全文HTML
--> --> -->设计制备可控和可重复的表面粗糙的金属纳米结构来获得高密度“热点”可有效提高基底的SERS活性[13-17]. 但二维金属纳米结构为主的SERS基底因表面积有限, “热点”数量一定, 在实际应用及器件性能的提高方面受到很大限制. 近年来, 一些三维纳米结构的SERS基底, 如银修饰锥形氧化锌(ZnO)纳米棒[18], 金修饰二氧化钛(TiO2)纳米管阵列[19], 金修饰碳纳米管阵列[20], 三维碳纤维/氧化锌/银纳米颗粒复合基底等[21], 逐渐成为研究热点. 相较于二维SERS基底, 三维SERS基底具有大比表面积、高孔隙率, 更有利于提高金属纳米颗粒附近探针分子的富集以及单位投影面积上“热点”的数量, 可有效放大金属纳米颗粒附近光场, 进一步增强探测分子的拉曼信号, 在探测高灵敏度的SERS信号方面有独特的优势[22]. Renishaw Diagnostics公司生产的Klarite型倒金字塔型芯片为生命科学和分析化学研究提供了独特的解决方案[23]. 但在实际应用中, 该芯片所采用的倒金字塔结合金纳米颗粒结构还存在一些问题, 如: 裸金纳米粒子易与氨基(NH2), 硫基(S), 或氯(Cl)等基团直接接触, 会导致电子密度分布和吸附发生变化, 从而影响SERS谱图; 分子官能团与金纳米粒子的直接接触会加速分子的光催化反应, 导致分子结构发生变化甚至生成新的产物. 相比较而言, 银纳米材料具有更高的SERS活性, 更适合被用来制备SERS活性基底, 但银纳米材料最大的问题就是不稳定、易被氧化, 也会与某些吸附分子反应, 使拉曼信号强度减弱. Klarite芯片的拉曼激发光波段主要集中在633和785 nm, 而罗丹明6G(R6G)分子的吸收波段在532 nm附近, 且沉积的银纳米颗粒极易被氧化. 为解决这一问题, 聚合物薄膜, 例如聚对苯二甲酸乙二醇酯(PET)[24]、聚甲基丙烯酸甲酯(PMMA)[25]、聚氯乙烯(PVC)[26]等已被用于柔性SERS的应用研究, 这类柔性材料有较高的透光性. 如Zhong等[27]通过在PMMA模板上自组装金纳米颗粒制备了一种柔性SERS基底, 并成功地在鱼鳞上检测到了痕量孔雀石绿. Shiohara等[28]制备了一种由聚二甲基硅氧烷(PDMS)和金纳米星组成的柔性透明底物, 用于原位检测水果果皮上的噻苯达唑残留. 卢思辰等[29]通过电沉积方法在柔性基底PET膜上合成三维花状金微结构作为SERS传感器, 对R6G和福美双进行高灵敏度检测. 到目前为止, 聚合物薄膜在SERS技术中一直作为柔性基底或转移层材料使用, 而研究结果进一步证实, 在多种聚合物薄膜中, 高分子透明材料P MMA具有良好的亲水性, 可以与任何分子的水溶液相结合, 其透光率高达92%, 在可见光波段可以让入射激光几乎无损透过. 且它的高电磁率可以使金属纳米颗粒周围产生较高的局域电场[30]. 若能将PMMA与银纳米颗粒和三维金字塔结构相结合, 不但可以解决银纳米结构的SERS活性不稳定问题, 还可以进一步提升基底的SERS活性.
基于此, 我们研究制备了一种PMMA包裹银纳米颗粒的三维金字塔立体复合SERS基底. 研究结果证实, 入射光在金字塔波谷内的多次有效振荡进一步创建高密度的纳米等离子体“热点”. 此外, 由于PMMA膜的存在为探针分子提供相应集中的热点区, 可在塔基位置获得更加均匀稳定的SERS信号. 且可有效防止银纳米颗粒的氧化, 提高基底的重复使用率与普适性. 通过调控银纳米颗粒在PMMA溶液中的分布密度, 得到在浓度为0.75 g/mL时, 达到最优的SERS活性, 其检测极限的拉曼信号增强因子可高达3.95 × 109. 此研究结果不仅提供了一种高性能、可重复使用的SERS基底的有效策略, 也将会对未来进一步设计改进三维结构的SERS基底产生重要的指导意义.
2.1.材 料
丙酮(CH3COCH3, 99.5%)、乙醇(C2H6O, 99.7%)、乙二醇(C2H6O2, 99.0%)、罗丹明6G(R6G)、硝酸银(AgNO3)和聚甲基丙烯酸甲酯(PMMA)购自国药控股化学试剂有限公司, 所有试剂均未进一步纯化. 聚乙烯吡咯烷酮(PVP, Mw = 55000)购自美国Sigma-Alorich试剂集团.2
2.2.银纳米颗粒的制备
银纳米颗粒的制备参考了Guo等[31]的化学合成制备方法, 以PVP为原料, 在乙二醇溶液的辅助下, 采用水浴法合成了纳米银粒子(AgNPs). 将0.25 g PVP和0.05 g AgNO3依次注入到20 mL的乙二醇溶液中, 持续搅拌混合溶液至油浴温度达到135 ℃左右, 保持1 h, 待混合溶液变为黄色透明胶体时, 停止搅拌并冰浴, 使反应混合物冷却到室温. 再向已冷却的银溶胶中加入60 mL的丙酮溶液促使银纳米颗粒的沉淀, 并将此沉淀用去离子水洗涤多次后, 得到全部银纳米颗粒的沉淀在氮气(N2)氛围下干燥、称量得到相应质量.2
2.3.三维金字塔立体复合SERS基底的制备
复合三维(3D)SERS基底结构示意图如图1所示. 将刻蚀好的具有金字塔结构的硅片(P-Si)依次用丙酮、酒精、去离子水超声清洗各15 min以去除硅片表面残留的有机物等杂质. 0.075 g的PMMA颗粒充分溶解在100 mL的丙酮溶液中形成PMMA丙酮溶液. 再将一定质量的银纳米颗粒分别加入到不同体积的PMMA丙酮溶液, 进行超声处理使其充分混合, 得到不同浓度的银纳米颗粒PMMA丙酮溶液(浓度 = 银颗粒的质量/溶液的体积). 然后将其滴在已清洗好的硅金字塔表面, 自然晾干, 形成复合三维立体包裹银纳米颗粒SERS基底(AgNPs@PMMA/P-Si). 为了进一步对比, 还将同样质量的银纳米颗粒分别加入对应体积的水溶液中进行充分混合, 并将其直接滴在硅金字塔表面及覆盖有PMMA层的硅金字塔表面, 形成AgNPs/P-Si和AgNPs/PMMA/ P-Si SERS基底.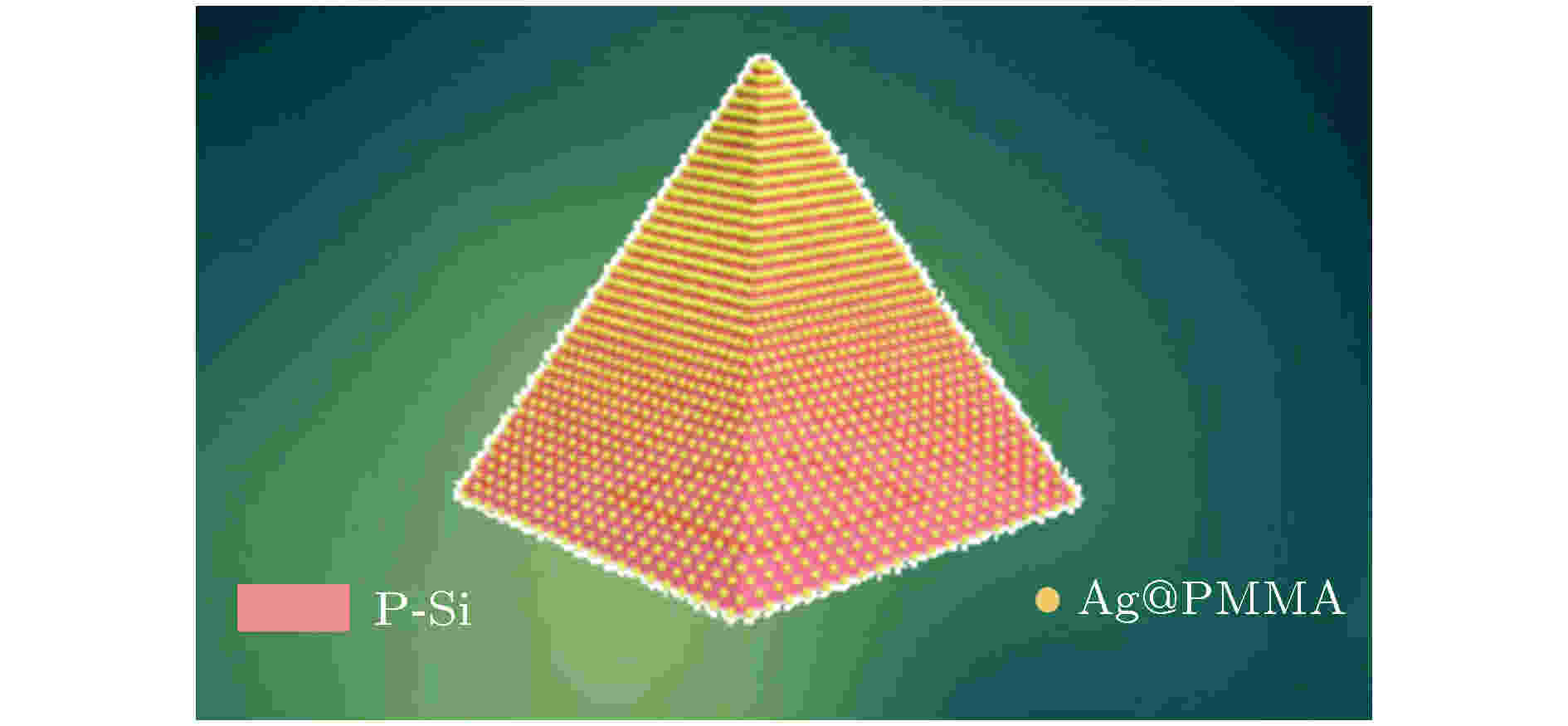
Figure1. Schematic diagram of AgNPs@PMMA/P-Si SERS substrate.
2
2.4.仪器介绍
用扫描电镜(SEM, ZEISS, Gemini Sigma 500)对制备的SERS基底进行形貌表征. 合成的银纳米颗粒及银纳米颗粒PMMA丙酮溶液的紫外可见吸收光谱使用双光束紫外可见分光光度计(TU-1900)进行表征. 使用拉曼光谱仪(Horiba, HR Evolution 800)记录50× 物镜下的SERS谱. 本实验中所使用的激光波长为532 nm, 激光光源的有效功率保持在0.048 mW, 所使用的衍射光栅为600 gr/nm, 积分时间为8 s.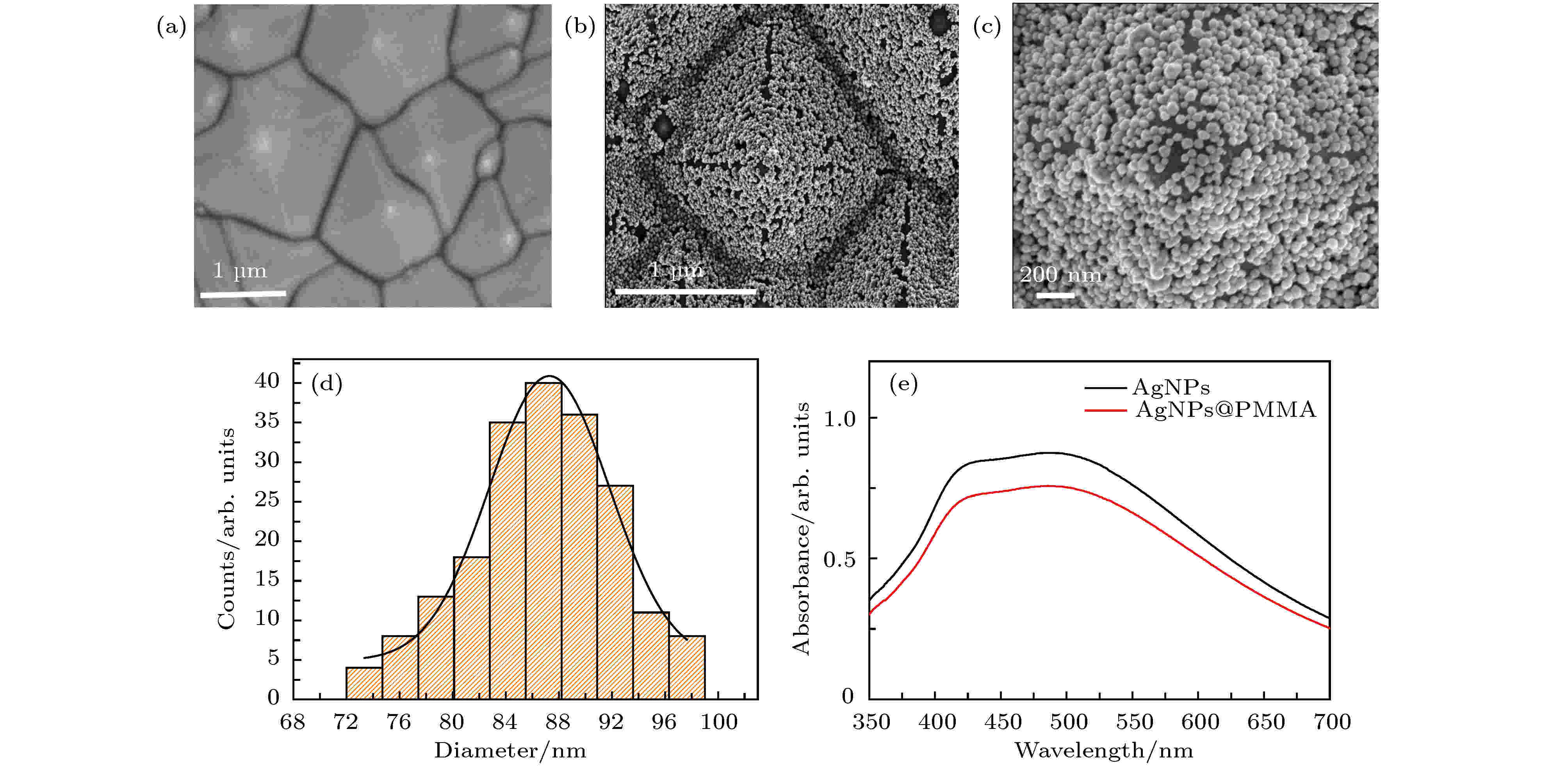
Figure2. (a) SEM image of P-Si substrate; (b) SEM image of AgNPs@PMMA/P-Si substrates with AgNPs concentration of 0.75 g/mL in different magnification; (c) size distribution of AgNPs; (d) UV-vis spectra of AgNPs and AgNPs@PMMA solution.
已有研究表明, 高密度“热点”主要集中在纳米结构的间隙或者尖端处, 通过物理和化学方法控制纳米结构的形状、尺寸和组成可有效提高基底的SERS活性[32,33]. 在本工作中, 银纳米颗粒的空间分布对基底的SERS活性有较大影响, 所以首先通过调节银纳米颗粒PMMA丙酮溶液浓度对复合基底SERS活性进行优化. 图3(a)—图3(e)显示了浓度分别为0.55, 0.65, 0.75, 0.85, 0.95 g/mL的银纳米颗粒PMMA溶液制备的AgNPs@PMMA/P-Si基底的表面形貌. 由SEM图可以看出不同浓度的溶液对基底“热点”的构建影响较大. 在浓度较小时(0.55 g/mL), 银纳米粒子随机分布于金字塔侧面, 粒子分布不均, 呈团簇状分布. 随着浓度的升高, 银纳米颗粒在金字塔侧面分布密度不断提高, 而由于PMMA自身的低黏滞性, 使银纳米粒子不但在塔基位置聚集, 同时也在塔身侧面分布. 且浓度越大, 相邻银纳米颗粒之间间距越小, 这有利于在银纳米颗粒之间产生三维“热点”效应. 而当浓度大于0.75 g/mL之后, 在塔基和侧面的大量银纳米颗粒由于空间分布有限, 在部分区域出现堆叠和团簇现象(图中画圈区域标出), 这不利于“热点”的构建.
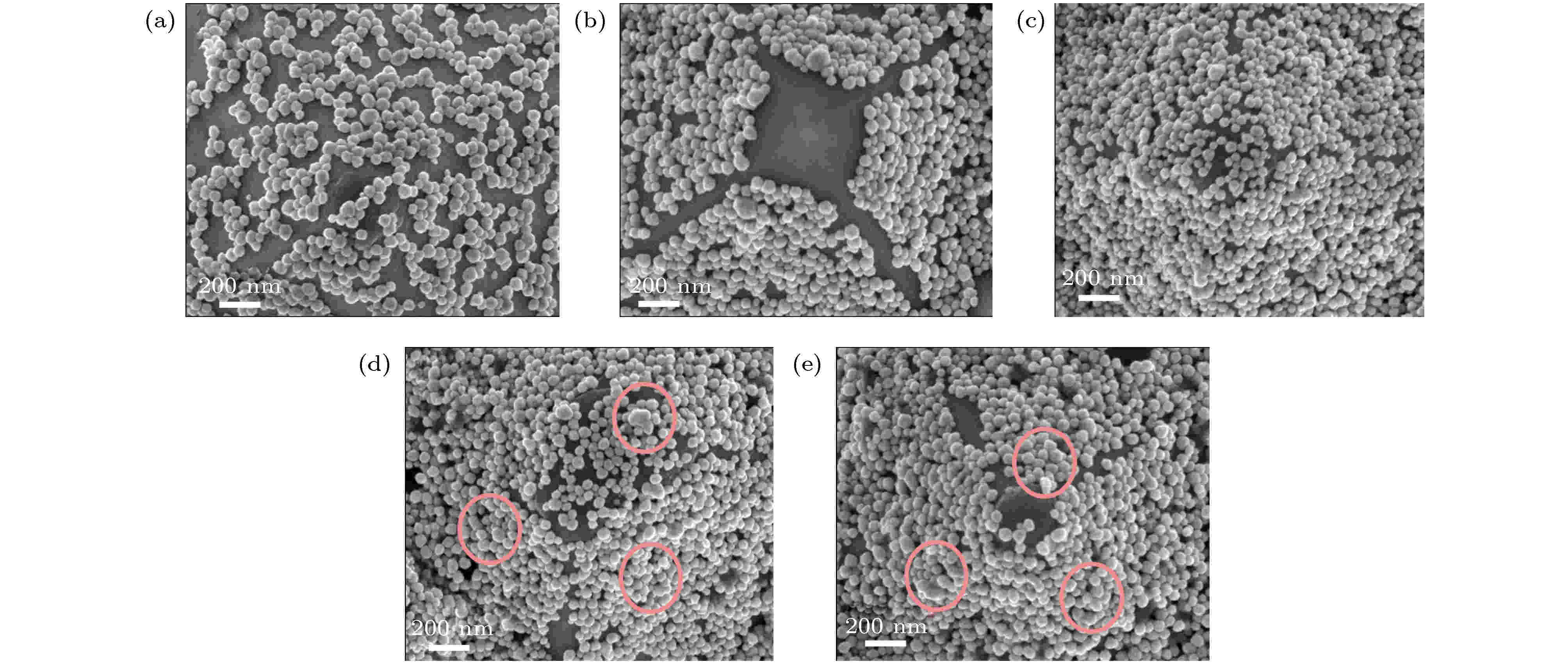
Figure3. (a)?(e) SEM images of AgNPs@PMMA/ P-Si substrates with different concentrations of AgNPs in PMMA acetone solution: (a) 0.55 g/mL; (b) 0.65 g/mL; (c) 0.75 g/mL; (d) 0.85 g/mL; (e) 0.95 g/mL
图4(a)展示了10–7 mol/L的R6G分子吸附在不同混合浓度的三维复合SERS基底上的拉曼光谱. 可以观察到R6G分子的拉曼谱在614, 774, 1183, 1365, 1512和1651 cm–1处显示出明显的特征峰[34]. 为了更直观地显示最优的SERS活性, 我们绘制了在614和774 cm–1特征峰处的拉曼峰强与不同混合浓度的直方图(图4(b)). 可以看出R6G分子的所有拉曼峰强度随银纳米颗粒在PMMA溶液中浓度的增加先增加后减小. 在浓度为0.75 g/mL时, R6G分子的拉曼信号达到最强. 当浓度较低时, 银纳米颗粒数量稀疏, 相应颗粒之间间距较大, 两个银颗粒之间几乎没有相互作用, 只有单个粒子产生的“热点”效应, 导致R6G分子的拉曼信号较弱. 随着银纳米颗粒浓度的增加, 银纳米颗粒的数量增加并且间距减小, “热点”空间区域增大, 拉曼信号逐渐增强. 但当银纳米颗粒浓度超过0.75 g/mL时, 浓度过大导致的银纳米颗粒聚合体不利于强电场的形成, 致使R6G分子的SERS信号减弱. 在我们的实验条件下, 银纳米颗粒浓度为0.75 g/mL时, 复合基底可产生最佳SERS活性.
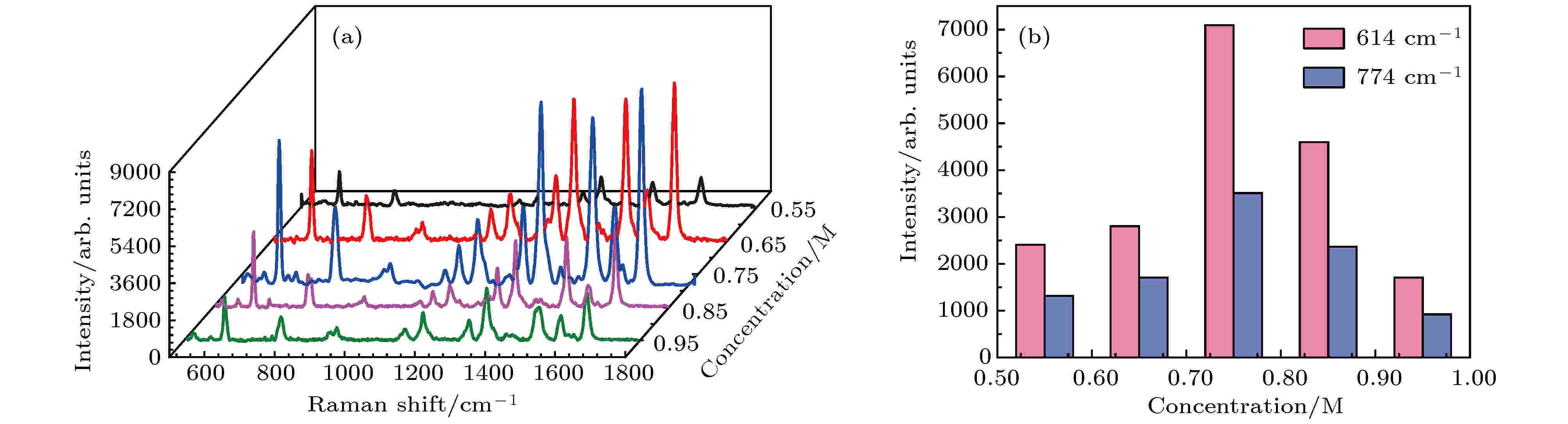
Figure4. (a) SERS spectra of R6G (10–7 mol/L) on the AgNPs@PMMA/P-Si substrates fabricated with different concentrations; (b) corresponding to the Raman peak at 614 and 774 cm–1 for the R6G molecule in Fig. (a).
图5(a)为不同浓度R6G分子吸附在银纳米颗粒浓度为0.75 g/mL的三维复合结构SERS基底上的拉曼光谱. 随着R6G分子浓度的降低, SERS信号有明显的下降趋势. 从图中可以看出, 在10–14 mol/L浓度时, 已有部分拉曼峰无法分析. 因此, 该基底的最低检测浓度可达到10–13 mol/L. 我们选择了614和1365 cm–1位置的峰值来进一步表明SERS信号强度和R6G分子浓度的关系. 如图5(b)所示, 两个R6G分子的拉曼峰的信号强度都随着浓度的变化呈现出良好的线性关系, 拟合之后的线性系数分别高达0.984和0.983. 选用614 cm–1的峰值计算了三维复合SERS基底的拉曼增强因子(enhancement factor, EF)[34], 其示意图如图5(c)所示, 公式为
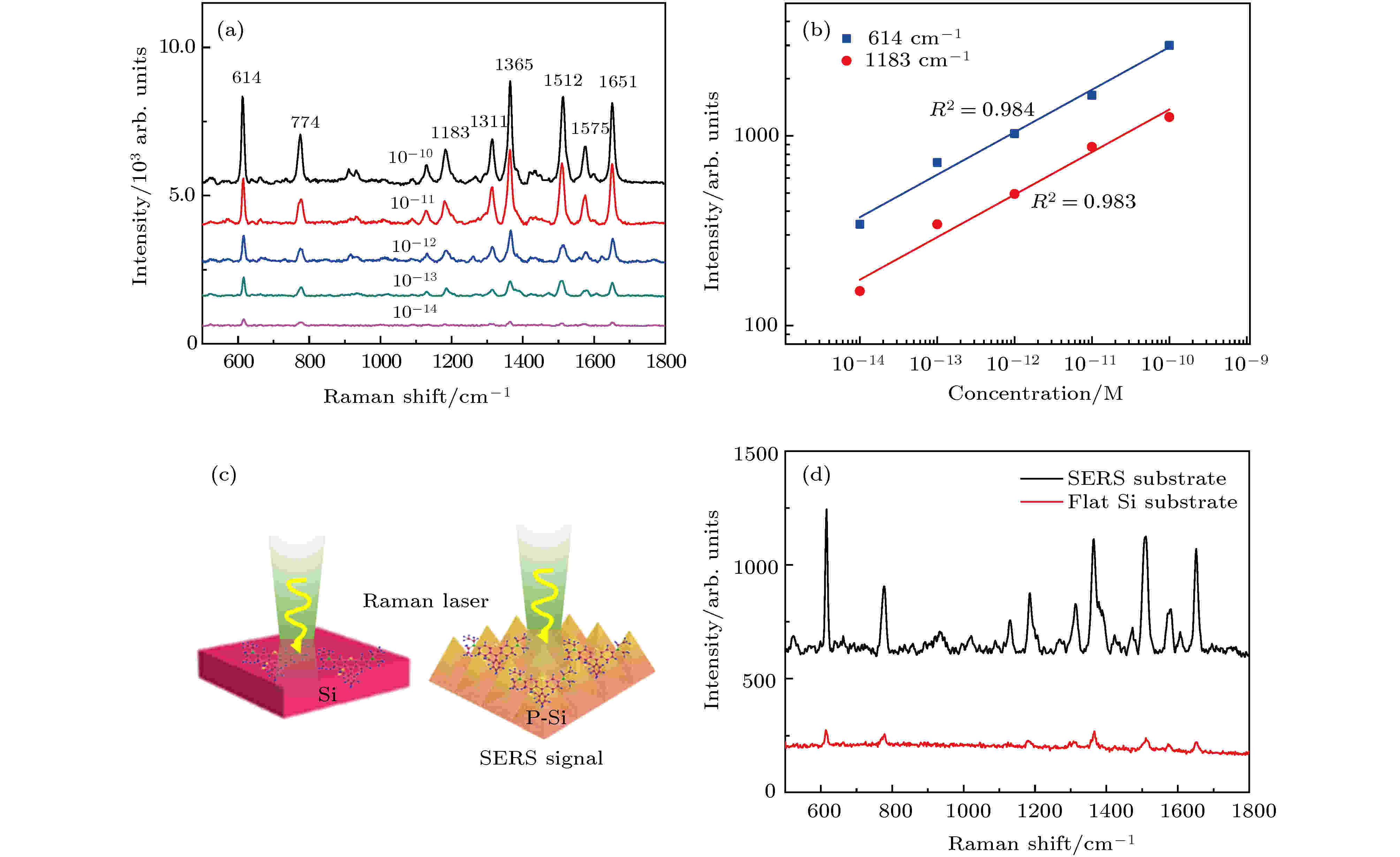
Figure5. (a) SERS spectra of R6G with concentrations from 10–10 to 10–14 mol/L on the AgNPs@PMMA/P-Si with AgNPs concentration of 0.75 g/mL substrate; (b) the intensity of R6G peak at 614 cm–1 and 1365 cm–1 changes as a function of R6G molecule concentration on the AgNPs@PMMA/P-Si substrates; (c) schematic illustration showing the Raman detection of SERS substrates; (d) comparison of the detection limit of the R6 G molecule on the 3D composite SERS substrate (10–13 mol/L) and the detection limit (10–4 mol/L) on the planar Si substrate.


在0.75 g/mL浓度的AgNPs@ PMMA/P-Si基底上对10–7 mol/L的R6G水溶液的拉曼光谱进行检测并选择了图中所示的方框区域内随机选择10个活性热点进行多次重复测量(图6(a)). 在三维立体金字塔结构中, 金字塔的倾斜面结构有利于水溶液中的探针分子在塔基底部聚集, 同时金字塔型可提供一个大的比表面积, 因此在单位投影面积上可以吸附更多的银纳米颗粒, 使探针分子聚集的同时又产生大量的“热点”. 图6(b)为相应光谱中614 cm–1峰的强度分布, 黑线表示10个峰值的平均强度, 最大强度偏差(D)按照以下公式计算:
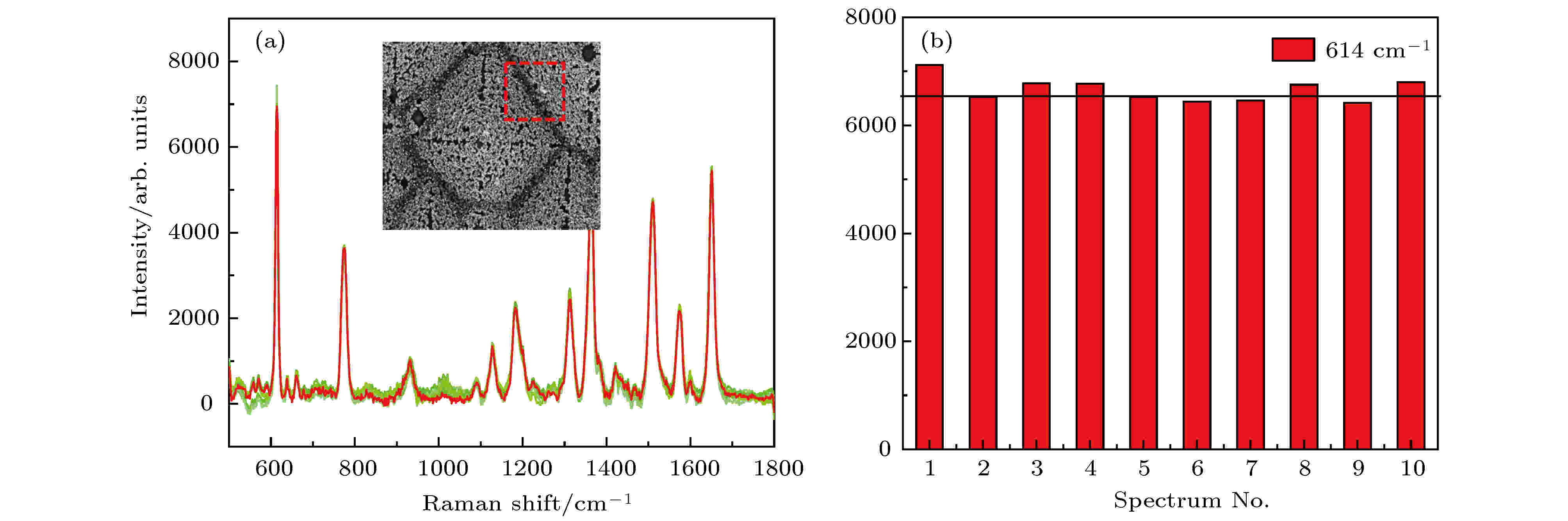
Figure6. (a) Average SERS spectrum of the 10–7 mol/L R6G molecules from 10 random positions in the base tower of AgNPs@ PMMA/P-Si with concentration of 0.75 g/mL substrate (red line is the average of 10 SERS spectrum lines); (b) statistical diagram of variation of Raman peak intensity at 614 cm–1 is in the corresponding Fig. (a).

我们对比了10–7 mol/L R6G分子分别吸附在AgNPs/PMMA/P-Si, AgNPs/P-Si以及AgNPs@ PMMA/P-Si三种SERS复合基底的SERS光谱(图7(a)). 可以看到, 全包裹型复合基底(AgNPs@PMMA/P-Si)的SERS活性要高于相应条件下其他两种基底. 根据文献表明[27], PMMA材料的电磁率高于空气, 银纳米颗粒产生电磁场效应在PMMA中产生影响空间范围较大, 使产生“热点”作用的范围扩大, 也就意味着会有更多的吸附分子会被作用. 因此, 收集到的分子的拉曼信号会被加强, 产生相应的SERS效应. 图7(b)进一步证明了所制备的SERS基底的稳定性. 随时间的增加, 在AgNPs@PMMA/P-Si基底上采集得到的R6G分子的SERS光谱有轻微的衰减, 而在AgNPs/PMMA/P-Si的SERS基底上, 其SERS强度表现出明显降低. 这是因为暴露在空气中的银纳米颗粒容易与空气中的成分反应, 极易被氧化, 而将银纳米颗粒嵌入PMMA材料中, 不仅可以有效防止银颗粒与周围环境相接触造成氧化, 还可以有效避免银纳米颗粒与探针分子直接接触引起的吸附探针分子变形导致的信号失真等问题.
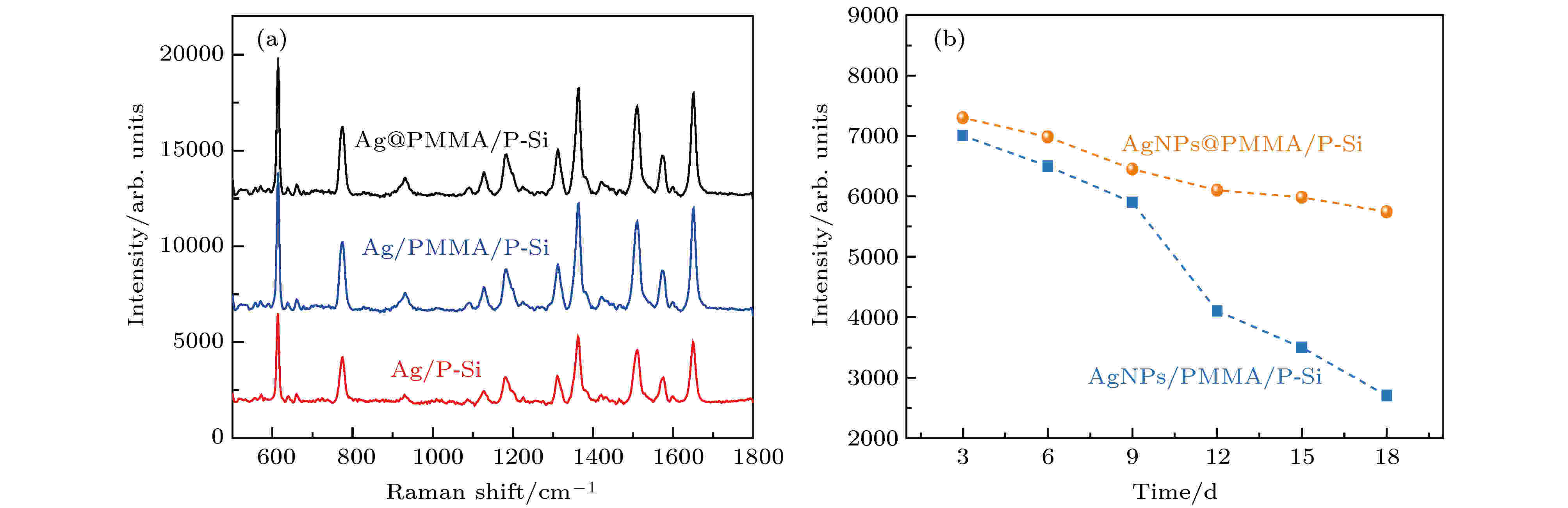
Figure7. (a) The Raman spectra of R6G molecule with 10–7 mol/L on the AgNPs@PMMA/P-Si, AgNPs@PMMA/F-Si, and AgNPs/P-Si substrates; (b) the intensity of the signal at 614 cm–1 of R6G (10–7 mol/L) collected with 18 days every three days respectively on the AgNPs@PMMA/P-Si and AgNPs/PMMA/F-Si substrate.