
收稿日期:2014-04-16 基金:
摘要
关键词:甘蓝型油菜; 单核苷酸多态性; 数量性状位点; 种子硫苷含量
Mapping Quantitative Traits Loci for Seed Glucosinolate Content in
JIAN Hong-Ju, WEI Li-Juan, LI Jia-Na, XU Xin-Fu, CHEN Li, LIU Lie-Zhao

* Corresponding author: LIU Lie-Zhao, E-mail:liezhao2003@126.com, Tel: 023-68250701 Fund:
Abstract
Keyword:
Show Figures
Show Figures
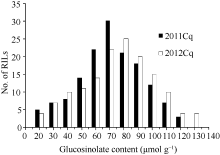
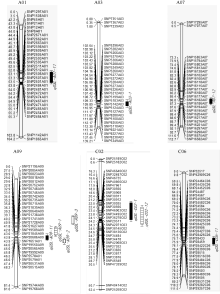
Rapeseed ( Brassica napus) is one of the most important oilseed crops in the world, and serves as the third largest source of plant oil. The residual meal, which is used in livestock feed mixtures, contains 38%-44% of high quality protein after oil extraction from rapeseed seeds[ 1]. Beta-thioglucoside-N-hydroxysulfates commonly known as glucosinolates were accumulated extensively in rapeseed. Glucosinolate plays the important biological and economic roles in plant defense and human nutrition[ 2]. Glucosinolate and their hydrolysis products are involved in different bioactivities. Degradation products of glucosinolate are thought to inhibit carcinogenesis by affecting cell cycle arrest and stimulating apoptosis[ 3, 4]. However, the presence of degradation products is not always beneficial. High intakes of glucosinolate and their degradation products can cause problems of palatability and are associated with goitrogenic, liver and kidney abnormalities[ 5]. Besides, certain degradation products of glucosinolate may be involved in growth regulation[ 6, 7, 8]. This particularly limits the use of the rich-protein meal as a feed supplement for monogastric livestock. In order to regulate and optimize the level of individual glucosinolate, breeders are interested in tissue-specific improvement of crop nutritional quality and resistance to diseases and insect pests.
In recent years, great achievements have been achieved in molecular genetics and biochemistry of glucosinolate biosynthesis in the model species Ar a bidopsis[ 2]. More than 20 genes involved in glucosinolate biosynthesis in Arabidopsis have been identified over the past three years. QTLs for total seed glucosinolate content have been detected using different oilseed rape populations. Uzunova et al.[ 9] first reported four QTLs in 1995, which explained 61.7% of seed glucosinolate content in the B. napus double haploid (DH) population. Howell et al.[ 10] mapped four QTLs using the B. napus population derived from Victor × Tapidor and three QTLs using the B. napus population derived from Bienvenu × Tapidor, which explained more than 90% of seed glucosinolate content. Zhao and Meng[ 12] detected three QTLs responsible for seed glucosinolate content. Hasan et al.[ 1]found that four genes involved in the biosynthesis of indole, aliphatic and aromatic glucosinolate might be associated with known quantitative traits loci for total seed glucosinolate content in B. napus. However, the densities of maps used in these studies were relatively low, which were constructed with traditional DNA markers, such as AFLP, RFLP, and SSR.
For QTL analysis, constructing a high density linkage map composed of DNA markers without large gaps can detect the minor QTLs. In this study, we analyzed QTLs for seed glucosinolate content in the B. napus recombinant inbred line (RIL) populations with the high density SNP linkage map, which contains 9164 SNP markers covering 1832.9 cM, with 1232 bins accounting for 7648 of the markers[ 13]. We identified 10 QTLs associated with seed glucosinolate content in oilseed rape through genetic analysis of a RIL population of B. napus over two years. The QTLs detected in this study would provide more information on QTLs that are associated for seed glucosinolate content. The results deposit candidate molecular markers closely linked to target genes/QTLs, which might be used not only in rapeseed breeding but also in map-based gene cloning.
1 Materials and Methods1.1 Plant materials and mapping populationsThe female parent GH06 was derived from the selfing generations of yellow-seeded B. napus, with 73.34 μmol g-1 of glucosinolate content. The male parent, Pl74, was derived from selfing generation of Youyan 2, with 18.75 μmol g-1 of glucosinolate content. The recombinant inbred lines (RILs) were the ninth generation by successively selfing from the GH06 × P174 cross. In this study, 172 RILs and their parents were planted in a randomized complete block design with three replicates in experimental field of Southwest University in 2011 and 2012. Each plot contained 20 plants in two rows, with 0.5 m between rows and 0.2 m between plants.
1.2 Glucosinolate content analysisSelfing seeds in each line from three plants were mixed as a sample. The total seed glucosinolate content and its components were tested using near infrared spectroscopy (NIR) by means of WinISI II software of an NIR System 6500. Each assay had three technical replicates, and the mean value was considered as a measurement.
1.3 SNP marker analysisThe Brassica 60 K SNP BeadChip Array was used to genotype 172 lines and parental plants. This array, which successfully assays 52 157 Infinium Type II SNP loci in B. napus, was developed by an international consortium using preferentially single-locus SNPs contributed from genomic and transcriptomic sequencing in genetically diverse Brassica germplasm (Isobel Parkin, Agriculture and AgriFood Canada, unpublished data).
Total genomic DNA was extracted from well- expanded leaves of RIL and the two parental lines, following the methodology of DP321-03 DNA extraction kits (Tiangen, Beijing, China). DNA sample preparation, hybridisation to the BeadChip, washing, primer extension and staining were performed according to the work flow described in the Infinium HD Assay Ultra manual. Imaging of the arrays was performed using an Illumina HiSCAN scanner after BeadChip washing and coating. Allele calling for each locus was performed using the GenomeStudio genotyping software V.2011 (Illumina, Inc.). SNP markers were named using SNP plus index numbers assigned by GenomeStudio, followed by the chromosome number.
1.4 Construction of genetic linkage map and QTL analysisA high-density genetic linkage map for the complex allotetraploid species B. napus (oilseed rape) was constructed using the above-referenced RIL population. This map was composed of genome-wide single nucleotide polymorphism (SNP) markers assayed by the Bra s sica 60 K Infinium BeadChip Array. Composite interval mapping (CIM) was carried out using the software package Windows QTL Cartographer 2.5[ 14]. The LOD thresholds for QTL significance were determined by a permutation test (1000 replications) with a genome-wide significance level P=0.01 to judge whether there exist QTLs. Running result of software can show additive effects of QTLs and phenotypic contribution rate. QTL nomenclature followed the protocol of McCouch et al.[ 15]. Take “ qGSL- A01-11” as an example, “q” refers to QTL; “GSL” refers to glucosinolate content, “A01” indicates the linkage group, and the number “11” indicates the year of field experiment (the first “1”, short form of 2011) and the series number of the QTL (the second “1”). This number is in one-digit for some QTLs, which means the QTL was identified in both years.
2 Results2.1 Seed glucosinolate content variation in the RIL populationThe total glucosinolate content of the parents (GH06 and P174) were 73.34 and 18.75 μmol g-1, respectively, with significant difference in B. napus that provided ideal material for QTLs analysis. The content of the RIL population was distributed from 12.00 to 117.75 μmol g-1 with an average of 63.9 μmol g-1 in 2011, and from 13.50 to 128.80 μmol g-1 with an average content was 70.80 μmol g-1 in 2012 (Table 1). As expected, the two years total glucosinolate contents were significantly correlated ( P< 0.01, R2= 0.997). The seed glucosinolate content from 50 to 100 μmol g-1 accounted for 80.6% of the RIL population (Fig. 1). The RIL population showed a continuous and bell-shaped distribution for glucosinolate content in two years, suggesting the multi-genic control of glucosinolate in B. napus. However, transgressive segregations in the RIL population were observed, indicating that glucosinolate synthesis in seeds was controlled by some genes in the two parents.
Table 1
Table 1
![]()
| Table 1 Variation of glucosinolate content in the RIL population of B. napus |
Fig. 1
Figure OptionViewDownloadNew Window | |
![]() | Fig. 1 Frequency distribution of seed glucosinolate content in the RIL populations over two years |
2.2 QTL analysis of glucosinolate contents in B. napus seeds The SNP linkage map contains 9164 SNP markers covering 1832.9 cM, with 1232 bins accounting for 7648 of the markers. A subset of 2795 SNP markers, with an average distance of 0.66 cM between adjacent markers, was applied for QTL mapping of seed glucosinolate content. Five significant QTLs for the total glucosinolate content in B. napus seed were detected on chromosome A03, C02, and A09 by analysis of the RIL population harvested in 2011 and 2012 (Fig. 2). LOD threshold values for significant QTLs in 2011 and in 2012 were determined to be 3.01-10.43 by the permutation test (Table 2). These QTLs explained 56.9% and 55.1% of the total phenotypic variance in 2011 and 2012, respectively. Locus qGSL-A09-2 detected in 2011 shared the highest contribution value (26.2%), while the QTL qGSL-C02-1 detected in 2011 explained the lowest phenotypic variance (4.9%). Four of these QTLs had the positive additive effect, indicating the female parent GH06 contributed to a strong increase in seed glucosinolate content except for the qGSL-C02-1. The qGSL-A09-2 and qGSL-A09-3 detected in 2011 explained the total phenotypic variances of 26.2% and 11.1%, respectively, and in 2012 were 19.0% and 16.5%, respectively.
Besides, five QTLs for total glucosinolate content were identified in either 2011 or 2012, which were mapped on chromosomes A01, A07, A09, C02, and C06. A single QTL explained 4.1%-7.9% of the phenotypic variance and the LOD threshold value varied from 2.18 to 3.93 (Table 2). Three QTLs ( qGSL-A01- 11, qGSL-A07-12, and qGSL-C02-12) had negative effect, showing the additive alleles from parent P174.
Fig. 2
Figure OptionViewDownloadNew Window | |
![]() | Fig. 2 QTLs associated with total seed glucosinolate content detected in the RIL from a cross of GH06×P174The bar length on the right represents one LOD supporting interval for each QTL and only QTL region markers are displayed on the linkage map. |
Table 2
Table 2
![]()
| Table 2 Quantitative traits loci involved in the seed gluconsinolate content of RIL populations |
3 DiscussionIn this study, we mapped QTLs of seed glucosinolate content in B. napus, a major oilseed crop in the world. Glucosinolate content is a complex trait and its expression is controlled by a gene network in the family Brassicaceae[ 16]. In previous studies, more than fifty genes have been found to be involved in glucosinolate biosynthesis, including transcription factors, core structure formation, secondary modification, and core-substrate pathways[ 2, 3]. In B. napus, however, the studies have primarily laid emphasis on the genetics of total glucosinolate c-ontents[ 17, 18]. Four QTLs on B. napus chromosomes N9, N12, N17, and N19 were detected independently in different studies, indicating that these QTLs represent major loci that influence seed glucosinolate content in different materials[ 9, 10, 11, 12, 19]. Uzunova et al.[ 9] detected two major and two minor QTLs for glucosinolate content, whereas Toroser et al.[ 17] detected two major and three minor QTLs. The genetic maps of B. napus developed by Parkin et al.[ 20] and Ferreira et al.[ 21] have been fully aligned. The results showed that the major QTLs GSL-1 and GSL-2, and the minor QTL GSL-4 in Toroser et al. correspond to GLN1, GLN2, and GLN4[ 10]. respectively.
In our study, two major QTLs were located on A09 in both 2011 and 2012, which are consistent with the previous results[ 9, 10, 12]. The two major QTLs for seed glucosinolate content on A09 were linked closely to the SNP marker SNP21741A09 and SNP21712A09, respectively. The two QTLs namely qGSL-A09-2 and qGSL- A09-3 explained 26.2%, 11.1%, and 19.0%, 16.5% of high phenotypic variance which in 2011, 2012, respectively. Comparative mapping has showed that the qGSL-A09-2 corresponded to GLN1 on N9 in Howell et al. There were another four QTLs detected in both two years namely qGSL-A03-1, qGSL-A03-2, qGSL-A09-1, and qGSL-C02-1, which are distributed on A03, A03, A09, and C02, respectively. The chromosome C02 is just N12 described in previous studies[ 10], but the contribution is relatively small. In addition to the two major QTLs for seed glucosinolate content, we also detected three minor QTLs in 2011 and two minor QTLs in 2012. These minor QTLs might be associated with several minor genes that associated with seed glucosinolate content but were affected readily by environments.
Quantitative trait lous (QTL) mapping of seed glucosinolates (GSL) content has been reported in Brassica napus. The key loci responsible for low GSL content are reported to be the transcription factors designated MYB28[ 22]. Scanning of the 600 kb regions flanking the QTLs found two possible genes, i.e. BZO1p1 and SUR1 involved in GSL biosynthesis. The exact adjacent relationships are as follows: Bra004132 (benzoate-CoAligase, physical region 17.01 Mb) located in qGSL-A07-12closed to SNP1618 6A07 and Bra 036703 ( S-alkylthioh-ydroximatelyase/carbon-sulfur lyase/tran-saminase, physical region 5.53 Mb) located in qGSL-A09-3region closed to SNP21712A09 marker. However, the gene BZO1p1 catalyzes side chain modification and leads to formation of different GSLs, but does not affect the GSL content[ 23, 24]. Another gene SUR1 catalyzes core structure formation of GSLs with production of GSLs intermediate metabolite but not final GSLs[ 25]. The possible reason should be studied in the future.
The bar length on the right represents one LOD supporting interval for each QTL and only QTL region markers are displayed on the linkage map.
4 ConclusionFive QTLs detected in both two years are located on A03, A09 and C02, respectively. Two QTLs distributed on A09 explained phenotype variation 26.2% and 11.1% in 2011, respectively, 19.0% and 16.5% in 2012, respectively. Furthermore, This overall control of glucosinolate level could be primarily affected by two genes, SUR1 and BZO1p1 , contained in QTL qGSL-A09-3 and QTL qGSL-A07-12, respectively.
The authors have declared that no competing interests exist.
作者已声明无竞争性利益关系。
参考文献View Option
原文顺序
文献年度倒序
文中引用次数倒序
被引期刊影响因子
[1] | |
[2] | |
[3] | |
[4] | |
[5] | |
[6] | |
[7] | |
[8] | |
[9] | |
[10] | |
[11] | |
[12] | |
[13] | |
[14] | |
[15] | |
[16] | |
[17] | |
[18] | |
[19] | |
[20] | |
[21] | |
[22] | |
[23] | |
[24] | |
[25] |