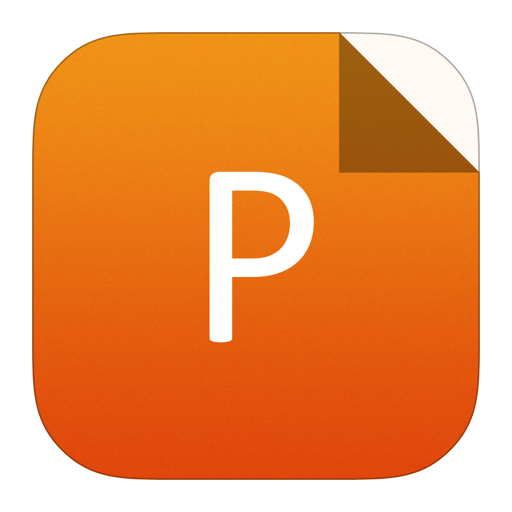
PowerPoint slide
Figure4.
(Color online) The normalized transient currents of AlGaN/ GaN HEMTs with various gate lengths. (a) Case A. (b) Case B.
With the aim to explain the effect of device dimension on the current collapse, the dynamic concentration of trapped holes (corresponding to the surface donor trapping effect) at the interface of the passivation/GaN cap layer is given in Fig. 5, where case A is taken as an example. The trapped holes denote the holes captured by the donor traps, which can be equivalent to the emitted electrons by the surface donors during the detrapping process[3]. Axis x is along the channel direction with zero point at the drain-side gate edge. Two device structures with different gate lengths are compared. As can be seen from Fig. 5, the concentration of trapped holes (emitted electrons) increases with the increasing time t after the device is turned on, which consequently results in the rising trend of the transient current. However, the variation of trapped holes in the short gate device (Lg = 90 nm) from t = 2 ms to t = 0.1 s is larger than that in the long gate device (Lg = 300 nm), leading to a relatively severe current collapse.

class="figure_img" id="Figure5"/>
Download
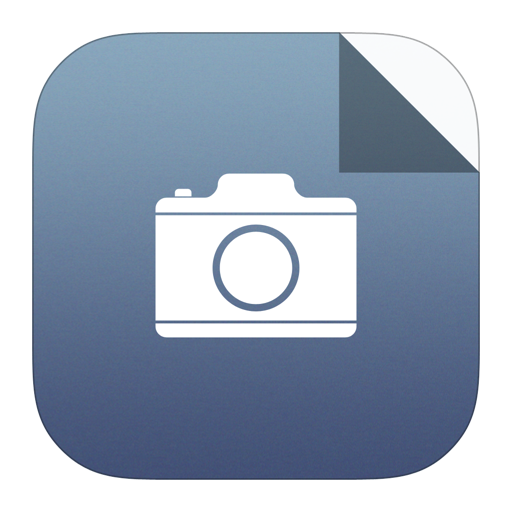
Larger image
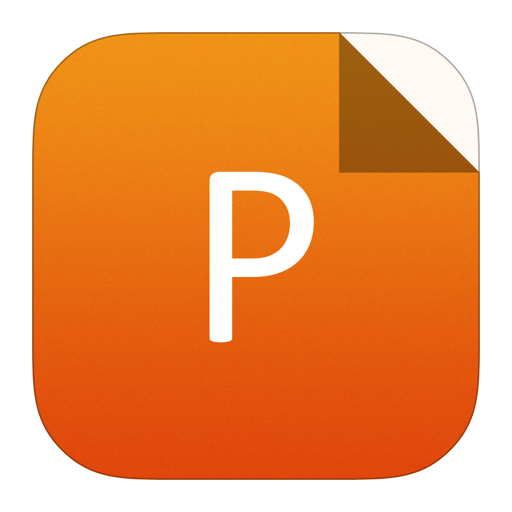
PowerPoint slide
Figure5.
(Color online) The dynamic concentration of holes captured by the surface donor traps in AlGaN/GaN HEMTs with different gate lengths.
Furthermore, the total current collapses of cases A and B are extracted as a function of gate length Lg, as illustrated in Fig. 6. Compared with case A considering only the surface traps in the model, the total current collapse is dramatically reduced for case B (e.g. from 18% to 4% for the 90 nm gate-length device), the mechanism of which will be discussed in the following.

class="figure_img" id="Figure6"/>
Download
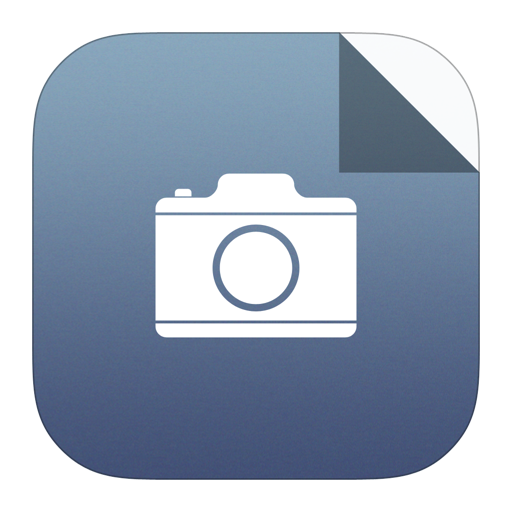
Larger image
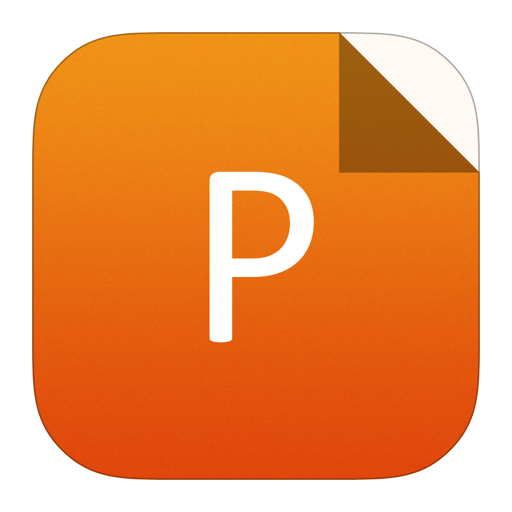
PowerPoint slide
Figure6.
(Color online) Comparison of the total current collapse between case A and case B.
The reduced current collapse of case B can be explained with the coeffect of the two types of trapping behaviors, and the mechanism of trapping effects is analyzed from the aspect of device physics in detail. The schematic band diagram of AlGaN/GaN HEMT with different models is shown in Fig. 7. For case A with only surface traps considered [Ed = 0.8 eV, see Figs. 7(a) and 7(b)], some of the electrons are captured by the surface traps during the trapping process in the pinch-off state, as shown in Fig. 7(a). When the device is turned on, the electron density in the 2DEG channel would be reduced, which induces a large surface trap-related current collapse. As the time increases, the captured electrons are emitted very slowly during the detrapping process in the on-state [see Fig. 7(b)], leading to a rising transient current. However, for case B with both the surface and bulk traps taken into account [Ed = 0.8 eV, Ea = 0.5 eV, see Figs. 7(c) and 7(d)], some of the electrons in the channel are captured by both the surface and bulk traps during the trapping process, as shown in Fig. 7(c). Since the bulk traps have a shorter time constant, the captured electrons are emitted quickly and go back to the 2DEG channel after the device is switched on [see Fig. 7(d)]. Therefore, the surface-related current collapse is mitigated in some degree due to the participation of bulk traps with short time constant.

class="figure_img" id="Figure7"/>
Download
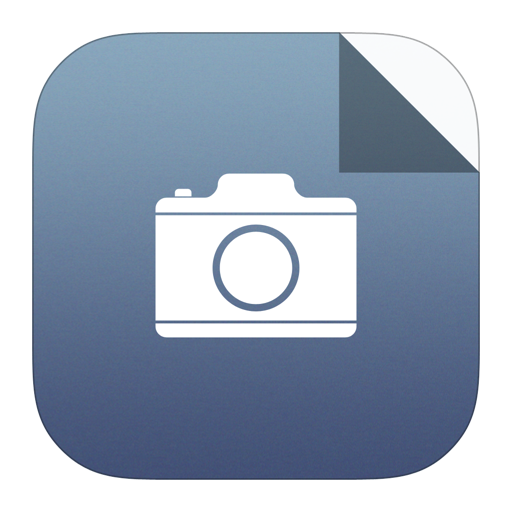
Larger image
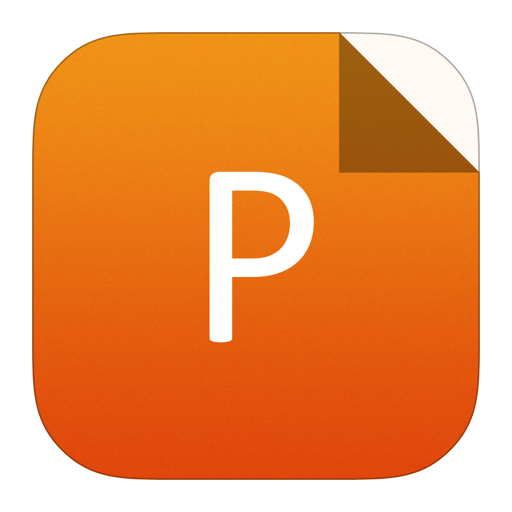
PowerPoint slide
Figure7.
(Color online) Schematic band diagram of AlGaN/GaN HEMT with different models: (a) during the trapping process in the pinch-off state and (b) during the detrapping process in the on-state for case A, (c) during the trapping process in the pinch-off state and (d) during the detrapping process in the on-state for case B.
4.
Conclusion
Based on the two-dimensional transient simulation with the calibrated model, the coeffect of surface- and bulk-trapping behaviors on the performance of GaN-based HEMTs is analyzed in this paper. It is found that the final steady-state current decreases when both the surface and bulk traps are taken into account in the model. Contrary to the expectation, the total current collapse is dramatically reduced due to the coeffect of surface- and bulk-trapping behaviors, which is explained from the aspect of device physics. The results indicate that the surface-related current collapse of GaN-based HEMTs can be partially eliminated by the participation of bulk traps with an optimized design of buffer layer.