1.
Introduction
Silicon (Si) is considered as the most important semiconductor material for the microelectronics and photovoltaics industry. However, as a typical indirect bandgap semiconductor, bulk Si is not a good candidate for photonic devices. It is because the radiative recombination of electrons and holes inside bulk Si must be assisted by phonons. Meanwhile, the lifetime of non-radiative recombination process, such as Auger recombination, free carrier absorption and so on, is much shorter than that of the radiative recombination process. As a consequence, the luminescence efficiency of Si-based materials is very low and the optical absorption is relatively small compared to the semiconductor materials with direct bandgap[1, 2].
In order to develop the Si-based photonic and optoelectronic devices, many kinds of Si structures have been studied, among which, Si nanocrystals (Si NCs) are one of the most promising candidates for photonic and photovoltaic applications[3–5]. For crystalline Si (c-Si), electrons are free to move in three dimensions. With the size decreasing, especially approaching the “excitation Bohr radius” of Si (~4.9 nm)[6], the carriers will be confined in a narrow space region and the band structures will be strongly modified due to the quantum confinement effect[7, 8]. For Si NCs, the quantum confinement effect will lead to the broadening of bandgap with the reduction of dot size and the radiative recombination probability is obviously improved because of the enhanced overlap between the electron wavefunction in conduction band and the hole wavefunction in valence band[9]. Therefore, it is believed that the efficient light emission can be realized by using Si NCs-based materials.
On the other hand, Si NCs are also an important material used in photovoltaic devices. The first-generation solar cells based on c-Si have been in the major position for several decades. However, further development of solar cells was strongly restricted by the high cost of bulk Si. Although the second-generation solar cells based on thin films have cut down the cost, the efficiency is still not high enough. Recently, the all Si-based tandem solar cells, which belong to the so-called third-generation solar cells, are proposed. Like the thin-film solar cells, the tandem solar cells are deposited on cheap substrates to reduce the cost of c-Si. Meanwhile, since the photons can be absorbed in several sub-cells, the efficiency is intensively improved. It is known that the optical bandgap of Si NCs can be broadened with the size decreasing, so the absorption wavelength can be effectively controlled by varying the dot size. Therefore, the broadband spectral response can be realized and the photoelectric conversion efficiency (PCE) can be enhanced obviously.
In this paper, we present an overview of the fabrication and device applications of Si NCs-based multilayers, especially in luminescent and photovoltaic devices. The paper is organized as follows: (1) The fabrication techniques to get multilayered structures containing Si NCs are briefly introduced. It is shown that periodic structures with dense Si NCs and smooth interfaces can be achieved. (2) the utilization of Si NCs multilayers in luminescent and photovoltaic devices are reviewed systematically. The possible ways to improve the device performance are also described. (3) We summarize the paper and give the perspective for further considerations.
2.
Fabrication and characterization of multilayer structures
In order to use the Si NCs in devices, it is very important to get the size-controllable Si NCs with the mature and low-cost techniques. So far, many approaches have been developed to fabricate Si NCs, such as molecular beam deposition[10, 11], sputtering[12, 13], low-pressure chemical vapour deposition (LPCVD)[14], plasma enhanced chemical vapor deposition (PECVD)[15, 16], electron-beam deposition[17], and so on. In most fabrication processes, Si NCs are formed after high temperature annealing of Si-rich Si-based alloys. Since the crystallization process of Si atoms can be influenced by the annealing temperature and the surrounding environment, the size of Si NCs cannot be well controlled. By using multilayered structure as shown in Fig. 1, the size of formed Si NCs can be well controlled by the thickness of the a-Si sublayer due to the constrained crystallization principle[18]. Moreover, the inter-dot distance in the vertical direction, which is usually the main path of carrier transport, is also well controlled by the thickness of dielectric layers. It is also indicated that the local crystallization can be realized by using laser crystallization method with the phase-shift grating mask to get the patterned Si NCs materials[19, 20]. The multilayered structure provides a platform to study the properties and device applications of Si NCs. Since then, the Si NCs/dielectrics (SiOx, SiNx, SiC, and so on) sandwiched and/or multilayered structures were widely used in Si NCs-based nano-devices.

class="figure_img" id="Figure1"/>
Download
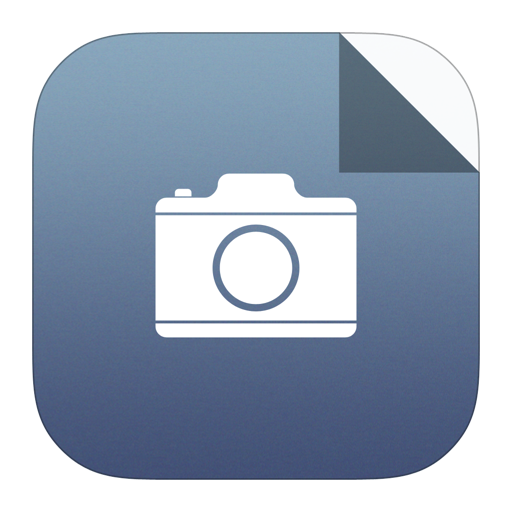
Larger image
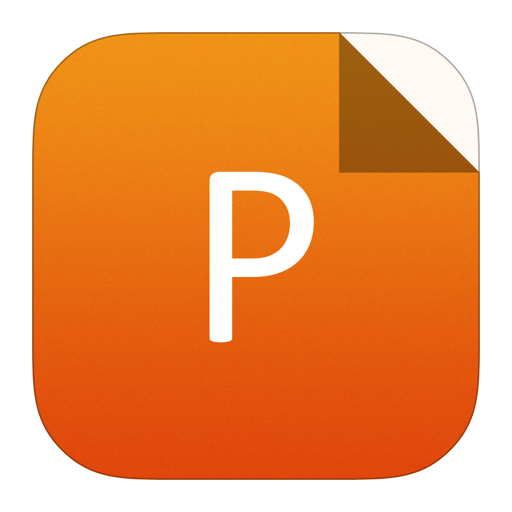
PowerPoint slide
Figure1.
(Color online) Schematic diagrams of Si nanocrystals-based multilayers.
The first experimental effort to realize the idea of constrained crystallization of ultrathin amorphous layers has been successfully employed in fabricating n-Si/a-SiNx supperlattices by Chen et al. in 1992[19]. The a-Si/a-SiNx multiquantum well superlattice samples were firstly prepared by PECVD. During the deposition, the composition of reactant gases alternated periodically between pure silane and a silane/ammonia mixture to fabricate a-Si layer and a-SiNx layer, respectively. Following by laser irradiation to crystallize the a-Si layers, high quality silicon crystallites with good controllable size were obtained in the a-Si layers under the constrained crystallization effect of multilayer structures. As shown in Figs. 2(a) and 2(b)[21], the clear multilayered structures can be well identified by cross-sectional transmission electron microscopy (TEM) image and with the laser fluence increasing, the crystallinity is gradually promoted as revealed by Raman spectra. Further, the size of Si nanocrystalline can be designed by varying the thickness of the as-deposited a-Si layers. It was reported that the a-Si layer exhibited enough constrained crystallization effect until its thickness beyond 15 nm[20], in which the Si NCs were distributed both in lateral and vertical directions rather than formation of a single layer of Si NCs.
Besides the Si NCs/SiNx multilayered structure, Si NCs/SiO2 multilayered structure has also been attracted much attention since it is compatible with the microelectronic industry. As mentioned before, the most common ways of Si NCs/SiO2 multilayers preparation are mainly upon the molecular beam epitaxy, PECVD and e-beam evaporation to obtain alternately stacked amorphous silicon layers (or silicon supersaturated layers) and SiO2 layers, followed by high temperature annealing process[10–17]. Gourbilleau et al. fabricated Si-rich Silica/SiO2 multilayers with 23 periods by reactive magnetron sputtering technique. They used hydrogen and argon mixed plasma to reduce the oxygen content originated from SiO2 target to get Si-rich silica sublayers[22]. The subsequent high temperature annealing was performed to get Si NCs/SiO2 multilayers. During the thermal treatment process, the amorphous silicon layers undergo a phase separation, resulting in the nucleation and growth of Si NCs. The Si NCs with dot size as small as 2 nm can be formed in the multilayered structure as shown in Fig. 2(c)[23]. Meanwhile, the dielectric layers can be replaced by SiC to form the Si NCs/SiC multilayers since the amorphous SiC material has a relatively bandgap compared to that of SiO2 or SiN films, which is helpful for improving the carrier transport or collection efficiency due to the reduced barrier height both for electron and hole. The fabrication method of Si NCs/SiC multilayers can also be realized by using PECVD system and high temperature annealing process[24]. It is worth mentioning here that, since the bandgap of Si NCs is directly related to the dot size, the graded-sized Si NCs/SiC multilayers was designed to enhanced broadband spectral response and energy conversion efficiency for relevant solar cells[25], which will be discussed below.

class="figure_img" id="Figure2"/>
Download
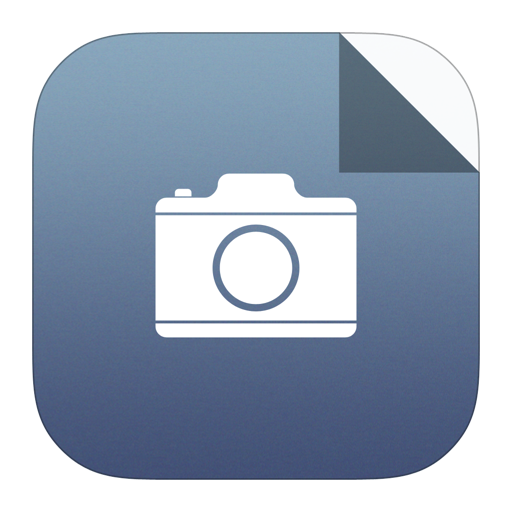
Larger image
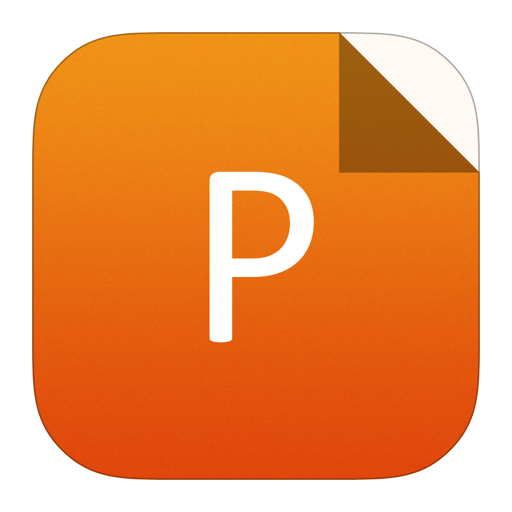
PowerPoint slide
Figure2.
(a) Cross-section TEM micrograph and (b) Raman scattering spectra of laser crystallized a-Si/a-SiN multiquantum well samples. Reprinted with the permission from Ref. [21], Copyright ? 1996 Published by Elsevier B.V.. (c) Cross-sectional TEM micrograph of Si NCs/SiO2 multilayers sample; Inset is the magnified image of a single Si NC. The diameter of Si NCs is about 2.0 nm, which is consistent with the Si layer. Reprinted with the permission from Ref. [23], Copyright ? 2016 Macmillan Publishers Limited, part of Springer Nature.
3.
Light emitting devices
Si-based light sources have attracted much attention due to its crucial role in realizing the on-chip optoelectronic integration. In 1990, strong visible photoluminescence (PL) from porous Si was observed at room temperature[26], which made it possible to prepare optical sources with Si nanostructures. The first observation of intense room-temperature visible PL was reported by Chen et al. in laser crystallized a-Si:H/a-SiNx:H multiquantum-well structure[19]. The size-dependent PL behaviors have been reported due to the quantum confinement effect[20, 27, 28]. Cen et al. prepared a-Si:H/SiNx multilayers and obtained dense Si NCs by laser irradiation and thermal annealing[20]. They found that the size of Si NCs was directly related to the initial a-Si:H film and the PL peak position was varied from 660 to 725 nm with increasing the Si film thickness. They explained the interesting phenomena with the quantum confinement theory: owing to the narrow space of quantum region, the optical band gap of Si can be blue-shifted and the recombination probability of electron hole pairs can be significantly enhanced when the dot size is decreasing. In many reports it was reported that the PL peak can be tuned in a wide spectral range by controlling the dot size of Si NCs-based multilayers[29–31]. However, in the Si NCs/SiO2 multilayers, both the size-dependent and size-independent luminescence were observed[11, 32–34]. For the luminescent peak regardless of the size of Si NCs, the defects or the radiative centers at the interface or in the dielectric matrix are often considered as the origins[35–38]. In 2010, it was reported that a short-lived light emission can be identified in Si NCs and the luminescence spectrum was enhanced and red-shifted with the smaller nanocrystal sizes. They claimed that the observed luminescence can be assigned to the phonon-free recombination of electron-hole pairs, which indicated the direct transition in Si NCs with reduced dot sizes[29]. More recently, based on the single-dot spectroscopy experiments and theoretical analysis, Luo et al. clearly pointed out that the direct-like radiative transition observed in the above report could be an effect of Auger or multi-exciton recombination, but not the energy shifting of the Γ-point[39].
Though the mechanism of light emission from Si NCs is still unclear, much efforts to get optical gain and efficient light emitting devices have been made in the past few decades. Since the first observation of optical gain in Si NCs[4], the value of optical gain in Si-based materials has not been improved obviously. Recently, it is reported that the enhanced optical gain even comparable with those based on GaAs or InP and the optical pumped Si lasing based on Si NCs are observed[40]. It is worth noting that the hydrogen passivation is critical in the fabrication process because the dangling bond defects must be saturated. It is also revealed in many other reports that passivation can significantly improve the optical performance of the Si NCs dispersed in solution or embedded in superlattices[41–44]. The optical pumped Si lasers are a progress to finally realize the electric pumped all-Si lasers.
For the Si NCs multilayered LEDs, p- or n-type c-Si wafers are used as the substrates and the Al or other metal electrodes are evaporated both on the front surface of multilayers and rear side of Si wafers. Wang et al. fabricated the light emitting devices based on the Si NCs/SiNx multilayers prepared by using KrF excimer laser annealing method. A stable electroluminescence (EL) was observed at room temperature under the applied voltage higher than 17 V. The EL peak was shifted from 780 to 600 nm with decreasing the Si sublayer thickness from 4 to 1 nm[45]. Rui et al. also studied the EL characteristics of Si NCs/SiC multilayers[46]. They prepared the multilayers with different dot sizes after high temperature annealing, and they found that the EL peak was blue-shifted and the intensity was improved with decreasing the dot size, which proved that the EL was originated from the recombination of electron-hole pairs in Si NCs. Further investigation of Si NCs/SiO2 multilayers was conducted by Mu et al.[47] Fig. 3(a) shows the EL spectrum under 12 V in the range of 250–1350 nm under DC driving mode. The black lines are detected spectra in visible and infrared range of device with Si QDs. The red dash line is the fitted curve and the blue lines are the two fitted bands. The green line is the EL emission of device without Si QDs. Up inset is the schematic diagram of light emitting device structure based on Si NCs/SiO2 multilayers; the below one is the EL pattern of the device under applied voltage of 12 V.

class="figure_img" id="Figure3"/>
Download
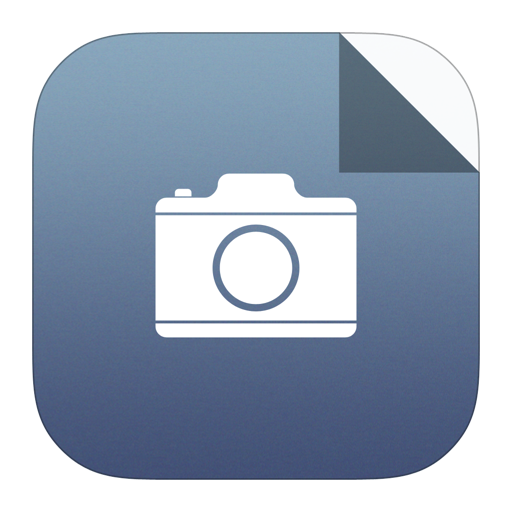
Larger image
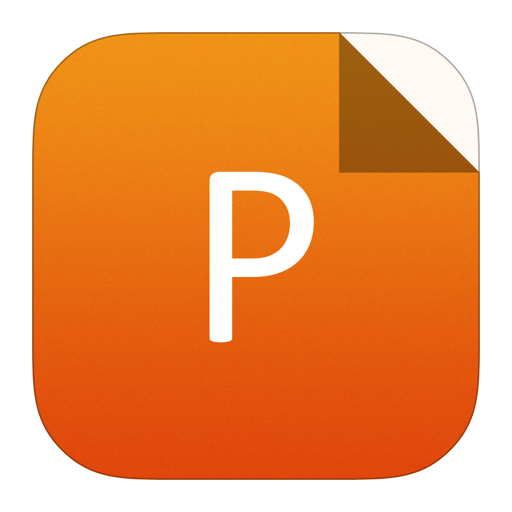
PowerPoint slide
Figure3.
(Color online) (a) DC EL spectrum of Si NCs/SiO2 multilayered LED. The up inset is the schematic structure and the below one is the EL pattern. (b) Stability of the Si NCs multilayered LED under DC and AC driving conditions in the elapsed working time of 3 h with a constant applied voltage of 20 V. Reprinted with the permission from Ref. [47], Copyright ? 2014 IEEE.
It is reported that the barrier materials play the important role in governing the EL behaviors since the dielectric sublayers are used as energy barriers to confine the carrier recombination process in the Si NCs layers. The composition and the thickness of the barrier materials can affect the carrier transport process obviously. By using Si-rich SiN multilayered structures, the EL efficiency is enhanced by more than one order of magnitude compared to that of single SiN layer, which is also reported in Si NCs/SiC multilayers[48, 49]. The improved performance of LEDs can be attributed to the variation in the band offset which improves the carrier injection efficiency[48]. Moreover, the EL efficiency can also be tuned by changing the Si/N ratio of barrier layers. The similar results are also present in Si NCs/SiO2 multilayers. The electron and hole bipolar injection is demonstrated in the devices containing multilayered Si NCs embedded in ultrathin SiO2 layers with thickness less than 2 nm. As shown in Fig. 4, the light emitting devices with efficiency of 0.17% have been realized and the turn-on voltage is as low as 1.7 V[50]. The high power efficiency and low turn-on voltage can be explained as the improved radiative recombination of excitons formed in Si NCs via the direct tunneling mechanism[50, 51].

class="figure_img" id="Figure4"/>
Download
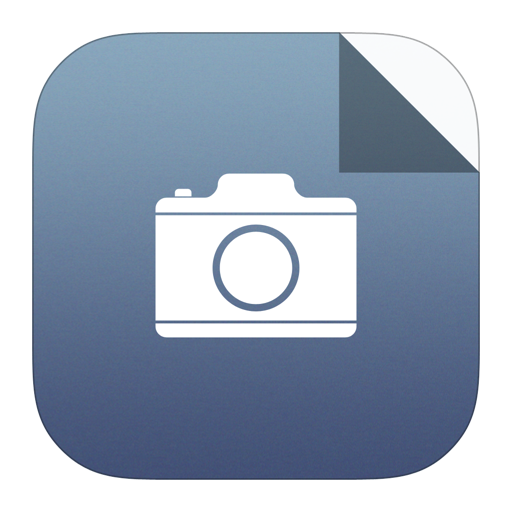
Larger image
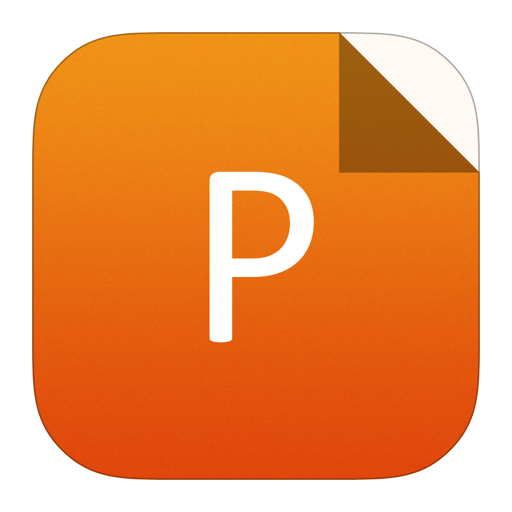
PowerPoint slide
Figure4.
(Color online) Power efficiency as a function of the injected current density for the LED device without a thick injection barrier. The dashed line indicates the current density which separates dominant bipolar injection, which is more efficient, from dominant unipolar injection through the FN tunneling. The inset shows the electroluminescence spectrum of device at an injection current of 10 A/cm2. Reprinted with the permission from Ref. [50]. Copyright ? 2009 American Institute of Physics.
It seems that the electroluminescence efficiency from Si NCs is far from the value they can reach. A good example is the colloidal Si NCs passivated by different ligands[52]. With different energy band types and passivation situation, the electric performance of LEDs is optimized. Fig. 5(a)[52] shows the schematic diagram of LEDs containing colloidal Si NCs passivated by phenylpropyl ligands (PhPr-Si NCs) and octyl ligands (Octyl-Si NCs), respectively. As shown in Fig. 5(b)[52], the EQE of PhPr-Si NCs based LED is much lower than that of Octyl-Si NCs based LED. Because the benzene rings in the phenylpropyl ligands enhance the electron transport and suppress the hole transport, the serious unbalance between the electron and hole injection exists in PhPr-Si NCs based LEDs, which leads to the comparably lower EQE. With the suitable passivation ligands, Octyl-Si NCs based LEDs realize the highest EQE of 6.2% which is among the best LEDs based on colloidal Si NCs. This report highlights the critical role of surface passivation for Si NCs. Meanwhile, Gu et al. used HAT-CN or MoO3 interlayers between ITO and PEDOT:PSS to enhance the hole transport and increase the work function of ITO, resulting in a high EQE of 2.4%[53]. It is indicated that a suitable carrier transport layer material can improve the electric performance of Si NCs based LEDs as well.

class="figure_img" id="Figure5"/>
Download
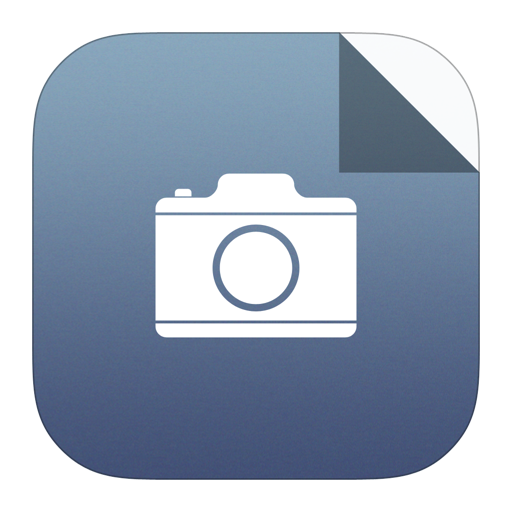
Larger image
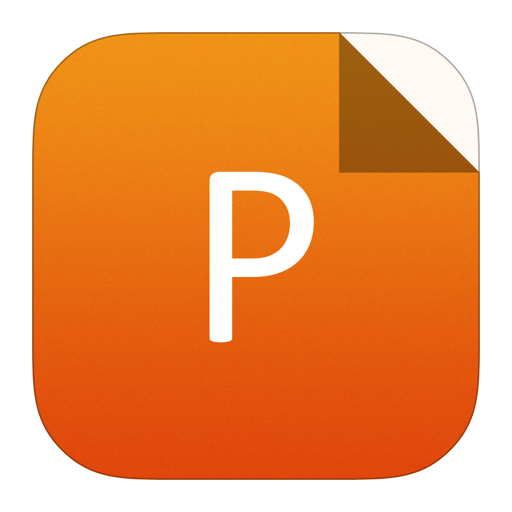
PowerPoint slide
Figure5.
(Color online) (a) Schematic of the structure of a Si NCs based LED. (b) EQE versus voltage for an Octyl-Si NCs based LED and a PhPr-Si NCs based LED. Reprinted with the permission from Ref. [52], Copyright ? 2018 American Chemical Society.
It is known that the external quantum efficiency (EQE) of light emitting devices can be enhanced by improving the internal quantum efficiency (IQE) or the light extraction efficiency. Kim et al. reported that the radiative recombination rate was increased by localized surface plasmas and the corresponding EL was enhanced by 434%[54]. Xu et al. fabricated the EL devices based on Si NCs/SiC multilayers embedded in PN junction to get p–i–n type device structures[55]. They found that the turn-on voltage was reduced and the luminescence efficiency was significantly enhanced due to the improved internal radiative recombination probability. Recently, it has been reported that the emission efficiency of Si NCs can also be effectively improved by introducing novel nanostructures to make the efficient photon management. By using the nanosphere lithography technique, one can get the nano-patterned Si substrates, which exhibit a broadband anti-reflection or light trapping effect[56, 57]. The depth can be controlled by changing the etching time, while the periodicity can be tuned by changing the diameter of nanospheres. Fig. 6(a)[57] shows the cross-section transmission electron microscopy image for Si NCs/SiO2 multilayers deposited on nano-patterned substrate. It is shown that the Si NCs/SiO2 multilayers keep the periodically patterned morphology on the nano-patterned substrates and the anti-reflection effect is further improved after the deposition of the multilayers. As shown in Fig. 6(b)[56], the EL intensity of Si NCs/SiO2 multilayers is enhanced by over one order of magnitude.

class="figure_img" id="Figure6"/>
Download
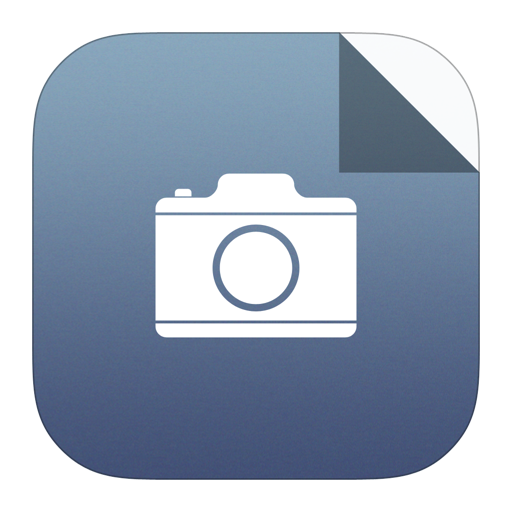
Larger image
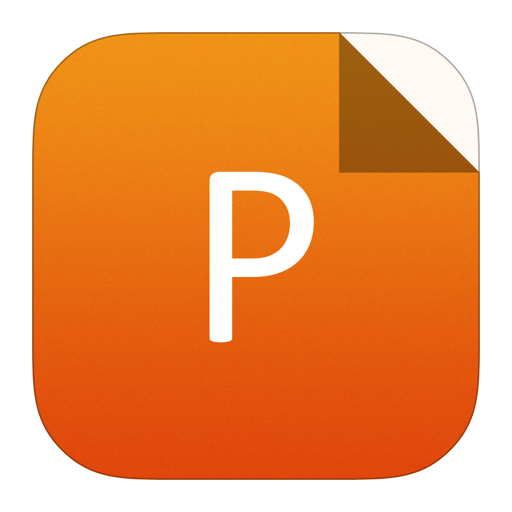
PowerPoint slide
Figure6.
(Color online) (a) The cross-section transmission electron microscopy image for Si NCs/SiO2 multilayers deposited on nano-patterned substrate. Reprinted with the permission from Ref. [57], Copyright ? 2011 Optical Society of America. (b) The integrated EL intensity versus the applied voltage for the flat and nanopatterned devices. Reprinted with the permission from Ref. [56], Copyright ? 2010 Optical Society of America.
Si nanowire array is another interesting structure to improve the light extraction efficiency of the light emitting devices. Zhai et al. prepared the Si NCs/SiO2 multilayers on the Si nanowires[58]. With the proper Si nanowire depth, the EL intensity was enhanced obviously and the turn-on voltage was as low as 3.5 V. Moreover, the EL intensity were further enhanced after hydrogen passivation of the Si nanowires, which can be attributed to the effective suppression of the non-radiative defects generated during the etching process. Similar phenomena were also observed previously by using hydrogen ion beam irradiation on Si NCs/SiO2 multilayers[59]. It was found that the hydrogen ion beam irradiation increased the interfacial roughness and simultaneously passivated the non-radiative Pb centers. Both factors enhanced the light emitting efficiency and reduced the turn-on voltage. Besides the hydrogen passivation, ultrathin high-k dielectric film is also helpful for reducing the surface defect states of Si nanowires. It was reported that, by inserting a 7 nm Al2O3 thin layer between the Si nanowires and Si NCs-based multilayers, the EL intensity was increased by 8.5 folds at the injection current of 130 mA comparing with that of un-passivated devices[60]. The enhancement can be attributed to the reducing numbers of interface states caused by the passivation of Al2O3 layer.
For industrial and commercial applications, the stability is an important question to be considered. In most cases, LEDs based on Si NCs were driven by direct-current (DC) sources. In that case, the hot electrons generated by high voltage will lead to the degradation of the oxide matrix and low device endurance[50]. Meanwhile, the charge accumulation at interface states under the DC conditions will decrease the injection efficiency. Beyer et al. applied AC voltage to the light emitting field effect transistors based on narrow layers of Si NCs to reduce the oxide degradation[61]. They increased the alternative-current (AC) frequency limit for effective EL signals. Mu et al. applied the AC sources to the Si NCs/SiO2 multilayers-based LED and obtained strong EL signals[47]. As shown in Fig. 3(b), in comparison to the DC driving samples, the EL intensity of AC driving keeps stable for over 3 h, which makes the Si NCs/SiO2 multilayers a potential material for reliable optoelectronic devices in the future. The reason for the excellent stability under AC driving mode may be attributed to the well-preserved multilayered structure even under the high voltage. Firstly, in AC driving conditions, the average flowing current is lower than that in dc conditions under the same applied voltage, results in less thermal degradation of the device. Secondly, the effective operation time for AC driving devices is less, which means longer working life. Thirdly, the trapped charges at the interface can be extracted in each period and fewer defects will be created. This is also helpful to improving the device endurance.
4.
Photovoltaic devices
Recently, Si NCs-based multilayers have attracted much attention due to their potential application in the next generation of solar cells[1, 62, 63]. Theoretically, the efficiency of single-junction bulk Si solar cells is limited to 29% (Shockley-Queisser limit), because of the incomplete utilization of high-energy photons and the transmission of low-energy photons[64]. Therefore, the tandem solar cells composed with semiconductors with various bandgaps are designed to improve the PCE[65]. Since the bandgap of the Si NCs can be modified by changing the dot size, the solar cells can be designed and fabricated by utilizing the Si NCs with various sizes to get the broadband spectral response[66]. In 2001, Green et al. proposed the concept of the third-generation solar cells[67], and the all-Si tandem solar cells based on Si NCs were designed by Cho et al.[68]. By taking into account of the radiation combination and Auger combination, they calculated the theoretical efficiency of two-junction and three-junction to be 42.5% and 47.5%, respectively, which has exceeded the Shockley-Queisser limit. To realize better PCE, Meillaud et al. studied the most compatible bandgaps of the all-Si tandem solar cells[69]. The tandem solar cells were assumed to meet two requirements: (1) A perfect balance of current densities exists between the top and bottom cells. (2) All photons transmitted by the up cells are absorbed by the bottom cell. The studies revealed that the best PCE could be achieved for a three-junction tandem solar cell when the bandgap for the up, middle and bottom p–n junction was 2, 1.5, and 1.1 eV, respectively.
In order to realize the all Si-based tandem type solar cells with high PCE by using Si NCs, the first step is to fabricate the solar cell structure containing Si NCs/c-Si heterojunction[70–72]. The initial effort was made to fabricate the P-doped Si NCs/SiO2 superlattice structure with the magnetron sputtering method followed by high temperature annealing process on p-type Si wafer. With the size of Si NCs decreasing, the IQE of the heterojunction solar cells in the visible light spectrum was strengthened, and the solar cells with 3-nm-sized Si NCs showed the best performance with PCE around 10%[71, 73]. Kim et al. obtained the high P doped Si rich silica/SiO2 multilayers structure and the highest PCE was reported to be 7.08% in laboratory[72].
Considering the comparably high barrier between Si and SiO2, the amorphous SiC or SiN films with lower energy gaps are chosen to replace the SiO2 films for better transportation and collection of photon-generated carriers[73–75]. For example, Song et al. fabricated the periodic multilayers with 6-nm-thick Si rich SiC and 2.5-nm-thick SiC sublayers. They obtained the Si NCs/SiC multilayers after high temperature annealing. The PCE of the solar cells reached 4.66% and the IQE at 400 nm was 35% which is higher than traditional c-Si cells[76]. Wu et al. fabricated the P doped Si rich SiN films by ECR-CVD and obtained Si NCs embedded in SiNx after annealing. The size of the NCs can be controlled by changing the Si to N ratio and the electric performance can be optimized. The best PCE was reported to be 8.6%[77]. The fabrication technique, structural characterization, optical and electrical performance of hydrogenated amorphous silicon carbide (a-SiC:H) films were also investigated by Li et al.[78]. They fabricated the a-SiC:H films in a conventional PECVD system with the layer-by-layer technique, and demonstrated that the hydrogen plasma annealing can improve the film quality. The photo-sensitivity is as high as 106. Cao et al. fabricated Si NCs/SiC multilayers based solar cells by annealing a-Si:H/SiC multilayers and obtained the Si NCs with the average size of about 4.8 nm[79]. As shown in Fig. 7, the solar cell has the open circuit of 532 mV, short circuit current density of 24.1 mA/cm2 and PCE of 6.28%. It is obvious that the photovoltaic performance is significantly improved. Because the Si NCs can enhance the absorption of incident photons, especially in the short wavelength range, the EQE is also improved. They further proposed and fabricated the graded-sized Si NCs/SiC multilayers-based heterojunction solar cells[25], as shown in Fig. 8(a). It was demonstrated that the optical absorption was enhanced in a wide spectral range (300–1200 nm) because the optical bandgaps of Si NCs with various sizes efficiently match the incident solar photons. The cell exhibited a short circuit current density of 28.36 mA/cm2 and a PCE of 7.45%. Meanwhile, the optical absorption was enhanced to 86.3% as shown in Fig. 8(b).

class="figure_img" id="Figure7"/>
Download
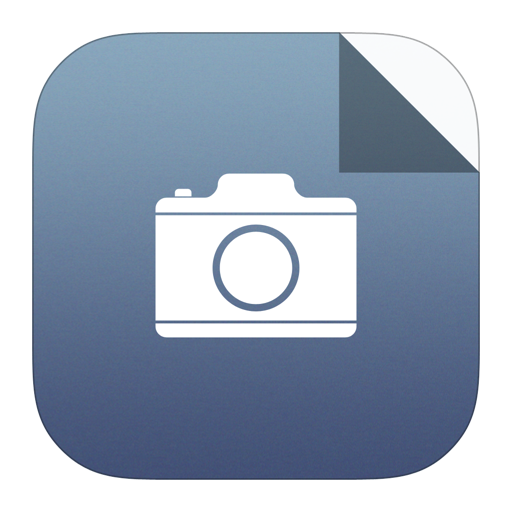
Larger image
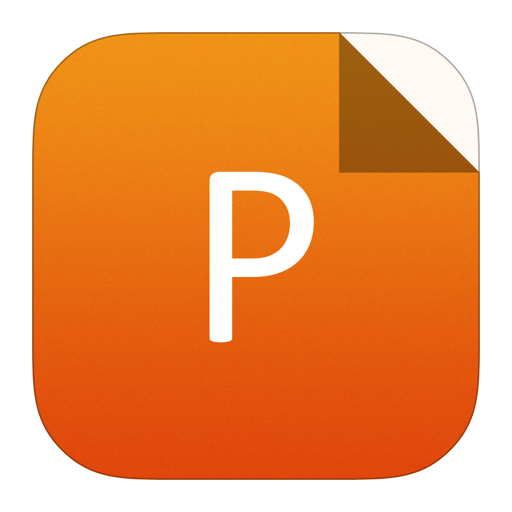
PowerPoint slide
Figure7.
(Color online) The current density of as-deposited and 900 °C annealed solar cells with p–i–n structures. Inset is the schematic diagram of the device structure. Reprinted with the permission from Ref. [79], Copyright ? 2014 Springer Open.

class="figure_img" id="Figure8"/>
Download
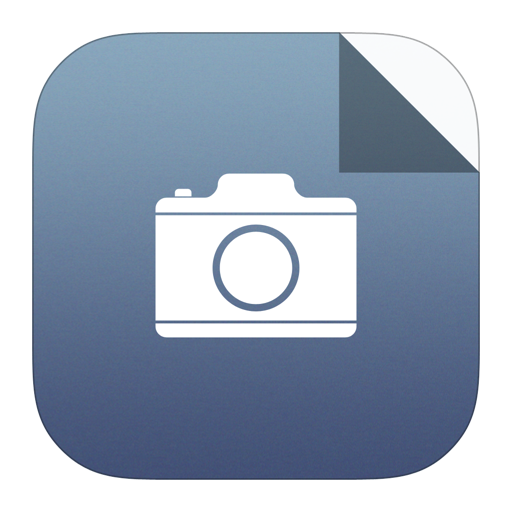
Larger image
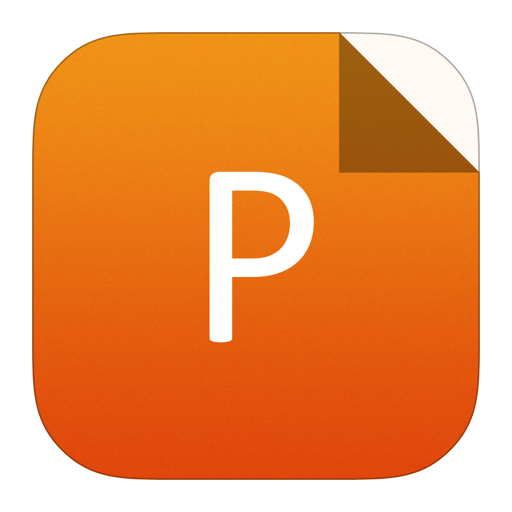
PowerPoint slide
Figure8.
(Color online) (a) Schematic diagram of hetero-junction solar cells containing Si QDs/SiC MLs on flat n-Si substrates and Si NWs substrates. (b) The illuminated J–V curves of graded-size Si NCs/c-Si and graded-size Si NCs/Si nanowires cells. The inset is the dark I–V curves. Reprinted with the permission from Ref. [25], Copyright ? 2015 The Royal Society of Chemistry.
Although the Si NCs are efficient to improve the performance of solar cells, it is still difficult to separate the contribution of Si NCs from that of bulk Si substrates. As shown in Fig. 9, it is desired to get the PV device on non-Si substrate, such as quartz or other glass substrate with transparent conductive oxide (TCO) as electrode, to study the photovoltaic properties of Si NCs-based MLs alone[80, 81]. Loper et al. proposed a device structure without the bulk Si substrate, which were used to clearly investigate the function of Si NCs[80]. For this device, the open circuit voltage was up to 370 mV and it was demonstrated that the photovoltaic activity originated only from the Si NC membrane p–i–n diode. However, the relatively low PCE implies that the losses both optically and electrically are serious in PV device only containing Si NCs.

class="figure_img" id="Figure9"/>
Download
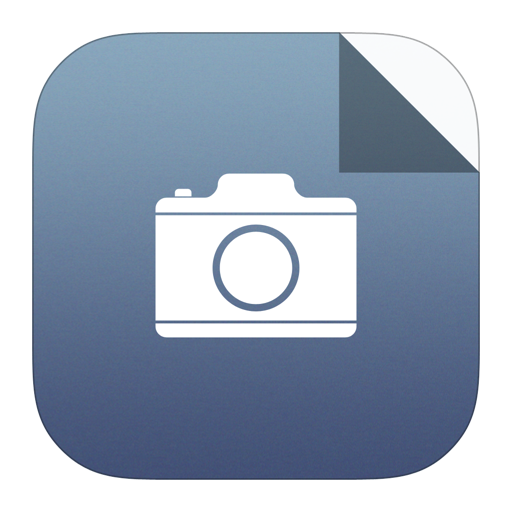
Larger image
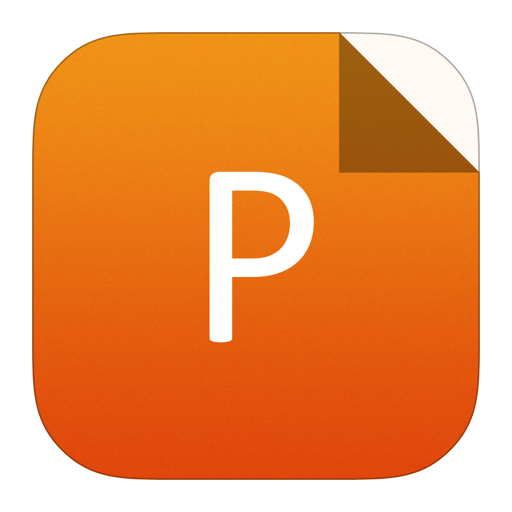
PowerPoint slide
Figure9.
(Color online) Schematic diagram of p–i–n solar cell structure changed from Si substrate to non-Si substrate with Si NCs-based multilayers in the intrinsic region for studying PV properties of Si NCs alone.
Fabrication of the anti-reflection structure is also an effective way to improve the optical and electrical performance of solar cells containing Si NCs, which is a quite attractive topic recently. Samukawa et al. prepared the two-dimensional periodically arranged nano Si structures, and obtained the uniformly distributed Si nano-pillars. The nano-structure exhibited extremely high absorption efficiency in the visible spectrum, and the heterojunction solar cells based on it showed the fill factor of 72% and PCE as high as 12.6%[82, 83]. Lu et al. utilized the nano-sphere lithography technique to get the periodically nano-patterned Si nanostructures and applied it in a-Si/c-Si heterojunction solar cells[84]. Due to the good antireflection performance in a wide range, both the IQE and EQE were improved. The periodically nano-patterned Si nanostructures are also used in the Si NCs/SiO2 multilayers heterojunction solar cells, and exhibit good anti-reflection characteristics and down-shifting effect, which leads to a much better cell performance[85]. For the Si NCs/SiC heterojunction solar cells, the antireflection structures also play an important role to improve the optical and electrical performance. As shown in Fig. 8(b), by applying nanowires in the solar cells based on Si NCs/SiC multilayers, both the open circuit voltage and short circuit current density are improved. The PCE is further enhanced from 7.45% to 12.8%[25].
5.
Conclusion and perspective
Si NCs-based multilayer, as one of interesting low dimensional materials, has opened a new route to the new-generation photonic and photovoltaic applications. Although a great deal of theoretical and experimental progress has been made, there are still many challenges. For the light emitting devices, the luminescence efficiency is far from the requirement of actual applications. How to improve the device performance is an open question. As discussed before, colloidal Si nanocrystals with well surface passivation exhibited the high EQE[52, 53, 86, 87]. It is indicated that the quantum efficiency can be enhanced by effective passivation and carrier injection, which suppresses the non-radiative recombination and promotes the radiative recombination. On the other hand, most of the light emission of Si NCs lies in the visible range, which is not compatible for the optical telecommunication (near infrared range). This has seriously restricted the development of Si NCs in Si-based on-chip optoelectronic integration. To realize the long-wavelength light emission, introduction of luminescence centers, like Er ions in Si NCs, is an effective method[88–90]. Recently, it was found that by doping of phosphorus or co-doping of phosphorus and boron impurities, Si NCs can generate the sub-band light emission which meets the wavelength requirement of optical telecommunications[23, 91, 92]. However, the physical mechanism behind the sub-band light emission is still unclear and how to achieve EL with doped Si NCs is a challenging topic.
For photovoltaic applications of Si NCs, the thickness of Si NCs layers in most cases is usually far less than that of the bulk Si substrates. Therefore, a lot of carriers are generated in the substrates, which has made it difficult to identify the role of Si NCs in the solar cells. Moreover, high temperature annealing process impedes the utilization of the cheap substrates like glass plates. Laser crystallization of amorphous Si-based multilayers to get size controllable Si NCs materials may be an effective approach to prepare solar cells based on Si NCs on glass substrates. Of course, to realize the p-type and n-type Si NCs is necessary to get suitable device structures.