

Cloning of potato heat shock transcription factor StHsfA3 gene and its functional analysis in heat tolerance
TANG Rui-Min

通讯作者:
收稿日期:2020-05-25接受日期:2020-08-19网络出版日期:2021-04-12
基金资助: |
Received:2020-05-25Accepted:2020-08-19Online:2021-04-12
Fund supported: |
作者简介 About authors
E-mail:

摘要
关键词:
Abstract
Keywords:
PDF (3680KB)元数据多维度评价相关文章导出EndNote|Ris|Bibtex收藏本文
本文引用格式
唐锐敏, 贾小云, 朱文娇, 印敬明, 杨清. 马铃薯热激转录因子HsfA3基因的克隆及其耐热性功能分析[J]. 作物学报, 2021, 47(4): 672-683. doi:10.3724/SP.J.1006.2021.04114
TANG Rui-Min, JIA Xiao-Yun, ZHU Wen-Jiao, YIN Jing-Ming, YANG Qing.
植物在生长发育过程中经常会受到各种不利环境如极端温度、盐渍化、干旱、重金属等胁迫[1]。其中, 热胁迫是对植物生长繁殖影响最严重且最不易控制的因素之一[2]。而且, 温室效应的积累加剧了高温的影响力, 从而造成农作物减产, 大大威胁了我国乃至全世界的粮食安全[1]。马铃薯营养丰富, 种植广泛, 是一种重要的粮蔬兼用型作物。然而马铃薯性喜凉冷, 不耐高温。在夏季马铃薯栽培过程中, 高温会严重影响其生长发育过程, 导致块茎产量和质量下降[3]。因此, 阐明马铃薯对热胁迫的响应机制, 发掘耐热相关基因, 对马铃薯耐热性改良具有重要意义。
热激转录因子(heat shock transcription factor, Hsf)作为热胁迫信号转导途径中的重要元件, 激活热胁迫响应基因的转录表达, 在植物热响应调控过程中发挥关键的作用[4]。与其他转录因子类似, Hsf家族成员的N端有1个保守的螺旋-转角-螺旋结构, 为Hsf的DNA结合域(DNA binding domain, DBD)[5]。与DBD区域相毗邻的是寡聚域(oligomerization domain, OD), 由2个疏水的七肽重复序列(hydrophobic heptad repeats, HR-A和HR-B)和1段插入序列组成[5,6]。根据HR-A/B之间插入氨基酸残基的数目不同, 可将Hsf分成A、B和C三大类。
与动物和酵母相比, 植物中的Hsf家族成员十分丰富[7]。例如在拟南芥(Arabidopsis thaliana)[8]、番茄(Solanum lycopersicum)[9]、水稻(Oryza sativa)[10]和杨树(Populus trichocarpa)[11]中, 分别鉴定得到21、24、25和30个Hsf家族成员。这些Hsf成员在植物对各种复杂的非生物胁迫响应过程中发挥重要作用[12]。例如, 在拟南芥中, HsfA1e、HsfA4a、HsfB2a和HsfC1表达会受到低温胁迫、盐胁迫以及渗透压胁迫的诱导[7,13-14]。在我们前期的研究中, 鉴定出27个马铃薯Hsf家族成员, 分属A、B和C三大类。这些马铃薯Hsf家族基因对热胁迫、干旱胁迫和冷胁迫均有不同程度的响应[15]。
HsfA3是热激转录因子A家族的一个成员。在正常条件下, HsfA3表达属于组成型表达, 存在于细胞质中; 而当植物受到热胁迫时, 其表达为诱导型表达, 作用在细胞核中[16]。研究表明, HsfA3在植物耐热调控方面起着重要的作用。例如, 在拟南芥中, HsfA3在DREB2A (dehydration-responsive element bindingprotein 2A)的调控作用下表达, 并对热胁迫做出响应, 从而级联调控其下游一系列热诱导基因表达, 使植株获得更高的耐热性[17]。在番茄中, HsfA3能够与MAP激酶(mitogen-activated protein kinase, MAPK)直接相互作用来调控植物对热胁迫的响应[18]。在马铃薯中, 我们也发现StHsfA3在热胁迫诱导下能够高效表达, 表明StHsfA3可能在马铃薯响应热胁迫的过程中起着重要的作用[15]。除了调控植物对热胁迫响应之外, HsfA3还参与了其他非生物胁迫应答。在水稻中发现, OsHsfA3不但参与了植物的耐热调控, 同时也通过调节依赖脱落酸和活性氧水平的多胺生物合成来提高植株的耐旱性[19]。在拟南芥中过表达百合HsfA3可以通过改变脯氨酸分解代谢来提高拟南芥植株的耐热性和盐敏感性[20]。
目前, 对HsfA3的研究主要集中在拟南芥、番茄等模式植物中, 在马铃薯中尚未见到有关HsfA3克隆和表达的报道。在前期研究中, 我们通过构建共表达网络预测, StHsp26-CP (PGSC0003DMG 400003219)和StHsp70 (PGSC0003DMG400027611)可能是StHsfA3的共表达基因, 它们之间可能存在某种调控关系[15]。为了研究StHsfA3在提高植物耐热性方面的作用以及StHsfA3与相关StHsps之间潜在的表达调控关系, 本研究从马铃薯品种Désirée中克隆了StHsfA3的cDNA序列, 通过在马铃薯中过表达该基因并对其耐热性功能进行研究, 以期为揭示StHsfA3的调控机制提供基础, 同时也为马铃薯耐热育种提供基因资源。
1 材料与方法
1.1 植物材料、菌株及载体
马铃薯品种Désirée, 由南京农业大学杨清教授实验室提供, 保存于MS培养基中, 置于温度为(22 ± 1)℃、光周期为光照(16 h)/黑暗(8 h)的温室中培养。将1月龄的组培苗转移至试管中炼苗, 1周后移至基质中生长。菌株为大肠杆菌(Escherichia coli) DH5α和农杆菌(Agrobacterium tumefaciens) GV3101。克隆载体pMD19-T (TaKaRa, 中国大连)和表达载体pBA002由南京农业大学农学院喻德跃教授课题组提供。1.2 马铃薯HsfA3的克隆及序列分析
1.2.1 总RNA的提取及cDNA的合成 取马铃薯品种Désirée的叶片约0.1 g, 利用RNA提取试剂盒(TaKaRa, 中国大连)提取样品总RNA, 用不含RNase的DNase I (TaKaRa, 中国大连)消除基因组DNA的干扰。对所得RNA的完整性和浓度进行检测后, 取0.5 μg RNA, 利用反转录试剂盒Transcriptor First Strand cDNA Synthesis Kit (Roche, Mannheim, Germany)合成cDNA第1链。将1 μL合成的cDNA用9 μL nuclease-free水稀释作为模板。1.2.2 马铃薯HsfA3的分子克隆 根据NCBI网站中查到的马铃薯HsfA3预测序列(XM_015306031.1)设计带有Xho I和Mlu I双酶切位点的引物(P1, 表1)。以上述合成的cDNA作为模板, 进行PCR扩增, 扩增程序为94℃ 5 min; 94℃ 50 s, 58℃ 50 s, 72℃ 90 s, 33个循环; 72℃ 10 min。PCR产物经电泳测定后通过凝胶回收试剂盒(Axygen Scientific Inc., Union city, USA)将目的片段回收, 然后将其连接至pMD19-T克隆载体中, 转化大肠杆菌DH5α, 经PCR检测后, 对阳性克隆进行测序检验, 将测序正确的菌液加甘油冻存。
Table 1
表1
表1本研究所使用的引物
Table 1
引物编号 Primer ID. | 名称 Name | 引物序列 Primer sequence (5′-3′) | 用途 Function |
---|---|---|---|
P1-F | CloneHsfA3-F | GCGCTCGAGATGAACCCATTTGATAAAAATCAAG | StHsfA3的克隆, 酶切位点用下画线标出 |
P1-R | CloneHsfA3-R | GTCACGCGTCTAAAAACTATCATTCTTTGGCTG | Gene cloning of StHsfA3; the restriction sites are underlined |
P2-F | HsfA3002-F | GGGTAATATCCGGAAACCTCCT | 转基因验证 |
P2-R | HsfA3002-R | GCTTTCTCCATTTCTACCCCAAG | Transgenic verification |
P3-F | DLHsfA3-F | CGGAAGTTCTTCTGGATCATCTG | 荧光定量分析 |
P3-R | DLHsfA3-R | CCATCTGCTTCTGTCTTTGTTCT | qRT-PCR analysis |
P4-F | DLsHsp3219-F | CGTCCAATGTCAGTGTTGGTGG | 荧光定量分析 |
P4-R | DLsHsp3219-R | CAGTGCCTTGGTTGTTTCCTCC | qRT-PCR analysis |
P5-F | DLHsp70-F | GTGGCAGTAATGGAAGGTGGGA | 荧光定量分析 |
P5-R | DLHsp70-R | TTCTCCGGATTCACCACCGATT | qRT-PCR analysis |
P6-F | EF1α-F | CTGTTAAGGATCTGAAGCGTGGT | 荧光定量分析, EF1α为内参基因 |
P6-R | EF1α-R | AATGTGGGAAGTGTGGCAGTCG | qRT-PCR analysis; EF1α is used as the reference gene |
新窗口打开|下载CSV
1.2.3 马铃薯HsfA3的序列分析及系统发育分析
由ExPASy的PrptParam工具 (
1.3 马铃薯HsfA3过表达载体的构建及重组质粒的转化
从上述保存的大肠杆菌菌液中提取质粒, 用Xho I和Mlu I对其进行双酶切, 回收目的片段; 同时用相同的酶将pBA002载体也进行双酶切并将载体大片段回收。然后将目的基因片段与酶切后的pBA002载体通过T4连接酶(Thermo Fisher Scientific, USA)连接, 并将连接产物转化到农杆菌GV3101中。通过菌液PCR验证、酶切验证以及测序验证后, 将测序正确的含有StHsfA3-pBA002重组质粒的农杆菌菌液加甘油保存到-80℃冰箱, 用于后续遗传转化试验。利用本实验室已经建立的马铃薯遗传转化体系[21], 将StHsfA3-pBA002重组子转入马铃薯品种Désirée外植体中。经预培养、共培养、愈伤诱导、芽分化和生根筛选后, 获得转基因马铃薯株系。
1.4 转基因植株的分子检测
利用SDS法提取得到的抗性马铃薯植株以及非转基因对照植株的总DNA。为了检测StHsfA3- pBA002重组质粒是否转入马铃薯植株内, 本研究设计了包含1段pBA002表达载体和1段StHsfA3基因片段的引物(P2, 表1)。以非转基因对照植株的总DNA作为阴性对照, StHsfA3-pBA002重组质粒作为阳性对照, 用该引物进行PCR验证, 程序如下: 94℃预变性4 min; 94℃变性50 s, 58℃退火45 s, 72℃延伸50 s, 33个循环; 最后72℃延伸10 min。得到的PCR产物再经电泳检测。1.5 相对含水量(RWC)测定
将1月龄的非转基因植株(wild type, WT)以及转基因阳性株系(transgenic lines, L)的组培苗移栽到基质中, 置于温度为(22±1)℃, 光照周期为14 h光照/10 h黑暗的光照培养箱中生长2周, 再将它们分别分成2组, 一组放在35℃光照培养箱中; 另一组留在原来的培养箱中生长。处理8 h后取其叶片测定相对含水量。相对含水量的测定具体步骤如下: 秤取鲜重(fresh weight, FW)约为0.5 g的新鲜马铃薯叶片, 将其浸入水中, 一段时间后取出, 滤纸吸干表面水分, 称重; 然后再把叶片浸入水中, 过段时间再取出, 吸干叶片表面水分后再称重; 重复操作几次, 直到重量稳定, 此时叶片的重量为饱和鲜重(saturated fresh weight, SFW)。之后把吸水饱和的叶片放入纸袋, 置于温度为105℃的烘箱内杀青10 min, 再将烘箱温度调到75℃继续烘干叶片, 直至其重量不再变化。这时将叶片取出, 冷却至室温, 记录其干重(dry weight, DW)。每个样本设置3个生物学重复和3次试验重复。相对含水量计算公式如下:$\text{RWC=}\frac{\text{FW}-\text{DW}}{\text{SFW}-\text{DW}}\times 100%$
1.6 丙二醛(MDA)含量测定
用与1.5同样的方法处理马铃薯植株, 不同温度处理8 h后取马铃薯叶片测定MDA的含量。采用硫代巴比妥酸(thiobarbituric acid, TBA)法测定MDA含量[22], 其含量用“μmol g-1 FW”来表示。取马铃薯植物新鲜叶片1 g, 剪碎后加入2mL 5% TCA溶液,于研 钵中研磨成匀浆, 再加8 mL TCA, 将残液移至10 mL离心管中。4℃, 4000 ×g离心10 min; 取上清2 mL (对照中加入2 mL蒸馏水)至试管中, 加入2 mL 0.6% TBA溶液, 混匀后于沸水浴中煮沸10 min, 取出冷却至室温, 3000×g离心15 min, 取上清量取其体积VS; 以对照管内溶液为空白, 测定530 nm、600 nm和450 nm处的吸光值。每个样本进行3个生物学重复和3次试验重复。MDA含量用下列公式计算:$C=6.45\left( {{A}_{530}}-{{A}_{600}} \right)-0.56{{A}_{450}}$
$\text{MDA}\text{=}\frac{C\times {{\text{V}}_{\text{S}}}}{\text{FW}\times \text{1000}}$
1.7 StHsfA3、StHsp26-CP和StHsp70的表达量检测
用与1.5同样的方法处理马铃薯植株, 处理2 h后分别取不同处理不同植株的叶片提取RNA, 采用荧光定量PCR方法检测非转基因植物和转基因不同株系中的StHsfA3、StHsp70和StHsp26-CP的表达量。这3个基因的引物见表1 (P3, P4, P5), 以EF1α为内参基因, 引物为P6。按照FastStart Universal SYBR Green Master (ROX) (Roche, Mannheim, Germany)的使用说明, 在ABI-7500实时定量PCR仪上(Applied Biosystems, Foster City, CA, USA)检测候选基因的表达量。PCR反应体系为20 μL, 包含10 μL SYBR-Green、6.8 μL ddH2O、2 μL稀释后的模板以及各0.6 μL 10 μmol L-1的正反向引物。PCR反应程序如下: 50℃ 2 min; 95℃预变性10 min, 95℃变性5 s, 60℃退火35 s, 共40个循环。根据2-??Ct法, 对每个基因的相对表达量进行标准化计算。每个样本进行3次生物学重复和2次试验重复。利用邓肯多重测试法对结果进行统计学分析。2 结果与分析
2.1 StHsfA3基因的分子克隆及序列分析
根据NCBI网站中预测得到的马铃薯HsfA3碱基序列设计加酶切位点的引物P1, 从马铃薯品种Désirée中克隆得到StHsfA3基因的cDNA全长序列。经软件分析, StHsfA3从起始密码子ATG到终止密码子TAG的长度为1506 bp (图1-A), 共编码501个氨基酸(图1-B)。利用ExPasy网站的PrptParam工具预测StHsfA3蛋白的相对分子量为55.23 kD, 理论等电点为4.9。本研究发现, 在StHsfA3氨基酸序列的C端含有4个中心是色氨酸残基的短肽基序(图1-B): DEELWSMGFE、GAELWDSFSS、LSDLWDIDPL和GVDKWPADGS, 该结构使得HsfA3具有高效的转录激活功能。图1
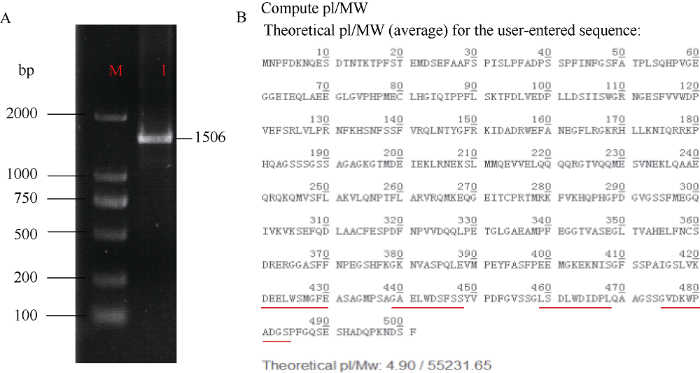
图1StHsfA3的扩增产物条带(A)及其氨基酸序列(B)
A图中: M: DNA 2000分子标记; 1: StHsfA3的扩增片段。B图中: 红线部分为4个中心是色氨酸(W)的短肽基序。
Fig. 1Amplified product stripe of StHsfA3 (A) and its amino acid sequence (B)
M: DNA 2000 marker; 1: amplified fragment of StHsfA3 in Fig. A; the motifs marked with red line are the four short peptide sequences containing tryptophan (W) in Fig. B.
对StHsfA3蛋白的二级结构预测显示, α螺旋、延伸链和无规则卷曲的氨基酸残基数分别为117、13和368个, 占整个氨基酸数目的23.35%、2.59%和73.45% (图2-A)。无规则卷曲构成了该蛋白的活性中心, 所占比例最高, 说明这部分结构不稳定。通过NCBI CD search功能对该蛋白进行分析, 预测出其含有热激转录因子Hsf典型的DBD结构域(图2-C)。其三级结构预测表明, 该DBD结构域含有3个α螺旋和4个β折叠片(图2-B), 构成了该蛋白最保守的结构域。利用Signal P-5.0预测StHsfA3信号肽发现, 该蛋白无信号肽(图2-D), 为非分泌蛋白。
图2
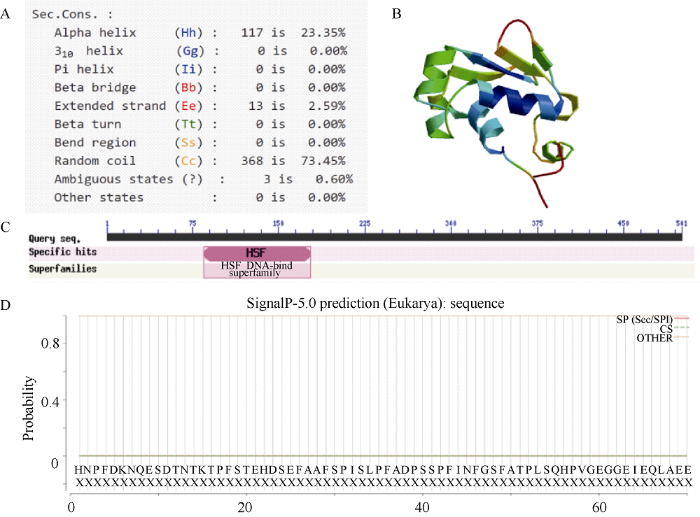
图2马铃薯StHsfA3的结构分析
A: 二级结构预测; B: 三级结构预测; C: 保守结构域预测; D: 信号肽预测。
Fig. 2Structural analysis of StHsfA3 in potato
A: secondary structure prediction; B: tertiary structure prediction; C: conservative domain prediction; D: signal peptide prediction.
利用ExPASy的ProtScale工具预测StHsfA3蛋白的疏水性发现, 构成StHsfA3蛋白的氨基酸中, 亲水性氨基酸的数量远远大于疏水性氨基酸(图3-A), 表明该蛋白属于亲水性蛋白。跨膜结构域预测分析表明, StHsfA3蛋白没有跨膜区, 不属于膜蛋白, 这与StHsfA3在细胞中的位置以及发挥的功能相适应。
图3
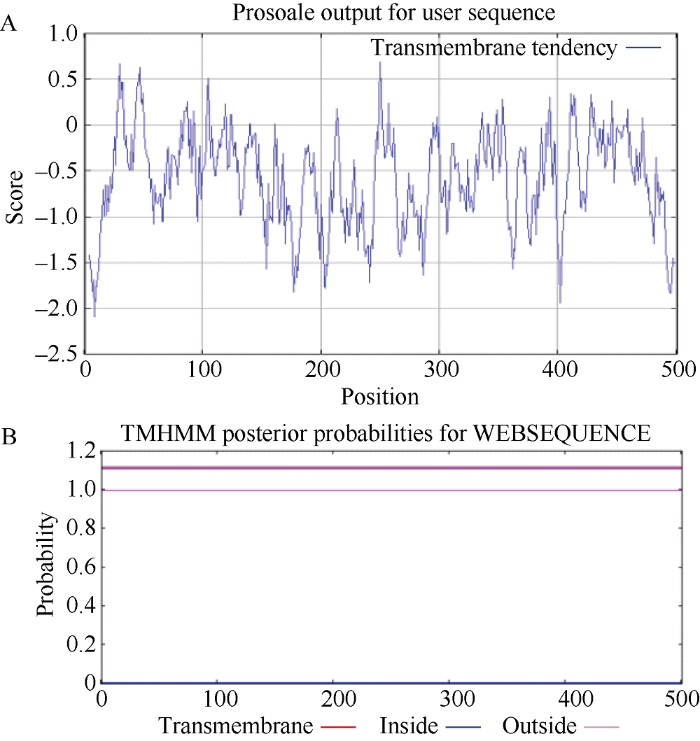
图3StHsfA3疏水性分析(A)和跨膜域分析(B)
A: 图中曲线得分在0以上说明这部分氨基酸为疏水性, 分值越高疏水性越强; 得分在0以下说明氨基酸为亲水性氨基酸, 分值越低亲水性越强。B: 没有跨膜结构域。
Fig. 3Hydrophobicity analysis (A) and transmembrane domain analysis (B) of StHsfA3
A: the curves above zero show that these amino acids are hydrophobic, and the higher score, the stronger the hydrophobicity; the curves below zero show that these amino acids are hydrophilic, and the lower score, the stronger the hydrophilicity. B: there is no transmembrane domain.
2.2 StHsfA3的系统进化分析
从NCBI数据库中搜索到15个来自于其他植物物种的HsfA3氨基酸序列, 将马铃薯HsfA3蛋白序列与其他物种中的同源序列进行比对, 用邻接法构建系统发育树(图4)。该系统发育树主要有两大分支, 双子叶植物HsfA3聚为一支, 单子叶植物HsfA3聚为一支。马铃薯HsfA3的氨基酸序列与番茄(Solanum lycopersicum)、辣椒(Capsicum annuum)和烟草(Nicotiana tabacum)这些茄科植物的亲缘关系比较近, 尤其与番茄的HsfA3亲缘关系最近。图4
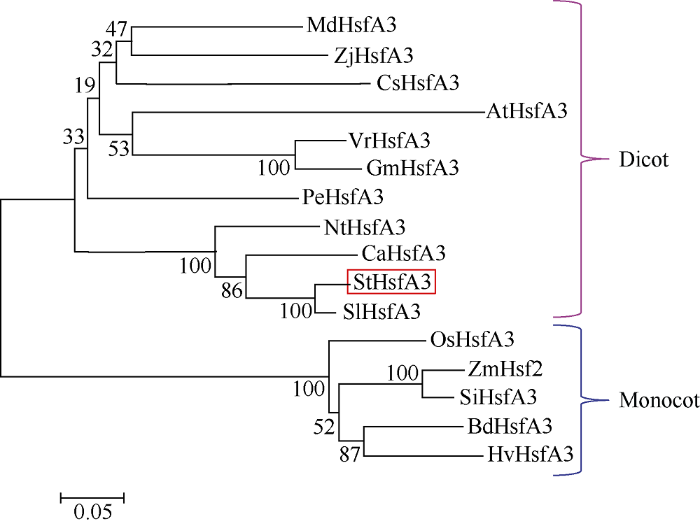
图416个物种HsfA3的系统发育树
利用ClustalX 2.1和MEGA 4.0软件构建来自16个物种的Hsf A3氨基酸序列的系统发育树, 重复计算次数设为1000。各物种名称缩写及NCBI数据库中的序列号如下: MdHsfA3: 苹果(XP_008391748.1); ZjHsfA3: 枣(XP_015898712.1); CsHsfA3: 柑橘(XP_006481891.1); AtHsfA3: 拟南芥(NP_195992.2); VrHsfA3: 豇豆(XP_014511066.1); GmHsfA3: 大豆(XP_003541445.1); PeHsfA3: 杨树(XP_011016541.1); NtHsfA3: 烟草(XP_016473897.1); CaHsfA3: 辣椒(XP_016542894.1); SlHsfA3: 番茄(NP_001234854.1); OsHsfA3: 水稻(XP_015623061.1); ZmHsfA3: 玉米(NP_001147968.1); SiHsfA3: 狗尾草(XP_004952622.1); BdHsfA3: 二穗短柄草(XP_003575055.1); HvHsfA3: 大麦(AEB26588.1)。
Fig. 4Neighbor-joining phylogenetic tree of HsfA3s from 16 plant species
The Neighbor-joining phylogenetic tree of HsfA3 amino acid sequences from 16 plant species was constructed by ClustalX 2.1 and MEGA 4.0, which was bootstrapped over 1000 cycles. Abbreviations for each species and database accession numbers are as follows: MdHsfA3: Malus domestica (XP_008391748.1); ZjHsfA3: Ziziphus jujube (XP_015898712.1); CsHsfA3: Citrus sinensis (XP_006481891.1); AtHsfA3: Arabidopsis thaliana (NP_195992.2); VrHsfA3: Vigna radiata var. radiate (XP_014511066.1); GmHsfA3: Glycine max (XP_003541445.1); PeHsfA3: Populus euphratica (XP_011016541.1); NtHsfA3: Nicotiana tabacum (XP_ 016473897.1); CaHsfA3: Capsicum annuum (XP_016542894.1); SlHsfA3: Solanum lycopersicum (NP_001234854.1); OsHsfA3: Oryza sativa Japonica Group (XP_015623061.1); ZmHsfA3: Zea mays (NP_001147968.1); SiHsfA3: Setaria italica (XP_004952622.1); BdHsfA3: Brachypodium distachyon (XP_003575055.1); HvHsfA3: Hordeum vulgare (AEB26588.1).
2.3 StHsfA3过表达载体的构建及转基因马铃薯植株的获得
将克隆获得的StHsfA3基因目的片段通过Xho I和Mlu I双酶切后插入到pBA002表达载体35S启动子, 构建成35S::StHsfA3过表达载体(图5-A), 并转化农杆菌GV3101。利用利福平(rifampicin, Rif)和壮观霉素(spectinomycin, Spec)筛选出含有过表达载体的农杆菌阳性菌落, 并用PCR验证。提取农杆菌质粒, 经限制性内切酶Xho I和Mlu I双酶切后, 除了载体片段, 还得到1条长度为1506 bp的目的片段(图5-B), 说明过表达载体已成功构建。图5
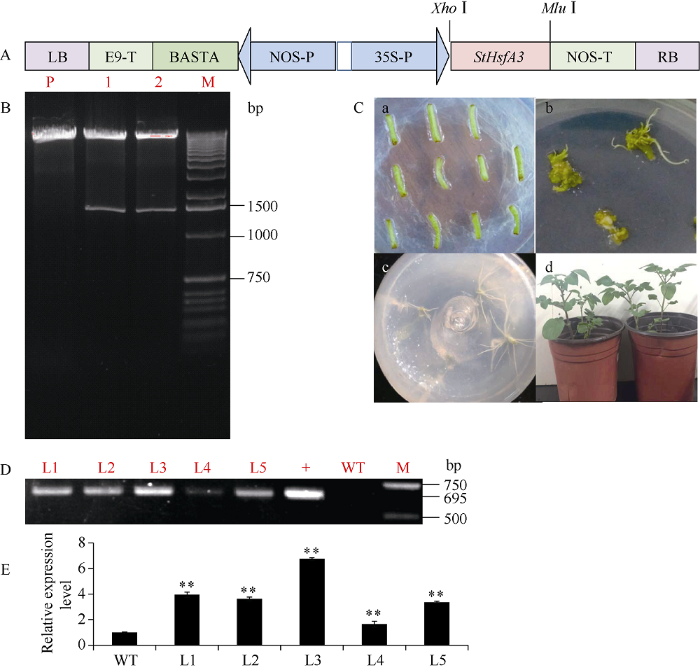
图5StHsfA3过表达载体的结构示意图(A)、过表达载体的酶切验证(B)、转基因马铃薯植株的再生(C)、StHsfA3过表达转基因植株的PCR检测(D)、不同植株中StHsfA3表达量的qRT-PCR检测(E)
A: 带有酶切位点的35S::StHsfA3载体示意图。B: M: 1 kb plus DNA marker; P: 含有pBA002-StHsfA3载体的农杆菌质粒; 1, 2: 含有pBA002-StHsfA3载体的农杆菌质粒酶切结果。C: a. 预培养; b. 芽分化; c. 生根筛选; d. 盆栽苗。D: M: DNA 2000 marker; WT: 非转基因植株, 作为阴性对照; +: pBA002-StHsfA3农杆菌质粒, 作为阳性对照; L1~L5: 转基因株系。E: 不同植株中StHsfA3的表达量测定。**表示在0.01水平差异显著。
Fig. 5Vector construction of 35S::StHsfA3 (A), enzyme digestion identification of 35S::StHsfA3 vector (B), regeneration of transgenic potato (C), PCR identification of StHsfA3 overexpression transgenic plant lines (D), and qRT-PCR determination of StHsfA3 in WT and StHsfA3 overexpression transgenic plant lines (E)
Schematic diagram of 35S::StHsfA3 vector with site of restriction enzymes in Fig. A. M: 1 kb plus DNA marker; P: pBA002-StHsfA3 plasmid; 1, 2: enzyme digestion identification of pBA002-StHsfA3 plasmid in Fig. B. a: preincubation in darkness; b: bud formation; c: selection of transgenic lines; d: plants in pot in Fig. C. M: DNA 2000 marker; WT: non-transgenic potato plant, used as a negative control; +: pBA002-StHsfA3 plasmid, used as a positive control; L1-L5: Transgenic lines in Fig. D. The expression levels of StHsfA3 were determined in different plants in Fig. E. **: significantly different at the 0.01 probability level.
将含有过表达载体的农杆菌菌液侵染马铃薯组培苗茎段, 经预培养、共培养、诱导愈伤、芽分化以及草丁膦生根筛选(图5-C), 初步得到12株抗性植株。提取其叶片DNA, 利用引物P33进行PCR验证, 最终鉴定得到5个StHsfA3的过表达马铃薯转基因株系(图5-D)。荧光定量结果显示, StHsfA3在过表达马铃薯转基因株系中的表达量显著高于非转基因植株(图5-E)。
2.4 转基因马铃薯植株的相对含水量(RWC)和丙二醛(MDA)含量分析
相对含水量和丙二醛含量是反应植物在逆境状态下生长状况的重要生理指标, 通常可用于鉴定植物抗逆境胁迫的能力。通过检测非转基因植株(WT)和转基因植株(L)在适温(22℃)和高温(35℃)条件下叶片中的相对含水量发现, 在适温条件下, 马铃薯叶片中的相对含水量均保持在80%以上(图6-A)。当热处理8 h之后, 不论是转基因植株还是非转基因植株, 其叶片的相对含水量都因叶片受到热胁迫而呈现不同程度的下降, 而且热处理后转基因马铃薯叶片中的相对含水量仍然显著高于非转基因植株, 表明StHsfA3过表达转基因植株比非转基因植株在热胁迫下的保水能力更强, 因此耐热性更高。图6
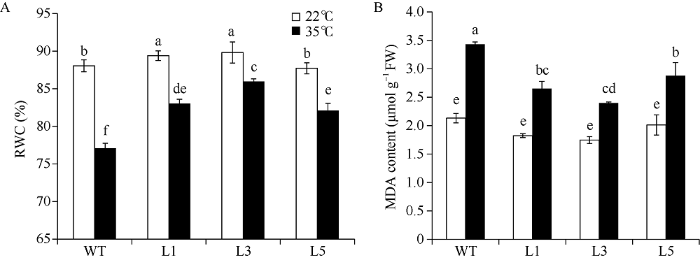
图6不同温度处理下非转基因植株与StHsfA3过表达转基因植株叶片中的相对含水量(A)和丙二醛含量(B)
WT: 非转基因植株; L: StHsfA3过表达转基因株系(L1、L3、L5三个株系); RWC: 相对含水量; MDA content: 丙二醛含量; 正常生长温度: 22℃; 高温胁迫: 35℃; 处理时间: 8 h; 图中的值是3个独立试验的结果, 每个试验3个重复, 以平均值±标准误表示。根据Duncan’s多重测试, 柱形图上不同小写字母表示P < 0.05的显著性差异水平。
Fig. 6RWC (A) and MDA (B) content in the leaves of non-transgenic plants and StHsfA3 overexpression transgenic plants under different temperature treatments
WT: non-transgenic plants; L: StHsfA3 overexpression transgenic plant lines; RWC: relative water content; MDA: malondialdehyde; temperature treatments: 22℃ (optimal temperature) and 35℃ (heat stress) for eight hours. Values are means ± SE of three independent experiments with three replicates in each experiment. Bars with different lowercase letters are significantly different at P < 0.05 according to Duncan’s multiple range tests.
对转基因马铃薯植株和非转基因植株在35℃高温胁迫下和22℃适温条件下叶片中MDA含量的检测(图6-B)发现, 热处理8 h后,植株叶片中的MDA含量均升高, 说明它们都受到了热胁迫引起的膜脂过氧化, 但转基因各株系叶片中的MDA含量显著低于非转基因植株, 说明StHsfA3过表达植株受到热胁迫导致的膜脂过氧化程度低于普通非转基因马铃薯, 因此, StHsfA3过表达转基因植株比非转基因植株耐热性强。
2.5 StHsfA3、StHsp26-CP以及StHsp70的表达量分析
利用qRT-PCR的方法, 本研究检测了适温(22℃)和高温(35℃)条件下非转基因植株和3个转基因株系叶片中StHsfA3的表达水平(图7-A)。在适温条件下, 转基因株系中StHsfA3的表达量均显著高于非转基因植株, L1、L3、L5中StHsfA3的表达量分别是非转基因植株中的3.95、7.05和3.36倍。在高温处理2 h后, 各植株中StHsfA3的表达量均受高温诱导而上升, 且3个转基因株系中该基因的表达量都显著高于非转基因植株。图7
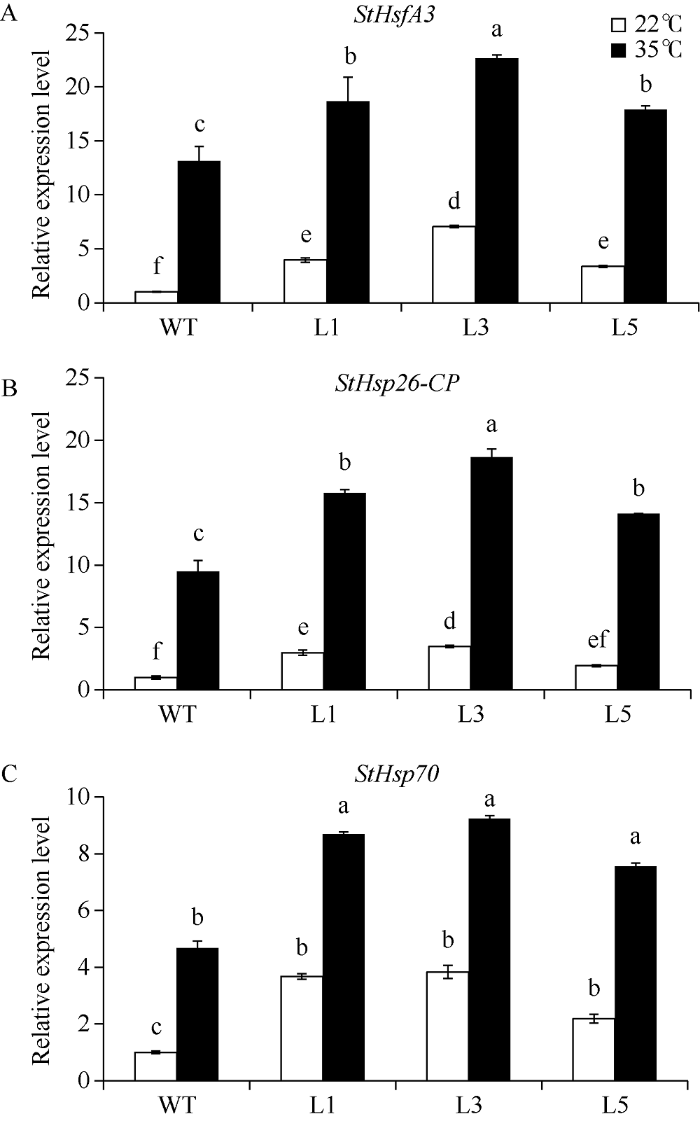
图7不同温度处理下非转基因植株(WT)和转基因植株(L)叶片中StHsfA3 (A)、StHsp26-CP (B)和StHsp70 (C)的表达水平
WT: 非转基因植株; L: StHsfA3过表达转基因株系(L1、L3、L5三个株系); 正常生长温度: 22℃; 高温胁迫: 35℃; 处理时间: 2 h; 图中的值是2次试验重复的结果, 每个试验3个生物学重复, 以平均值±标准误表示。根据邓肯多重测试, 柱形图上不同小写字母表示P < 0.05的显著性差异水平。
Fig. 7Expression levels of StHsfA3 (A), StHsp26-CP (B), and StHsp70 (C) in the leaves of non-transgenic plants (WT) and transgenic plant lines (L) under different temperature treatments
WT: non-transgenic plants; L: StHsfA3 overexpression transgenic plant lines; temperature treatments: 22℃ (optimal temperature) and 35℃ (heat stress) for two hours. Values are means ± SE of two experimental replicates with three biological replicates in each experiment. Bars with different lowercase letters are significantly different at P < 0.05 according to Duncan’s multiple range tests.
在正常生长条件下, StHsfA3过表达转基因植株中StHsp26-CP和StHsp70的表达量均显著高于非转基因植株中, 说明StHsfA3过表达诱导了StHsp26-CP和StHsp70在转基因植株中的表达, 但Hsp26-CP和StHsp70是否是StHsfA3的靶基因, 是否被StHsfA3直接调控, 还需要进一步研究。当35℃处理2 h后, 所有植株中StHsp26-CP和StHsp70的表达量都在高温诱导下显著上升, 且各转基因株系中这2个基因的表达量显著高于非转基因植株(图7-B, C)。
3 讨论
马铃薯地上部分生长的最适温度为20~25℃, 块茎形成的最适温度为15~20℃[23,24]。高温抑制马铃薯块茎的形成和发育, 从而严重影响营养物质的积累。因此马铃薯耐热基因的开发和耐热机制的研究对提高马铃薯的抗热性具有重要意义。热激转录因子(Hsf)广泛存在于各植物中, 在热胁迫应答方面具有十分重要的作用。随着马铃薯全基因组测序工作的完成, 课题组前期已鉴定出27个马铃薯Hsf基因家族成员。其中, StHsfA3介导了一系列高温胁迫响应基因的转录调控, 包括Hsps以及一些其他的功能基因, 在植物的热响应调控过程中发挥重要作用[15]。
本研究从马铃薯品种Désirée中克隆得到StHsfA3基因。序列分析显示, StHsfA3的cDNA长度为1506 bp, 编码501个氨基酸(图1)。大多数A族Hsf具有1个包含氨基酸激活序列(AHA)的C端激活域(C-terminalactivation domain, CTAD)[25]。StHsfA3虽然属于HsfA家族成员, 但在其CTAD区域不含AHA基序, 而是含有4个中心是色氨酸残基的短肽基序(图1-B)。该结构在番茄HsfA3中也有发现, 它与AHA功能相似, 使得HsfA3具有高效的转录激活功能[16]。经研究证实, 不含AHA基序的HsfA可与其他HsfA成员结合形成异聚体, 从而激活其转录活性[10,26]。StHsfA3蛋白的二级结构预测显示, 无规则
卷曲所占比例最高(图2-A), 它们构成了该蛋白的活性中心, 激活了Hsf三聚体的形成, 促进了该三聚体与Hsp基因启动子区的有效结合[5,6]。其DBD结构域的三级结构模型含有3个α螺旋和4个β折叠片(图2-B)。α螺旋和β折叠片一般作为蛋白质的支架, 因此这部分构成该蛋白最保守的结构域。生物信息学软件预测到StHsfA3是无跨膜结构域和信号肽的亲水性蛋白, 表明它既不是分泌蛋白也不是膜蛋白, 这与其在细胞中的位置以及发挥的功能相适应。马铃薯HsfA3的氨基酸序列与番茄、辣椒和烟草这些茄科植物的亲缘关系比较近, 尤其与番茄的HsfA3亲缘关系最近, 表明马铃薯HsfA3可能与番茄HsfA3具有相似的功能和作用机制。
为了研究HsfA3基因的功能, 本研究通过农杆菌介导法将该基因转入马铃薯植株, 最终获得了5个独立的HsfA3过表达转基因株系。在我们验证的3个转基因株系中, StHsfA3的表达量均显著高于非转基因植株, 表明StHsfA3基因在强启动子CaMV 35S带动下高效表达[27]。然而在这3个转基因株系中(L1、L3和L5), StHsfA3的表达量存在差异, 在L3中表达量最高, 在L5中表达量最低, 这可能是与目的基因插入的位点和在马铃薯中的拷贝数不同有关[27]。相对含水量(RWC)反映了植物的保水能力, RWC越小, 说明水分亏缺越严重, 对逆境的耐受性越弱[28]。在高温胁迫下, 活性氧的积累导致植物生物膜脂发生过氧化产生丙二醛(MDA), 因此MDA含量越高, 说明植物生物膜受伤害程度越深, 对逆境的耐受性越弱[29]。因此, 这2个指标侧面反映植物耐热性的强弱。本研究通过检测各植株在高温处理后叶片中的RWC和MDA含量的变化发现, 高温处理后转基因植株中的RWC显著高于非转基因植株, MDA含量显著低于非转基因植株。这说明StHsfA3过表达转基因植株比非转基因植株在热胁迫下的保水能力更强, 受到热胁迫导致的膜脂过氧化程度更低, 预示马铃薯植株内StHsfA3过表达提高了马铃薯植株的耐热性。
在前期研究中, 通过构建StHsfs共表达网络发现, StHsp26-CP、StHsp70与StHsfA3处于同一个共表达模块中, 表明StHsp26-CP和StHsp70可能是StHsfA3的共表达基因[15]。本研究发现, 在正常条件下生长时, StHsfA3过表达马铃薯转基因株系中StHsp26-CP和StHsp70的表达量均显著高于非转基因植株, 说明StHsfA3在马铃薯植株中的过表达诱导了StHsp26-CP和StHsp70表达。当受到热胁迫后, 转基因植株中StHsfA3受高温诱导而大量表达, 与此同时, StHsp26-CP和StHsp70也呈现出与StHsfA3表达量变化一致的趋势。这说明StHsfA3对StHsp26-CP和StHsp70表达起到一定的正调控作用, 与我们前期的研究结果相一致。StHsp26-CP属于叶绿体sHsp家族。研究发现, 拟南芥中的叶绿体sHsp (AtHsp21)能够与一种质体核蛋白pTAC5 (plastid transcriptionally active 5)结合, 来维持质体编码RNA聚合酶(plastid-encoded RNA polymerase, PEP)的功能, 从而保护叶绿体[30]。马铃薯StHsp26-CP是否具有与AtHsp21相似的作用, 还需要进一步的试验验证。Hsp70是一类结构最保守、分布最广泛的热激蛋白。它能够帮助蛋白质正确折叠, 或者将蛋白聚合物降解, 最后将其转运到细胞外, 从而减轻热胁迫对细胞和组织的破坏[31]。
类似地, 在拟南芥中也发现一些Hsp基因表达与HsfA3的丰度有密切的关系。Sakuma等[32]通过在拟南芥中过表达AtHsfA3的上游基因AtDREB2A CA发现, AtHsfA3和3个Hsp基因(Hsp18.1-CI、Hsp26-MII和Hsp70)的表达量均显著上升。随后, Yoshida等[17]进一步证明, 热胁迫下拟南芥HsfA3能够直接被DREB2A调控而上调表达, 从而产生一系列Hsps等热诱导基因的级联表达, 如Hsp70b、Hsp19.9-P和Hsp22.0。然而, Hsf介导的转录调控是一个非常复杂的过程, 往往是不同的Hsf分子共同作用的结果。如Li等[33]研究表明, 拟南芥中HsfA2和HsfA3在相同的耐热调控途径中发挥作用, 且它们在蛋白水平上能够互作, 从而诱导下游耐热基因大量表达。因此, 在马铃薯中StHsp26-CP和StHsp70是否是作为StHsfA3的靶基因而受到StHsfA3直接调控, 还需进一步试验验证。
4 结论
本研究从马铃薯品种Désirée中克隆得到StHsfA3基因, 该基因cDNA全长为1506 bp, 编码501个氨基酸。该蛋白的相对分子量为55.23 kD, 理论等电点为4.9, 无跨膜结构和信号肽, 属于亲水性蛋白。StHsfA3与茄科植物番茄HsfA3的亲缘关系最近。通过农杆菌介导的遗传转化法共得到5个StHsfA3过表达转基因马铃薯株系。StHsfA3过表达增强了植株的相对含水量, 降低了植株的MDA含量, 表明StHsfA3基因在马铃薯响应热胁迫过程中起正调控作用。StHsfA3过量表达诱导了StHsp26-CP和StHsp70表达, 推测StHsfA3可能协同StHsp26-CP和StHsp70增强过表达转基因株系的耐热性。附图1
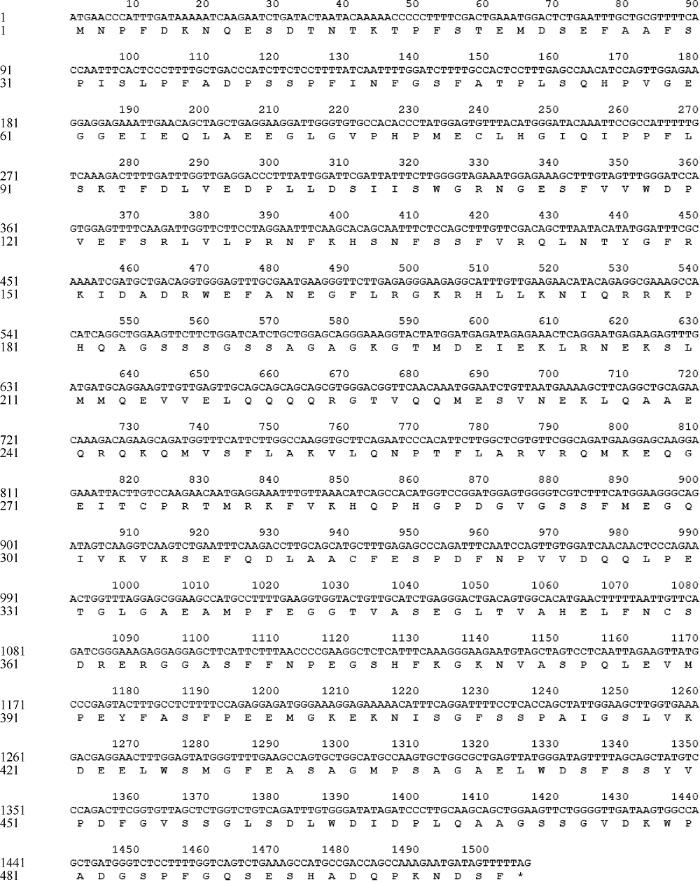
附图1马铃薯HsfA3基因的cDNA序列和对应的氨基酸序列
Fig. S1cDNA sequence of potato HscfA3 and corresponding amino acid sequence
参考文献 原文顺序
文献年度倒序
文中引用次数倒序
被引期刊影响因子
[本文引用: 2]
[本文引用: 1]
[本文引用: 1]
DOI:10.1016/j.pbi.2007.04.011URLPMID:17482504 [本文引用: 1]

Plants have evolved a variety of responses to elevated temperatures that minimize damage and ensure protection of cellular homeostasis. New information about the structure and function of heat stress proteins and molecular chaperones has become available. At the same time, transcriptome analysis of Arabidopsis has revealed the involvement of factors other than classical heat stress responsive genes in thermotolerance. Recent reports suggest that both plant hormones and reactive oxygen species also contribute to heat stress signaling. Additionally, an increasing number of mutants that have altered thermotolerance have extended our understanding of the complexity of the heat stress response in plants.
[本文引用: 3]
[本文引用: 2]
[本文引用: 2]
[本文引用: 1]
[本文引用: 1]
URLPMID:18407058 [本文引用: 2]
[本文引用: 1]
[本文引用: 1]
[本文引用: 1]
[本文引用: 1]
URLPMID:27148315 [本文引用: 5]
[本文引用: 2]
[本文引用: 2]
[本文引用: 1]
[本文引用: 1]
URLPMID:29394377 [本文引用: 1]
DOI:10.1111/jipb.12136URLPMID:24304603 [本文引用: 1]

Anthocyanins are a class of products of plant secondary metabolism and are responsible for tubers color in potato. The biosynthesis of anthocyanins is a complex biological process, in which multiple genes are involved including structural genes and regulatory genes. In this study, StAN11, a WD40-repeat gene, was cloned from potato cultivar Chieftain (Solanum tuberosum L.). StAN11 (HQ599506) contained no intron and its open reading frame (ORF) was 1,029 bp long, encoding a putative protein of 342 amino acids. In order to verify its role in anthocyanin biosynthesis, StAN11 was inserted behind the CaMV-35S promoter of pCMBIA1304 and the recombination vector was introduced into the potato cultivar Desiree plants by Agrobacterium-mediated transformation. The color of transgenic tuber skin was significantly deepened, compared to the wild-type control, which was highly consistent with the accumulation of anthocyanin and expression of StAN11 in transgenic lines tuber skin. Further analysis on the expression of Flavonone-3-hydroxylase (F3H), Dihydroflavonol reductase (DFR), Anthocyanidin synthase (ANS), and Flavonoid 3-O-glucosyl transferase (3GT) in transgenic plants revealed that only DFR was upregulated. This result suggested that StAN11 regulated anthocyanin biosynthesis in potato by controlling DFR expression and accumulation of anthocyanin could be increased through overexpression of StAN11 in the tubers with the genetic background of anthocyanin biosynthesis.
[本文引用: 1]
[本文引用: 1]
[本文引用: 1]
[本文引用: 1]
URLPMID:25416131 [本文引用: 1]
DOI:10.1046/j.1467-7652.2003.00029.xURLPMID:17166131 [本文引用: 2]

Mycoparasitic fungi are proving to be rich sources of antifungal genes that can be utilized to genetically engineer important crops for resistance against fungal pathogens. We have transformed cotton and tobacco plants with a cDNA clone encoding a 42 kDa endochitinase from the mycoparasitic fungus, Trichoderma virens. Plants from 82 independently transformed callus lines of cotton were regenerated and analysed for transgene expression. Several primary transformants were identified with endochitinase activities that were significantly higher than the control values. Transgene integration and expression was confirmed by Southern and Northern blot analyses, respectively. The transgenic endochitinase activities were examined in the leaves of transgenic tobacco as well as in the leaves, roots, hypocotyls and seeds of transgenic cotton. Transgenic plants with elevated endochitinase activities also showed the expected 42 kDa endochitinase band in fluorescence, gel-based assays performed with the leaf extracts in both species. Homozygous T2 plants of the high endochitinase-expressing cotton lines were tested for disease resistance against a soil-borne pathogen, Rhizoctonia solani and a foliar pathogen, Alternaria alternata. Transgenic cotton plants showed significant resistance to both pathogens.
[本文引用: 1]
[本文引用: 1]
[本文引用: 1]
[本文引用: 1]
URLPMID:16678092 [本文引用: 1]
[本文引用: 1]
[本文引用: 1]