全文HTML
--> --> -->基于p-i-n结构的PeLEDs器件的空穴传输层通常为PEDOT:PSS, 而PSS的不稳定性促使一些科学家研究n-i-p结构的钙钛矿发光器件来避免使用PEDOT:PSS材料, 其中电子注入材料对n-i-p型PeLEDs器件性能影响很大. Yu等[15]引入乙醇胺(EA)溶剂对TiO2层表面进行处理, 降低TiO2层和钙钛矿层之间的注入势垒并钝化了TiO2的表面, 显著增强了电子提取和注入, 减少TiO2层的缺陷, 从而实现高效的钙钛矿光电子器件, 报道了绿光PeLEDs器件结构为ITO/TiO2/EA/MAPbBr3/SPB-02T/MoO3/Au, 最大亮度为544 cd·m–2, 最大EQE为0.051%. Friend课题组[16]在ZnO电子传输层和钙钛矿发光层之间引入聚乙烯亚胺(PEI)中间层, PEI的高浸润性表面促进高质量钙钛矿膜的形成, 并且可改性ZnO层[17], 降低ZnO的功函数以改善电子注入. 制备的PeLEDs器件结构为ITO/ZnO/PEI/钙钛矿/TFB/MoOx/Au, 其开启电压很低, 表明了载流子有效的注入和复合[16]. 基于相似的策略, Wang等[18]在ZnO层的顶部使用基于氨基酸的自组装单分子层, ZnO和羧基连接, 氨基可以改善ZnO层的润湿性以促进MAPbBr3钙钛矿的成核生长. 这种表面改性策略, 与氯苯辅助快速结晶方法配合, 导致钙钛矿良好的表面覆盖率, 降低了钙钛矿薄膜的缺陷态密度, 最终制备的PeLEDs实现了0.43%的EQE和5000 cd·m–2的亮度. Shi等[19]使用CsPbBr3量子点作为有源层, n-MgZnO作为电子注入材料, p-MgNiO作为空穴注入材料. n-MgZnO具有致密堆积结构, 溅射的MgZnO层不仅可以对水分子扩散起到阻挡作用, 而且还可以将钙钛矿发光层与金属隔离开来, 展示了高效稳定的PeLEDs, 最佳的PeLEDs实现了3809 cd·m–2的亮度, 最大EQE为2.39%. Zhang等[20]采用无机CsPbBr3钙钛矿与少量MABr混合形成高质量钙钛矿薄膜, 在ZnO层和钙钛矿层之间引入聚乙烯吡咯烷酮(PVP)绝缘层实现平衡的电子空穴注入, 得到最大EQE为10.4%的绿光PeLEDs器件. 最近Chiba等[21]使用含卤素离子的烷基铵(OAM-I)和芳基铵盐(An-HI), 让其I–与CsPbBr3钙钛矿的Br–阴离子交换, 使制备的钙钛矿量子点发光红移至649 nm. 使用酯溶剂乙酸乙酯(AcOEt)作为洗涤溶剂来阻止钙钛矿量子点中的阴离子缺陷, 最终开发出EQE效率达21.3%且高色纯的深红色钙钛矿量子点发光器件.
本文提出采用等离子增强化学气相沉积(PECVD)的方法制备n型纳米晶硅氧(n-nc-SiOx:H)电子注入层, 并将其应用至钙钛矿发光器件中, 相较于SnO2材料等, 其拥有着更加匹配的能级结构、更高的电子迁移率. 另外, 向钙钛矿材料中引入合适比例的甲基溴化胺(MABr)、反溶剂中引入一定量的苯甲胺(PMA), 通过MABr和PMA的协同作用进一步改善了钙钛矿薄膜的结晶质量, 减少了钙钛矿薄膜表面的非辐射复合中心, 改善了电荷的注入平衡, 显著提升了PeLEDs的器件性能.
2.1.材料准备
钙钛矿材料(MAPbBr3)的制备: 使用DMF和DMSO (3︰7 v/v)混合溶剂, 溶解制备0.65 mol/L的甲基溴化胺(MABr, 上海迈拓崴公司, 纯度99%)以及0.5 mol/L的溴化铅(PbBr2, 美国Sigma-Aldrich公司, 纯度99.999%), 60 ℃加热搅拌12 h使溶质完全溶解.钙钛矿材料(CsPbBr3)的合成与提纯: 称取10 mmol的溴化铯(CsBr, 美国Sigma-Aldrich公司, 纯度99.999%)溶于3 mL水中, 向PbBr2溶液(10 mmol, 溶于8 mL氢溴酸溶液)中, 一边搅拌一边逐滴缓缓滴加CsBr溶液, 产生橙黄色的钙钛矿沉淀. 用无水乙醇洗涤离心钙钛矿沉淀四次, 将得到的橙黄色沉淀物置于烘箱中60 ℃下干燥12 h以备使用.
氯苯(CB, 纯度99.5%)、苯甲胺(PMA, 纯度99.00%)、氢溴酸(48 wt.%的HBr水溶液)和三氧化钼粉末(MoO3, 纯度99.9%)购自Aladdin公司; 4,4-二(9-咔唑)联苯(CBP, 纯度99.9%)购买自西安宝莱特公司.
2
2.2.器件制备
ITO玻璃按顺序依次使用工业清洗剂、去离子水、酒精和异丙醇4个步骤的超声振荡清洗, 每个步骤均持续15 min. 将清洗后的ITO玻璃用氮气枪吹干后, 在ITO表面采用PECVD方法沉积n型纳米晶硅(n-nc-Si:H)和n型纳米晶硅氧(n-nc-SiOx:H), 然后使用等离子清洗机处理ITO表面15 min以增加ITO表面浸润性. 钙钛矿层采用一步法工艺[22], 取70 μL钙钛矿滴加到电子注入层表面, 第一阶段先低速1000 r/min旋涂5 s, 第二阶段以4000 r/min旋涂50 s, 100 μL氯苯反溶剂在第二阶段进行26 s时滴加, 将得到的钙钛矿薄膜基片以90 ℃退火5 min, 退火后的基片于手套箱中冷却至室温. 然后, 在基片上以2000 r/min旋涂浓度23 mg·mL–1的CBP溶液得到器件的空穴注入层, 最后采用热蒸发法在真空腔室压强为4 × 10–5 Pa下, 分别以0.1 ?/s和1.0 ?/s的蒸发速率蒸镀20 nm厚的MoOx层和80 nm厚的铝电极, 器件的有效发光面积为8.565 mm2.2
2.3.测试与表征
钙钛矿薄膜的光吸收透过特性通过紫外-可见-近红外光谱仪来测试(Cary 5000 UV-vis-NIR), 测试波长范围为300—800 nm. 钙钛矿薄膜的表面形貌及粗糙度由原子力显微镜测试获得(NanoNavi-SPA400). 钙钛矿表面的扫描电子显微镜图由(JEOL JSM-7500F)获得. 钙钛矿薄膜的PLQY、光致发光(PL)光谱和时间分辨光致发光(TRPL)光谱由光致发光光谱仪(Edinburgh Instruments, FS5)测试获得. 薄膜物质结构分析特性使用X 射线衍射分析仪(Rigaku ATX-XRD), 以Cu Kα作为射线源(λ = 1.5405 ?), 在10°—90°的2θ范围内测试获得. 钙钛矿发光器件的电致发光特性由Keithley 2400数字源表获得, 与光谱扫描仪(Photo Research PR-735)配合使用, 测试所加偏压为0 V到6 V, 偏压间隔为0.2 V, 发光器件未进行封装, 测试环境为室温下氮气氛围.3.1.基于n-nc-SiOx:H的PeLEDs的结构设计
应用于PeLEDs器件的有机空穴注入材料的载流子迁移率(~10–4—10–2 cm2·V–1·s–1)通常比电子注入材料的载流子迁移率高一两个量级, 从而导致钙钛矿发光器件中电子和空穴注入速率的不平衡, 降低了PeLEDs的发光效率[23]. 因而可以引入电子迁移率相对较高的无机电子注入层, 如ZnO, TiO2和SnO2等来制备n-i-p型PeLEDs. 但是钙钛矿材料在ZnO薄膜上成膜质量差, 而TiO2的导电性不好, 电导率较低至约1.1 × 10–5 S·cm–1, 不利于改善钙钛矿发光器件注入不平衡的问题[24—26]. 如图1(a)所示, SnO2材料的导带约为4.2 eV, 与钙钛矿层之间存在较大的电子注入势垒, 也不适宜作为高效的电子注入材料直接应用于钙钛矿发光器件[27].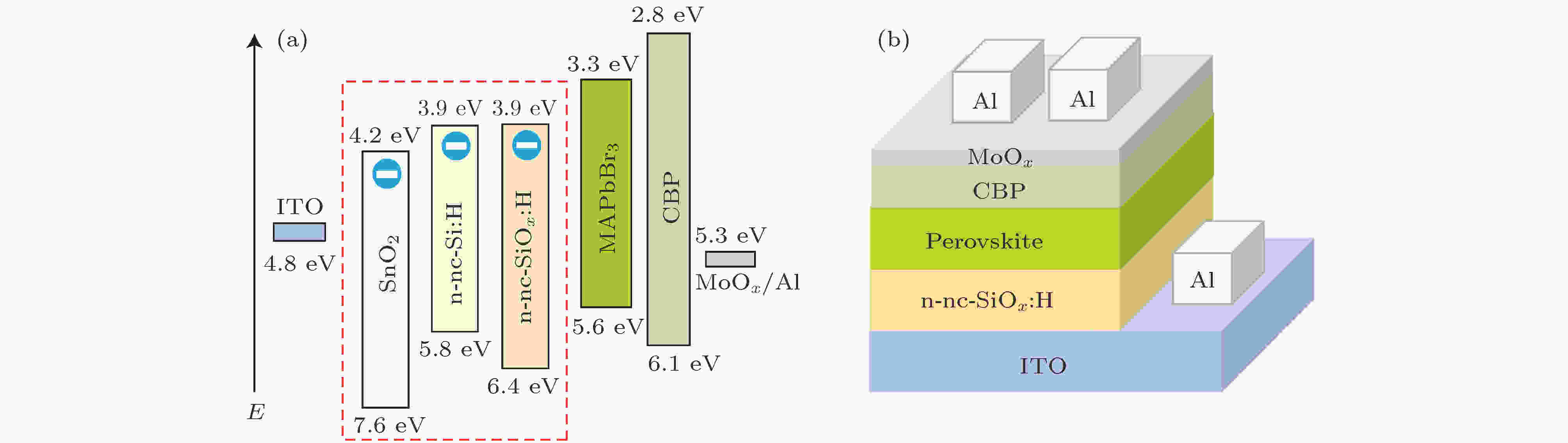
Figure1. Energy-level diagram and device structure: (a) band alignment of each functional layer; (b) structure diagram of PeLEDs device.
根据固体材料中Richardson-Schottky方程[28]:


本文制备的n-i-p型PeLEDs的器件结构见图1(b): ITO/电子注入层/钙钛矿/CBP/MoOx/Al. 该结构中, 采用PECVD方法制备两种新型电子注入材料, n型纳米晶硅(n-nc-Si:H)和n型纳米晶硅氧(n-nc-SiOx:H). 它们拥有着更匹配的能级及较高的电导率, n-nc-SiOx:H的电导率约为3.20 × 10–2 S·cm–1, n-nc-Si:H的电导率约为3.26 S·cm–1. 相较于n-nc-Si:H, n-nc-SiOx:H是在PECVD制备过程中通入一定量的二氧化碳(CO2)作为气源实现氧原子的掺杂, 其禁带宽度进一步拓宽至约2.5 eV, 且光学折射率也大幅降低[30,31], 这使得n-nc-SiOx:H作为电子注入层更有利于PeLEDs器件中光的有效提取.
2
3.2.基于n-nc-Si:H和n-nc-SiOx:H的钙钛矿发光器件的初步研究
从图2(a)可以看出, n-nc-Si:H的表面粗糙度(13.2 nm)明显较n-nc-SiOx:H (5.4 nm)更高, 因而基于这两种电子注入层所生长的MAPbBr3钙钛矿薄膜因衬底不同而有所差异. 在衬底上使用一步旋涂溶液法制备钙钛矿薄膜, 并对所制备的薄膜进行X射线衍射分析, 结果如图2(b)所示. 以n-nc-Si:H和n-nc-SiOx:H为电子注入层所生长的钙钛矿薄膜均在14.94°, 30.14°出现了明显的峰值, 分别对应MAPbBr3晶体的(001), (002)衍射峰. 随着电子注入层从n-nc-Si:H到n-nc-SiOx:H的改变, 对应于(001), (002)衍射峰也逐渐提高, 说明晶体的结晶性有所提高. 图2(c)中, 对所制备的薄膜进行PL光谱分析, 可以看到, 基于n-nc-Si:H电子注入层的钙钛矿薄膜的PL峰值较低, 其半高宽为22 nm. 当采用n-nc-SiOx:H作为电子注入层制备钙钛矿薄膜, 其PL峰值增高, 更利于载流子的辐射复合发光, 同时PL峰半高宽略有降低, 亦证明此时钙钛矿薄膜的结晶质量更高. 如表1所列, 以n-nc-SiOx:H为电子注入层所制备的器件, 其性能显著提升, 最大亮度(Lmax)达到2100 cd·m–2, 最大电流效率(CE)为1.37 cd·A–1, 最大EQE为0.43%, 实验上表明了n-nc-SiOx:H可以作为新型电子注入材料应用于PeLEDs中.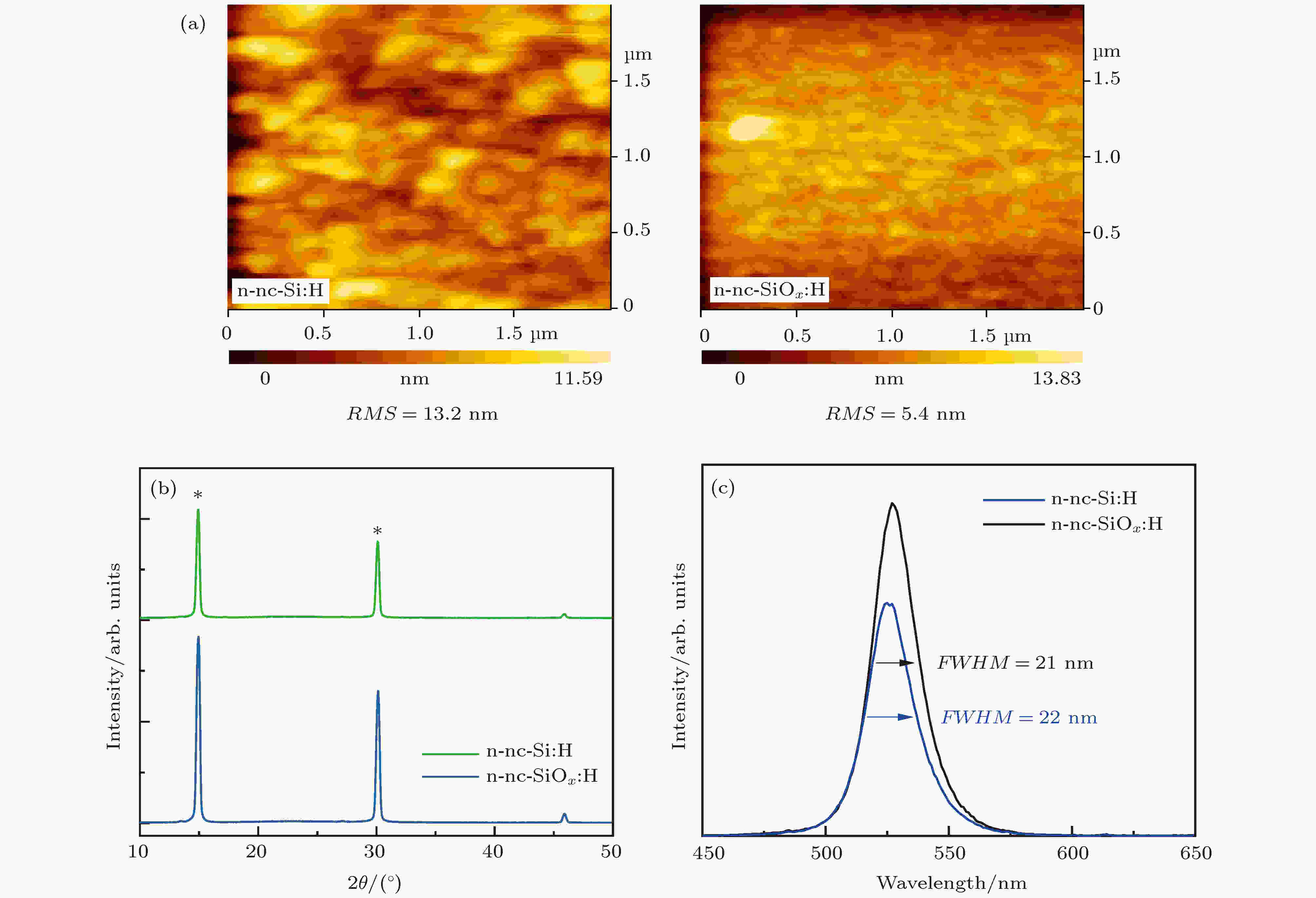
Figure2. Influence of different substrates on perovskite films: (a) Atomic force microscopy images of different substrate surfaces; (b) X-ray diffraction patterns of perovskite films on different substrates; (c) photoluminescence spectra of perovskite films on different substrates.
电子注入层 | Lmax/cd·m–2 | CE/cd·A–1 | EQE/% |
n-nc-Si:H | 650 | 0.4 | 0.1 |
n-nc-SiOx:H | 2100 | 1.37 | 0.43 |
表1基于两种不同电子注入层的PeLEDs器件性能的比较
Table1.Performance of PeLEDs based on different electron injection layers.
2
3.3.基于n-nc-SiOx:H的PeLEDs器件性能提高的研究
为了进一步降低电子注入势垒, 采用无机CsPbBr3钙钛矿材料, 其导带约为3.6 eV, 相较于MAPbBr3材料, 电子注入势垒降低了一半. 而且, 无机CsPbBr3钙钛矿具有更好的热稳定性, 更平衡的电子空穴迁移率-寿命乘积(μτ), 更长的载流子寿命(2.5 μs)等优点[32]. 但是无机CsPbBr3钙钛矿薄膜覆盖率较低, 薄膜孔洞较多导致缺陷复合严重.图3(a)所示为采用三种钙钛矿成膜工艺来试图改进钙钛矿薄膜质量. 方法一是在采用一步法旋涂工艺, 采用氯苯反溶剂, 得到的CsPbBr3钙钛矿薄膜, 其表面粗糙度为28.7 nm, 从图3(b)可看出, 钙钛矿薄膜的孔洞很多, 制备的钙钛矿发光器件无法启亮, 无器件效率.
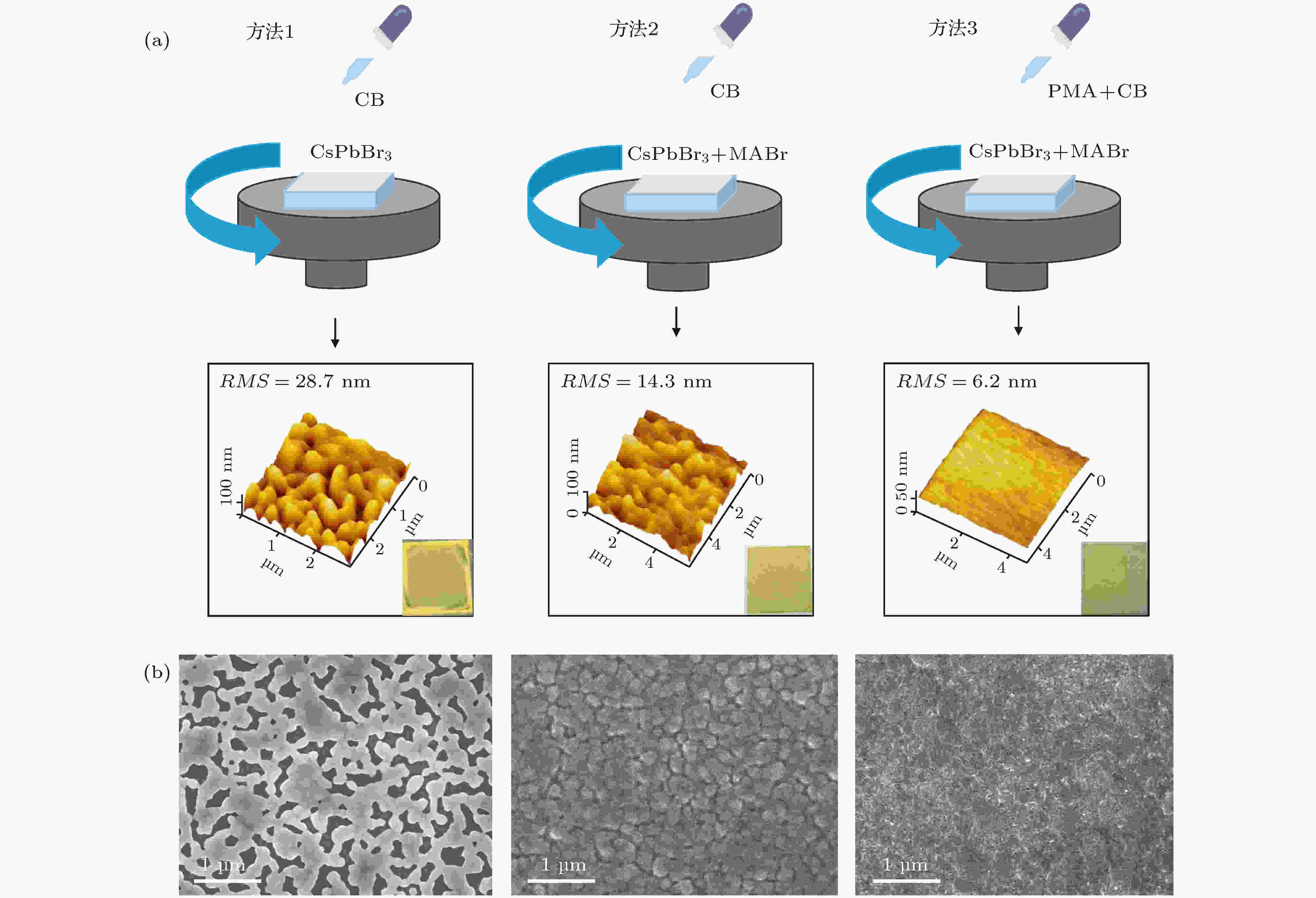
Figure3. Synthesis of perovskite film: (a) Different fabrication processes of perovskite films and the corresponding atomic force microscopy images and photographs; (b) planar scanning electron microscopy images of the perovskite films based on different fabrication processes.
方法二是在CsPbBr3钙钛矿前驱液中加入不同摩尔比(20%, 40%, 60%, 80%, 100%, 120%)的MABr, 一步法旋涂完的钙钛矿薄膜的表面孔洞明显减少, 粗糙度平均下降到14.3 nm, 但是方法二的钙钛矿薄膜退火之后, 其PL的热猝灭现象很严重, 不同MABr浓度下的钙钛矿薄膜的PLQY均很低(< 10%), 所制备发光器件的EQE低于0.5%.
方法三则是在氯苯反溶剂中加入一定体积的PMA试剂. PMA作为一种大体积的胺配体, 常用来减缓钙钛矿的结晶速率[33,34]. 通过钙钛矿成核过程中MABr和PMA的共同作用, 使得钙钛矿表面粗糙度进一步下降至6.2 nm, 薄膜表面的孔洞显著减少. 我们推测产生这一现象的原因是: 一步法钙钛矿成核过程中, 纯无机钙钛矿CsPbBr3与有机分子PMA之间的结合力较差, 无法有效包覆在钙钛矿晶粒表面, 因而无法抑制钙钛矿的快速结晶生长. 此外, 密度泛函理论表明CsPbBr3中存在的卤素空位(VBr)会在带隙内产生缺陷, 可能成为非辐射复合中心[35], 不利于获得优异的钙钛矿发光器件. 而在钙钛矿中引入MABr, 一方面是因为MABr可以钝化钙钛矿薄膜缺陷[20], 前驱体溶液中富含Br会抑制薄膜中VBr的形成, 从而降低非辐射复合; 另一方面由于MABr在DMSO中的溶解度远远大于CsPbBr3, 因而在一步法旋涂过程中CsPbBr3优先析出成核, MABr随后析出并包附在CsPbBr3晶粒表面[36], 此时加入的氯苯反溶剂中的PMA和有机分子MABr之间有着很好的结合力, 从而使得PMA和MABr共同作用来延缓钙钛矿晶体的生长, 减少钙钛矿薄膜中的孔洞. 如图4(a)所示, 当MABr的浓度不低于60%时, MABr与PMA的协同作用有助于抑制钙钛矿的发光猝灭. 向CsPbBr3钙钛矿中引入不同摩尔比的MABr (60%, 80%, 100%, 120%), 如果反溶剂氯苯中不加入PMA, 条件简写作0.6-CB, 0.8-CB等. 测试不同条件下薄膜的吸收度曲线, 结果如图4(b)所示, 钙钛矿薄膜的吸收峰不变, 均在530 nm处. 如果在反溶剂氯苯中加入1.0 vol.%的PMA (简写作0.6-PMA等), 滴加的反溶剂体积均为100 μL, 从图4(c)可知钙钛矿薄膜的PL谱随着MABr比例的增加而不断蓝移, 而图4(b)显示MABr含量的增加不会改变钙钛矿材料的带隙, 我们推测发光蓝移是因为随着有机分子MABr量的增加, 反溶剂中更多的有机分子PMA得以和MABr结合包附钙钛矿晶体, 并有部分PMA进入CsPbBr3/MABr晶体中, 改变了钙钛矿的禁带宽度. 实验过程中发现, 使用方法三刚旋涂完的钙钛矿薄膜在紫外灯的激发下表面微微泛蓝, 随着退火过程中PMA的挥发以及钙钛矿晶体尺寸的增大, 退火后的钙钛矿薄膜发生了明显的发光红移, 其在紫外灯激发下成绿色.
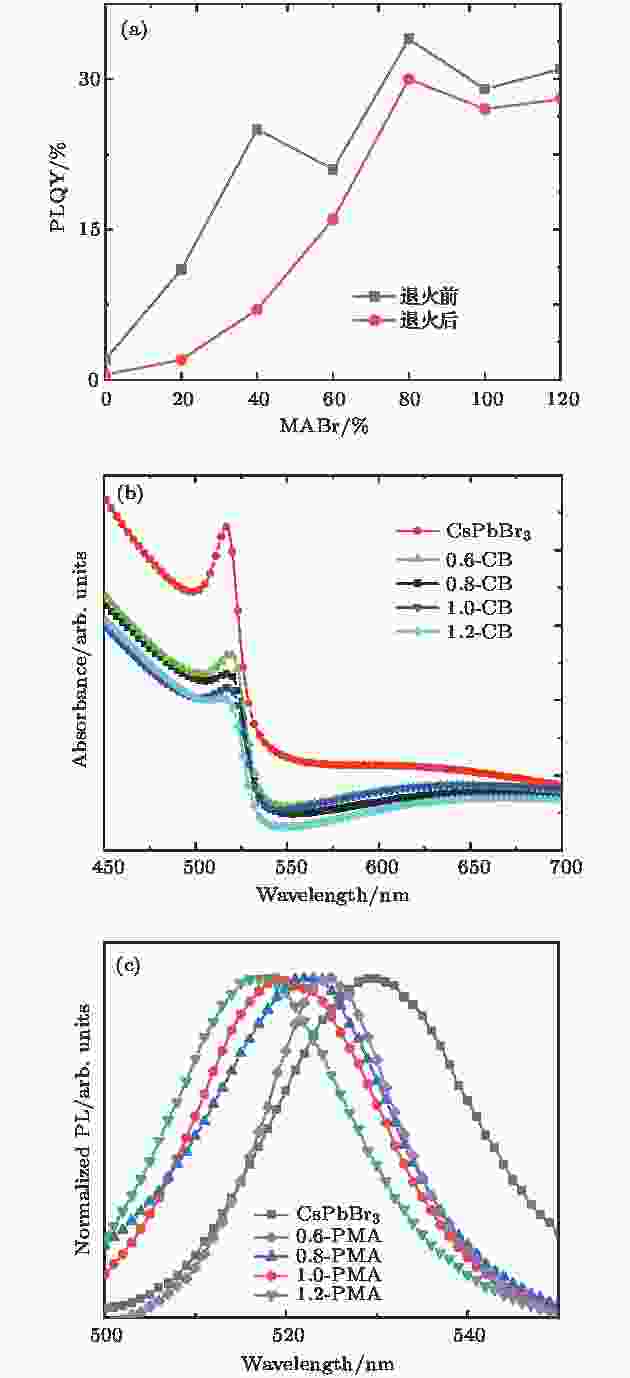
Figure4. Optical characterization of perovskite films: (a) PLQY of perovskite films before and after annealing at different concentrations of MABr; (b) absorbance spectra of perovskite films; (c) normalized PL spectra of perovskite films.
光致发光衰退反映了器件工作时的非辐射复合过程, 其衰退曲线可以用(2)式进行拟合:
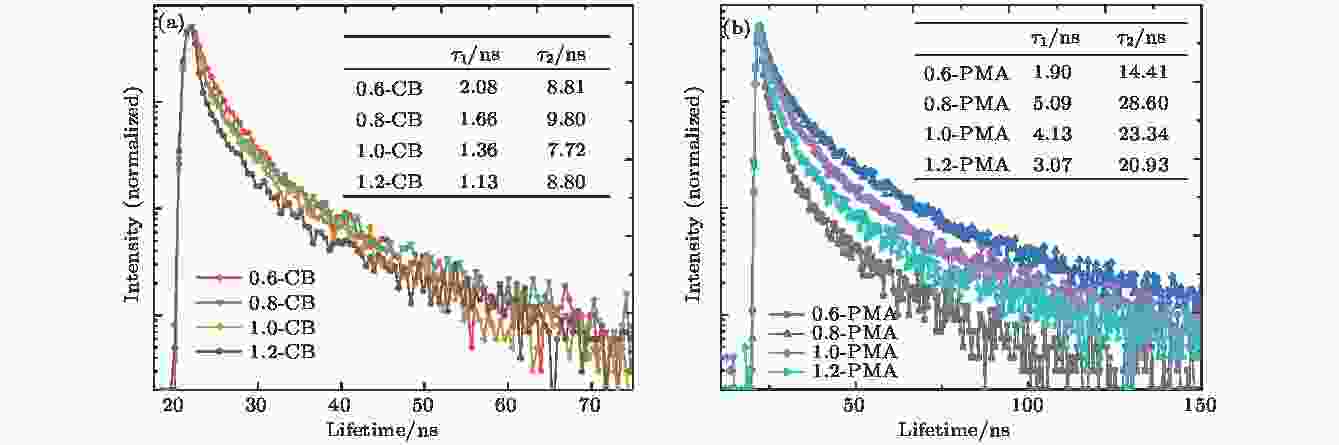
Figure5. TRPL spectra of perovskite films on n-nc-SiOx:H: (a) TRPL spectra of perovskite films at different MABr concentrations without PMA additive; (b) TRPL spectra of perovskite films at different MABr concentrations with PMA additive.
将优化后的钙钛矿成膜工艺应用到以n-nc-SiOx:H为电子注入层的钙钛矿发光器件中, 得到的PeLEDs电致发光表现如图6(a)—(c)所示. 随着外置电压的升高, 注入电子空穴能力更强, 器件的电流密度随之提高. 不断注入的电子空穴使得钙钛矿发光层内辐射复合概率增加, 器件的光强也随之不断变高. 可看出, 器件的开启电压为3.4 V, 在偏置电压为5 V, 电流密度为4.7 mA·cm–2时, 器件的CE及EQE达到了最高值, 之后器件效率开始滚降, 随着电压的升高, 器件性能不断衰退, 这可能是源于俄歇复合所导致的发光猝灭, 或是由电流热效应导致钙钛矿内部不断积聚的热量所引发的[8,37]. 图6(d)所示为PeLEDs器件的CIE 1931色度图, 根据发光的CIE坐标可知该PeLEDs为绿光器件. 最终基于n-nc-SiOx:H电子注入材料, 通过优化钙钛矿成膜工艺, 获得了最大CE为7.93 cd·A–1, EQE为2.13%的n-i-p型PeLEDs, 器件性能较钙钛矿层优化前的 0.43%有了明显提升, 后续可通过继续改进器件结构, 提高钙钛矿薄膜质量来进一步提升PeLEDs的发光性能.
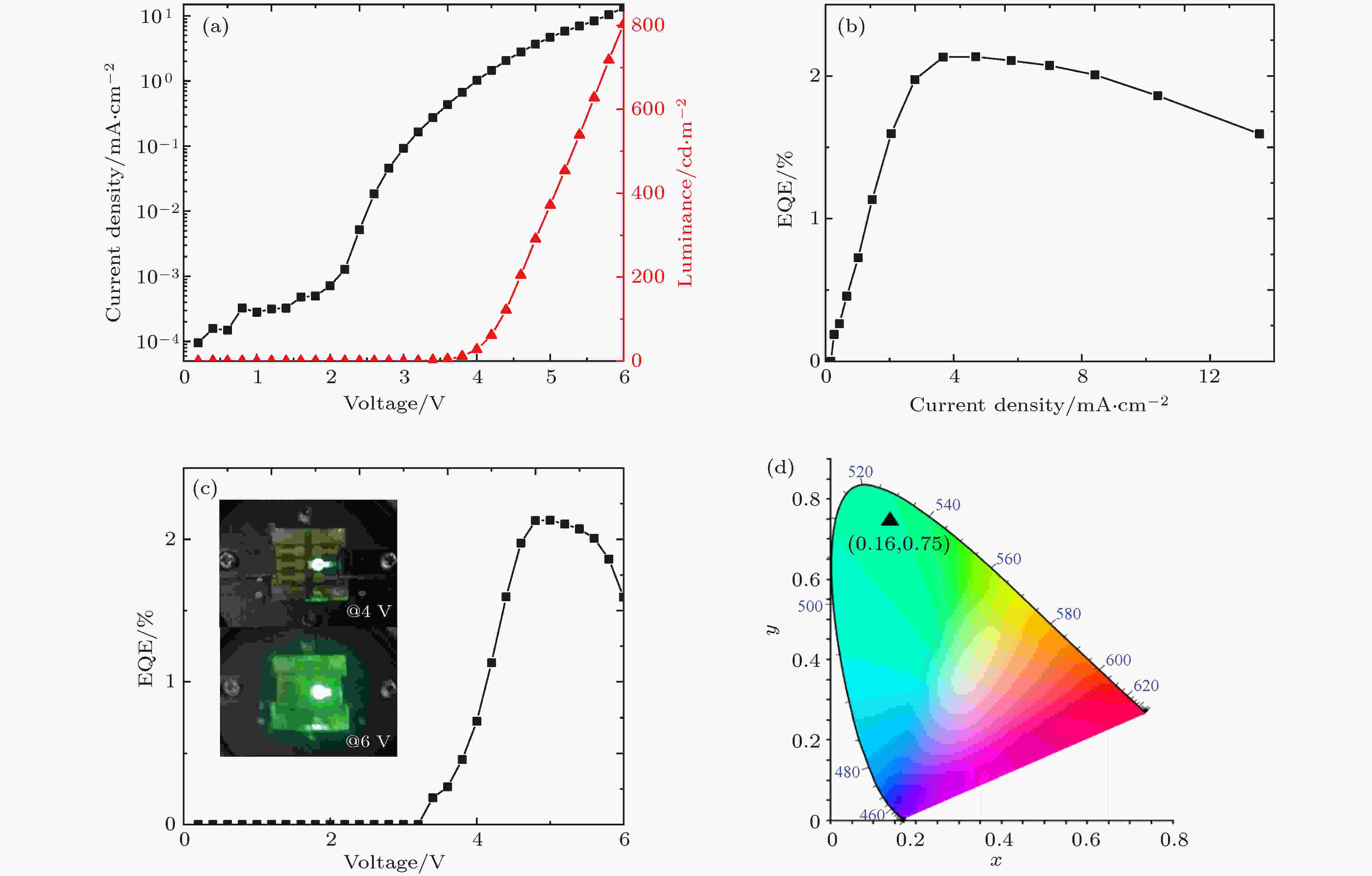
Figure6. Electroluminescence of PeLEDs: (a) Current density and luminance of the device as a function of voltage; (b) EQE of the device as a function of current density; (c) EQE of the device as a function of voltage; (d) the corresponding CIE coordinate.