
Response of HSP20 Genes to Artificial Aging Treatment in Maize Embryo
XING Lu-Man, LYU Wei-Zeng, LEI Wei, LIANG Yu-Huan, LU Yang, CHEN Jun-Ying
通讯作者:
第一联系人:
收稿日期:2018-03-20接受日期:2018-07-20网络出版日期:2018-07-30
基金资助: |
Received:2018-03-20Accepted:2018-07-20Online:2018-07-30
Fund supported: |

摘要
关键词:
Abstract
Keywords:
PDF (480KB)元数据多维度评价相关文章导出EndNote|Ris|Bibtex收藏本文
本文引用格式
邢芦蔓, 吕伟增, 雷薇, 梁雨欢, 卢洋, 陈军营. 玉米种胚HSP20基因对人工老化处理的响应[J]. 作物学报, 2018, 44(11): 1733-1742. doi:10.3724/SP.J.1006.2018.01733
XING Lu-Man, LYU Wei-Zeng, LEI Wei, LIANG Yu-Huan, LU Yang, CHEN Jun-Ying.
热激蛋白(heat shock protein, HSP)又称热休克蛋白或应激蛋白, 是细胞或生物体在受到高温等逆境刺激后新合成的或合成量增加的一类蛋白质[1], 在生物体的抗逆反应中起着重要的作用。胁迫条件下, HSP具有稳定细胞结构、维持细胞正常生理功能和提高机体对胁迫的适应性的功能[2]。HSP按其分子量可被分为HSP100、HSP90、HSP70、HSP60和小分子热激蛋白(sHSP) 5种类型[3]。sHSP的分子量介于12~43 kD[4,5], 含有可变的N-末端和C-末端和约90个氨基酸的高度保守区域(ACD结构域)[6]。根据氨基酸序列、细胞定位及免疫学分析, 植物sHSP至少可被分为6类[7], 3类 sHSP (称之为CI、CII、CIII)定位于细胞质或细胞核[8,9], 另外3类分别定位于质体[10]、内质网[8]和线粒体, 称之为CIV、CV和CVI[2,9,11]。植物所含小热激蛋白中, HSP20家族表达量最高[9]。在细胞内sHSP能够阻止蛋白变性, 保持细胞正常的生理活性, 使细胞免受热胁迫伤害。sHSP能够正确识别细胞内某些结构和功能发生改变的蛋白质并与之结合形成sHSP-底物复合物, 阻止其发生不可逆的聚集[12,13]。向日葵热激转录因子HaHSFA9 在烟草中的过表达, 提高CI、CII类sHSP的表达量, 从而增强种子活力并延长种子寿命[14]。高温胁迫下, CaHSP20的产生, 可以减轻辣椒热损伤[15]。莲NaHSP17.5基因在拟南芥种子中的过表达, 提高拟南芥种子的发芽率及耐高温能力[16]。水稻种子中, OsHSP18.2蛋白可以限制ROS的积累, 使蛋白质免遭不可逆的破坏, 维持种子活力[17]。过表达小麦sHSP26基因, 也可以提高拟南芥的耐高温能力及种子活力[18]。Muthusamya等[19]检测到163个小麦TaHSP20基因, 这些基因在对生物和非生物胁迫的应激反应中起着重要作用。玉米ZmHSP16.9基因在烟草中过表达增强了烟草植株耐高温以及抗氧化胁迫的能力, 提高了种子的发芽率及叶片中抗氧化酶的活性[20]。过表达ZmHSP17.7基因的拟南芥在种子萌发和植株生长过程中表现出更强的高温和干旱耐受性[21]。在玉米幼苗期, 热胁迫诱导叶片中sHSP26基因的表达, 可保护玉米叶绿体结构免受热胁迫的损坏[22]。
HSP20蛋白响应生物和非生物胁迫的研究较多, 在玉米上也有关于HSP20基因功能的相关报道[20,21,22], 而玉米种胚中HSP20基因在老化过程中的表现则未见报道。本研究利用转录组技术筛选出人工老化处理前后种胚中25个差异表达的HSP20基因进行分析, 以期为揭示玉米种子衰老的分子机制提供依据。
1 材料与方法
1.1 材料及其处理
以玉米杂交种郑单 958 种子为材料, 参照Basak等[23]人工老化处理方法, 稍加修改。取整齐一致的完整种子置老化网上, 在高温(45°C)、高湿(相对湿度100%)的人工老化箱内分别处理1、2、3、4、5 d, 并于室内阴凉处风干备用, 以未老化处理的种子为对照。1.2 种子活力测定
玉米发芽试验参考Zhang等[24]的方法, 略有改动。先将河沙过0.8 mm孔径的土壤筛并洗净, 置130°C烘箱烘干; 加入适量水, 搅拌均匀并分装到发芽盒中, 取每个处理20粒种子置发芽盒中, 重复3次, 将发芽盒置光照培养箱中, 设置温度28°C, 相对湿度70%, 光照强度13 mmol m-2 s-1及16 h/8 h的光照/黑暗交替, 培养1周, 记录每天的发芽数并测量芽(或苗)长, 按下列公式计算发芽率、发芽指数、活力指数和平均发芽日数。发芽率(GR) = G7/N×100%, 式中, G7为第7天的总发芽数, N为供试种子总数;
发芽指数(GI) = ∑(Gt/Dt), 式中, Dt为发芽日数, Gt为与Dt相对应的每天发芽种子数;
活力指数(VI) = GI×S, 式中, S为一定时期内正常的幼苗长度(cm);
平均发芽日数(MLIT) = ∑(Gt×Dt)/∑Gt。
1.3 生理指标测定
1.3.1 种胚中H2O2含量的测定 分别剥取人工老化处理1~5 d 玉米种子的种胚, 以未老化处理的种子为对照, 按照Jana等[25]的方法, 测定各处理种胚中过氧化氢(H2O2)含量, 3次重复。1.3.2 过氧化氢酶活性的测定 采用分光光度法, 并稍作修改, 测定各处理种胚中过氧化氢酶(CAT)活性, 3次重复[26]。
1.3.3 丙二醛(MDA)含量的测定 利用硫代巴比妥酸法测定各处理种胚中丙二醛(MDA)含量[26]。
1.4 HSP20差异表达基因的筛选和表达分析
1.4.1 总RNA的提取、转录组测序以及HSP20差异基因的筛选 以未老化处理和老化3 d的玉米种胚为材料, 按 TransZol Plant试剂盒(全式金公司, 北京)使用说明提取RNA, 通过琼脂糖凝胶电泳检测其完整性, -80°C储藏备用。RNA-seq由华大基因公司完成。参照曹广灿等[27]的方法进行转录组测序以及HSP20差异基因的筛选。1.4.2 HSP20基因的生物信息学分析 通过NCBI (http://www.ncbi.nlm.nih.gov/)和MaizeGDB (http://www. maizegdb.org/)网站对玉米基因表达谱检测结果进行初步注释, 未被注释上的基因用Blastn进行核酸序列比对, 获得一致性较高的序列, 并进行注释。用ESLpred软件(http://crdd.osdd.net/cgibin/eslpred/eslpred.pl)和ProtComp 9.0软件(http://www.softberry.com/berry.phtml?topic= protcompan&group=programs&subgroup=proloc)预测筛选出来的HSP20蛋白的亚细胞定位。将筛选出来基因的氨基酸序列用Conserved Domain Database工具进行蛋白质保守结构域的分析。
1.5 HSP20基因表达的qRT-PCR分析
用Primer5.0软件设计荧光定量PCR引物(附表1)。以玉米基因 Actin1 (NM_001155179.1)为内参, 利用qRT-PCR技术分析其在人工老化过程中的表达模式。从每个RNA样品取 1 μg反转录为cDNA模板, 稀释10倍备用。使用 Thermo Scientific Maxima SYBR Green qPCR Master Mix (2×), ROX Solution provided试剂盒的反应体系, 采用荧光定量PCR仪(Bio-Rad)进行扩增反应。PCR条件为95°C 3 min; 95°C 30 s, 59°C 30 s, 72°C 30 s, 40个循环; 72°C 4 min, 12°C保存。Supplementary table 1
附表1
附表1HAP20S基因表达分析的引物
Supplementary table 1
基因ID号 Gene ID | 引物序列 Primer sequence (5'-3') | 退火温度 Annealing temperature (℃) | 产物长度 Product length (bp) | |
---|---|---|---|---|
GRMZM2G049767 | F | GCTGTACTACAGGTAGTATAAC | 59 | 105 |
R | GCACAACACTTCCATACA | 59 | ||
GRMZM2G306679 | F | TAGTGATTATCCGTTATGTACTC | 59 | 141 |
R | ACAGAACAGATTGTATTAGGTT | 59 | ||
GRMZM2G479260 | F | GTCTTCGTCTTAGTGTTGAT | 58.9 | 109 |
R | TACTCAAGACAGCCATGA | 59 | ||
GRMZM2G375517 | F | AGATGTGAATGTGATCTGAC | 59 | 116 |
R | AGTACATATTAACTCACGCAA | 58.9 | ||
GRMZM5G858128 | F | TCTAACAGAACTCGCTGA | 59 | 179 |
R | CATCATCATACCACCGATC | 59.1 | ||
GRMZM2G346839 | F | CATCATCATACCACCGATC | 59.1 | 179 |
R | TCTAACAGAACTCGCTGA | 59 | ||
GRMZM2G335242 | F | GTACGTCTGAATTCTGGTC | 59.1 | 98 |
R | CTCACTCGGATTACTTGC | 58.9 | ||
GRMZM2G080724 | F | GATTCATCAGGACGATGAG | 59 | 101 |
R | AAGAAGAGTGGTAGCTAGAT | 58.9 | ||
GRMZM2G012455 | F | AGACCATCGAGATTAAGGT | 59 | 179 |
R | ACACATCAGGTAGGATGAT | 59 | ||
GRMZM2G311710 | F | ACACATCAGGTAGGATGAT | 59 | 179 |
R | AGACCATCGAGATTAAGGT | 59 | ||
GRMZM2G333635 | F | GAGGACAAGAACGACAAG | 59.1 | 154 |
R | TCTTCACCTCCGTCTTAG | 59.1 | ||
GRMZM2G158232 | F | TGAGGTGAAGGCTATTGA | 59 | 89 |
R | ATATCGTAGAAGACACAGGA | 59 | ||
GRMZM2G331701 | F | ATGACTCAATGACGGAGA | 59.1 | 90 |
R | GCATATACGCTGTCACAA | 58.9 | ||
GRMZM5G849535 | F | ATATCGTAGAAGACACAGGA | 59 | 110 |
R | GATATCTGGTTGAGCATCC | 59 | ||
GRMZM2G034157 | F | GGTATCACCAATCATCCTATC | 59.2 | 83 |
R | GTTACAAGAGACATGTAGAGAT | 58.9 | ||
GRMZM2G083810 | F | ACCAATTCCTATCTGATGTATC | 59 | 112 |
R | GTCAATGAAGACTACGGATTA | 59 | ||
GRMZM2G332512 | F | GCTTGTTGTGTTGGTTTC | 58.9 | 117 |
R | CCTTCCAGAAGAACAGAAG | 59 | ||
GRMZM2G404274 | F | ACACATCAGGTAGGATGAT | 59 | 179 |
R | AGACCATCGAGATTAAGGT | 59 | ||
GRMZM2G085934 | F | CGATGGACATAGTGGAGA | 59.2 | 113 |
R | CTTCATCACTAGCATCCG | 58.7 | ||
GRMZM2G149647 | F | GGTCTTTGTGTAGCACTAG | 59.1 | 109 |
R | ATAGCAGAATTGAAGCGTT | 59 | ||
GRMZM2G098167 | F | AGAGTAGCAGCAGTAGAAT | 59 | 154 |
R | CAGAGACACGATTGAATAATTC | 59 | ||
GRMZM2G046382 | F | GTCGTAATGTCGTAGGATG | 59 | 85 |
R | ATCCATACATAGATTCAGACAC | 59 | ||
AC208204.3_FGT006 | F | CAACGTCCTTCAGATCAG | 58.9 | 175 |
R | CACAGTGACTGTAAGCAC | 59.4 | ||
NM-001155179.1(Actin1) | F | GTATGAGCAAGGAGATCAC | 58.9 | 194 |
R | TTAGAAGCACTTCATGTGG | 59.0 |
新窗口打开|下载CSV
2 结果与分析
2.1 人工老化处理对玉米种子活力的影响
0~2 d的种子发芽率均为100%; 人工老化处理3 d后, 种子发芽率下降到86.67% (下降幅度为13.33%); 处理4 d后, 发芽率下降到66.67%; 处理5 d的种子发芽率下降到21.67%。发芽指数与活力指数的变化趋势同发芽率一样, 老化处理3 d后急剧下降, 老化5 d后最低。其平均发芽日数随着种子老化时间的延长逐渐增加, 发芽速率降低, 未经老化处理的种子平均发芽日数为2.17, 老化处理1 d的种子平均增加为0.36; 处理2 d后平均增加0.03; 处理3 d后增加0.30; 处理4 d后平均增加0.62; 处理5 d后, 平均发芽日数达到最大值4.11 (表1)。上述结果表明, 随着老化时间的延长, 种子活力逐渐降低, 老化3 d后种子活力急剧下降(图1)。Table 1
表1
表1人工老化处理对种子不同活力指标的影响
Table 1
处理时间 Treatment time | 发芽率 Germination rate (%) | 发芽指数 Germination index | 活力指数 Vigor index | 平均发芽日数 Mean days of germination (d) |
---|---|---|---|---|
0 d | 100±0.00 a | 9.44±0.25 a | 124.14±5.57 a | 2.17±0.08 b |
1 d | 100±0.00 a | 8.22±0.54 b | 100.07±4.93 b | 2.53±0.16 b |
2 d | 100±0.00 a | 8.11±0.25 b | 101.32±1.17 b | 2.56±0.08 b |
3 d | 86.67±0.06 b | 6.29±0.32 c | 75.73±10.45 c | 2.86±0.03 b |
4 d | 66.67±0.08 c | 3.96±0.50 d | 41.63±5.39 d | 3.48±0.11 ab |
5 d | 21.67±0.10 d | 1.11±0.42 e | 8.95±1.84 e | 4.11±1.02 a |
新窗口打开|下载CSV
图1
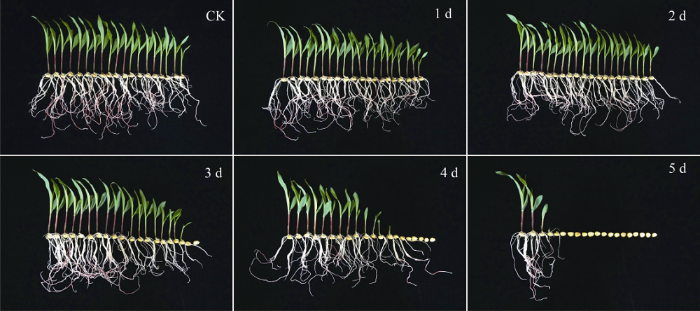
图1人工老化对种子活力的影响
Fig. 1Effect of artificial aging treatment on seed vigor
2.2 人工老化处理对玉米种胚生理特性的影响
为了研究人工老化过程种胚中ROS的积累情况以及对种胚的氧化损伤程度, 分别检测了不同老化时间内过氧化氢酶的活性、H2O2的含量及种胚中MDA的含量。随着老化时间的延长, 玉米种胚CAT 活性呈下降趋势, 未经老化处理的CAT活性为0.39 U g-1 FW min-1, 老化3 d后CAT活性显著下降到0.14 U g-1 FW min-1。H2O2含量表现为先升高后降低的趋势, 未经老化处理的H2O2含量为0.38 μmol g-1 FW, 人工老化处理3 d时达到峰值(0.51 μmol g-1 FW), 随后又逐渐降低。种胚中MDA含量逐渐增加, 人工老化处理3 d后急剧增加, 老化5 d时增幅最大, 为1.08 μmol g-1 FW (表2)。表明人工老化处理造成细胞膜的氧化损伤加剧, 使CAT活性下降、H2O2积累、膜脂氧化损伤加重。Table 2
表2
表2人工老化处理对种胚中不同生理指标的影响
Table 2
处理时间 Treatment time | 过氧化氢酶活性 CAT activity (U g-1 FW min-1) | 过氧化氢含量 H2O2 content (μmol g-1 FW) | 丙二醛含量 MDA content (μmol g-1 FW) |
---|---|---|---|
0 d | 0.39±0.11 a | 0.38±0.03 b | 0.16±0.11 c |
1 d | 0.26±0.13 a | 0.40±0.02 b | 0.18±0.03 c |
2 d | 0.18±0.12 ab | 0.42±0.02 b | 0.33±0.10 c |
3 d | 0.14±0.07 b | 0.51±0.06 a | 0.43±0.19 c |
4 d | 0.14±0.03 b | 0.43±0.02 ab | 0.87±0.26 b |
5 d | 0.06±0.02 b | 0.29±0.03 c | 1.955±0.21 a |
新窗口打开|下载CSV
2.3 人工老化处理后HSP20基因差异表达分析
利用转录组技术分析表明, 人工老化处理后玉米种胚中差异基因有4713个(上调2874个, 下调1839个)。利用KEGG分析方法对差异表达基因进行功能注释和分类表明, 可被KEGG 数据库注释的差异表达基因有2470个。其中HSP20蛋白相关基因有25个, 均表现为显著上调表达(3.10~8.84倍)。表3表明编码CI类小热激蛋白的基因有13个, 其中HSP18.3、HSP17.9、HSP17.8、HSP17.4、HSP15.7及HSP3存在于细胞质或细胞核中; GRMZM5G849535编码的分子量为16.9 kD的小热激蛋白属于类CIHSP1蛋白, 定位于线粒体中。GRMZM2G 311710等5个基因编码的HSP17.0、HSP17.6、HSP17.8属于CII类小热激蛋白, 定位在细胞质或细胞核中。GRMZM2G085934基因编码分子量为18.6 kD的热激蛋白属于CIII类热激蛋白, 该蛋白分布在细胞质中。GRMZM5G858128为22.0 kD CIV类热激蛋白基因, 该蛋白为线粒体蛋白; GRMZM2G149647编码的蛋白为HSP26, 分布在叶绿体中; GRMZM2G332512、GRMZM2G 404274编码的小热激蛋白分别为HSP18c、HSP18a, 均为核蛋白, 分布在细胞核上。GRMZM2G080724编码的逆转录转座子蛋白质, 该蛋白为线粒体蛋白, 也具有分子伴侣的功能。Table 3
表3
表3人工老化处理条件下HSP20相关基因及其注释
Table 3
基因ID Gene ID | log2比值 log2 Ratio | P值 P-value | 错误发现率 False discovery rate | 基因注释 Gene annotation | 亚细胞定位 Subcellular localization |
---|---|---|---|---|---|
GRMZM2G481605 | 8.84 | 1.89E-259 | 5.63E-257 | 17.9 kD class I heat shock protein | Nuclear |
GRMZM2G049767 | 8.19 | 1.07E-164 | 2.04E-162 | 17.9 kD class I heat shock protein | Nuclear |
GRMZM2G306679 | 7.37 | 0 | 0 | 17.9 kD class I heat shock protein | Nuclear |
GRMZM2G479260 | 6.71 | 0 | 0 | 17.9 kD class I heat shock protein | Nuclear |
GRMZM2G375517 | 6.70 | 0 | 0 | 17.8 kD class I heat shock protein | Cytoplasmic |
GRMZM5G858128 | 6.53 | 6.93E-222 | 1.83E-219 | 22.0 kD class IV heat shock protein | Mitochondria |
GRMZM2G306714 | 6.28 | 0 | 0 | Class I heat shock protein 3 | Nuclear |
GRMZM2G346839 | 5.97 | 7.04E-66 | 5.89E-64 | 18.3 kD class I heat shock protein | Cytoplasmic |
GRMZM2G335242 | 5.96 | 0 | 0 | 15.7 kD class I heat shock protein | Cytoplasmic |
GRMZM2G080724 | 5.61 | 0 | 0 | Retrotransposon protein [Zea mays] | Mitochondrial |
GRMZM2G012455 | 5.19 | 8.52E-135 | 1.34E-132 | 17.6 kD class II heat shock protein | Cytoplasmic |
GRMZM2G311710 | 4.98 | 0 | 0 | 17.0 kD class II heat shock protein | Nuclear |
GRMZM2G333635 | 4.48 | 0 | 0 | 17.9 kD class I heat shock protein | Nuclear |
GRMZM2G158232 | 4.33 | 0 | 0 | 17.9 kD class I heat shock protein | Nuclear |
GRMZM2G331701 | 4.31 | 0 | 0 | 18.3 kD class I heat shock | Cytoplasmic |
GRMZM5G849535 | 4.30 | 0 | 0 | 16.9 kD class I heat shock protein 1-like | Mitochondrial |
GRMZM2G034157 | 4.27 | 2.84E-22 | 8.22E-21 | 17.8 kD class II heat shock protein | Cytoplasmic |
GRMZM2G083810 | 4.18 | 0 | 0 | 17.8 kD class II heat shock protein | Cytoplasmic |
GRMZM2G332512 | 3.94 | 1.36E-70 | 1.22E-68 | Heat shock protein18c (hsp18c) | Nuclear |
GRMZM2G085934 | 3.88 | 3.28E-10 | 4.36E-09 | 18.6 kD class III heat shock protein | Cytoplasmic |
GRMZM2G404274 | 3.88 | 1.34E-197 | 3.16E-195 | 18.0 kD heat shock protein 18a | Nuclear |
GRMZM2G149647 | 3.52 | 0 | 0 | Hsp26 - heat shock protein 26 | Chloroplast |
GRMZM2G098167 | 3.49 | 0 | 0 | 17.0 kD class II heat shock protein | Cytoplasmic |
GRMZM2G046382 | 3.22 | 0 | 0 | 17.9 kD class I heat shock protein | Nuclear |
AC208204.3_FG006 | 3.10 | 0 | 0 | 17.4 kD class I heat shock protein | Nuclear |
新窗口打开|下载CSV
2.4 HSP20 ACD保守结构域分析
对25个HSP20基因编码的HSP20 ACD保守结构域分析发现, 有19个HSP20基因编码的蛋白序列中含有小热激蛋白特殊的α晶体结构域(alphacrystallin domain, ACD结构域)。ACD结构域由7~8个反相平行的β链折叠形成紧密的sandwich结构, 可分为CRI和CRII两个比较大的保守区域, 中间由一个可变长度的疏水区域β6-loop所隔开[7,9,28]。保守区CRI由β2 (DWKE)、β3 (AHVFKADL)、β4 (DVKVEV)、β5 (RVLRVSGE)组成, 保守区CRII由β6 (KWHRV)、β7 (ERSSGQFVRRFR)、β8 (VKAGLE)、β9 (VLTVTVP)组成(图2)。Siddique等[29]研究表明, ACD结构参与了底物相互作用, 对HSP20的分子伴侣功能有重要作用。图2
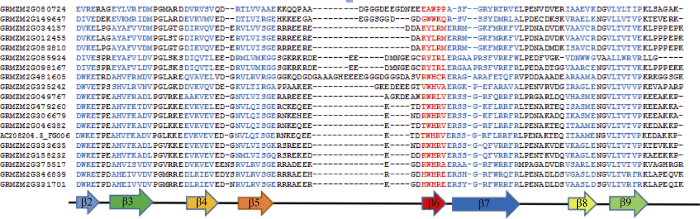
图2HSP20保守结构域
Fig. 2Conserved domain of HSP20
已有研究表明精氨酸(Arg)、赖氨酸(Lys)、脯氨酸(Pro)、苏氨酸(Thr)等氨基酸为氧化损伤的主要氨基酸残基[30]。对19个HSP20蛋白ACD结构域序列分析显示(表4), 该结构域由约90个氨基酸组成。不同HSP20蛋白ACD结构域中易氧化的氨基酸含量不同, 平均含有精氨酸(Arg) 11.2%, 赖氨酸(Lys) 7.2%, 脯氨酸(Pro) 4.2%, 苏氨酸(Thr) 3.9%。精氨酸平均含量最高, 其中GRMZM2G331701编码的ACD, 精氨酸含量最高(20.7%), 其次为GRMZM 2G375517、GRMZM2G346839编码的ACD, 其精氨酸含量分别为18.4%、18.3%; 对于赖氨酸, GRMZM2G333635、GRMZM2G158232编码的ACD中含量最高为12%; 脯氨酸的平均含量次之, 其中GRMZM2G335242编码的ACD, 脯氨酸含量最高为7.2%; 平均含量最低的为苏氨酸, 19个基因编码的ACD中, 苏氨酸含量最高的为GRMZM2 G479260 编码的ACD, 其含量为8.7%。由此推测, 在种子老化过程中, ROS的积累可能使HSP20蛋白结构域中的精氨酸(Arg)、赖氨酸(Lys)、脯氨酸(Pro)、苏氨酸(Thr)等氨基酸(特别是Arg、Lys)发生氧化, 造成HSP20蛋白 ACD结构破坏, 分子伴侣功能丧失, 导致种子活力下降。
Table 4
表4
表4ACD结构域序列分析
Table 4
基因ID Gene ID | ACD氨基酸数 Amino acid number | 个数/百分比Number/percentage (%) | |||
---|---|---|---|---|---|
精氨酸 Arg (R) | 赖氨酸 Lys (K) | 脯氨酸 Pro (P) | 苏氨酸 Thr (T) | ||
GRMZM2G481605 | 109 | 12/11.0 | 3/2.7 | 3/2.7 | 4/3.7 |
GRMZM2G049767 | 96 | 14/14.6 | 5/5.2 | 4/4.2 | 3/3.1 |
GRMZM2G306679 | 92 | 9/9.8 | 10/10.9 | 4/4.3 | 3/3.3 |
GRMZM2G479260 | 92 | 8/8.7 | 9/9.8 | 3/3.3 | 8/8.7 |
GRMZM2G375517 | 87 | 16/18.4 | 4/4.6 | 4/4.6 | 3/3.4 |
GRMZM2G346839 | 93 | 17/18.3 | 2/2.3 | 3/3.2 | 2/2.2 |
GRMZM2G335242 | 97 | 6/6.2 | 8/8.2 | 7/7.2 | 4/4.1 |
GRMZM2G080724 | 97 | 10/10.3 | 4/4.1 | 6/6.2 | 3/3.1 |
GRMZM2G012455 | 97 | 11/11.3 | 5/5.2 | 2/2.1 | 3/3.1 |
GRMZM2G333635 | 92 | 8/8.7 | 11/12 | 4/4.3 | 3/3.3 |
GRMZM2G158232 | 92 | 8/8.7 | 11/12 | 4/4.3 | 4/4.3 |
GRMZM2G331701 | 92 | 19/20.7 | 2/2.2 | 3/3.3 | 2/2.3 |
GRMZM2G034157 | 98 | 10/10.2 | 5/5.1 | 3/3.1 | 2/2.0 |
GRMZM2G083810 | 87 | 10/11.5 | 6/6.9 | 3/3.4 | 2/2.3 |
GRMZM2G085934 | 78 | 9/11.5 | 5/6.4 | 4/5.1 | 1/1.3 |
GRMZM2G149647 | 107 | 6/5.6 | 11/10.3 | 3/2.8 | 3/2.8 |
GRMZM2G098167 | 113 | 11/9.7 | 10/8.8 | 7/6.2 | 6/5.3 |
GRMZM2G046382 | 92 | 8/8.7 | 9/9.8 | 4/4.3 | 7/7.6 |
AC208204.3_FG006 | 92 | 8/8.7 | 9/9.8 | 4/4.3 | 7/7.6 |
平均值Mean | 95 | 11/11.2 | 7/7.2 | 4 /4.2 | 4/3.9 |
新窗口打开|下载CSV
2.5 HSP20基因表达模式分析
用qRT-PCR方法对23个差异表达的HSP20基因进行定量分析(见附图), 并挑选4个代表性的基因(包含细胞质HSP20基因、线粒体HSP20基因、叶绿体HSP20基因)表达模式分析(图3)表明, 4个HSP20相关基因的表达量随着老化时间的延长逐渐升高。其中细胞质HSP20蛋白基因GRMZM2G346839 (HSP18.3)的表达量在老化第4天明显升高, 老化5 d达最大值; 细胞质HSP20基因GRMZM 2G012455 (HSP17.6)表达量在老化第3 天显著增加, 老化3 d、4 d、5 d差异不显著; 叶绿体HSP20基因GRMZM2 G149647 (HSP26)、线粒体HSP20基因 GRMZM5G849535 (HSP16.9)表达量在老化第3 天明显升高, 且达到最大值, 随后又下调表达, 与老化过程中H2O2含量的变化趋势一致, 表明HSP20基因可能对阻止或缓解人工老化对种子活力有重要影响。附图1
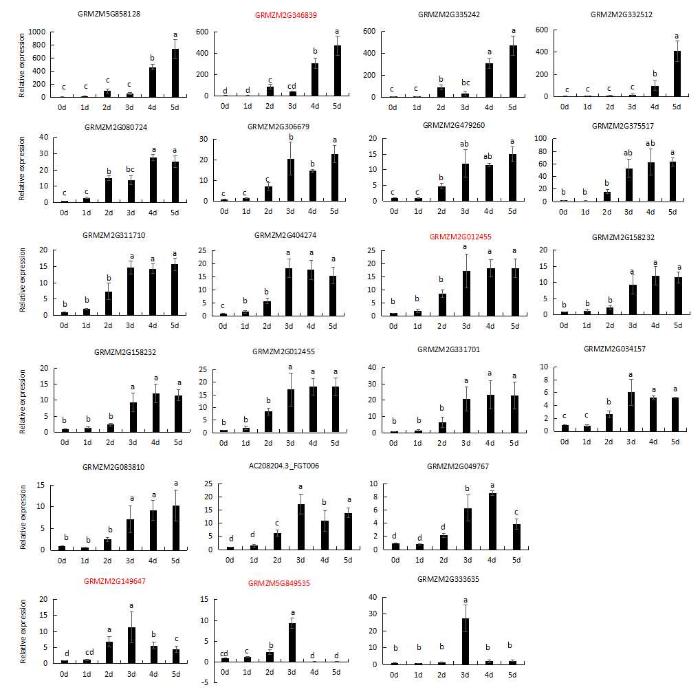
附图1HSP20s基因在不同老化时间内的表达
图中红色字体为文中挑选的4个基因。
Supplementary fig. 1The red word in the figure were the four selected genes in the article.
The red word in the figure were the four selected genes in the article.
图3
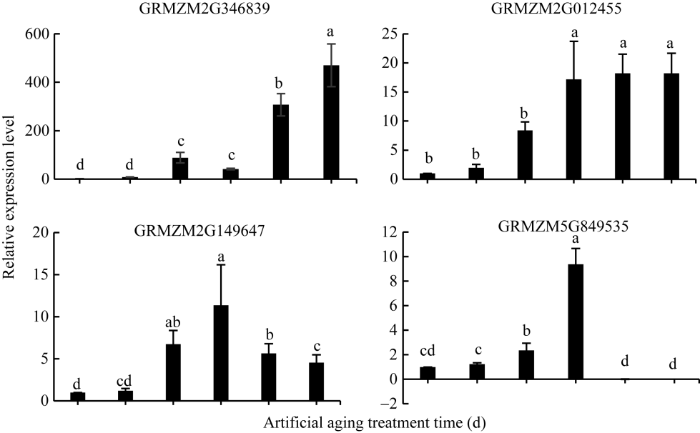
图3HSP20基因在不同老化时间内的表达
误差线上不同小写英文字母表示在0.05水平上的差异显著。
Fig. 3Expression patterns of HSP20 genes in different artificial aging treatment times
Bars superscripted by different letters are significantly different at the 0.05 probability level.
3 讨论
3.1 种子老化过程中ROS的积累可能是种子活力下降的主要原因
生物在有氧代谢过程中会产生ROS, 过量的ROS积累会造成细胞结构和大分子物质的伤害, 如蛋白质中含巯基的氨基酸易被氧化生成二聚体, 导致蛋白质的变性或失活。正常情况下, 高活力种子内的抗氧化系统可以清除细胞内多余的ROS。但随着储藏时间的延长, 种子内抗氧化体系作用能力下降, 种子内产生和积累的ROS引起膜质过氧化、蛋白质和酶变性等系列变化, 最终导致种子活力丧失[31,32,33]。Rajjou等[34]研究表明人工老化处理使种子中ROS的含量增加, 蛋白质错误折叠率升高, 从而蛋白质功能丧失, 种子生活力下降。本研究表明, 老化1~3 d的种子, 抗氧化酶(CAT)活性逐渐下降, 种胚中H2O2含量逐渐上升, 说明细胞内的抗氧化酶体系遭到破坏, ROS (H2O2)清除能力降低, 与前人研究结果一致。3.2 HSP20基因对人工老化处理的响应
植物细胞质sHSP蛋白可阻止热变性或化学变性的底物蛋白聚集, 并协助变性的底物蛋白质恢复活性[35]。叶绿体sHSP26蛋白能够在植物遇到氧化胁迫或热胁迫时保护光系统II (PSII)免受伤害[36]。水稻叶绿体OsHSP26基因在积极响应热胁迫的同时, 也受到H2O2的氧化胁迫[37]。包含部分保守的甲硫氨酸结构域的线粒体sHSP蛋白可能具有抗氧化作用, 在植物遇到氧化胁迫时, 能够保护电子传递体复合物I免受氧化损伤, 从而保护电子传递过程[38]。而Lund等[39]研究认为, 线粒体sHSP蛋白是影响植物线粒体耐热性的主要因子, 对大分子、细胞器具有保护功能。作为分子伴侣, 烟草CI小热激蛋白HSP18, 可以协助变性的蛋白质恢复活性[40], 西伯利亚野生黑麦(Elymus sibiricus L.), 小热激蛋白EsHSP16.9提高了生物体对高温、干旱、氧化等逆境的抗性[41]。拟南芥小热激蛋白AtHSP17.8在植株响应干旱及盐胁迫过程中起着至关重要的作用[42]。受到热胁迫的向日葵种子, 其HaHSP18.6基因表现上调表达, HaHSP18.6基因在向日葵响应热胁迫的过程中起着积极作用[43]。过表达小麦叶绿体TaHSP26基因的拟南芥, 可提高种子在高温胁迫下的发芽率[44]。本研究中的25个基因编码的蛋白大部分为细胞质、细胞核蛋白, 还有线粒体和叶绿体蛋白。qRT-PCR检测结果显示, 5个编码细胞质HSP20蛋白的基因(GRMZM2G346839、GRMZM2G335242、GRMZM2G012455、GRMZM2 G311710、GRMZM2G158232)其表达量随着老化时间的延长呈上升趋势, 而编码叶绿体HSP20蛋白的基因(GRMZM2 G149647)及编码线粒体HSP20蛋白的基因(GRMZM2G 158232)的表达量在老化第3天达到最大值, 随后降低。在玉米种子老化过程中, 编码叶绿体HSP26蛋白的基因及编码线粒体HSP20蛋白的基因的表达量与H2O2含量的变化趋势一致, 说明叶绿体HSP26基因和线粒体HSP20基因对维持种胚内ROS (H2O2)含量平衡有重要作用, 由此推测该基因对阻止或延缓种子衰老具有积极作用。不同基因的表达模式差异也反映了HSP20基因在玉米种子人工老化中的重要性。然而, HSP20蛋白在响应ROS产生和积累的同时, 也不可避免地遭受ROS的攻击和破坏。HSP20蛋白的ACD保守结构域有较高含量的Arg、Lys、Pro、Thr等容易氧化的氨基酸残基(表5)。这些氨基酸的氧化破坏了HSP20蛋白的结构, 影响其发挥相应的功能, 从而影响种子活力。如何量化HSP20基因对种子衰老的贡献将是我们下一步着手解决的科学问题。
参考文献 原文顺序
文献年度倒序
文中引用次数倒序
被引期刊影响因子
DOI:10.1016/j.jksus.2010.06.022URL [本文引用: 1]

Plants as sessile organisms are exposed to persistently changing stress factors. The primary stresses such as drought, salinity, cold and hot temperatures and chemicals are interconnected in their effects on plants. These factors cause damage to the plant cell and lead to secondary stresses such as osmotic and oxidative stresses. Plants cannot avoid the exposure to these factors but adapt morphologically and physiologically by some other mechanisms. Almost all stresses induce the production of a group of proteins called heat-shock proteins (Hsps) or stress-induced proteins. The induction of transcription of these proteins is a common phenomenon in all living things. These proteins are grouped in plants into five classes according to their approximate molecular weight: (1) Hsp100, (2) Hsp90, (3) Hsp70, (4) Hsp60 and (5) small heat-shock proteins (sHsps). Higher plants have at least 20 sHsps and there might be 40 kinds of these sHsps in one plant species. It is believed that this diversification of these proteins reflects an adaptation to tolerate the heat stress. Transcription of heat-shock protein genes is controlled by regulatory proteins called heat stress transcription factors (Hsfs). Plants show at least 21 Hsfs with each one having its role in regulation, but they also cooperate in all phases of periodical heat stress responses (triggering, maintenance and recovery). There are more than 52 plant species (including crop ones) that have been genetically engineered for different traits such as yield, herbicide and insecticide resistance and some metabolic changes.In conclusion, major heat-shock proteins have some kind of related roles in solving the problem of misfolding and aggregation, as well as their role as chaperones.
DOI:10.1007/BF00042230URL [本文引用: 2]
DOI:10.1038/29106URLPMID:9707123 [本文引用: 1]

The principal heat-shock proteins that have chaperone activity (that is, they protect newly made proteins from misfolding) belong to five conserved classes: HSP100, HSP90, HSP70, HSP60 and the small heat-shock proteins (sHSPs). The sHSPs can form large multimeric structures and have a wide range of cellular functions, including endowing cells with thermotolerance in vivo and being able to act as molecular chaperones in vitro; sHSPs do this by forming stable complexes with folding intermediates of their protein substrates. However, there is little information available about these structures or the mechanism by which substrates are protected from thermal denaturation by sHSPs. Here we report the crystal structure of a small heat-shock protein from Methanococcus jannaschii, a hyperthermophilic archaeon. The monomeric folding unit is a composite beta-sandwich in which one of the beta-strands comes from a neighbouring molecule. Twenty-four monomers form a hollow spherical complex of octahedral symmetry, with eight trigonal and six square 'windows'. The sphere has an outer diameter of 120 A and an inner diameter of 65 A.
DOI:10.1007/PL00012492URLPMID:12475175 [本文引用: 1]

Small Hsps (sHsps) encompass a widespread but diverse class of proteins. These low molecular mass proteins (15 42 kDa) form dynamic oligomeric structures ranging from 9 to 50 subunits. sHsps display chaperone function in vitro, and in addition they have been suggested to be involved in the inhibition of apoptosis, organisation of the cytoskeleton and establishing the refractive properties of the eye lens in the case of -crystallin. How these different functions can be explained by a common mechanism is unclear at present. However, as most of the observed phenomena involve nonnative protein, the repeatedly reported chaperone properties of sHsps seem to be of key importance for understanding their function. In contrast to other chaperone families, sHsps bind several nonnative proteins per oligomeric complex, thus representing the most efficient chaperone family in terms of the quantity of substrate binding. In some cases, the release of substrate proteins from the sHsp complex is achieved in cooperation with Hsp70 in an ATP-dependent reaction, suggesting that the role of sHsps in the network of chaperones is to create a reservoir of nonnative refoldable protein.
DOI:10.1007/s00239-004-0013-zURL [本文引用: 1]
[本文引用: 1]
DOI:10.1093/jxb/47.3.325URL [本文引用: 2]

The -crystallin-related, small heat shock proteins (smHSPs) are ubiquitous in nature, but are unusually abundant and diverse in higher plants as opposed to other eukaryotes. The smHSPs range in size from 17 to 30 kDa and share a conserved C-terminal domain common to all eukaryotic smHSPs and to the -crystallin proteins of the vertebrate eye lens. In higher plants six nuclear gene families encoding smHSPs have been defined. Each gene family encodes proteins found in a distinct cellular compartment, including the cytosol, chloroplast, ER, and mitochondrion. Evolutionary analysis suggests that the smHSP gene families arose by gene duplication and divergence prior to the radiation of angiosperms. In general, the smHSPs are not found in normal vegetative tissues, but accumulate to high levels in response to heat stress. Specific smHSPs are also expressed during various phases of plant development as part of the endogenous developmental programme. Thus, although the smHSPs are apparently not essential for basal cell functions as are the high molecular weight HSPs such as HSP90, HSP70 and HSP60, their functions are likely to be critical for survival and recovery from heat stress as well as for specific developmental processes. Biochemical analysis indicates that smHSPs are found in high molecular weight complexes between 200-400 kDa that are most likely composed solely of multiple smHSP subunits. Purified recombinant plant smHSPs facilitate reactivation of chemically denatured enzymes in a nucleotide-independent fashion and also prevent heat-induced aggregation or reverse inactivation of protein substrates. Based on these data, it is suggested that smHSPs act in vivo as a type of molecular chaperone to bind partially denatured proteins preventing irreversible protein inactivation and aggregation, and that smHSP chaperone activity contributes to the development of thermotolerance.
.
[本文引用: 2]
DOI:10.1146/annurev.pp.42.060191.003051URL [本文引用: 4]
[本文引用: 1]
DOI:10.1007/BF00017810URL [本文引用: 1]
DOI:10.1074/jbc.M607677200URL [本文引用: 1]
DOI:10.1016/j.bbrc.2005.07.065URLPMID:16055090 [本文引用: 1]

Small heat-shock proteins (sHSPs) represent an abundant and ubiquitous family of molecular chaperones. The current model proposes that sHSPs function to prevent irreversible aggregation of non-native proteins by forming soluble complex. The chaperone activity of sHSPs is usually determined by the capacity to suppress thermally or chemically induced protein aggregation. However, sHSPs were frequently found in the insoluble complex particularly in vivo. In this report, it is clearly revealed that the insoluble sHSP/substrate complex is formed when sHSP is overloaded with non-native substrates, which is the very case under in vivo conditions. The proposal that sHSPs function to prevent the protein aggregation seems misleading. sHSPs appear to promote the elimination of protein aggregates by incorporating into the insoluble protein complex.
DOI:10.1104/pp.106.087817URL [本文引用: 1]
[本文引用: 1]
[本文引用: 1]
[本文引用: 1]
DOI:10.1111/j.1365-3040.2012.02525.xURL [本文引用: 1]
DOI:10.1016/j.jplph.2017.01.004URL [本文引用: 1]
[本文引用: 2]
[本文引用: 2]
[本文引用: 2]
[本文引用: 2]
[本文引用: 1]
[本文引用: 1]
DOI:10.1016/0304-3770(82)90026-2URL [本文引用: 1]

The glycolate metabolism of three submersed aquatic macrophytes, Potamogeton pectinatus L., Vallisneria spiralis L. and Hydrilla verticillata (L.f.) Royle, was studied by estimating the endogenous levels of glycolate and hydrogen peroxide (HP 2O 2) and assaying the activities of glycolate oxidase and catalase. The highest rate glycolate oxidase activity was recorded at pH 8.0, 7.6 and 7.4 for Potamogeton, Vallisneria and Hydrilla, respectively, while pH levels above and below this value markedly reduced the glycolate oxidase activity. The optimum glycolate oxidase activity of the three species was obtained when the reaction time was restricted to 10 min. Both the glycolate content and the activity of glycolate oxidase in the three leaf age groups (young, mature and old) were highest in mature leaves of these aquatic species. The glycolate content in mature leaves was highest in Hydrilla which also showed the lowest glycolate oxidase activity. Lowest glycolate content and highest glycolate oxidase activity were recorded in Potamogeton. An intermediate picture was observed in Vallisneria. The H 2O 2 content gradually increased with leaf age, while the catalase activity decreased. The content of H 2O 2 in mature leaves was highest in Vallisneria and lowest in Hydrilla. The catalase activity was also lowest in the former of the three species. During ageing of isolated mature leaves in the dark, there was a gradual decline in glycolate metabolism with increase in leaf age in Vallisneria and Hydrilla, but not in Potamogeton which showed a rise in glycolate oxidase activity after 3 days of induced ageing. Kinetin promotd glycolate metabolism in mature leaves of the three submersed plants during induced ageing, while ethrel and abscisic acid (ABA) showed an opposite effect.
北京: 高等教育出版社, 2000. pp 164- 169,
URL [本文引用: 2]

本书全面地介绍了植物生理生化实验原理及其技术。全书分为两篇。第一篇为实验原理,着重介绍了植物生理生化实验技术的一般原理和离心技术、层析技术、电泳技术、红外线Co2气体分析技术、光学分析技术、气体测压技术、电化学分析技术、免疫化学技术的基本原理。第二篇为实验技术部分,共选编了55个实验项目,内容涉及植物的细胞生理、水分生理、矿质营养、光合作用、呼吸作用、抗性生理等。
Beijing: Higher Education Press, 2000. pp 164- 169,
URL [本文引用: 2]

本书全面地介绍了植物生理生化实验原理及其技术。全书分为两篇。第一篇为实验原理,着重介绍了植物生理生化实验技术的一般原理和离心技术、层析技术、电泳技术、红外线Co2气体分析技术、光学分析技术、气体测压技术、电化学分析技术、免疫化学技术的基本原理。第二篇为实验技术部分,共选编了55个实验项目,内容涉及植物的细胞生理、水分生理、矿质营养、光合作用、呼吸作用、抗性生理等。
[本文引用: 1]
[本文引用: 1]
DOI:10.1016/j.tibs.2011.11.005URLPMID:3460807 [本文引用: 1]

The small heat shock proteins (sHSPs) and the related α-crystallins (αCs) are virtually ubiquitous proteins that are strongly induced by a variety of stresses, but that also function constitutively in multiple cell types in many organisms. Extensive research has demonstrated that a majority of sHSPs and αCs can act as ATP-independent molecular chaperones by binding denaturing proteins and thereby protecting cells from damage due to irreversible protein aggregation. As a result of their diverse evolutionary history, their connection to inherited human diseases, and their novel protein dynamics, sHSPs and αCs are of significant interest to many areas of biology and biochemistry. However, it is increasingly clear that no single model is sufficient to describe the structure, function or mechanism of action of sHSPs and αCs. In this review, we discuss recent data that provide insight into the variety of structures of these proteins, their dynamic behavior, how they recognize substrates, and their many possible cellular roles.
DOI:10.1016/0076-6879(90)86141-HURL [本文引用: 1]
[本文引用: 1]
DOI:10.1007/BF01276928URL [本文引用: 1]

Rapid loss of peanut seed quality during storage is a common problem in seed production for which little information concerning the physiological mechanism(s) is known. This study examined changes in esterase activity of unimbibed and imbibed peanut seeds previously exposed to ambient storage conditions. A high quality seed lot was divided into three subsamples. One was immediately placed into cool storage and classified high quality. The remaining two were exposed to ambient conditions until their seed quality declined to a medium or low quality level at which time they were also placed in cool storage. Total specific esterase activity of unimbibed seeds declined from 100 % to 91 % to 84 % in the cotyledon and 100 % to 92 % to 87 % in the axes of high, medium, and low quality seeds, respectively. After 3 days imbibition, total specific esterase activity for the low quality seed cotyledons and axes was 94 % of the high quality seeds. Examination of the isoesterase profiles using isoelectric focussing demonstrated that these changes in esterase activity were not uniform among all isoesterase species. These changes were not associated with damaged isoesterase species found in unimbibed seeds following seed storage. We conclude that specific isoesterases are prone to deterioration during ageing of peanut seeds and that other isoesterases associated with germination are preferentially synthesised in deteriorated peanut seeds during imbibition
URL [本文引用: 1]

Abstract Seeds of Phaseolus vulgaris L. were artificially aged by exposing them to 42 C and 100% relative humidity during one to sixteen days. The percentage of germination decreased from 10% at 0-4 days of accelerated aging (AA), to 22% at six days and finally to 0% after 16 days of AA, while the average germination period increased as the AA period was prolonged. The in situ analyses and dosages material revealed a disorganization and partial loss of the protein content during this period. A large number of very small starch grains suggests that degradation occurred of this material during AA, in spite of the dosages obtained by the Anthrone method which were not significantly different. The nuclei became pyknotic in 81% of the cells analyzed after 16 days of AA. The nucleoli disappeared during this period, also confirming the viability loss of cotyledon cells during aging.
[本文引用: 1]
.
[本文引用: 1]
[本文引用: 1]
DOI:10.1007/s10529-011-0769-3URL [本文引用: 1]
DOI:10.1016/S0378-1119(00)00043-3URLPMID:10717479 [本文引用: 1]

A rice ( Oryza sativa L. cv. Nakdong) cDNA clone, Oshsp26, encoding the chloroplast-localized small heat shock protein (smHSP) was isolated. Southern blot analysis of genomic DNA and the result of screening of a cDNA library indicated that the Oshsp26 gene is encoded by a single gene in the rice genome. The Oshsp26 gene was expressed following heat stress: the transcript level was highest when rice leaves were treated at high temperatures for 2 h at 42 C, and the transcripts became detectable after 20 min and reached a maximum level after 2 h. It was also found that the Oshsp26 gene was expressed following oxidative stress even in the absence of heat stress. Treatment of rice plants with methyl viologen (MV) in the light and treatment with hydrogen peroxide (H 2O 2), either in the light or in the dark, both caused a significant accumulation of the transcripts and the protein. Since MV treatment in the light leads to the generation of H 2O 2 inside the chloroplast, it is likely that H 2O 2 by itself acts to induce the expression of the Oshsp26 gene. These results suggest that the chloroplast smHSP plays an important role in protecting the chloroplast against damage caused by oxidative stress as well as by heat stress.
DOI:10.1104/pp.126.3.1266URL [本文引用: 1]
[本文引用: 1]
DOI:10.1046/j.1365-313x.2000.00837.xURLPMID:10998182 [本文引用: 1]

Summary NtHSP18P (HSP18), a cytosolic class I small heat-shock protein from tobacco pollen grains, was expressed in Escherichia coli. The viability of these cells was improved by 50% at 50 C, demonstrating its functionality in vivo . Purified recombinant protein formed 240 kDa HSP18 oligomers, irrespective of temperature. These oligomers interacted with the model substrate citrate synthase (CS) to form large complexes in a temperature-dependent manner. Furthermore, HSP18 prevented thermally induced aggregation of CS at 45 C. The fluorescence probe bis-ANS revealed the exposure of HSP18 hydrophobic surfaces at this temperature. Reactivation of chemically denatured CS was also significantly enhanced by HSP18. Surprisingly, HSP18 function was inhibited (in contrast to the related chaperone -crystallin and plant sHSPs studied so far) by the presence of ATP in a concentration-dependent manner. The conformational changes of HSP18 imposed by ATP binding were indicated by the difference in the quenching of intrinsic tryptophan fluorescence, and implied more compact structure with ATP. Fluorescence measurements with bis-ANS showed that the conformational shift of HSP18 is suppressed in the presence of ATP. Decreased chaperone activity of HSP18 in the presence of ATP is caused by the lower affinity of conformationally blocked HSP18 for the substrate, as demonstrated by a higher susceptibility of model substrate, malate dehydrogenase, to proteolytic cleavage. Our results suggest that the chaperone activity of some plant sHSPs could be regulated by the availability of ATP in the cytoplasm, which would provide a mechanism to monitor the cell environment, control biological activity of sHSPs, and coordinate it with other ATP-dependent chaperones such as HSP70.
[本文引用: 1]
DOI:10.1007/s00299-013-1506-2URL [本文引用: 1]
DOI:10.1007/BF00019473URL [本文引用: 1]
DOI:10.1111/j.1365-3040.2012.02525.xURL [本文引用: 1]