1.
Introduction
According to Moore's law, the existing adhesion/barrier stack Ta/TaN cannot satisfy the continuous shrinkage of feature size in the great-large scale integrated circuits and increase in the number of copper interconnect layers[1]. The metal cobalt (Co) relying on its low resistivity, high stability, high step coverage and good adhesion properties with copper and other advantages, is identified as the new generation of copper interconnection barrier material when the feature size of GLSI continues shrinking to 10 nm and below[2–4]. Because of the huge difference of the standard electrode potential between copper and cobalt, galvanic corrosion will happen at the interface between Cu and Co, which results in serious interface corrosion, so that the effect of multi-layer wiring flattened is reduced, the reliability of the device is reduced, and the development of microelectronic technology is restricted. Benzotriazole (BTA) as a corrosion inhibitor is widely used in the copper interconnect process. But the use of BTA has brought a lot of problems. For example, BTA forms a thick hydrophobic film on the Cu surface which leads to the generation of watermarks. The adsorption of BTA layer on the Cu surface is directly linked to the time dependent dielectric breakdown degradation. If BTA remains on Cu film buried below the barrier layers and dielectric, thermal cycling can result in delamination and device failure due to the decomposition of the adsorbed BTA film[5]. It has also been reported that 1,2,4-triazole (TAZ) (Fig. 1) performs better during Cu post-CMP cleaning because of the formation of thin and readily removable TAZ film on the Cu surface and also makes the Cu surface hydrophilic rather than hydrophobic in post-CMP cleaning solution[6]. Zhong et al.[7] studied the effect of TAZ on reducing the galvanic corrosion between Cu and Co, and analyzed the inhibition mechanism and adsorption behavior of TAZ on the Co surface. But the effect of TAZ on the removal rate of Cu and Co during the chemical mechanical polishing process was not studied. He et al.[8] studied the synergetic effects of H2O2, 1,2,4-triazole (TAZ) on cobalt (Co) corrosion and polishing properties in acidic slurry. But the mechanism of 1,2,4-triazole on cobalt, especially in the current much-needed alkaline conditions was not studied.

class="figure_img" id="Figure1"/>
Download
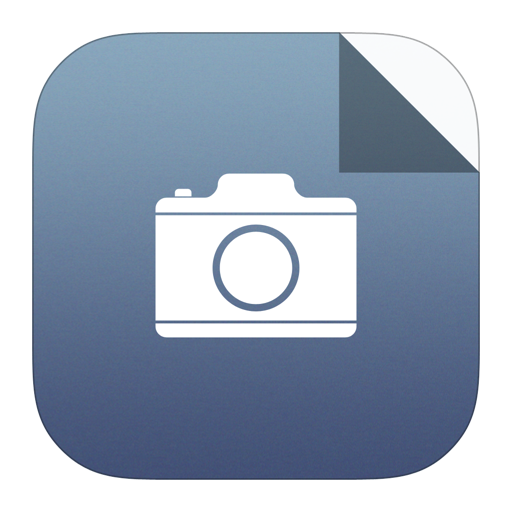
Larger image
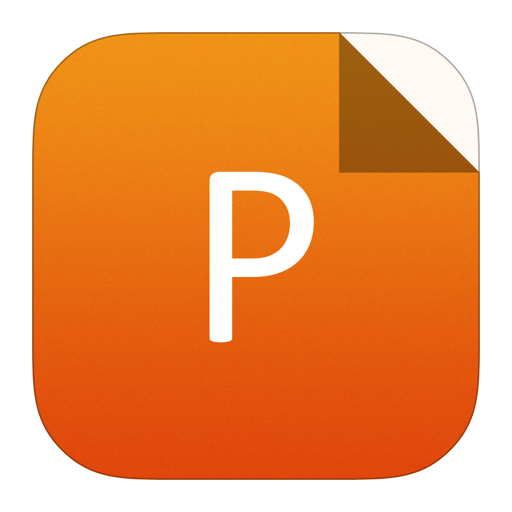
PowerPoint slide
Figure1.
Molecular formula of 1,2,4-triazole.
In this paper, the influence of TAZ on galvanic corrosion between Co and Cu in alkaline solution at pH 10 was characterized by the electrochemical analysis method and contact corrosion potential difference method. The effects of TAZ on the removal rate of Co and Cu during the CMP process in alkaline solution at pH 10 was studied by chemical mechanical polishing.
2.
Experimental
2.1
Electrochemical experiments
Electrochemical measurements were performed on a Shanghai Chenhua-CHI660E electrochemical workstation. A three-electrode glass cell using a saturated calomel electrode (SCE) as the reference electrode, a platinum (Pt) electrode as the counter electrode, and a cobalt or copper coupon as the work electrode was employed in the experiments. The cobalt coupon was a square electrode having a length of 20 mm, a width of 10 mm, and a thickness of 1 mm, and the purity was 99.99%. The copper coupon was a square electrode having a length of 20 mm, a width of 10 mm, and a thickness of 1.5 mm, and the purity was 99.99%. Open circuit potential (Eoc) was measured for 600 s, where the scan range was ?1 to 1 V. Open circuit voltage up and down amplitude of 500 mV was the voltage range of the dynamic potential polarization curve. The scanning rate of the dynamic potential polarization curve was 10 mV/s. The working area of the working electrode was a small square of 1 × 1 cm2, and the rest was wrapped with insulating epoxy. Before the experiment, copper and cobalt coupons need to be treated as follows: first, cobalt and copper coupons were polished using 2000# alumina sandpaper; then they were cleaned by anhydrous ethanol and de-ionized water; finally, they were dried with high purity nitrogen.
2.2
Polishing experiments
Cobalt disc and copper disc were polished on a France-E460E polisher from Alpsitec. The polishing pad used in the polishing was politex regularly. The diameter of the copper disc and cobalt disc used for the polishing experiments was 76.2 mm. The purity of cobalt disc and copper disc were 99.99%. The composition of polishing slurries were colloidal silica with the particle size between 70 to 90 nm, and formulated with chelating agent, hydrogen peroxide with a mass fraction of 30% and 1,2,4-triazole. The work pressure was set up to 1 psi. The rotating speed of the polishing head was set up to 87 rpm. The rotating speed of the polishing pad was set up to 93 rpm. The slurry flow rate was set up to 300 ml/min. To ensure the reliability of the results, perform three separate polishing tests to take the average. The Co and Cu removal rate (RR) was calculated by weighing method (Mettler Toledo ML503, precision 0.1 mg). The removal rate was calculated by the following formula:
$${ m RR} = frac{{Delta m}}{{ ho tS}}, $$ ![]() |
where Δm is the difference between the mass before and after polishing; ρ is the density of the substrate; t is the polishing time; and S is the area of the substrate.
3.
Results and discussion
All the experiment slurries were composed of 0.5 wt% colloidal silica, 0.05 wt% chelating agent (FA/O), 0.1 vol%. H2O2 and the different concentration of 1,2,4-triazole. The pH of the slurries was adjusted to 10 using diluted HNO3 and KOH. The 1,2,4-triazole molecular formula is shown in Fig. 1. The chelating agent (FA/O) was devised by Hebei University of Technology (HEBUT). It was a unique and faint yellow viscous liquid with the molecular weight about 500 alkaline additive and it has multiple functional groups such as tertiary amine, hydroxyl and ethanol, which can form complex ions with copper ion[9, 10]. The formula of the FA/O chelating agent is shown in Fig. 2.

class="figure_img" id="Figure2"/>
Download
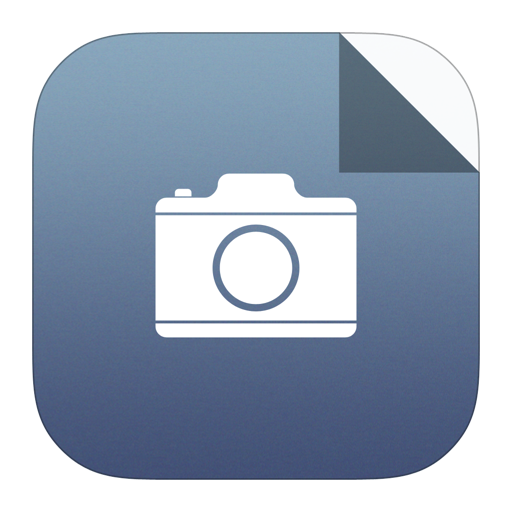
Larger image
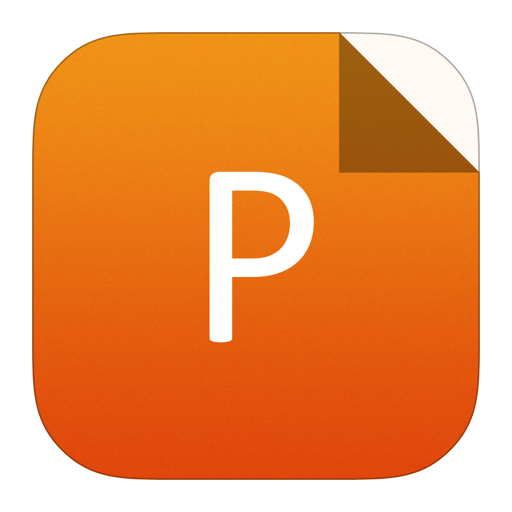
PowerPoint slide
Figure2.
Formula of an FA/O chelating agent.
3.1
The effect of 1,2,4-triazole on the galvanic corrosion between Co and Cu.
The corrosion potential, corrosion current density and corrosion potential difference of Cu and Co at the different concentrations of 1,2,4-triazole are shown in Table 1. The corresponding Tafel diagram is shown in Fig. 3. The corrosion potential (Ecorr) and the corrosion current density (Icorr) were calculated by the extrapolation method on the Tafel plot. The galvanic potential (Egc) and the galvanic corrosion current density (Igc) were determined from the (E, I) coordinates of the point of intersection between the cathodic Tafel branch of Cu (Co) and the corresponding anodic branch of Co (Cu)[11].
TAZ concentration (ppm) | Cobalt | Copper | |ΔEcorr| (mV) | Igc (μA/cm2) | |||
Ecorr (mV) | Icorr (μA/cm2) | Ecorr (mV) | Icorr (μA/cm2) | ||||
50 | 53 | 0.00991 | 119 | 0.008195 | 66 | 0.0066 | |
200 | 147 | 0.024945 | 174 | 0.021135 | 27 | 0.007 | |
345 | 168 | 0.02987 | 167 | 0.028 855 | 1 | 0.00002 | |
500 | 91 | 0.006795 | 86 | 0.006195 | 5 | 0.000 32 |
Table1.
The Icorr (corrosion current density), Ecorr (corrosion potential) and Igc (gavalnic corrosion current density) with different concentration of 1,2,4-triazole without abrasion.
Table options
-->

Download as CSV
TAZ concentration (ppm) | Cobalt | Copper | |ΔEcorr| (mV) | Igc (μA/cm2) | |||
Ecorr (mV) | Icorr (μA/cm2) | Ecorr (mV) | Icorr (μA/cm2) | ||||
50 | 53 | 0.00991 | 119 | 0.008195 | 66 | 0.0066 | |
200 | 147 | 0.024945 | 174 | 0.021135 | 27 | 0.007 | |
345 | 168 | 0.02987 | 167 | 0.028 855 | 1 | 0.00002 | |
500 | 91 | 0.006795 | 86 | 0.006195 | 5 | 0.000 32 |
These solutions shown in Fig. 3 contain 0.05 wt% FA/O chelating agent, 0.1 %vol. H2O2, and are free of abrasive.

class="figure_img" id="Figure3"/>
Download
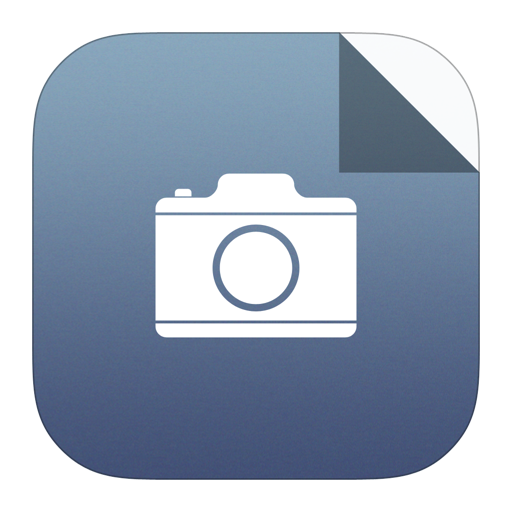
Larger image
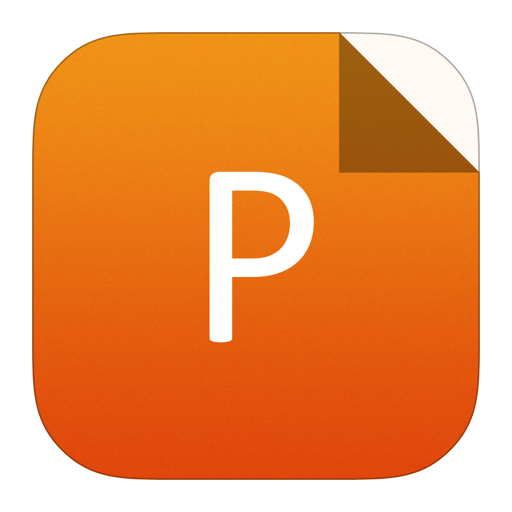
PowerPoint slide
Figure3.
(Color online) The potentiodynamic polarization plots for Co and Cu in the solution with different concentrations of 1,2,4-triazole. (a) 0 ppm. (b) 50 ppm. (c) 200 ppm. (d) 345 ppm. (e) 500 ppm.
In the electrochemical experiment of Co, the corrosion potential of Co (CoEcorr) decreased from 66 to 53 mV and the corrosion current density of Co (CoIcorr) decreased from 6350 to 9.91 nA/cm2 with the addition of 50 ppm TAZ. With the TAZ concentration increased from 50 to 345 ppm, the corrosion potential of Co increased from 53 to 168 mV and CoIcorr increased from 9.91 to 29.87 nA/cm2. When the concentration of TAZ increased to 500 ppm, CoEcorr decreased to 91 mV, and CoIcorr also decreased to 6.795 nA/cm2.
In the electrochemical experiment of Cu, the corrosion potential of Cu (CuEcorr) increased from 29 to 119 mV and the corrosion current density of Cu (CuIcorr) decreased from 5640 to 8.195 nA/cm2 with the addition of 50 ppm TAZ. With the TAZ concentration increased from 50 to 200 ppm, the corrosion potential of Cu increased to 174 mV, and CoIcorr increased to 21.135 nA/cm2. When the concentration of TAZ increased to 500 ppm, CoEcorr decreased to 86 mV, and CoIcorr slightly elevated to 28.855 nA/cm2 and then down to 6.195 nA/cm2.
Therefore, with the TAZ concentration increased from 50 to 345 ppm, the potential corrosion difference of copper and cobalt was reduced from 66 to 1 mV and Igc decreased from 6.6 to 0.02 nA/cm2. When the concentration of TAZ continually increased 500 ppm, the potential corrosion difference of copper and cobalt slightly increased to 5 mV and Igc increased to 0.32 nA/cm2.
Jiang et al.[12] studied that 1,2,4-triazole existed in two types of 1,2,4-TAH (about 60%) and 1,2,4-TA- (about 40%) at pH 10. The molecular structures of BTA and 1,2,4-triazole have a similar ring structure as used in copper inhibit- ors[12]. The formation of a TAZ passive film on the Cu surface was similar to what was observed when BTA passivated Cu. The specific adsorption of TAZ onto the Co surface was similar with the BTA inhibiting effect on the Co surface under different pH conditions[7].
In the inexistence of TAZ in the solution, the Reactions (1)–(3) occurred on the cobalt surface when cobalt acted as anodes[13], the Reactions (6)–(8) occurred on the copper surface when copper acted as anodes[14] and the Reactions (4) and (5) occurred on the cathode (Pt acted as cathode in the separated electrochemical experiments of cobalt and copper). The Reactions (9) and (10) happened on the surface of copper, and the reaction rate was closely related to pH.
In the presence of TAZ in the solution, the adsorbed 1,2,4-TAH and 1,2,4-TA– forms an inert mental–TAZ complex. The Reactions (11), (12) occurred on the cobalt surface when cobalt acted as anodes[13], and the Reactions (13)–(16) occurred on the copper surface when copper acted as anodes[12]. Each of these anodic processes was driven by an attendant cathodic process, for instance the oxygen reduction reaction. Reaction (5) can individually mix, respectively, with Reactions (12), (15) and (16) and get the Reactions (17)–(19).
$${ m{Co}} + 2{ m{O}}{{ m{H}}^-} = { m{Co}}{left( {{ m{OH}}} ight)_2} + 2{{ m{e}}^-},$,$ ![]() | (1) |
$${ m{Co}}{left( {{ m{OH}}} ight)_2} + { m{O}}{{ m{H}}^-} leftrightarrow { m{CoOOH}} + {{ m{H}}_2}{ m{O}} + {{ m{e}}^-},$,$ ![]() | (2) |
$$3{ m{Co}}{left( {{ m{OH}}} ight)_2} + 2{ m{O}}{{ m{H}}^-} leftrightarrow { m{C}}{{ m{o}}_3}{{ m{O}}_4} + 4{{ m{H}}_2}{ m{O}} + 2{{ m{e}}^-},$,$ ![]() | (3) |
$${{ m{H}}_2}{{ m{O}}_2} + 2{{ m{e}}^-} leftrightarrow 2{ m{O}}{{ m{H}}^-},$,$ ![]() | (4) |
$${{ m{O}}_2} + 2{{ m{H}}_2}{ m{O}} + 4{{ m{e}}^-} leftrightarrow 4{ m{O}}{{ m{H}}^-},$,$ ![]() | (5) |
$${ m{Cu}} + { m{O}}{{ m{H}}^-} to { m{CuOH}} + {{ m{e}}^-},$,$ ![]() | (6) |
$${ m{Cu}} + 2{ m{O}}{{ m{H}}^-} to { m{Cu}}{left( {{ m{OH}}} ight)_2} + 2{{ m{e}}^-},$,$ ![]() | (7) |
$${ m{CuOH}} + { m{O}}{{ m{H}}^-} leftrightarrow { m{CuO}} + {{ m{H}}_2}{ m{O}} + {{ m{e}}^-},$,$ ![]() | (8) |
$${ m{CuOH}} leftrightarrow { m{C}}{{ m{u}}^ + } + { m{O}}{{ m{H}}^-},$,$ ![]() | (9) |
$${ m{Cu}}{left( {{ m{OH}}} ight)_2} leftrightarrow { m{C}}{{ m{u}}^{2 + }} + 2{ m{O}}{{ m{H}}^-},$,$ ![]() | (10) |
$${ m{Co}}^{2+} + 2left( {1,2,4-{ m{TAH}}} ight) leftrightarrow { m{Co}}{left( {1,2,4-{ m{TA}}} ight)_2}+2{ m H}^+,$$ ![]() | (11) |
$${ m{Co}} + 2{(1,2,4-{ m{TA}})^-} leftrightarrow { m{Co}}{left( {1,2,4-{ m{TA}}} ight)_2} + 2{{ m{e}}^ - },$$ ![]() | (12) |
$${ m{Cu}}^+ + 1,2,4-{ m{TAH}} leftrightarrow { m{Cu}}left( {1,2,4-{ m{TA}}} ight)+{ m H}^+,$$ ![]() | (13) |
$${ m{Cu}}^{2+} + 2(1,2,4-{ m{TAH}}) leftrightarrow { m{Cu}}{left( {1,2,4-{ m{TA}}} ight)_2}+2{ m H}^+,$$ ![]() | (14) |
$${ m{Cu}} + {(1,2,4-{ m{TA}})^-} leftrightarrow { m{Cu}}{left( {1,2,4-{ m{TA}}} ight)_2} + {{ m{e}}^ - },$$ ![]() | (15) |
$${ m{Cu}} + 2{(1,2,4-{ m{TA}})^-} leftrightarrow { m{Cu}}{left( {1,2,4-{ m{TA}}} ight)_2} + 2{{ m{e}}^ - },$$ ![]() | (16) |
$$begin{split}{ m{Co}} + & (1/2){{ m{O}}_2} + {{ m{H}}_2}{ m{O}} + 2{(1,2,4 - { m{TA}})^ - }& = { m{Co}}{left( {1,2,4 - { m{TA}}} ight)_2} + 2{ m{O}}{{ m{H}}^ - },end{split}$$ ![]() | (17) |
$$begin{split}2{ m{Cu}} + & (1/2){{ m{O}}_2} + {{ m{H}}_2}{ m{O}} + 2{(1,2,4 - { m{TA}})^ - } & = 2{ m{Cu}}left( {1,2,4 - { m{TA}}} ight) + 2{ m{O}}{{ m{H}}^ - },end{split}$$ ![]() | (18) |
$$begin{split}{ m{Cu}} + & (1/2){{ m{O}}_2} + {{ m{H}}_2}{ m{O}} + 2{(1,2,4 - { m{TA}})^ - } & = { m{Cu}}{left( {1,2,4 - { m{TA}}} ight)_2} + 2{ m{O}}{{ m{H}}^ - },end{split}$$ ![]() | (19) |
$${ m{Co}}{left( {{ m{OH}}} ight)_2} + { m{O}}{{ m{H}}^-} = { m{HCoO}}_2^-+ {{ m{H}}_2}{ m{O}}.$$ ![]() | (20) |
From the above reaction, it can be seen that in the electrochemical experiment of Co, with adding 50 ppm TAZ, the chelating reaction of the chelating agent in the solution played a major role due to there being less concentration of TAZ in the solution, which promoted the dissolution of Co and made the corrosion potential of Co slightly lower. The reaction of the cathode was suppressed due to the presence of the passivating agent in the solution, thereby affecting the reaction as the surface of the anode Co, therefore CoIcorr decreased. With the TAZ concentration increasing (increase to 345 ppm), the Co surface was covered with a layer of insoluble Co–TAZ due to the enhancement of the passivation effect of TAZ, which inhibited corrosion of cobalt. Therefore, CoEcorr increased. But Reaction (20)[11] was still present in the solution, which was promoted with the progress of Reaction (17), so that CoIcorr increased. When the TAZ concentration further increased (such as 500 ppm) in the solution, which would result in a fragile soluble complex formation, and the corrosion potential of Co reduced due to the partial dissolution of the film. With the increase of OH– concentration in the solution, the reaction of the cathode was suppressed, so that Reactions (11) and (12) were suppressed. Therefore, CoIcorr reduced.
There are two passivation paths for the passivation of 1,2,4-triazole on the copper surface. The first one is the direct growth of the Cu–1,2,4-triazole passivating film on the copper surface. The second one is the re-deposition of Cu–1,2,4-triazole complex on the oxidized copper surface. The formation of Cu–1,2,4-triazole complex by the second passivating path was affected by the concentration of local complexing agent and the particle size of Cu–1,2,4-triazole complex. Therefore, the second path only serves as a complement and accounts for a small proportion of the whole passivation[12].
In the electrochemical experiment of Cu, with adding 50 ppm TAZ, according to Reactions (18) and (19), it is known that the Cu surface was covered with a layer of insoluble Cu–TAZ film, so that CuEcorr increased and CuIcorr decreased. With the increase of TAZ concentration (increased to 200 ppm), the passivation of TAZ was enhanced and CuEcorr continued to increase, but CuIcorr increased due to the ionization of Reactions (9) and (10). With continually increasing the concentration of TAZ to 500 ppm, excessive concentration of TAZ made Cu and TAZ generate soluble complexes, which were easy to dissolve and reduce CuEcorr. When the concentration of TAZ increased to 345 ppm, CuIcorr raised due to the dissolution of the membrane to promote the Cu reaction in the solution. When the concentration of TAZ continued to increase, the dissolution of Cu was promoted, so that the concentration of OH– in the solution increased, the cathodic reaction was inhibited, and the reaction 13 to 16 was inhibited, so that CuIcorr reduced.
As can be seen from the above, TAZ as a passivating agent can reduce the corrosion potential difference between Co and Cu, and weaken galvanic corrosion between Co and Cu.
Without the addition of TAZ, the corrosion potential of Co (CoEcorr) was 66 mV, the corrosion potential of Cu (CuEcorr) was 29 mV, and the corrosion potential difference between copper and cobalt (|Ecorr| = |CoEcorr – CuEcorr|) was 37 mV. The corrosion potential of Co (CoEcorr) decreased to 53 mV, the corrosion potential of Cu (CuEcorr) increased to 119 mV and the potential corrosion difference of copper and cobalt increased to 66 mV with the addition of 50 ppm TAZ. At this time the chelating reaction of the chelating agent in the solution played a major role due to there being less concentration of TAZ in the solution, which promoted the dissolution of Co and made the corrosion potential of Co slightly lower. But according to Reactions (18) and (19), it is known that the Cu surface was covered with a layer of insoluble Cu–TAZ film, so that CuEcorr increased. So that the corrosion potential difference between copper and cobalt increases to 66 mV. With the TAZ concentration increased to 200 ppm, the corrosion potential of Co increased to 147 mV, the corrosion potential of Cu increased to 174 mV, and the corrosion potential difference between copper and cobalt decreased to 27 mV. Under these circumstances, the Co surface was covered with a layer of insoluble Co–TAZ due to the enhancement of the passivation effect of TAZ, which inhibited the corrosion of cobalt. Therefore, CoEcorr increased, and CuEcorr continued to increase, due to the copper surface of the passivation film continuing to further increase. It causes a reduction in the corrosion potential difference between copper and cobalt. With the TAZ concentration increased to 345 ppm, the corrosion potential of Co increased to 168 mV, the corrosion potential of Cu decreased to 167 mV and the corrosion potential difference between copper and cobalt decreased to 1 mV. This moment, the Co surface was covered with a layer of insoluble Co–TAZ due to the enhancement of the passivation effect of TAZ, which inhibited corrosion of cobalt and CoEcorr increased. Simultaneously, excessive concentration of TAZ made Cu and TAZ generate soluble complexes, which were easy to dissolve and reduce CuEcorr. That led to a reduction in the corrosion potential difference between copper and cobalt. When the concentration of TAZ continually increased 500 ppm, the corrosion potential of Co decreased to 91 mV, the corrosion potential of Cu decreased to 86 mV and the corrosion potential difference between copper and cobalt slightly increased to 5 mV. Now the corrosion potential of Co reduced due to the partial dissolution of the film, the mechanism of action of Cu is similar to Co.
3.2
The effect of 1,2,4-triazole on Co and Cu rate selectivity
The removal rate of Co and Cu with different concentrations of 1,2,4-triazole was shown in Fig. 4. In the CMP experiment of Co, due to the synergistic effect of H2O2 and FA/O chelating agent in the polishing slurry, the Co surface formed a loose passive film, which was more easily mechanically removed because the passive film hardness is less than that of the Co metal element[15]. Therefore, the removal rate of Co was accelerated. Similarly, the removal rate of Cu was also accelerated, and because the chelating effect of the FA/O chelating agent on Cu was stronger than that of Co, so that the removal rate of Cu was much higher than that of Co. Cobalt RR was 14.778 nm/min and copper RR was 252.144 nm/min in the presence of oxidant (H2O2) and FA/O chelating agent without a component of TAZ in the solution.

class="figure_img" id="Figure4"/>
Download
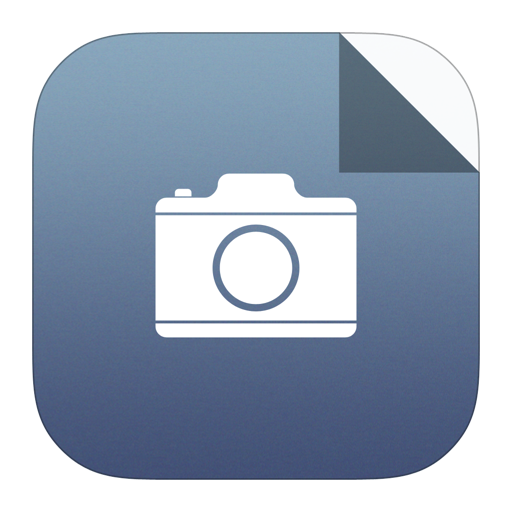
Larger image
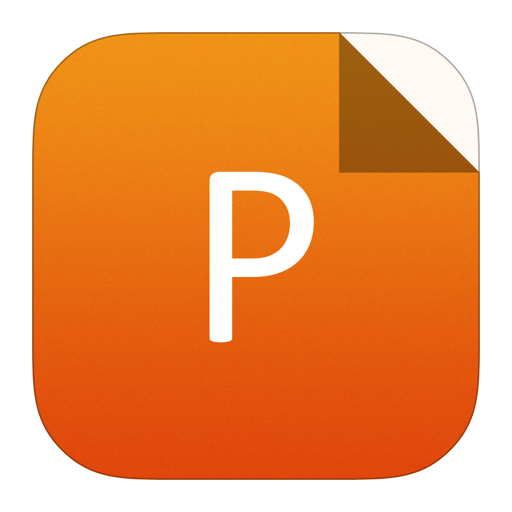
PowerPoint slide
Figure4.
(Color online) The RR of the Co and Cu in the solution with different concentrations of 1,2,4-triazole.
The cobalt RR increased to 36.124 nm/min when the 50 ppm TAZ was added to the solution. With the concentration of TAZ continuing to increase to 200 ppm, cobalt RR increased to 65.68 nm/min. The cobalt RR decreased gradually when the TAZ concentration continued to increase.
When the concentration of TAZ was very low in the solution, the two states of TAZ react with Co to form Co–TAZ film. Under mechanical action, the passive film formed on the Co surface was easily removed, so cobalt RR increased. When the TAZ concentration was further increased, because the Cu–1,2,4-triazole complex was a film in the form of nano-particles, Co also had a similar property, so that a relatively thin Co–TAZ film was formed on the surface of Co, which was easy to remove. So in a certain concentration of TAZ, cobalt RR increased. When the concentration of TAZ outstrips 200 ppm, the cobalt RR decreased due to the Co–TAZ film gradually becoming dense and preventing the removal of Co, even if the removal rate was small under mechanical action.
The copper RR decreased to 62.016 nm/min when the 50 ppm TAZ was added to the solution. With the concentration of TAZ continuing to increase to 345 ppm, copper RR decreased to 47.328 nm/min. The copper RR slightly increased when the TAZ concentration continued to increase.
When the concentration of TAZ was very low in the solution, the copper RR was rapidly reduced due to the formation of the passive film. With the increase of TAZ concentration, the Cu–TAZ film was more and more dense, and it was not easy to remove even in the mechanical grinding state, so copper RR would continue to decrease, but the rate of decline was getting slower. When the concentration of TAZ outstrips 345 ppm, the copper RR increased due to the excess concentration of TAZ, where the weaker passive film was easily dissolved.
When the 1,2,4-triazole concentration was 200 ppm, cobalt RR reached 65.68 nm/min, copper RR reached 48.96 nm·min?1, and the removal rate ratio of cobalt and copper was 1.34 : 1. When the 1,2,4-triazole concentration was 345 ppm, cobalt RR reached 62.396 nm/min, copper RR reached 47.328 nm/min, and the removal rate ratio of cobalt and copper was 1.32 : 1. Since the removal rate of Co was higher than that of Cu, there was a certain correcting function for dishing bits caused by the copper CMP process due to the difference removal rate of metallic copper and the barrier material in the process of CMP.
4.
Conclusion
In this paper, the effect of 1,2,4-triazole in alkaline polishing slurry on galvanic corrosion between Co and Cu and the effect on the selectivity of copper and cobalt in the barrier CMP process were studied. It was concluded that adding a certain amount of 1,2,4-triazole in the polishing slurry could effectively reduce the galvanic corrosion between cobalt and copper. At the same time in the CMP process, Co and Cu polishing rates were effectively controlled. The removal rate of cobalt was faster than that of copper, which helped to correct the dishing pits created by copper in chemical mechanical polishing due to the difference removal rate of metallic copper and the barrier material in the process of CMP. When the slurry was composed of 0.5 wt% colloidal silica, 0.1 vol% hydrogen peroxide, 0.05 wt% FA/O, 345 ppm 1,2,4-triazole, the corrosion potential difference between Co and Cu decreased to 1 mV and the galvanic corrosion current density reached 0.02 nA/cm2. Therefore, the galvanic corrosion could be reduced effectively. Meanwhile, the removal rate of Co was 62.396 nm/min, the removal rate of Cu was 47.328 nm/min, so that the removal rate ratio of cobalt and copper was 1.32 : 1, which was a good amendment to the dishing pits. Therefore, it could well meet the requirements of the barrier CMP.