全文HTML
--> --> -->天线的作用是将电磁波辐射进入自由空间. 传统上, 可以通过增大信号源功率以提升天线的辐射功率, 然而受物理机制、加工工艺等因素影响, 信号源功率增幅有限[5]; 也可以通过增大天线的辐射口径以增大辐射功率, 然而由于装配环境的限制, 辐射口径不可能无限制增大, 因此对有限口径的利用就显得尤为重要.
相控阵天线能够充分利用辐射口径. 一方面, 通过将天线单元组成阵列, 天线阵有更好的辐射方向性; 另一方面, 相控阵天线波束可以灵活偏转, 使得辐射电磁波可以覆盖更多区域.
目前对相控阵天线的研究主要集中在带宽提升和扫描范围扩大上. Munk[6]在2003年提出的紧耦合天线阵在近20年得到了巨大发展, 和传统相控阵天线相比, 紧耦合天线阵具有很宽的带宽和较大的扫描范围. 文献[7]中设计的紧耦合天线阵利用馈线地板带过孔的Marchand巴伦和频率选择表面匹配层, 在6.2∶1 (0.5—3.1 GHz)的带宽内、天线单元有源驻波比小于3.2的标准下, 实现了E面75°和H面60°的扫描性能. 文献[8]中设计的紧耦合天线阵利用微带-槽线的馈电结构和多层频率选择表面匹配层, 在5∶1 (1—5 GHz)的带宽内、天线单元有源驻波比小于3的标准下, 实现了E面70°和H面70°的扫描性能.
然而, 关于有限口径辐射功率的提升很少有****进行研究. 本文从影响有限口径辐射功率的因素进行分析, 设计了一个可用于提升有限口径辐射功率的紧耦合相控阵天线, 并对天线阵进行了加工和测试. 仿真和测试结果表明, 在1.7—5.4 GHz的带宽内、天线单元有源驻波比小于2的标准下, 天线阵能实现E面65°、H面45°和D面60°的扫描性能, 测试结果和仿真结果具有良好的一致性. 和文献中的其他设计相比, 按相同辐射口径面积换算, 本文设计的天线阵具有更高辐射功率.


在有限口径下布置更多单元, 意味着单元尺寸要尽量小. 紧耦合天线阵的单元间距小于0.5λhigh[9], 便于紧凑化设计. 同时, 可以采用高介电常数介质基板和紧凑型微带-共面带线的巴伦使得天线单元小型化[10].
天线阵辐射效率的提高, 意味着损耗要减小. 采用低损耗的介质基板减小介质损耗, 采用两层双面的频率选择表面匹配层改善天线阵和自由空间的阻抗匹配[7], 通过参数调整改善巴伦和天线的阻抗匹配, 从而提升天线阵的辐射效率.
2
2.1.天线单元设计
紧耦合天线阵利用临近单元的电容耦合, 抵消低频时来自地板的电感, 天线阵低频的性能表现得到改善, 从而具有较宽的阻抗带宽. 紧耦合天线单元一般由三个部分组成: 宽带巴伦、偶极子辐射单元和宽带宽角匹配层. 其中, 宽带巴伦起着平衡馈电的作用, 同时也起着馈电端和偶极子辐射单元的阻抗匹配的作用; 宽带宽角匹配层主要用于改善天线阵在大角度扫描时的性能表现.紧耦合天线阵在E面扫描时具有较宽的阻抗带宽, 在H面扫描时的阻抗带宽较窄. 因此, 在设计时, 先优化天线阵在E面扫描时的性能, 再加上宽带宽角匹配层改善天线阵在H面扫描时的性能. 天线单元设计的具体步骤如下:
1) 根据设计天线的最高工作频率和介质基板相关参数(介电常数和厚度), 确定天线单元间距;
2) 根据天线单元间距, 设计出满足宽带馈电和阻抗变换需求的紧凑型巴伦;
3) 调节耦合贴片宽度和偶极子辐射单元离地板的高度, 改善天线阵在E面宽角扫描时的性能;
4) 加载两层双面的匹配层, 经过参数优化, 改善天线阵在H面宽角扫描时的性能.
本文设计的天线单元结构如图1所示, 由微带-共面带线的巴伦、偶极子单元、两层双面的匹配层共三部分组成. 介质基板型号为Rogers 6110, 介电常数10.2, 损耗正切0.0023, 厚度0.64 mm. 偶极子两臂印制在介质基板的两面, 便于临近单元进行电容耦合. 天线单元相关的参数在图1(a)中标示, 具体数值为: A = 29.3 mm, B = 8 mm, C = 3.7 mm, D = 0.8 mm, E = 3.2 mm, F = 4.2 mm, G = 3 mm, H = 2.5 mm, I = 2.2 mm, J = 2.2 mm, K = 3.7 mm, L = 0.8 mm, W1 = 0.4 mm, W2 = 0.3 mm, W3 = 0.2 mm, W4 = 0.6 mm, W5 = 0.6 mm, L1 = 3.5 mm, L2 = 3.5 mm, L3 = 1.8 mm, L4 = 2 mm, L5 = 2.5 mm, Gap=0.8 mm. 天线单元在x和y方向周期均为0.144λhigh, 高度为29.3 mm (约为0.166λlow, 其中λlow为自由空间中最低工作频率对应的波长).
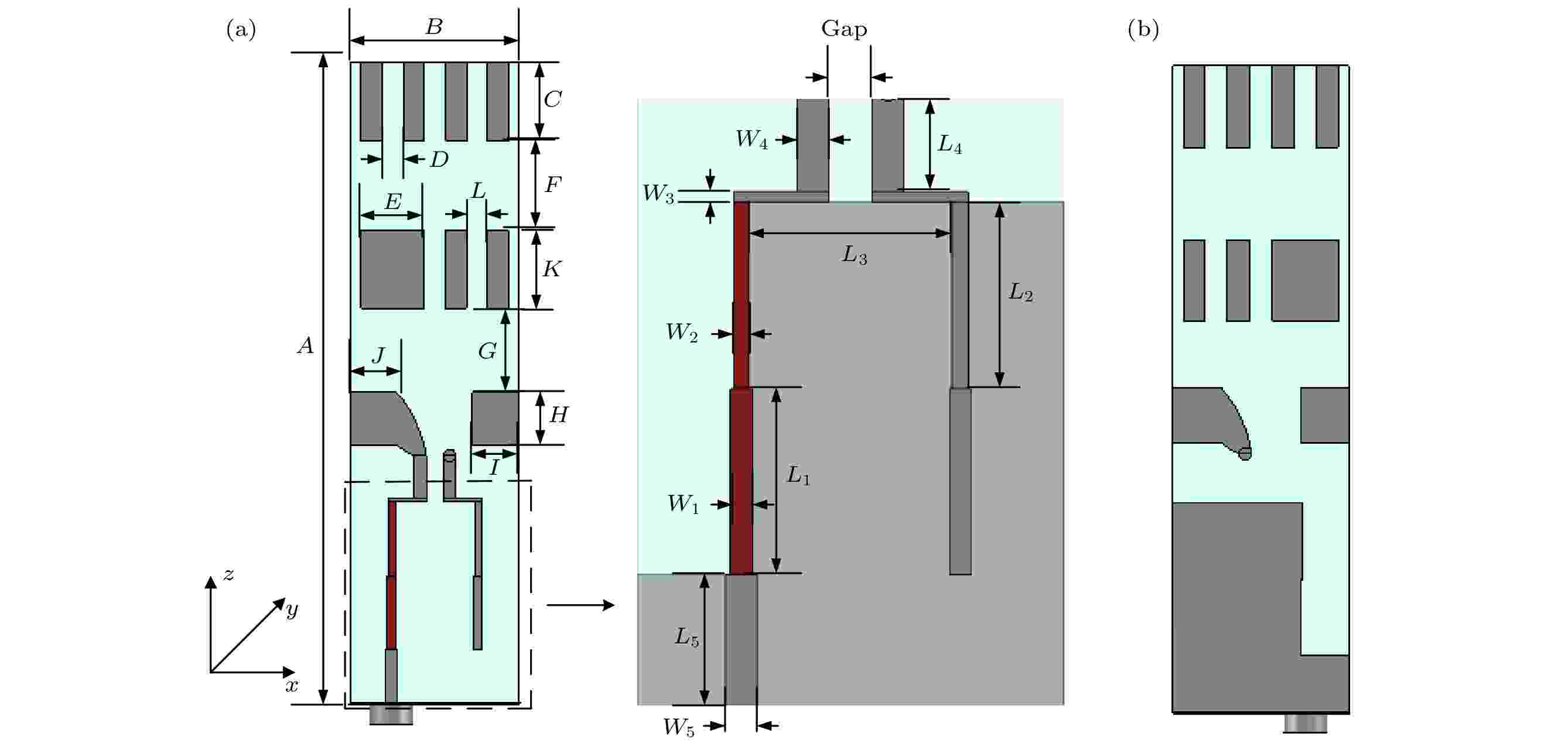
Figure1. Unit Cell of the TCDA: (a) Front view of the unit cell (the ground of the red parts is etched); (b) back view of the unit cell.
3
2.1.1.宽带巴伦的设计
本文设计的天线单元采用了加载空气补偿的微带-共面带线的巴伦, 结构如图1(a)所示. 巴伦的红色馈线部分下方地板被移除, 通过这种空气补偿的方式, 可以产生新的谐振点, 新的谐振点和原来的谐振点离得很近时, 可以拓宽巴伦的工作带宽[11]. 从图2中的仿真结果可以看出, 采用空气补偿的巴伦相比于未采用空气补偿的巴伦具有更宽的工作带宽. 此外, 由于天线单元要求占据较小的口径面积, 经优化设计, 减小L3的值, 使得巴伦结构更为紧凑.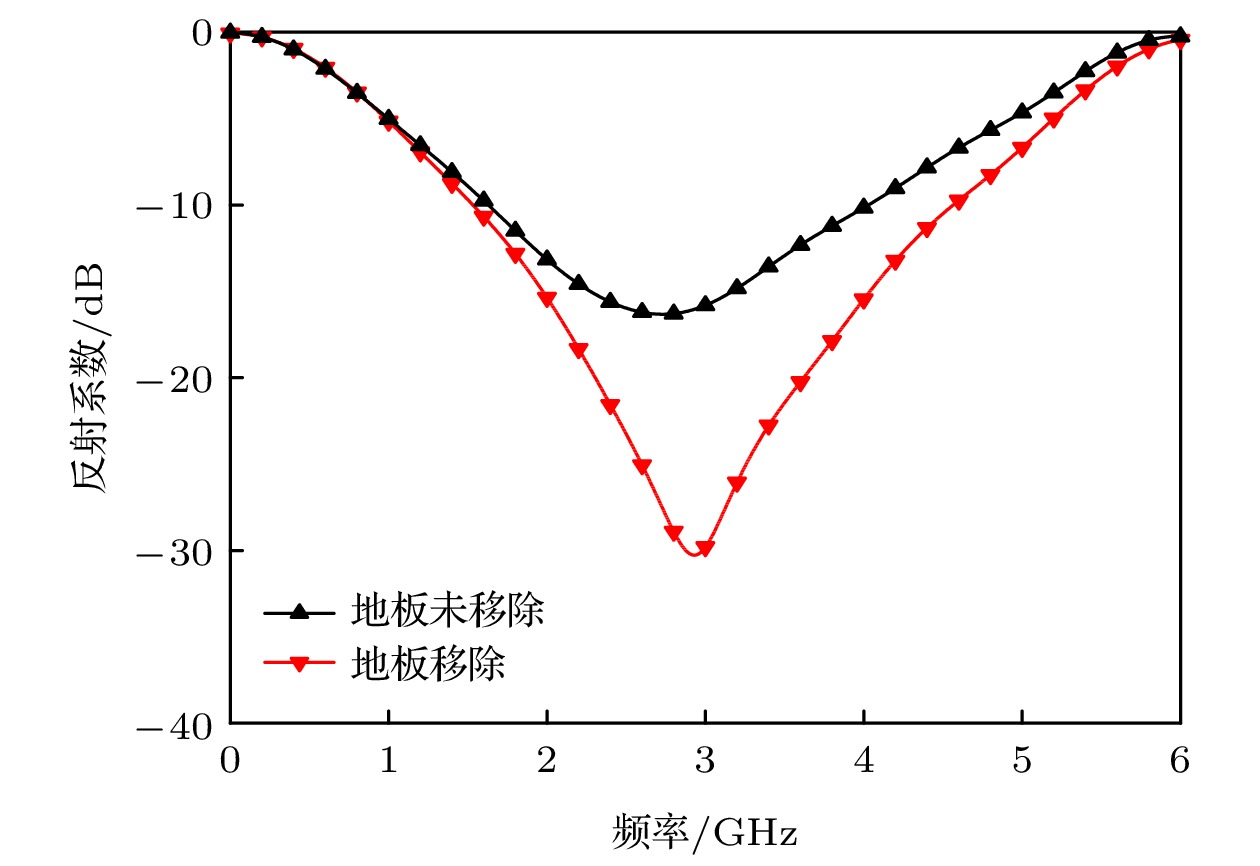
Figure2. Reflection coefficients of the balun with and without the etched ground of the red feeding parts.
3
2.1.2.宽带宽角匹配层的设计
相控阵天线扫描时, 天线单元的辐射电阻随着扫描角度的变化而变化. 一般在天线上方放置一块介质匹配层, 使得天线阵和自由空间有更好的阻抗匹配[12], 以改善天线阵的宽角扫描性能. 周期结构的频率选择表面匹配层可以替代传统的笨重且昂贵的介质匹配层. 周期结构的频率选择表面可视为超材料的一种, 其等效相对介电常数和相对磁导率可以根据文献[13]计算求得. 从图3可以看出, 天线阵加载两层双面的频率选择表面匹配层, 和无匹配层加载时相比, 在H面扫描时具有更好的阻抗匹配表现.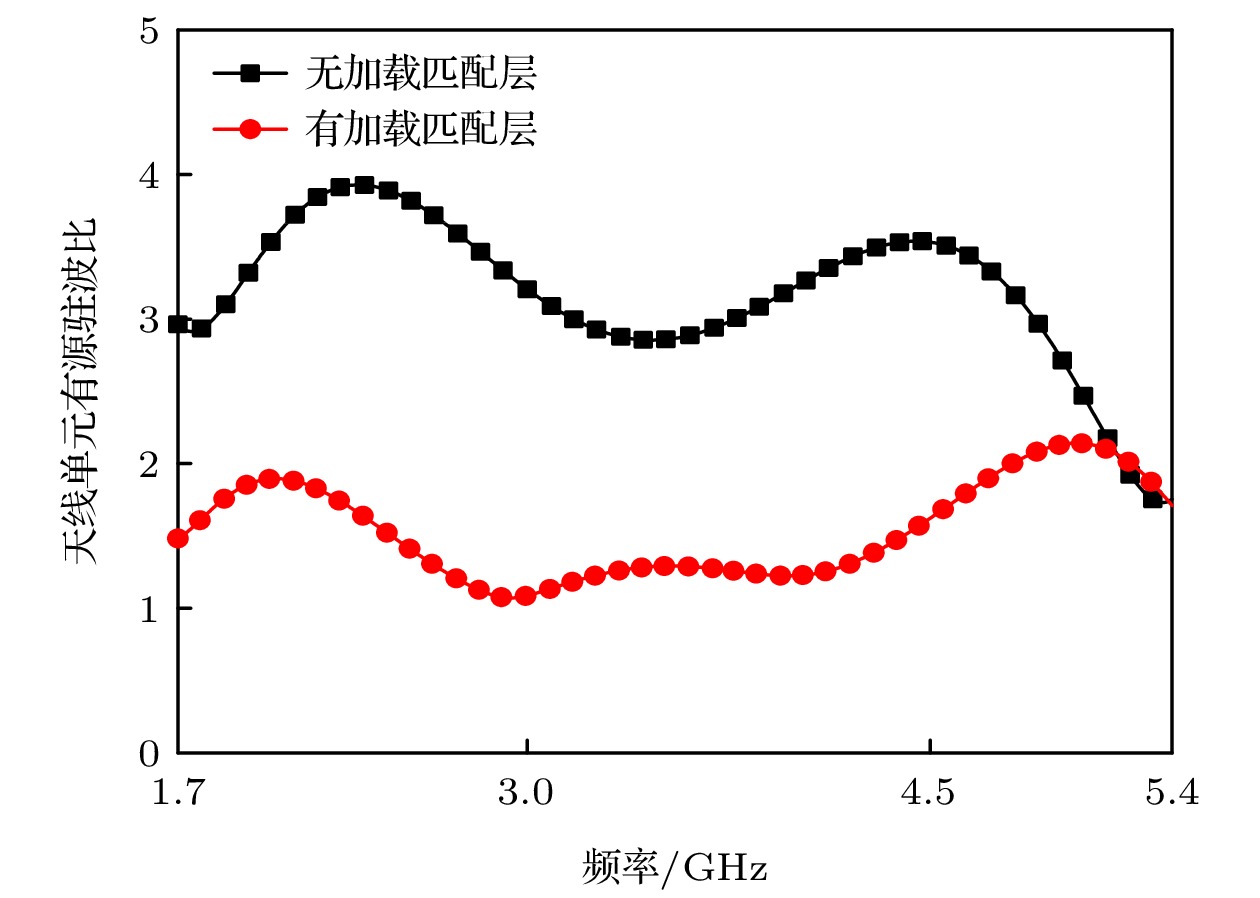
Figure3. Active VSWRs of the unit cell at 45° scanning in the H plane in infinite array simulation with and without frequency selective surfaces.
2
2.2.无限大阵列仿真结果
32.2.1.交叉极化比
图4(a)是无限大阵列在不同平面不同角度扫描时的交叉极化比. 可以看出, 在边射和H面45°扫描时, 在大多数频段, 交叉极化比均小于–15 dB; 在E面65°扫描时, 在大多数频段, 交叉极化比均小于–20 dB. 同时也注意到, 在边射、E面65°、H面45°和D面60°扫描时, 随着频率的升高, 交叉极化变差. 这是因为: 1) 偶极子的两臂印制在介质基板的两面, 电场和天线所在的平面不平行, 垂直于天线所在平面存在电场分量, 频率越高, 交叉极化恶化越严重, antipodal Vivaldi天线也有类似表现[14]; 2) 天线单元之间间距较小, 导致沿着H面的临近单元(y方向)会产生强耦合, 如图4(b)所示, 这些强耦合会恶化交叉极化水平, 频率越高, 耦合越强, 交叉极化恶化越严重.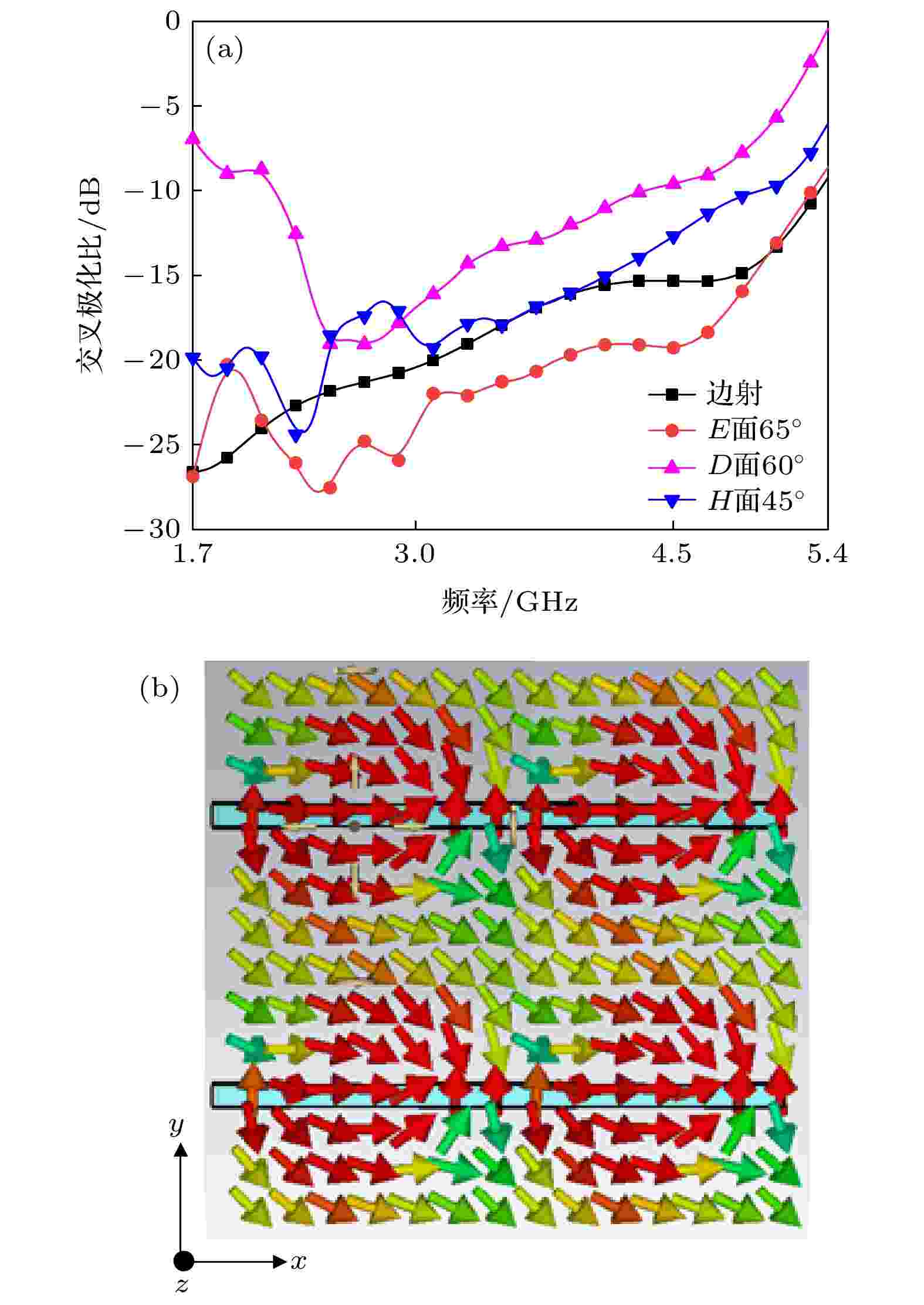
Figure4. Cross polarization level in infinite array simulation: (a) Cross polarization ratio at different angles scanning in different planes; (b) electric field on radiation aperture at 3 GHz.
3
2.2.2.辐射效率
图5给出了无限大阵列在不同平面不同角度扫描时的辐射效率. 在边射时, 阵列效率最低值为67%, 在大多数频段, 效率均大于75%; 在E面65°扫描时, 在大多数频段, 效率均大于70%; 在H面45°扫描时, 在大多数频段, 效率均大于75%; 在D面60°扫描时, 在大多数频段, 效率均大于65%. 然而, 在高频情况下, 在H面45°和D面60°扫描时, 天线阵辐射效率变低, 这是由于在高频时巴伦的阻抗匹配变差所致.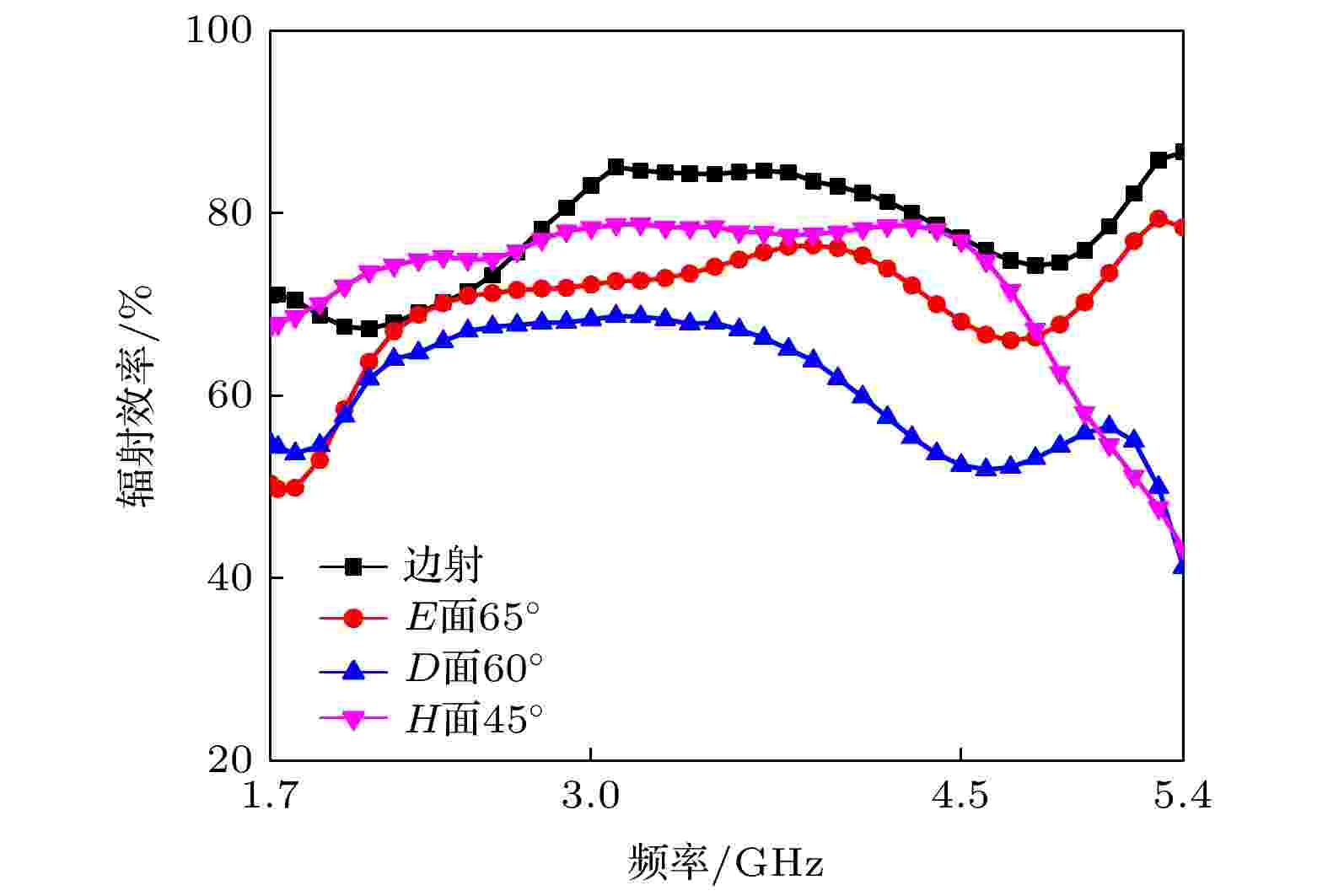
Figure5. Radiation efficiency of the proposed antenna array at broadside, 65° scanning in the E plane, 45° scanning in the H plane and 60° scanning in the D plane.
3
2.2.3.天线单元有源驻波比
从图6可以看出, 无限大阵列在边射、E面65°、H面45°和D面60°扫描时, 在1.7—5.4 GHz的频段内, 天线单元有源驻波比均小于2, 说明该阵列具有宽带宽角扫描性能, 同时保持良好的阻抗匹配.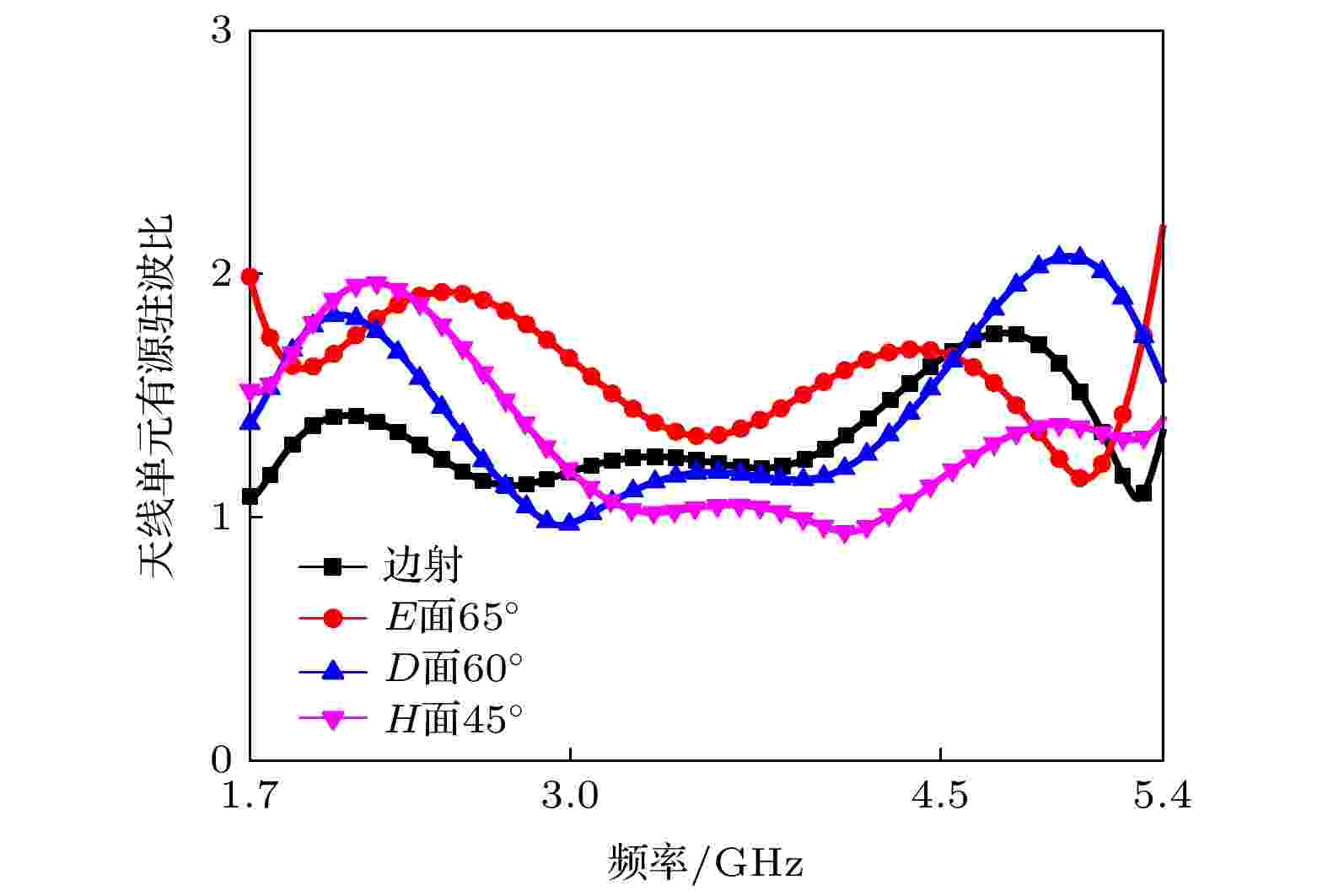
Figure6. Active VSWRs of the unit cell in infinite array simulation at broadside, 65° scanning in the E plane, 45° scanning in the H plane and 60° scanning in the D plane.
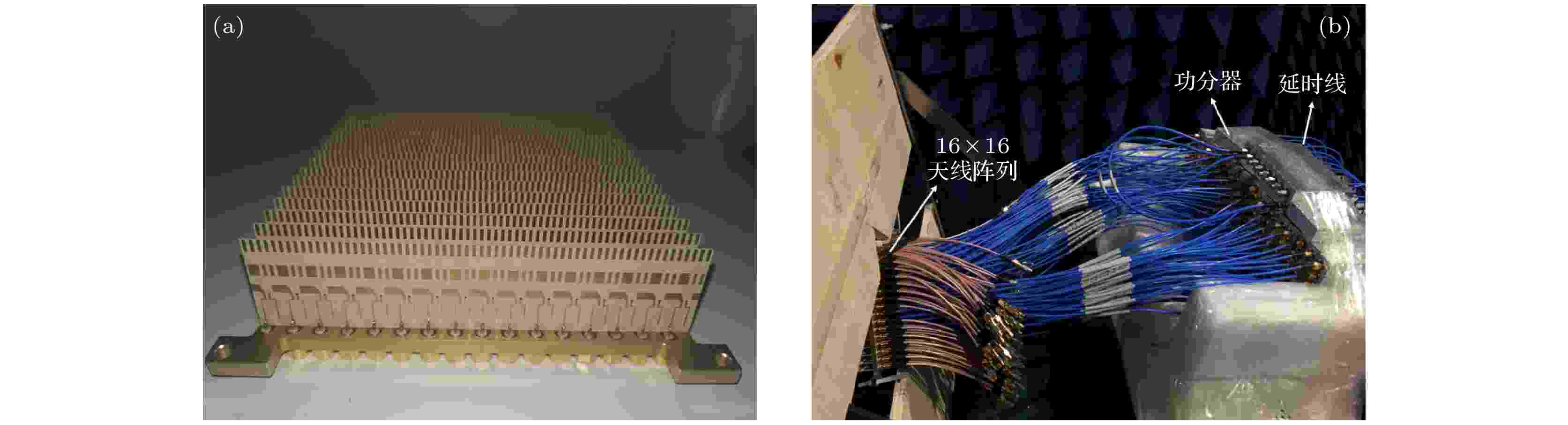
Figure7. Antenna array fabrication and measurement: (a) Fabricated prototype of 16 × 16 antenna array; (b) measurement setup and environment.
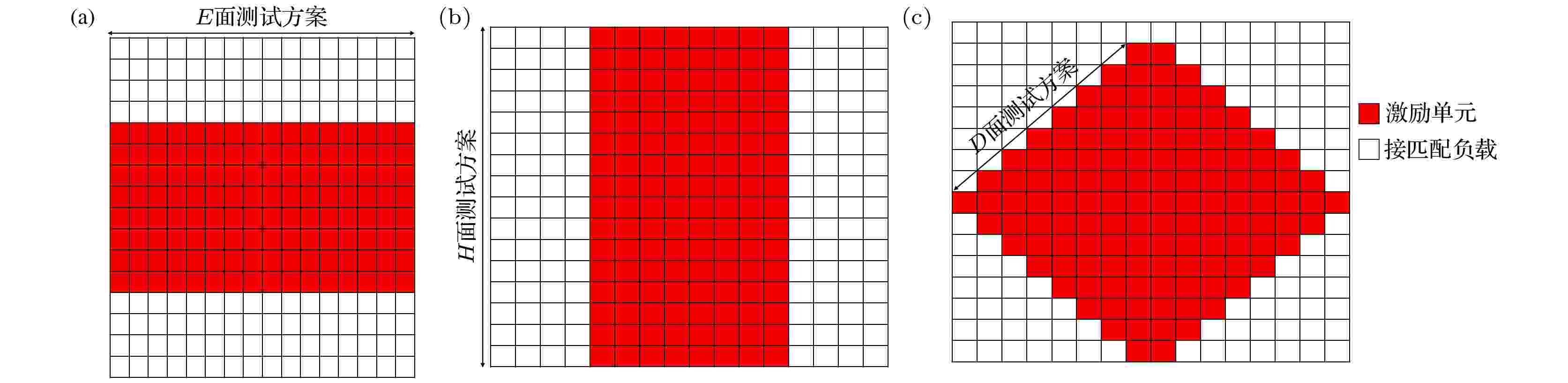
Figure8. Measurement scheme: (a) E plane; (b) H plane; (c) D plane.
2
3.1.远场测试结果
在3和5 GHz时, 天线阵E面、D面、H面在不同角度扫描时的测试和仿真的归一化方向图如图9—图11所示. 可以看出, 在低频时, 天线阵的主波束较宽; 在高频时, 天线阵的主波束较窄, 这是由于低频时天线阵辐射口径电尺寸较小、高频时天线阵辐射口径电尺寸变大所致. 同样地, 由于天线阵辐射口径的电尺寸较小, 在低频时, 阵列的主波束扫描角度未能到达最大扫描角度. 采用更大的阵列结构, 会改善这种情况.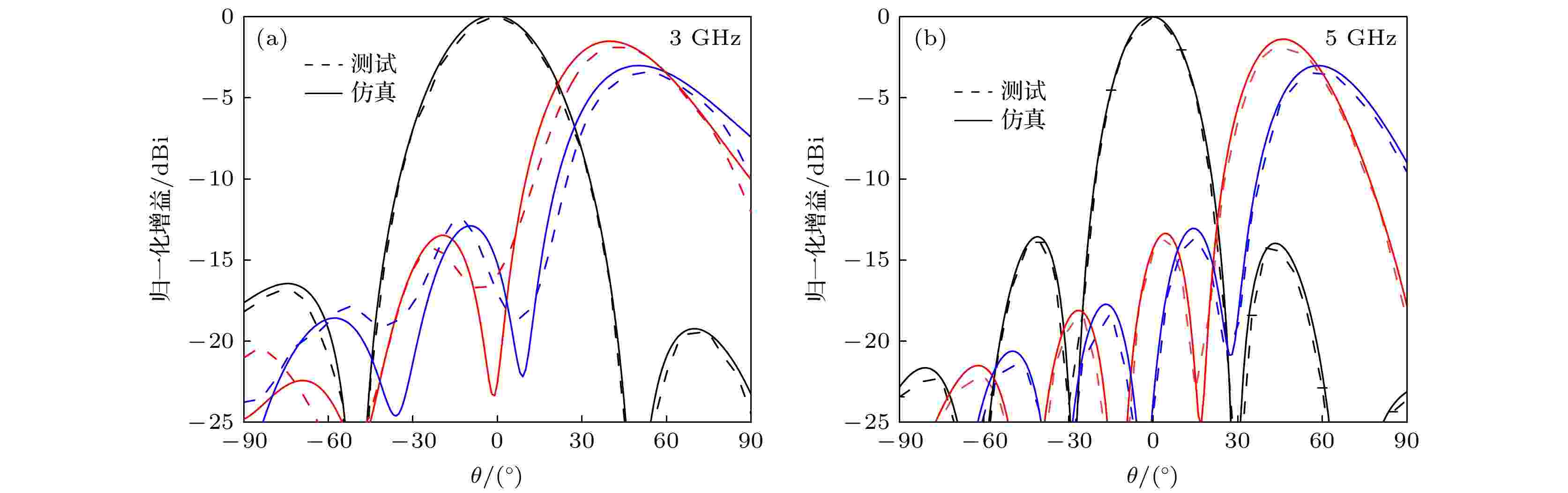
Figure9. Normalized radiation patterns at 0°, 45° and 65° scanning in the E plane: (a) 3 GHz; (b) 5 GHz.
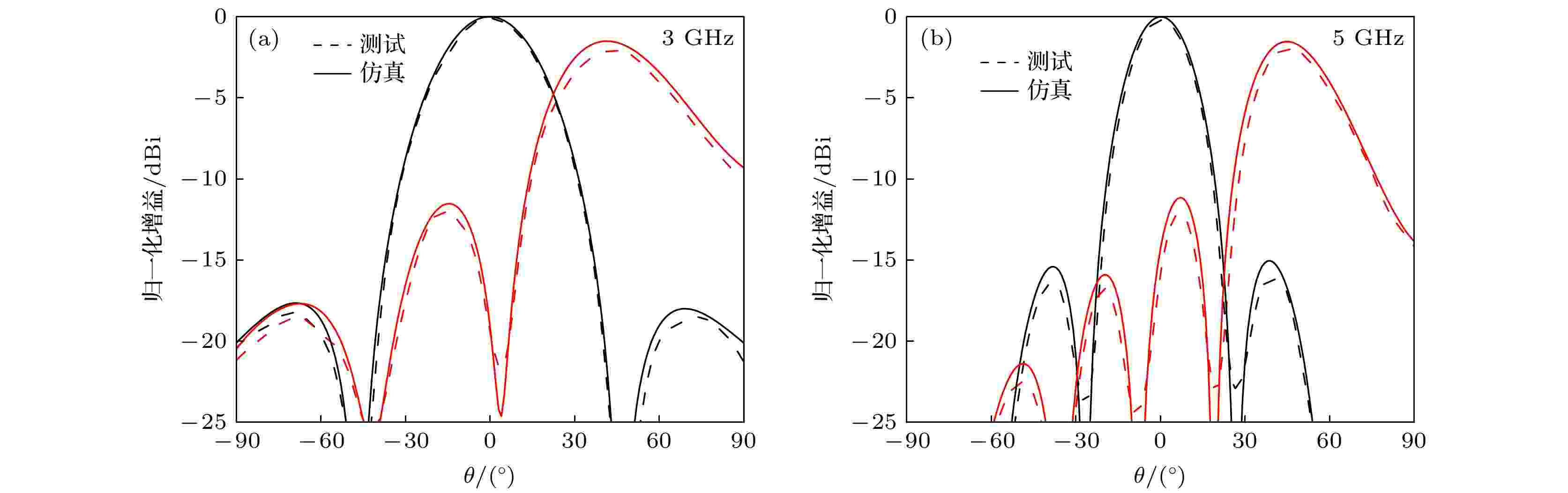
Figure10. Normalized radiation patterns at 0° and 45° scanning in the H plane: (a) 3 GHz; (b) 5 GHz.
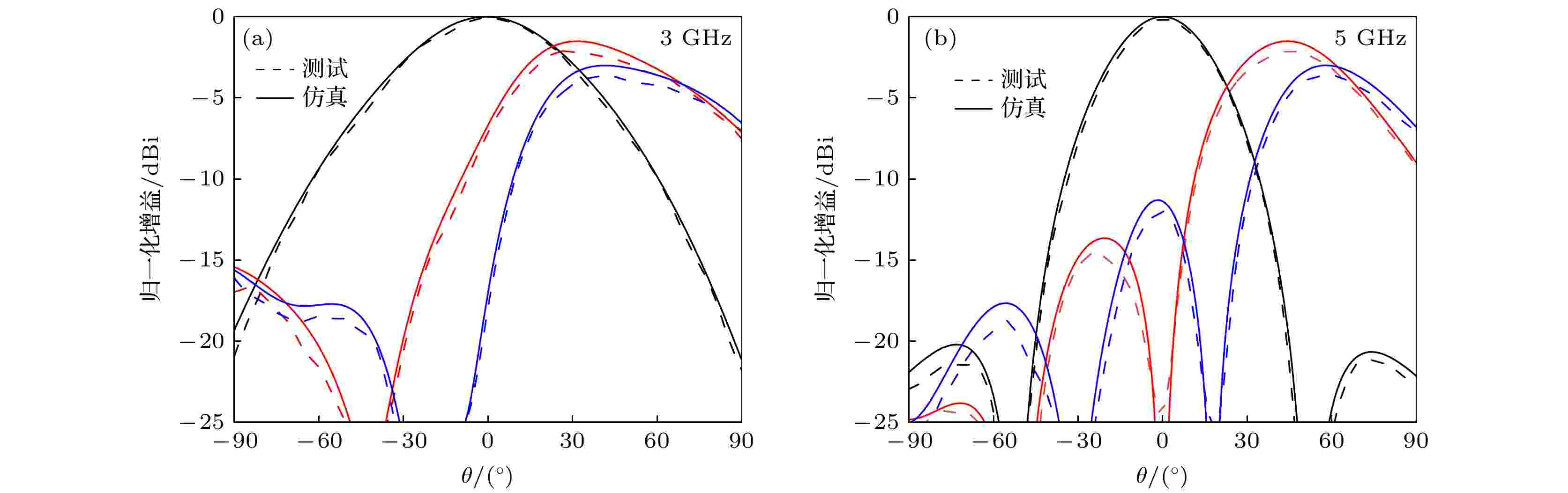
Figure11. Normalized radiation patterns at 0°, 45° and 60° scanning in the D plane: (a) 3 GHz; (b) 5 GHz.
随着扫描角度的增大, 主波束增益遵循cosθ的变化规律, 因此在大角度扫描时, 主波束增益有一定的下降[16]. 从图9—图11也可以看出, 天线阵的方向图有一定的不对称, 这主要是由于馈电结构的不对称所致. 在高频大角度扫描时, 测试增益和仿真增益相比有一定下降, 这主要是由接头、测试线缆的损耗所致.
天线阵边射时, 测试和仿真的同极化增益以及理想口径增益对比如图12所示. 可以看出, 低频时, 测试和仿真的同极化增益和理想口径增益比较符合; 高频时, 测试和仿真的同极化增益和理想口径增益差异增大, 这是由于在高频时交叉极化变差, 线缆和接头的损耗也变大, 导致同极化增益减小. 在5.4 GHz时, 测试的同极化增益比理想口径增益小2.3 dB.
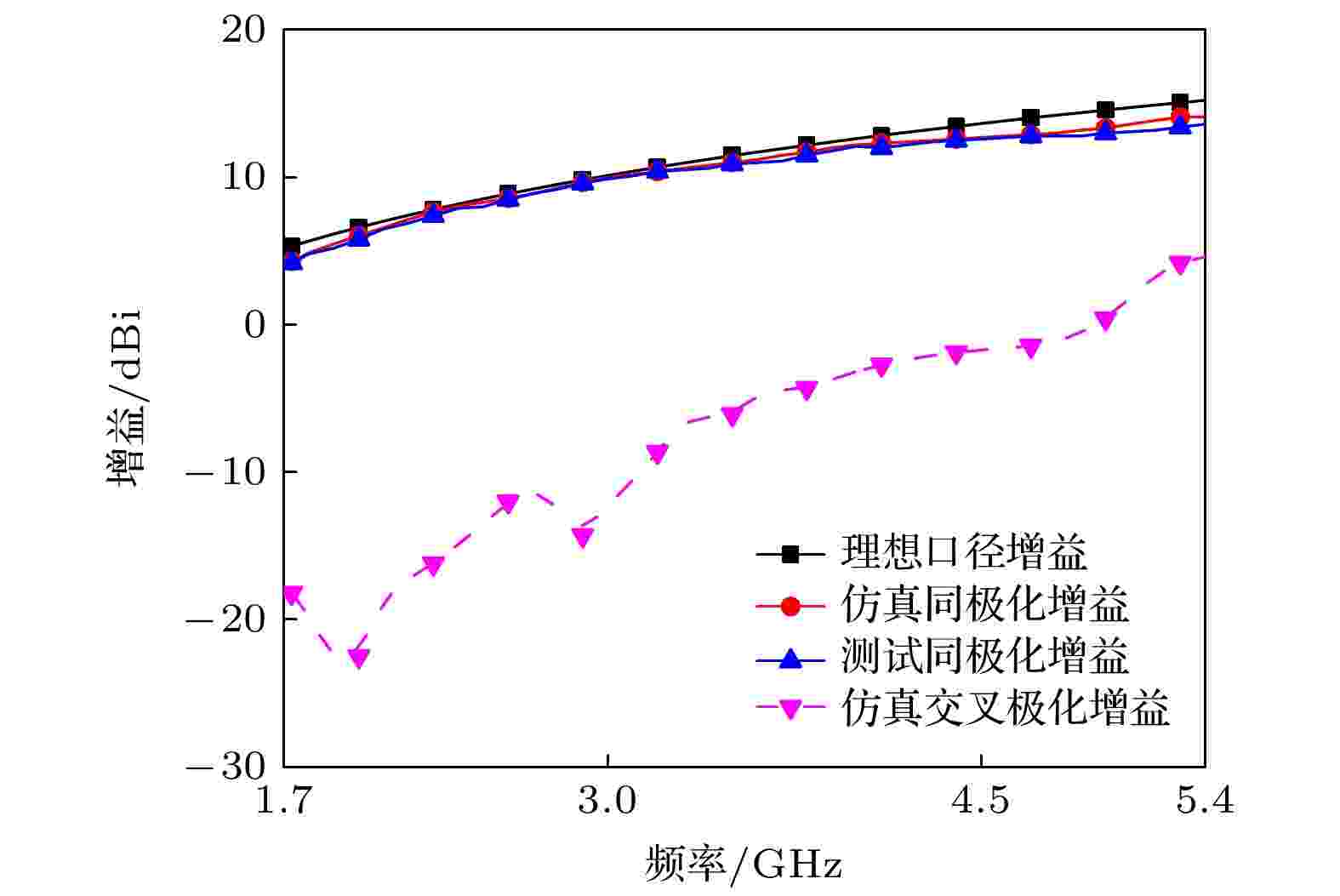
Figure12. Comparisons between measured and simulated results at broadside radiation.
2
3.2.与文献中其他设计的性能对比
假设每个天线单元输入功率为1 W, 工作在边射最高频率, 按相同辐射口径(10λhigh × 10λhigh)换算, 文献[16-21]以及本文设计的天线阵辐射功率对比如表1所列. 可以看出, 由于本文提出的天线单元具有最小的电尺寸, 因此, 在10λhigh × 10λhigh的口径下可以布置多达4822个天线单元; 同时, 本文设计的天线阵具有较高的辐射效率, 最终天线阵辐射功率为4050 W, 是文献中其他阵列辐射功率的5.5倍以上.参考文献 | 工作频率/GHz | 单元周期/λhigh × λhigh | 扫描角度 | 有源驻波比 | 单元个数 | 辐射效率 | 辐射功率/W |
[16] | 0.75—3.85 GHz (5.1∶1) | 0.36 × 0.36 | E-70° H-60° | < 3 | 771 | 95% | 732 |
[17] | 0.68—5.00 GHz (7.35∶1) | 0.45 × 0.45 | E-45° H-45° | < 3 | 493 | 70% | 345 |
[18] | 0.80—4.38 GHz (5.5∶1) | 0.35 × 0.35 | E-70° H-55° | < 3 | 816 | 87% | 710 |
[19] | 3.13—11.63 GHz (3.7∶1) | 0.36 × 0.36 | E-75° H-60° | < 3 | 771 | 84% | 647 |
[20] | 6.5—14.5 GHz (2.23∶1) | 0.45 × 0.45 | E-50° H-50° | < 2 | 493 | 95% | 468 |
[21] | 7.8—13.5 GHz (1.7∶1) | 0.45 × 0.39 | E-70° H-70° | < 2 | 569 | 90% | 512 |
本文设计 | 1.7—5.4 GHz (3.2∶1) | 0.144 × 0.144 | E-65° H-45° | < 2 | 4822 | 84% | 4050 |
表1按相同辐射口径换算, 不同文献中天线阵的辐射功率对比
Table1.Comparisons of radiated power of antenna arrays in literatures on the same conversion size radiation aperture.
需要指出的是, 文献[16-19]中天线阵的带宽和扫描角度是在天线单元有源驻波比 < 3的标准下给出的, 而本文的带宽和扫描角度是在天线单元有源驻波比小于2的更优标准下给出的.