1.
Introduction
The organic dye-based photovoltaic devices have received increasing attention over the last few decades. Compared to inorganic conventional photovoltaic materials, these organic photovoltaic devices are gaining importance due to their light weight, flexibility, availability of ingredients and smooth fabrication procedure[1–6]. Though the performance of such devices is developing steadily, these devices are still facing some limitation in improving the electronic properties. Earlier works have reported[7–9] that the addition of single walled carbon nanotubes (SWCNTs) in organic dye-based photovoltaic devices improves its photovoltaic properties like open circuit voltage, short circuit current, fill factor and device efficiency. However, its effect on the series resistance (Rs) is not well studied, and that remains a vexing unanswered question. It is also well known that the dark current–voltage (I–V) characteristic of any diode shows linearity in the semi logarithm scale at low voltage. However, the characteristic deviates from the linearity with increasing bias voltage. The presence of Rs has been recognized as one of the basic reasons behind these phenomena. As organic devices are disordered in nature, carriers acquiring high velocities collided with each other at high voltage and the tendency of recombination or trapping at the trap centers get increased[10, 11]. These trapping states contribute an internal resistive property of organic substances. Moreover, interfaces between the active layer blend and inter-layers or metallic contacts results in partial energy alignment, which impacts on the optimal charge transfer into the bulk region of the organic dye. The mismatch of energy level alignment leads to adding more resistance in series. Finally charge carrier transport within the active layer itself could also be a source for increasing series resistance. High value of series resistance limits the conductivity of the device. It was described in many works[8, 9] that the value of series resistance of any organic diode is usually very high compared to inorganic diodes. It is needless to mention that the performance of an organic photovoltaic struggles by this high series resistive impact. Attention should be given to minimize the value of Rs because the efficiency of the photovoltaic strongly depends on this parameter. Unfortunately not much work is available to show the possibilities of lowering Rs in an organic semiconductor based photovoltaic. So observations of this work will be very helpful to realize the contribution of SWCNT, which has an influence on Rs. In the present work we will report the effect of SWCNT on Rs of Rose Bengal (RB) and Methyl Red (MR) dye-based photovoltaic devices. A thin layer of organic dye is sandwiched between two electrodes. The device follows the mechanism of the Schottky diode. The Schottky effect is followed by the conducting carriers in the device across the metal–semiconductor interface. This report will be fruitful for physical understanding and practical implementations of CNTs on organic diodes.
2.
Materials
Fig. 1(a) shows the structural representation of RB which was procured from Loba Chemie Private Ltd, India. RB is a poly-halogenated tetra cyclic carboxylic acid dye[9]. RB is used basically for its excellent photosensitive property. The formula of this dye is C20H4Cl4I4O5. The absorption coefficient of the dye is high enough in the visible spectra range. Fig. 1(b) represents the diagram of MR, which was procured from Finar Chemicals, India. The molecular formula of MR is NC6COOH. The delocalized electrons of its atoms is the reason for the absorption of spectra in the visible range of frequency, which generates the color of the dye. The mentioned dyes have been selected for experiment because these dyes show convincing optoelectronic sensation. Fig. 1(c) is the representation of SWCNT, which was procured from SRL, India. ITO coated glass is used as the front electrode whereas aluminum coated Mylar (Al-M) is used as the counter electrode of the device.

class="figure_img" id="Figure1"/>
Download
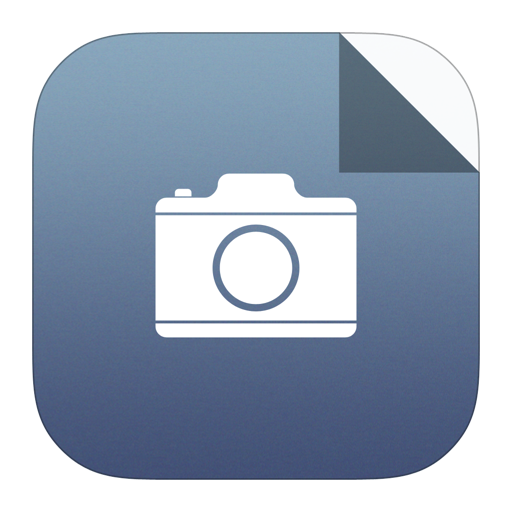
Larger image
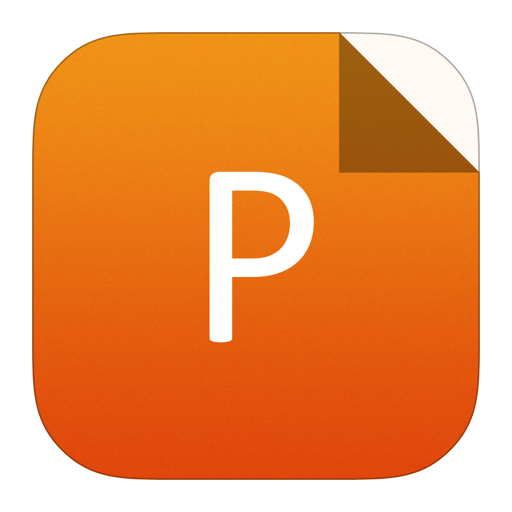
PowerPoint slide
Figure1.
(Color online) Structural diagram of (a) Rose Bengal (RB), (b) Methyl Red (MR), and (c) single walled carbon nanotube (SWCNT).
3.
Sample preparation and experiments
In a pre-cleaned test tube, 1 mg PVA is mixed with 10 mL of distilled water. PVA works as an adhesive organic gum. The PVA–water mixture is stirred for 30 min in a magnetic stirrer for the formation of a homogeneous PVA solution. Then 1 mg RB dye is mixed with this solution and again left to stir gently for another 0.5 h to get the RB dye solution. Then the prepared solution is taken in two different test tubes. Two test tubes are named as test tube A and test tube B. A 1 mg amount of SWCNT is incorporated in RB dye solution of test tube A. The blend of dye component and SWCNT are well stirred again for around 1 h. Now the solution is deposited on the ITO glass plate by a spin coater to coat a layer of RB dye solution of test tube B with a rotation of 1500 rpm and then dried at 3500 rpm. Coating on the Al-M back electrode is processed with the same solution in a similar way. ITO and Al-M electrodes are then sandwiched to form the device when they are in a semi dry state. The same process is followed for the preparation of the device using the solution of test tube A. Devices fabricated with MR dyes with and without incorporating SWCNT are formed similarly by the procedure as mentioned earlier. The prepared devices were vacuumed for 12 h to make them ready for experiments.Fig. 2 expresses a representation of a prepared device.

class="figure_img" id="Figure2"/>
Download
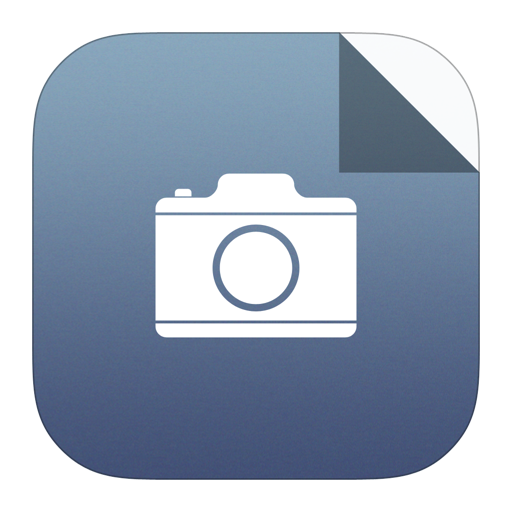
Larger image
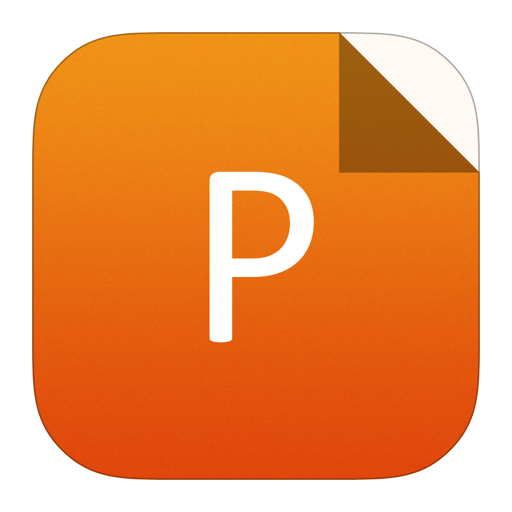
PowerPoint slide
Figure2.
(Color online) Schematic diagram of prepared photovoltaic device. Organic dye layer is sandwiched between ITO coated front electrode and Aluminum coated mylar sheet back electrode.
Fig. 2 shows the schematic diagram of the prepared devices. In order to study Rs, the I–V characteristics have been measured. The device is connected with a DC bias. The front electrode is connected to the positive terminal and the negative terminal of the battery is connected to a counterpart of the device for dark I–V measurement. Bias voltage is applied from 0–6 V in steps with a variation of 0.2 V with 1500 ms delay. The current and voltage are measured with a Keithley 6? digit multimeter, model number 2000. The experiments have been done in a clean open atmosphere in the laboratory at a room temperature of 22 °C.
4.
Results and discussions
The dark I–V characteristics of RB and MR dye-based organic photovoltaic devices (OPV) are shown in Fig. 3. The value of dark current is quite low for the dyes under experiment; however, the current increases almost three times when SWCNTs are incorporated with these dyes.

class="figure_img" id="Figure3"/>
Download
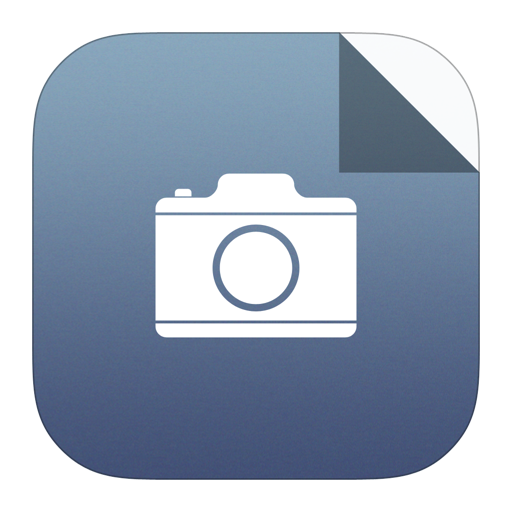
Larger image
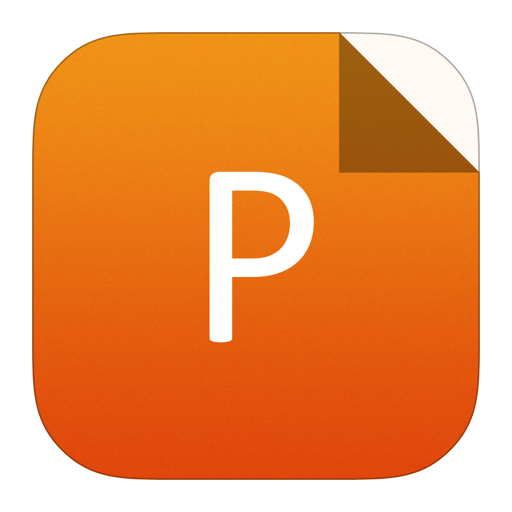
PowerPoint slide
Figure3.
(Color online) Dark I–V characteristics of (a) Rose Bengal (RB) and (b) Methyl Red (MR) dye-based OPV in presence and in absence of SWCNT.
It is observed for all the devices that the current conduction changes after a certain transition voltage. There are several factors that affect the on current conduction process in organic devices. The most crucial factor in our consideration is Rs. The non linear behaviour of these devices occurs due to the existence of Rs because lowering of this parameter gives a comparatively greater range of linearity over which the I–V relation follows a straight line at high voltage.
The I–V plot of an organic diode can be analyzed from the following relation[12–16]
$${I} = {{I}_0}{ m {exp}}left( {frac{{{q}({V} - {I}{{R}_{ m s})}}}{{{nKT}}}} ight),$$ ![]() | (1) |
where I0 is the saturation current given by
$${I_0} = A{A^*}{T^2}exp left( { - frac{{qphi }}{{kT}}} ight),$$ ![]() | (2) |
where A is the contact area, A* is the Richardson constant, T is the temperature in K,

$${{n}} = frac{{{q}}}{{{{kT}}}} {frac{{{ m{d}}V}}{{{ m{d}};{ m{ln}}I}}}. $$ ![]() | (3) |
The value of ideality factor obtained from Eq. (3) is usually very high for organic devices, which is attributed to the high value of Rs. The reason for such high Rs is due to the presence of organic material between ITO/Al electrodes, which causes a change in energy level in the metal–semiconducting interface. Such a change in energy level leads to a non ideal current–voltage behavior of the device. Basically the energy difference of the organic semiconductor and work function of the metal are realigned for interaction between conductor and semiconductor, which in turn changes the electron affinity of the semiconductor at the organic/inorganic semiconductor interface, and this results an increment of barrier height[20]. To realise the role of Rs, an equivalent circuit model of a standard photovoltaic device may be recollected. The circuit model of Rs is depicted in Fig. 4.

class="figure_img" id="Figure4"/>
Download
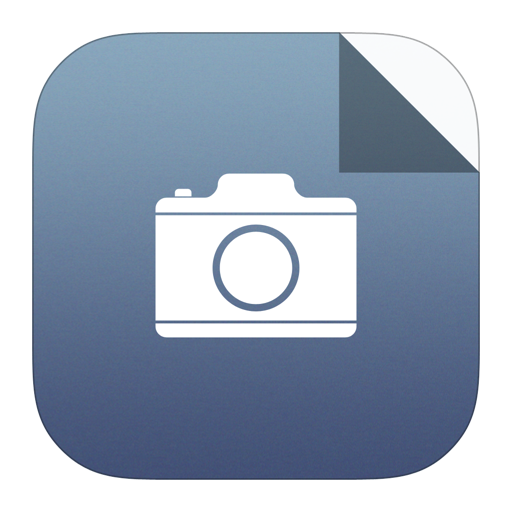
Larger image
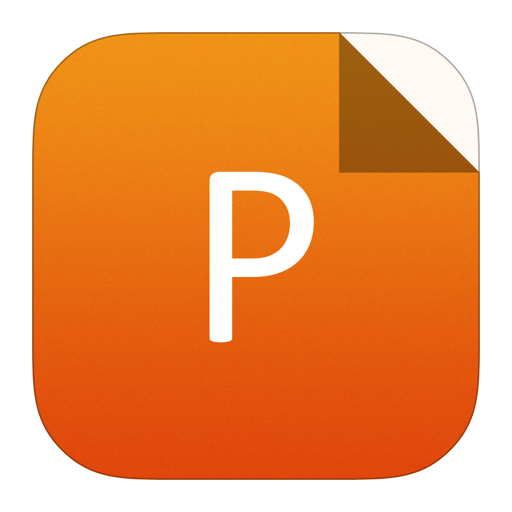
PowerPoint slide
Figure4.
Equivalent circuit model of photovoltaic devices. The output current directly passes through series resistance Rs. A little change in Rs has a strong influence on output.
4.1
Estimation of Rs using I–V characteristics
In order to estimate the series resistance, our first approach deals with I–V characteristics using the formula of Rakhshani et al.[17]. Typically, I–V characteristics for high series resistance of a solar cell can be explained by[17, 23]
$${I} = {{{I}}_{{ m L}}} - {{I}_0}left[ {{{ m e}^{{q}left( {{V} - {I}{{R}_{ m s}}} ight)/{nkT}}} - 1} ight] - frac{{{V} - {I}{{R}_{ m s}}}}{{{{R}_{ m {sh}}}}}.$$ ![]() | (4) |
The corresponding model is shown in Fig. 4. IL denotes photocurrent in the device. Since there is no photocurrent in dark I–V conditions so the value of IL will be zero. The last term of Eq. (4) is neglected for the assumption of a high value of Rsh. So, the equation can be written as[23]
$${I} = {{I}_0}left[ {{{{{ m e}}}^{{q}left( {{V} - {I}{{R}_{ m s}}} ight)/{nkT}}} - 1} ight].$$ ![]() | (5) |
Voltage can be represented in terms of current as
$$V = frac{{nkT}}{q}{ m{ln}}left( {frac{I}{{{I_0}}} + 1} ight) + I{R_{ m{s}}}.$$ ![]() | (6) |
Taking the derivative of both sides with respect to current we get
$$frac{{{ m{d}}V}}{{{ m{d}}I}} = frac{{nkT}}{q}frac{1}{{ {frac{I}{{{I_0}}}} + 1}}{ m{}}frac{1}{{{I_0}}} + {R_{ m{s}}}.$$ ![]() | (7) |
Total current I is much higher than the reverse saturation current I0 in the high current region. So Eq. (7) becomes
$$Ifrac{{{ m{d}}V}}{{{ m{d}}I}} = {R_{ m{s}}}I + { m{}}frac{{nkT}}{q}.$$ ![]() | (8) |
So from this consideration I(dV/dI) is linearly dependent on total current I. Dark I(dV/dI)–I characteristics for all devices are shown in Fig. 5.

class="figure_img" id="Figure5"/>
Download
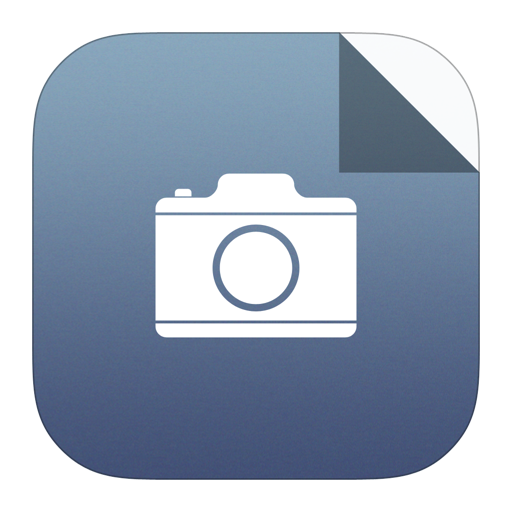
Larger image
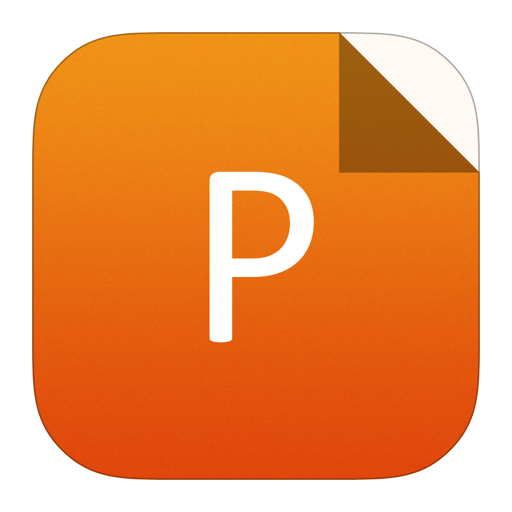
PowerPoint slide
Figure5.
(Color online) I(dV/dI)–I characteristics of (a) RB, (b) MR, (c) RB + SWCNT, and (d) MR + SWCNT.
It can be seen from Fig. 5 that there are two distinct regions in the I(dV/dI)–I plot. In the low current region R-I, there is no considerable change in I with increase in I(dV/dI). Current I follows a linear relationship with I(dV/dI) at high current region R-II. At the highest current region current conduction deviates from linearity, which has been shown by the dotted line of R-II. So linear fitting of the straight line portion of R-II for all dye-based devices are taken into consideration to extract the value of Rs from Eq. (8). Rs can be estimated from the slope of the fitting curve at the high current region. Fitting of I(dV/dI)–I characteristics is shown in Fig. 6.

class="figure_img" id="Figure6"/>
Download
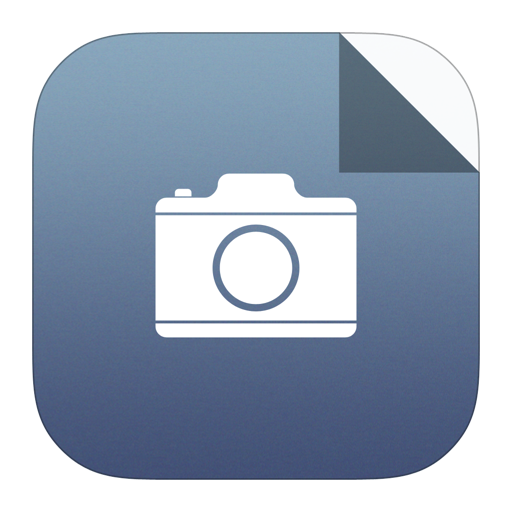
Larger image
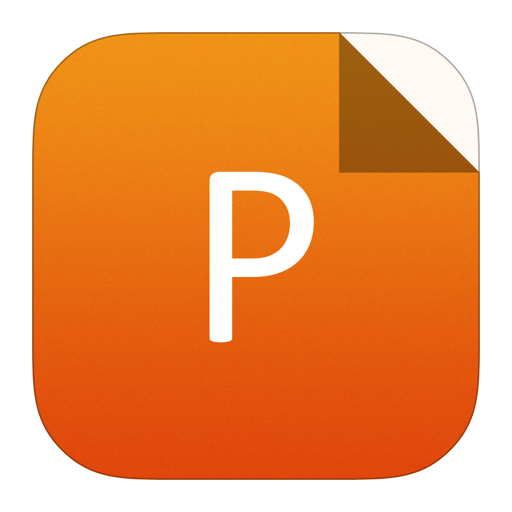
PowerPoint slide
Figure6.
(Color online) Linear fitting of the straight line portion of I(dV/dI)–I characteristics of R-II region of (a) RB, (b) MR, (c) RB + SWCNT, and (d) MR + SWCNT for dye-based OPV.
4.2
Estimation of Rs using Cheung–Cheung method
The corresponding series resistance of the devices at the high current level is also obtained by the method proposed by Cheung and Cheung[18, 19]. In this method, the ideality factor, series resistance, and barrier height of organic diodes can be determined from the following relation
$$frac{{{ m{d}}V}}{{{ m{dln}}I}} = frac{{nkT}}{q} + I{R_{ m{s}}},$$ ![]() | (9) |
$$Hleft( I ight) = V - frac{{nkT}}{q}{ m{ln}}left( {frac{I}{{A{A^*}{T^2}}}} ight),$$ ![]() | (10) |
and
$$Hleft( I ight) = I{R_{ m{s}}} + n phi .$$ ![]() | (11) |
The representation of (dV/dlnI)–I plot at the high voltage region is given in Fig. 7. From the linear region of the (dV/dlnI)–I curve, Rs is extracted, while n is obtained from its interception with the y axis. The estimated value of n is found to be less when the SWCNT has been incorporated in organic dyes. The obtained values of ideality factor for RB and MR dye-based devices are 29.01 and 50.6 respectively. In presence of SWCNT, the values become 24.14 and 41.72 respectively for RB and MR dye-based devices. So a significant reduction is also observed in the ideality factor due to the incorporation of SWCNT.

class="figure_img" id="Figure7"/>
Download
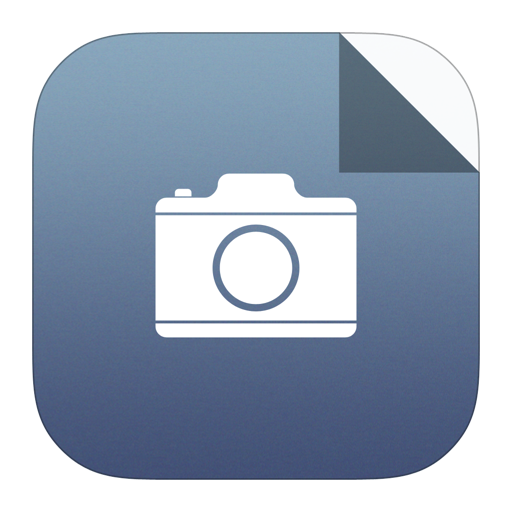
Larger image
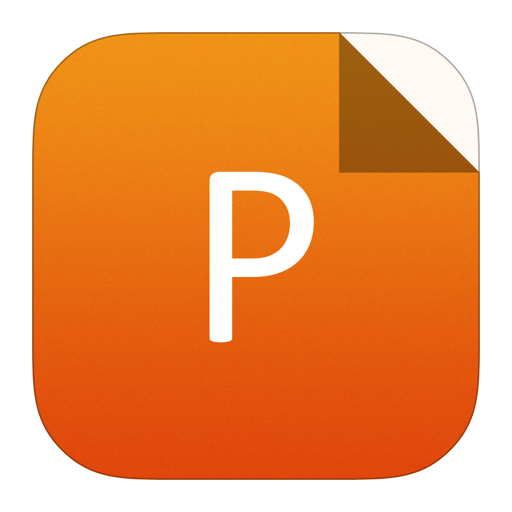
PowerPoint slide
Figure7.
(Color online) (dV/dlnI)–I characteristics of (a) RB, (b) RB + SWCNT, (c) MR, and (d) MR + SWCNT based OPV.
The values of Rs are also obtained from the H(I)–I plot[20–22]. The H(I)–I plot in Fig. 8 shows a straight line with the intercept at the y axis equal to n?. The slope of this plot can be used to verify the accuracy of the Cheung method. The extracted values of Rs are shown in Table 1. As can be seen in the following tables, the estimated values of Rs and its reduction percentage in the presence of SWCNT from the (dV/dlnI)–I and H(I)–I plot shows a really good accuracy with each other.
Dye | I(dV/dI)–I plot | (dV/dlnI)–I plot | H(I)–I plot |
RB | 202 | 239 | 230 |
RB + SWCNT | 48 | 39 | 38 |
MR | 439 | 462 | 453 |
MR + SWCNT | 157 | 152 | 150 |
Table1.
Extracted values of Rs (kΩ) using different methods.
Table options
-->

Download as CSV
Dye | I(dV/dI)–I plot | (dV/dlnI)–I plot | H(I)–I plot |
RB | 202 | 239 | 230 |
RB + SWCNT | 48 | 39 | 38 |
MR | 439 | 462 | 453 |
MR + SWCNT | 157 | 152 | 150 |

class="figure_img" id="Figure8"/>
Download
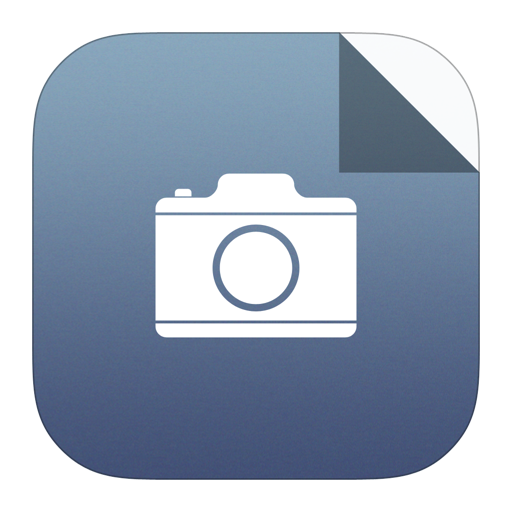
Larger image
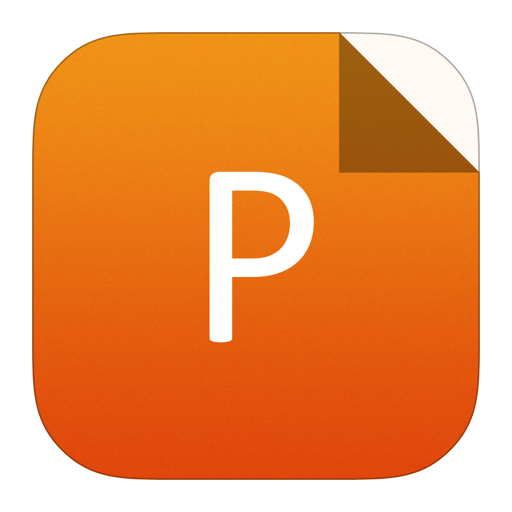
PowerPoint slide
Figure8.
H(I)–I characteristics of (a) RB, (b) RB + SWCNT, (c) MR, and (d) MR + SWCNT based OPV.
From the above table it is observed that Rs is reduced with incorporation of SWCNT in the devices. The reduction percentage of Rs is in Table 2.
Dyes underconsideration | I(dV/dI)–I plot | (dV/dlnI)–I plot (Cheung method) | H(I)–I plot |
For RB (with SWCNT) | 76.08 | 83.56 | 83.01 |
For MR (with SWCNT) | 64.23 | 67.10 | 66.89 |
Table2.
Estimated reduction percentage (%) of Rs in presence of SWCNT for both dyes.
Table options
-->

Download as CSV
Dyes underconsideration | I(dV/dI)–I plot | (dV/dlnI)–I plot (Cheung method) | H(I)–I plot |
For RB (with SWCNT) | 76.08 | 83.56 | 83.01 |
For MR (with SWCNT) | 64.23 | 67.10 | 66.89 |
Basically, high values of n indicate a wide distribution of barrier height (?), which can be attributed to the effect of series resistance. The value of ? has been extracted from Eq. (9) and H(I)–I graph. Estimated data is listed in Table 3. The Schottky mechanism is followed by the experimental devices in the present work. The Schottky effect based organic photovoltaic device is utilized for its fast switching operation and there is no junction capacitance effect[33–35]. Our evaluation suggests that the interface states properties and series resistance can be controlled by using SWCNT. Incorporation of SWCNT provides an additional percolation network, which helps to inject more charges into the active region reducing the barrier height of diodes, resulting in enhancement of the conduction of carriers lowering the value of Rs.
Dye under consideration | Value of barrier height ? (eV) |
RB | 0.95 |
RB + SWCNT | 0.86 |
MR | 0.99 |
MR + SWCNT | 0.94 |
Table3.
Estimated values of ? for different dyes.
Table options
-->

Download as CSV
Dye under consideration | Value of barrier height ? (eV) |
RB | 0.95 |
RB + SWCNT | 0.86 |
MR | 0.99 |
MR + SWCNT | 0.94 |
5.
Conclusion
In the present paper we investigate the effect of SWCNT on the Rs of RB and MR dye-based OPVs. Values of Rs for mentioned organic dye-based devices are estimated from I–V characteristics and the Cheung function. The accuracy of the value of Rs obtained from the Cheung function has also been verified by comparing with the values extracted from the H(I)–I plot. The values obtained by using the Cheung method shows a good consistency with estimated values from the H(I)–I plot. Utilizing the different methods for measuring Rs, it is observed that incorporation of SWCNT on those dyes reduces Rs dramatically. The values of ideality factor n are also determined. Experimental observation concludes that the ideality factor is reduced in the presence of SWCNT for both dyes. The barrier height also decreases 9.5% and 5.1% for RB and MR dyes respectively with incorporation of SWCNT.
Acknowledgement
The authors thank the University Grants Commission (UGC), India and the Council of Scientific and Industrial Research (CSIR), India and Jadavpur University, India for providing the required support for the experiment. The first author is also thankful to S. Sen for his cooperation in this work.