

Regulatory Mechanism of Thioredoxin (Trx) in Chloroplasts
Tong Qin1,2, Zhen Huang

通讯作者:
收稿日期:2018-02-17接受日期:2018-05-23网络出版日期:2019-01-30
基金资助: |
Corresponding authors:
Received:2018-02-17Accepted:2018-05-23Online:2019-01-30

摘要
关键词:
Abstract
Keywords:
PDF (5409KB)摘要页面多维度评价相关文章导出EndNote|Ris|Bibtex收藏本文
引用本文
秦童, 黄震, 康振辉. 叶绿体硫氧还蛋白系统的调节机制. 植物学报, 2019, 54(1): 119-132 doi:10.11983/CBB18047
Qin Tong, Huang Zhen, Kang Zhenhui.
硫氧还蛋白(Thioredoxin, Trx)是一类保守的多功能酸性小蛋白, 广泛存在于植物、细菌、酵母和动物中, 分子量约为12 kDa (Meyer et al., 2009; Michelet et al., 2013; Buchanan, 2016)。Trx含有保守的二硫醇/二硫化物基序, 活性中心序列为WC(G/P)PC (Montrichard et al., 2009; Buchanan et al., 2012; Balsera et al., 2014)。该活性序列赋予Trx还原活性, 通过双分子亲核取代反应还原靶蛋白中的二硫键, 从而参与细胞的许多生化反应过程, 对维持机体氧化还原平衡具有重要作用(Lindahl and Kieselbach, 2009; K?nig et al., 2012; Couturier et al., 2013)。在还原反应完成后, 被氧化的Trx又被Trx还原酶(Trx reductase, TR)还原(Jacquot et al., 2009)。Trx的这种TR依赖性还原被称为Trx系统。
拟南芥(Arabidopsis thaliana)基因组编码20个Trx异构体。根据氨基酸序列的不同, Trx可分为7个亚家族: h、f、m、z、x、y和o型(Geigenberger and Fernie, 2014)。不同类型的Trx在不同生物以及细胞内不同细胞器中分布也不同。Trx o只在线粒体中分布, 而Trx h则广泛分布于细胞质、细胞核、内质网和线粒体(Meyer et al., 2012)。在叶绿体中主要有5种Trx, 分别是f、m、y、z和x型(Ojeda et al., 2017; Díaz et al., 2018)。因此, 叶绿体Trx系统包括Trx以及Trx类似蛋白、铁氧还蛋白(Ferredoxin, Fd)依赖的硫氧还蛋白还原酶(Fd Trx reductase, FTR)和还原型烟酰腺嘌呤二核苷磷酸(NADPH)依赖的硫氧还蛋白还原酶C (NADPH Trx reductase C, NTRC)。
巯基基团氧化还原状态的变化(二硫键-巯基)是生物体内普遍存在的现象。在很多情况下, 二硫键的打开和闭合具有调节功能(Cook and Hogg, 2013)。其化学原理是为1个过渡态二硫蛋白提供1个氢供体, 通过二硫键氧化还原状态的变化来改变目标蛋白活性(Hall et al., 2010)。在植物中, 对Trx系统作用的研究表明其主要与抗逆性相关, 如抗旱、耐热、抗氧化胁迫以及抗逆基因调控方面(孙虎等, 2010; Dietz and Pfannschmidt, 2011; Chae et al., 2013; Wang et al., 2014; Belin et al., 2015)。叶绿体作为光合作用的场所, 其Trx系统在叶绿体的代谢途径中发挥重要作用(Geigenberger and Fernie, 2014; Nikkanen et al., 2016)。FTR除了通过还原Trx激活与Calvin- Benson循环直接相关的酶类, 如果糖1, 6二磷酸酶(Fructose-1,6-bisphosphatase, FBPase) (Wang et al., 2014), 同时还参与质体中的转录过程(Gütle et al., 2017)。NTRC和一些Trx类似蛋白参与叶绿素代谢、氮同化、蛋白转运和氧化应激胁迫等(Stenbaek and Jensen, 2010; Pérez-Ruiz et al., 2014; Bolter et al., 2015)。同时, FTR和NTRC还共同参与淀粉和四吡咯的合成以及过氧化物(peroxiredoxins, Prx)的代谢反应(Kirchsteiger et al., 2009; Cejudo et al., 2012)。此外, 某些Trx类似类囊体膜蛋白还组成1条跨类囊体膜的还原势传递途径, 为类囊体腔蛋白提供电子(Karamoko et al., 2013; Kang and Wang, 2016)。本文主要对叶绿体Trx系统的调节作用进行归纳总结, 同时论述了利用叶绿体Trx系统改善植物光合效率的应用前景。
1 叶绿体Trx系统
1.1 Trx与Trx类似蛋白
在叶绿体所含5种Trx中, 只有Trx f来源于真核生物, 其它4种均属于原核起源(Rouhier et al., 2015)。Trx f、Trx m、Trx x、Trx y和Trx z分别以2、4、1、2和1种异构体的形式存在(即Trx f1-2、Trx m1-4、Trx x、Trx y1-2和Trx z) (Da et al., 2017; Yoshida and Hisabori, 2017)。Trx m在叶绿体Trx总蛋白中含量最丰富, 约占70%, 而其余分别为22.2% (Trx f)、69.1% (Trx m)、6.3% (Trx x)、1.3% (Trx y)和1.1% (Trx z); 其中Trx m1、Trx m2和Trx m4含量相近, 而Trx m3含量最少(Okegawa and Motohashi, 2015)。Trx蛋白表面电荷分布及氧化还原电位(Em)的差异决定了它们的生化特性不同(表1)。Trx f、Trx m、Trx x和Trx y都可以通过还原2-Cys Prx参与抗氧化代谢(Pulido et al., 2010); Trx f和Trx m能够还原FBPase以及柠檬酸循环中的苹果酸脱氢酶(NADP- malate dehydrogenase, NADP-MDH) (Née et al., 2009; Okegawa and Motohashi, 2015), 其中Trx f为主要还原剂, Trx m次之; Trx m3参与胞内蛋白质运输和分生组织维持(Benitez-Alfonso et al., 2009); Trx m4影响环式电子传递(Courteille et al., 2013)。Trx m1、Trx m2和Trx m4共同作用于光系统II (photosystem II, PSII)的生物发生(Wang et al., 2013)。Trx x通过作用于2-Cys Prx参与胁迫响应(Bernal-Bayard et al., 2014)。Trx y2通过调控甲硫氨酸亚砜还原酶(methionine sulfoxide reductase, MSR)的活性参与高光条件下的硫代谢(Laugier et al., 2013); Trx z通过调控质体编码的RNA聚合酶(plastid-encoded RNA polymerase, PEP)参与质体转录(Arsova et al., 2010; Chibani et al., 2011; Díaz et al., 2018)。此外, 叶绿体基质还含有CDSP32 (Cain et al., 2009; Tarrago et al., 2010)、6种非典型性半胱氨酸和组氨酸富含型(atypical Cys His-rich Trx, ACHT) (Dangoor et al., 2009, 2012; Eliyahu et al., 2015)及2种非典型性WCRKC型蛋白(Chibani et al., 2012)。它们均属于Trx类似蛋白, 在活性位点上含有非典型氧化还原活性基序。其中, CDSP32受干旱和高光诱导, 并可调节MSRB1的活性(Tarrago et al., 2010)。ACHT1蛋白在光照下处于还原态, 可还原2-Cys Prx, 但电子供体未被鉴定(Dangoor et al., 2012)。ACHT4除了可以还原2-Cys Prx外, 还直接为腺苷二磷酸葡萄糖焦磷酸化酶(ADP-glucose pyrophosphorylase, AGPase)的小亚基ASP1提供电子(Eliyahu et al., 2015)。除了可溶性Trx之外, 叶绿体含有与类囊体结合的更高分子量的Trx类似蛋白(HCF164、LOT1和SOQ1) (Motohashi and Hisabori, 2010; Karamoko et al., 2011; Brooks et al., 2013)。它们在自身的氧化还原活性位点序列上存在修饰, 控制着类囊体膜与腔蛋白的氧化还原状态。此外, 生物信息学预测表明, 还有一些假定的Trx类似蛋白定位于类囊体膜上(plastid proteome database, PPDB), 但功能未知。
Table 1
表1
表1拟南芥叶绿体Trx系统
Table 1
名称 | 登录号 | 氧化还原电位* | 功能 | 参考文献 |
---|---|---|---|---|
FTRA1 | AT5G23440 | -356 mV | 还原酶 | Balsera et al., 2013; Wang et al., 2014; Yoshida and Hisabori, 2017 |
FTRA2 | AT5G08410 | -356 mV | 还原酶 | Balsera et al., 2013; Wang et al., 2014; Yoshida and Hisabori, 2017 |
FTRB | AT2G04700 | -356 mV | 还原酶 | Balsera et al., 2013; Wang et al., 2014; Yoshida and Hisabori, 2017 |
NTRC | AT2G41680 | -330 mV | 还原酶 | Michalska et al., 2009; Chae et al., 2013; Bernal-Bayard et al., 2014; Puerto-Galán et al., 2015; Carrilloet al., 2016; Naranjo et al., 2016b; Pérez-Ruiz et al., 2017 |
Trx f1 | AT3G02730 | -321 mV (pH7.5) | 调节FBPase和ATP合酶(CF 1 γ亚基)活性, 氧化胁迫 | Hisabori et al., 2013; Yoshida et al., 2015 |
Trx f2 | AT5G16400 | -321 mV (pH7.5) | 调节FBPase和ATP合酶(CF 1 γ亚基)活性, 氧化胁迫 | Hisabori et al., 2013; Yoshida et al., 2015 |
Trx m1 | AT1G03680 | -335 mV (pH7.5) | 调节Calvin-Benson循环酶活性 | Okegawa and Motohashi, 2015 |
Trx m2 | AT4G03520 | -335 mV (pH7.5) | 调节Calvin-Benson循环酶活性 | Okegawa and Motohashi, 2015 |
Trx m3 | AT2G15570 | -316 mV (pH7.5) | 胞内蛋白运输和分生组织维持 | Benitez-Alfonso et al., 2009 |
Trx m4 | AT3G15360 | -312 mV (pH7.5) | 调节Calvin-Benson循环酶活性, 环形电子传递 | Okegawa and Motohashi, 2015 |
Trx y1 | AT1G76760 | -296 mV (pH7.5) | 胁迫响应 | Okegawa and Motohashi, 2015 |
Trx y2 | AT1G43560 | -295 mV (pH7.5) | 硫代谢 | Laugier et al., 2013 |
Trx x | AT1G50320 | -310 mV (pH7.5) | 胁迫响应 | Bernal-Bayard et al., 2014 |
Trx z | AT3G06730 | -276 mV (pH7.5) | 质体转录胁迫应激 | Arsova et al., 2010; Chibani et al., 2011; Díaz et al., 2018 |
HCF164 | At4G37200 | -224 mV ** | Cyt b6f复合体装配 | Motohashi and Hisabori, 2010 |
LTO1 | AT4G35760 | -180 mV *** | 蛋白折叠 | Wang et al., 2011; Karamoko et al., 2011, 2013 |
SOQ1 | At1G56500 | 未知 | 光抑制淬灭 | Brooks et al., 2013 |
CDSP32 | AT1G76080 | -337 mV (pH7.9) | 调节MSRB1的活性 | Tarrago et al., 2010 |
AtACHT1 (Lilium 5) | AT4G26160 | -237 mV | 抗氧化 | Dangoor et al., 2009, 2012 |
AtACHT2a (Lilium 2) | AT4G29670.1 | -239 mV | 未知 | Dangoor et al., 2009 |
AtACHT2b (Lilium 2) | AT4G29670.2 | 未知 | 未知 | Dangoor et al., 2009 |
AtACHT3 (Lilium 4) | AT2G33270 | 未知 | 未知 | Dangoor et al., 2009 |
AtACHT4a (Lilium 1) | AT1G08570.1 | -240 mV | 淀粉合成 | Dangoor et al., 2009; Eliyahu et al., 2015 |
AtACHT4b (Lilium 1) | AT1G08570.2 | 未知 | 淀粉合成 | Dangoor et al., 2009; Eliyahu et al., 2015 |
AtACHT5 (Lilium 3) | AT5G61440 | 未知 | 未知 | Dangoor et al., 2009 |
WCRKC1 | AT5G06690 | 未知 | 冷胁迫 | Chibani et al., 2011 |
WCRKC2 | AT5G04260 | 未知 | 冷胁迫 | Chibani et al., 2011 |
新窗口打开|下载CSV
Calvin-Benson循环中关键酶的光诱导活化是叶绿体Trx系统的标志性作用。Trx缺失会对酶活性产生直接影响。Trx系统可激活甘油醛-3-磷酸脱氢酶(glyce raldehyde-3-phosphate dehydrogenase, GA- PDH) (Gütle et al., 2016)、FBPase (Nikkanen et al., 2017)、景天庚酮糖-二磷酸酶(sedoheptulose- 1,7-bisphosphatase, SBPase) (Gütle et al., 2016)、磷酸核糖激酶(phosphoribulokinase, PRK) (Nikkanen et al., 2017)以及Rubisco活化酶(Yoshida et al., 2014)。淀粉合成途径中的AGPase和NADP-MDH (Michalska et al., 2009)以及氧化戊糖磷酸途径(oxidative pentose phosphate pathways, OPPP)中的关键酶6-磷酸葡萄糖脱氢酶(glucose-6-phosphate dehydrogenase, G6PDH) (Nee et al., 2009)均受Trx系统调控。
1.2 FTR与NTRC
植物含有2种类型的Trx系统, 分别由FTR和NTR扮演TR的角色。叶绿体Trx由FTR和NTRC两种TR还原, 是TR的下游电子受体。在拟南芥中, FTR是一种异二聚体铁-硫蛋白, 由1个催化亚基和1个可变亚基组成, 分别由2个单拷贝的基因编码(Balsera et al., 2013)。FTR含有Fd和Trx的结合位点。FTR具有氧化还原活性二硫键, 能够介导电子从Fd转移到Trx中的二硫键上。这种Fd依赖性系统通过Fd和FTR从PSI传递还原势至叶绿体蛋白(Wang et al., 2014)。因此, FTR是叶绿体游离Trx的主要还原剂(Yoshida and Hisabori, 2016)。拟南芥NTRC由单个核基因编码, 含有1个NADPH依赖性TR结构域, 为NTRC功能所必需, 由NADPH提供电子(Pérez-Ruiz et al., 2009)。因其C端含有1个Trx结构域, 故命名为NTRC。因此, 与FTR不同, NTRC在叶绿体中能够形成完整的NADPH依赖性Trx系统。NTRC不但为Trx提供电子, 还能够还原自身的Trx结构域, 只是NTRC的NTR结构域可以还原自身的Trx结构域, 但其还原性Trx再进一步还原其它游离的Trx蛋白时活性较低(Wulff et al., 2011)。FTR与NTRC由于蛋白结构和依赖因子不同(Fd与NADPH), 二者在行使功能时的背景不同。在光照条件下, PSI将电子传递给还原性Fd, 此时FTR才具有活性; 由于NADPH的产生并不依赖光照, 故NTRC依赖性Trx系统在光照和黑暗条件下均有活性。Trx系统在维持叶绿体整体的氧化还原平衡中发挥重要作用(Pérez-Ruiz et al., 2017), 这主要归因于FTR和NTRC对Trx和2-Cys Prx的精确调控。由于每个Trx亚型的氧化还原电位不同导致被FTR还原的程度各异。Trx f和Trx m的异构体无论在体内还是体外均可被FTR还原(Sanz-Barrio et al., 2012)。已证实Trx x和Trx y在体外可被FTR还原(Yoshida et al., 2015)。FTR不能还原Trx z, 这可能是由于Trx z具有独特的表面结构, 使其无法与FTR相互作用。NTRC虽然可以还原Trx z, 但电子的传递机制与其它Trx可能有所不同。Trx m、Trx f、Trx x和Trx y在体外都具备还原Trx z的能力, 因此Trx z活性可能依赖于这些游离的叶绿体Trx (Bohrer et al., 2012)。双分子荧光互补(BiFC)实验表明, NTRC可以与叶绿体中的几种可溶性Trx (f1、m1、m3、y1和x)在体内相互作用(Nikkanen et al., 2016), 而且NTRC过表达(Toivola et al., 2013)与敲除实验(Pulido et al., 2010)以及ntrc/trxf1双突变体表型分析(Thorm?hlen et al., 2015)均证明, NTRC具有向Trx f传递电子的能力, 这在幼苗子叶叶绿体发育过程中的作用更为重要(Ojeda et al., 2017)。此外, 如果突变发生在Trx系统调控通路中的某一关键环节, 那么叶绿体整体的氧化还原平衡将会被打破。在ntrc单突变体(Pulido et al., 2010)和trxm1/m2/m4三突变体中(Wang et al., 2013), 活性氧自由基的水平随之升高。氧化应激改变了叶绿体中的过氧化物水平(如2-Cys Prx和H2O2), 后者又进一步影响叶绿体内某些酶类的氧化还原状态(Pérez-Ruiz et al., 2017), 在这种情况下, 酶活性的降低并不能直接归因于Trx的敲除, 而是由于叶绿体中过氧化物的水平升高所致。这说明Trx系统除了调节叶绿体酶类的活性, 还通过拮抗机制调节叶绿体中的氧化还原平衡(Cheng et al., 2014)。
在叶绿体中, FTR和NTRC并非两个相互独立的途径, 而是存在部分功能交叉。FTR并非在所有光能自养生物中都存在, 在某种程度上, 其功能可被NTRC所代替(Balsera et al., 2013)。在淀粉合成的氧化戊糖磷酸途径(oxidative pentose phosphate path- way, OPPP)中, 如果FTR失活, 则可以启动NTRC旁路途径调控AGPase的活性(Kirchsteiger et al., 2012)。在拟南芥ntrc突变体中过表达TR结构域失活的NTRC基因, 其表型能够被部分恢复, 暗示FTR能够作用于NTRC中的Trx结构域, 尽管其TR结构域已失活(Toivola et al., 2013; Nikkanen et al., 2016)。对ntrc/trxf1f2/Δ2cp三突变体的研究表明, NTRC与FTR共同参与调节2-Cys Prx的氧化还原平衡(Puerto- Galán et al., 2015; Thorm?hlen et al., 2017)。这可能由于在拟南芥ntrc突变体中, 氧化态2-Cys Prxs的积累导致Trx总还原能力过度消耗, 因此其靶蛋白的氧化还原调节受损; 反之, 相应地减少2-Cys Prxs的含量, 则降低了对Trx总还原力的消耗, 从而恢复Trx对目标蛋白的氧化还原调节。
Trx系统可以响应不同的光照条件以调节FBP- ase活性和Calvin-Benson循环。拟南芥ntrc突变体生长发育延迟, 叶片呈灰绿色, 并且在短日照下表型更加明显, 暗示了NTRC在光周期调控中的重要作用(Lepist? et al., 2009)。在拟南芥和豌豆(Pisum sativum)中, Trx m和Trx f1控制碳同化的短期变化(Thorm?hlen et al., 2013)。短日照和弱光会影响trx f1突变体植株的生长(Thorm?hlen et al., 2015; Naranjo et al., 2016a)。
叶绿体Trx基因的过表达研究显示, Trx对提高植物适应性有实际应用意义。过表达Trx f的转基因烟草(Nicotiana tabacum)株系表现出生物量和淀粉含量显著增加(Sanz-Barrio et al., 2013), 但在过表达Trx m的转基因株系中却未检测到生物量和淀粉含量的显著变化(Rey et al., 2013)。在拟南芥中, 过表达NTRC基因可促进植株生长, 在正常光照条件下即可使生物量加倍, 增加叶片中的淀粉积累, 并增强转基因株系抗光氧化和干旱胁迫的能力(Toivola et al., 2013; Kim et al., 2017)。由于Trx f和NTRC都能够在本底水平上满足叶绿体中的几个主要生物合成过程的需要, 因此过量的Trx f或NTRC通常会促进叶绿体的合成代谢。有****将NTRC与Trx f过表达株系中增加的生物量归功于Trx f、Trx m和NTRC的分子伴侣功能(Sanz-Barrio et al., 2012; Chae et al., 2013)。然而, 这并不能解释Trx m的过表达为何达不到NTRC和Trx f过表达的效应。因此, 过表达Trx f和NTRC改善植物适应性的分子机制尚不十分清楚。
2 Trx系统的调控机制
在自然界中, 植物生长环境的光照情况不断变化, 包括日照时数的季节性变化以及由于云量等环境因素导致的光照强度的日变化。不同光照条件下光合作用的优化需要维持其光能吸收和热耗散之间的平衡, 该过程在很大程度上通过叶绿体中氧化还原调控实现。由于FTR是在光照条件下从Fd接收电子, 然后通过还原Trx向Trx传递电子, 在叶绿体中, Trx是很多酶类的电子供体(Serrato et al., 2013), 因此Trx成为光照和酶活之间的关系纽带。2.1 Trx系统调控网络
Trx系统在叶绿体光合作用和胁迫应激调控中表现出多点交叉及调控循环网络的特征(图1)。在叶绿体的生物发生过程中, Trx能够激活叶绿素合成途径中镁原卟啉IX甲基转移酶和镁螯合酶活性(Richter et al., 2013, 2015; Richter and Grimm, 2013)。此外, ATP合酶γ亚基中具有氧化还原活性的Cys残基在光照下被迅速还原, 在黑暗中则被氧化, Trx系统正是发挥了叶绿体ATP合成中的开关作用(Carrillo et al., 2016)。此外, FTR与NTRC同时作为ATP合酶的关键调节子, 在低光照下还原γ亚基, 且二者功能并不冗余(Luo et al., 2012; Hisabori et al., 2013)。质子梯度调控蛋白PGR5和质子梯度调控类似蛋白PGRL1依赖的环式电子传递也受Trx调节(Hertle et al., 2013; Strand et al., 2016)。在高光照下, NTRC控制非光化学淬灭和光合电子传递, 暗示了NTRC在光胁迫条件下的作用(Naranjo et al., 2016b)。图1
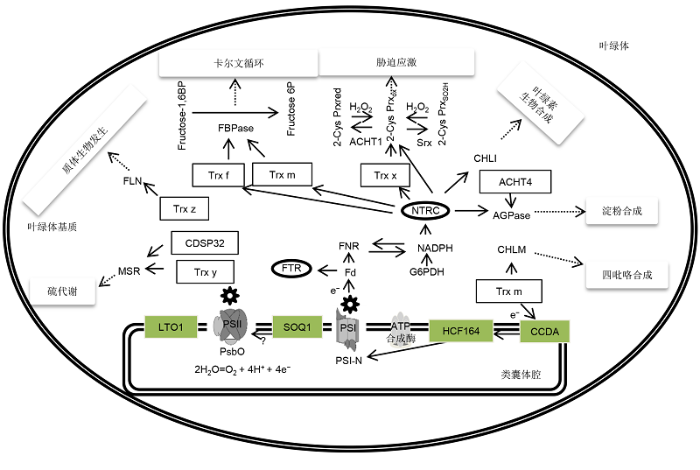
图1叶绿体Trx系统及其作用
叶绿体Trx系统在卡尔文循环中关键酶类、质体生物发生、硫代谢、胁迫应激及叶绿素、四吡咯和淀粉合成中发挥重要调节作用。FTR对5种Trx蛋白都有作用, 故图中未标出。SOQ1对PSII的亚基作用靶点未知。
Figure 1Chloroplast Trx systems and their functions
The Trx systems in chloroplast play important roles in regulating key enzymes of Calvin cycle, plastid biogenesis, sulfur metabolism, stress response, and the synthesis of chlorophyll, tetrapyrrole, and starch. Since FTR affects all 5 Trx proteins, the lines are not marked in the figure. The target of SOQ1 on the PSII subunits is still unknown.
由于不同Trx以异构体的形式存在, 因此需构建多重突变体来研究它们之间的相互作用, 如trx f1/f2双突变体和trx m1/m2/m4三突变体。这些研究进一步丰富了Trx系统在光系统功能及光合作用相关酶类的活性调节中的作用。拟南芥trx m1和trx m2单突变体以及trx m1/m2双突变体在正常光照下并无表型差异, 只有在Trx m4基因进一步突变或沉默后才表现出生长迟缓的表型, trx m1/m2/m4三突变体光合能力下降50% (Wang et al., 2013)。双分子荧光互补实验表明, NTRC与PRK和FBPase存在相互作用, 而且这2种酶在过表达NTRC基因的拟南芥叶片中表现出更高的活化水平(Nikkanen et al., 2016)。在ntrc/trxf1双突变体中, FBPase光依赖的氧化还原活性比ntrc或trx f1单突变体中的活性更低(Thorm?hlen et al., 2015)。以上结果表明, NTRC除了直接调节Calvon- Benson循环中的酶活性, 还间接活化Trx f, 而Trx f又直接控制FBPase和PRK的活性。
2.2 Trx m是类囊体腔蛋白的原始电子供体
植物类囊体腔是光合电子传递和跨膜质子梯度形成的场所(Simionato et al., 2015)。但截止目前还没有证据表明类囊体腔含有可溶性Trx。叶绿体基质Trx m作为高叶绿素荧光蛋白HCF164还原力的供体, 向HCF164位于类囊体腔侧的催化结构域传递电子(Motohashi and Hisabori, 2010)。然而, 超过40%的类囊体腔蛋白受氧化还原信号调节。由此看来, 氧化还原调控许多类囊体腔蛋白的活性与功能, 使它们在光系统装配和光合电子传递中发挥不可或缺的作用(J?rvi et al., 2013; Rouhier et al., 2015)。Trx系统对类囊体腔蛋白的氧化还原调控主要体现在植物PSII的装配以及对不同光强的适应。例如, PSII的外周蛋白PsbO的2个亚基(PsbO1和PsbO2)通过分子间二硫键与PSII反应中心蛋白共价连接。在PSII装配过程中, PsbO必须及时与PSII反应中心形成分子间二硫键, 如果长时间处于还原态, 则容易被蛋白酶降解(Lindahl and Kieselbach, 2009)。Trx系统可以还原性激活某些蛋白酶, 如DEG1 (张艳玲等, 2009); PsbO蛋白中二硫键的还原导致构象发生变化, 从而被DEG1识别(Nikkanen and Rintam?ki, 2014)。叶黄素循环途径中玉米黄质脱环氧化酶(violaxanthin de-epoxidase, VDE)的活性受二硫键状态的影响(Goss and Lepetit, 2015)。VDE的1个Cys残基(C362)靠近酯钙蛋白结构域假定的底物结合位点, 受Trx的影响, DTT也可以抑制VDE的活性(Fufezan et al., 2012)。这说明类囊体腔中的氧化还原控制反应对不同光强下植物的适应性调节至关重要。类囊体腔可能通过Trx氧化还原调控方式, 在短时间内控制VDE的活化水平。由于二硫键还原会使VDE失活, 因而重新形成二硫键后会再次被激活。
2.3 HCF164和SOQ1参与类囊体腔的还原势传递
植物类囊体腔起源于细菌周质。在叶绿体基质和类囊体腔之间, 人们发现了类似细菌周质的巯基代谢通路(Kang and Wang, 2016)。细菌通过Dsb蛋白系统将蛋白分泌到周质。所转入蛋白的巯基基团先被可溶性蛋白DsbA氧化, 而还原型DsbA又被膜蛋白DsbB氧化, 把电子转移给醌。DsbC和DsbG维持蛋白的正确构象, DsbD位于细胞质膜上, 作为一个巯基-二硫键转运子从胞质向周质传递还原势, 将DsbC和DsbG及胞质Trx系统有机结合起来(Lindahl et al., 2011)。然而, 在类囊体腔中并没有发现可溶性Trx。越来越多的研究表明, 还原势是从叶绿体基质转入到类囊体腔。高等植物叶绿体具有一套跨类囊体膜从基质侧到类囊体腔侧的还原势传递系统。相比细菌跨质膜DsbA-C/G-D氧化还原通路参与跨膜蛋白转运, 类囊体腔的这套系统则由LTO1、HCF164/SOQ1和CCDA组成, 参与跨膜还原势的传递。HCF164的拓扑学结构特征、氧化还原活性及电子势表明, 其具有从叶绿体基质向类囊体腔传递还原电子的能力(Motohashi and Hisabori, 2010): (1) HCF164是1个类囊体膜Trx类似蛋白, 其含有二硫簇基团的亲水羧基末端朝向类囊体腔; (2) 重组HCF164蛋白在体外显示二硫键氧还酶活性; (3) Trx m的电子势为-320 mV, 可溶性HCF164蛋白的氧化还原电子势为-224 mV (羧基末端的Trx结构域)。实验证据表明, PSI-N是HCF164的靶蛋白之一。在生物学功能方面, HCF164参与Cyt b6f复合体的成熟或装配, 其分子机制可能是HCF164的活性位点与Cyt b6f的2个亚基Cyt f和Rieske Fe-S蛋白形成分子间二硫键。高叶绿素荧光突变体hcf164的Cyt b6f复合体亚基不能正常装配, 因此表现出光合电子传递缺陷。
HCF164参与PSI和Cyt b6f复合体的组装, 而淬灭抑制子1 (SOQ1)与PSII功能相关(Brooks et al., 2013)。SOQ1的Trx类似/β-螺旋结构域位于类囊体腔, 对其维持光吸收效率是必需的。soq1突变体在PsbS缺失的情况下(npq4)具备高NPQ, 其依赖光密度且动力学恢复缓慢。SOQ1阻止PSII天线淬灭的缓慢可逆形式的形成。与HCF164功能类似, SOQ1确保在类囊体腔氧化的环境中使目标蛋白处于还原态, 由此直接或间接阻止天线蛋白激发能qI类型的淬灭(Onoa et al., 2014)。
2.4 由CCDA介导的跨膜还原势传递
拟南芥ccda突变体表现出与hcf164突变体类似的表型, 原因是它们都不能合成Cyt b6f复合体。蛋白序列分析显示, 植物CCDA与一个原核生物中巯基二硫键转运子蛋白同源。CCDA属于类囊体膜蛋白, 含有6个跨膜区域, 可被Trx m还原, 作为介导因子参与还原势从基质到类囊体腔的传递(Motohashi and Hisabori, 2010)。在拟南芥中, CCDA被定义为Cyt b6f装配不可缺少的因子, HCF164很可能是CCDA的下游因子。然而, 目前尚无实验证据表明HCF164与CCDA之间存在直接相互作用。HCF164之所以被Trx m特异性地还原, 可能由CCDA决定, CCDA先于HCF164与基质Trx m反应。但是目前还不能解释的是, CCDA只含有1个二硫键, 且2个Cys残基都嵌入类囊体膜内, 但其朝向可能是面对基质侧。由此可见, 类囊体膜上还可能存在其它因子参与还原势的传递, 并且该因子应该是1个类囊体膜锚定、Trx类似结构域朝向基质的蛋白。2.5 LTO1参与类囊体腔中二硫键的生成
LTO1 (Lumen Thiol Oxidoreductase 1)是1个类囊体膜蛋白, 由2个结构域组成。N端结构域是1个与哺乳动物维生素K还原酶同源的VKOR结构域, 与细菌DsbB功能类似; 另一个是Trx类似结构域, 具有与细菌巯基氧化还原酶蛋白DsbA同源的Trx类似序列(Dutton et al., 2010; Wang et al., 2011; Karamoko et al., 2011, 2013)。Trx类似结构域可向VKOR类似结构域传递电子(Feng et al., 2011)。LTO1与PSII亚基PsbO互作。在体外, LTO1的Trx类似结构域能够向PsbO中导入1个二硫键。PsbO巯基的氧化还原状态对其稳定性和PSII的装配起决定性作用。由此可见, lto1突变体中PSII的累积受损是由于PsbO与PSII之间的二硫键未能及时形成引起。但LTO1是否可以协助PsbO转入类囊体腔还需实验验证。目前, 普遍认为叶绿醌可能是LTO1依赖的二硫键形成通路的电子受体(Furt et al., 2010), 其原因是VKOR还原维生素K时以叶绿醌作为辅因子。LTO1可以在体外促进AtFKBP13二硫键的形成, 表明LTO1是保证类囊体腔蛋白活性的根源(Lu et al., 2013)。
2.6 Trx系统维持光合效率
当入射光强超过一定限度时, 光合作用会达到一个饱和点。超过饱和点的入射光强在光合膜上引起多余的能量吸收而使叶绿素分子产生过多的激发态, 如不能通过转化成化学能及时将能量传递出去, 则会传递给周围的氧气, 产生活性氧(ROS)自由基, 而ROS会氧化光合膜, 造成光合膜被破坏, 反而抑制光合作用(Goss and Lepetit, 2015)。为了保护光合膜免遭光破坏, 产生光抑制, 在长期的进化过程中, 植物形成了一系列的光淬灭和光保护机制。依赖于叶黄素循环的热耗散被认为是植物光合机构免受过剩光能伤害的主要机制(Arnoux et al., 2009)。类囊体腔VDE催化紫黄质脱环氧生成单环氧玉米黄质, 然后继续脱环氧生成玉米黄质。玉米黄质的生成与VDE活性有关, 受类囊体腔pH值的严格控制(Fufezan et al., 2012)。当类囊体腔pH值小于6.2且由抗坏血酸作为电子受体时, VDE可被激活。VDE含有酯钙蛋白结构域, 富含Cys和Glu结构域, 后者为其功能所必需。二硫键的还原导致其功能丧失, 并且在低温条件下热稳定性降低(Hallin et al., 2015)。VDE含有13个Cys, 12个Cys都比较保守, VED的活性依赖于它们的氧化状态, 因为只有当形成的6个二硫键完全处于氧化状态时才具有活性, 可被特异性抑制剂DTT抑制(Simionato et al., 2015)。
叶绿体通过维持氧化还原平衡来优化PSI与PSII之间的能量分配。激酶STN7参与催化光捕获复合体LHCII的可逆磷酸化, 是控制低光照下PSI与PSII之间能量分配的重要机制。STN7激酶是1个跨类囊体膜蛋白, 催化位点位于叶绿体基质侧, 氧化还原活性位点在类囊体腔(Betterle et al., 2015)。在STN7激酶缺乏的情况下, 光密度波动导致LHCII效率的波动, 主要影响PSII的激发, 导致一系列电子传递体氧化还原失衡(PSI除外), 使stn7突变体信号转导发生混乱并最终延迟生长(Tikkanen et al., 2010)。STN7使还原态PQ结合到Cyt b6f的Qo位点(Betterle et al., 2015)。STN7形成亚基之中/之间二硫键, 这些二硫键的还原可能诱导了STN7的单体化, 并破坏其与Cyt b6f的链接, 进而使其丧失功能。
LTO1可以催化PsbO二硫键的形成, 把类囊体腔蛋白转运与PSII装配紧密联系起来。刚转运至类囊体膜的PsbO, 由于处于过渡态很容易被蛋白酶解(Kieselbach, 2013)。成熟态PsbO由于具有二硫键相对比较稳定, 但二硫键被还原后其会变得不稳定, 易于被蛋白酶水解。新转运的PsbO蛋白的二硫键处于还原态, 因此为了保证PsbO稳定折叠并能结合在PSII上, 二硫键的形成和蛋白的迅速折叠很有必要。由于腔内的氧化环境, 二硫键的形成可能是缓慢自发的, 所以需要酶辅助的催化反应最大限度地减少新转入PsbO的丢失。由此看来, LTO1对PsbO二硫键的形成和PSII的装配非常重要。
3 Trx系统靶蛋白的研究方法
Trx的靶点蛋白在整个叶绿体的亚细胞器结构中都有分布(Hall et al., 2010)。由Trx调节的潜在靶蛋白覆盖了叶绿体中几乎所有必需的过程, 包括质体的生物发生、基因表达、光合作用、抗氧化和应激反应以及生物合成代谢(Belin et al., 2015; Yoshida et al., 2015)。Trx位点蛋白的鉴定主要通过检测蛋白二硫键实现, 相关技术方法可分为2种。Trx一般含有WCG- PC-基序, 该基序N末端Cys残基与目标蛋白形成二硫键中间产物, 后续可被第2个Cys残基亲核攻击打破, 该反应的产物是1个氧化态的Trx和1个还原态的目标蛋白。而暴露在目标蛋白中新产生的二硫簇易被一些二硫簇试剂(如荧光染料溴二胺(mono bromobimane, mBBr)和碘乙酰胺(iodoacetamide, IAM))标记, 形成的复合物可用仪器进行检测。第2种方法是利用突变Trx亲和色谱和质谱技术鉴定Trx靶蛋白。该技术利用Trx还原特殊二硫键的特性, 在靶位二硫键被完全还原之前, Trx和靶蛋白之间形成1个瞬时异二硫键, 当2个Cys中的1个被突变后, 可使正常的瞬时异二硫键稳定, 与Trx共价结合的目标蛋白可以被二硫苏糖醇(dithiothreitol, DTT)洗脱。4 研究展望
Trx利用氧化还原机制调控靶蛋白二硫键的状态和蛋白活性。每个Trx都有各自的Em, 虽是氧化还原反应的基础, 但这并不是Trx选择其靶蛋白的决定因素。目前已知1个Trx可以有多个靶蛋白。例如, Trx m可以还原SEPase和CHLM等而参与不同的叶绿体合成途径; 同时1个靶蛋白又可能由几个Trx共同作用, 而且每个Trx对它的作用效率不同。这也可能与Trx和靶蛋白中关键氨基酸残基的带电性质及其导致的空间构象不同有一定关系。表面静电势在氧化还原调节中的作用还需要进一步研究。因此, 对Trx及其靶蛋白共结晶结构的测定将直接揭示表面电荷相互作用所需的关键氨基酸残基。NTRC是一种光能自养生物质体中特有的NTR。在细菌和动物中还广泛存在另外2种NTR, 一种在细菌和酵母中, 由分子量为35 kDa的同型二聚体组成; 另一种在哺乳动物中, 也是由同型二聚体组成, 分子量为55 kDa, 而且在C端倒数第2个残基中含有1个稀有氨基酸硒代半胱氨酸(Sec)。哺乳动物TR是吡啶核苷酸二硫化物氧化还原酶, 其采用Sec代替氧化还原活性四肽Gly-Cys-Sec-Gly基序中更常用的半胱氨酸(Cys)来催化巯基/二硫化物交换反应。在TR的催化过程中, Sec作为电子受体, 比Cys具有更好的亲核亲电性质, 因此更具有化学反应性。通过定点突变方法将NTRC活性基序中的Cys突变成Sec, 研究其生化特性以及在植物体内的生理功能, 将会更加丰富NTRC的研究意义。
叶绿体Trx的活性升高能够促进植物的营养生长和有机物的积累(如过表达NTRC可增强植株光能利用率; 过表达Trx f可提高叶片中淀粉和可溶性糖的含量), 因此可以通过调控Trx系统改善植株光合活性, 加速有机物的合成, 最终激发植物生产生物燃料的潜能, 或生产其它有价值的糖基化合物。此外, 叶绿体大约含有100个Trx靶点, 分别参与多种重要的生命进程。因此, Trx系统为经济作物的分子育种提供了有价值的靶点。然而, NTRC和Trx f的活性增加能否促进植物生长, 以及这种修饰是否在压力条件下(包括波动的光)影响生长还需要进一步评估。虽然Trx m、Trx y、Trx x和CDP32已被证明在胁迫条件下调控叶绿体蛋白, 但这些Trx的活性升高对提高植物抗逆性的机制仍有待阐明。
参考文献 原文顺序
文献年度倒序
文中引用次数倒序
被引期刊影响因子
DOI:10.3969/gab.029.000748URL [本文引用: 1]

硫氧还蛋白是一类催化二硫键氧化还原的小蛋白,它通过调控细胞中氧化还原状态发挥重要的作用。在植物中,硫氧还蛋白系统尤为复杂,参与了植物的新陈代谢、转录翻译调控、信号传导以及植物的抗逆反应等。本文主要通过对植物硫氧还蛋白分类、活性位点、结构以及3种硫氧还蛋白系统研究现状进行概述,并对植物的硫氧还蛋白及系统进行了展望,从而较为全面地综述了植物的硫氧还蛋白系统,为进一步了解硫氧还蛋白在植物体内的作用机制奠定基础,也为今后的相关研究提供参考。
DOI:10.3969/j.issn.1674-3466.2009.01.004URL [本文引用: 1]

DEGP家族蛋白酶广泛分布于原核生物和真核生物细胞中.在拟南 芥中有16个DEGP类似的蛋白酶,根据蛋白质组学数据,其中有4个定位于叶绿体中,分别命名为DEG1、DEG2、DEG5和DEG8.结合生物化学和 分子生物学等研究手段对拟南芥叶绿体中的DEGP蛋白酶进行了分析,现有的研究初步证明了这些蛋白酶参与光系统Ⅱ(PSⅡ)复合物反应中心D1蛋白的降 解,从而在PSⅡ复合物的修复循环和功能维护中起重要作用.该文概述了拟南芥叶绿体中DEG蛋白酶的结构和功能的最新研究进展.
.
[本文引用: 1]
.
DOI:10.1105/tpc.109.071001URLPMID:20511297 [本文引用: 1]

Here, we characterize a plastidial thioredoxin (TRX) isoform from Arabidopsis thaliana that defines a previously unknown branch of plastidial TRXs lying between x- and y-type TRXs and thus was named TRX z. An Arabidopsis knockout mutant of TRX z had a severe albino phenotype and was inhibited in chloroplast development. Quantitative real-time RT-PCR analysis of the mutant suggested that the expressions of genes that depend on a plastid-encoded RNA polymerase (PEP) were specifically decreased. Similar results were obtained upon virus-induced gene silencing (VIGS) of the TRX z ortholog in Nicotiana benthamiana. We found that two fructokinase-like proteins (FLN1 and FLN2), members of the pfkB-carbohydrate kinase family, were potential TRX z target proteins and identified conserved Cys residues mediating the FLN RX z interaction. VIGS in N. benthamiana and inducible RNA interference in Arabidopsis of FLNs also led to a repression of PEP-dependent gene transcription. Remarkably, recombinant FLNs displayed no detectable sugar-phosphorylating activity, and amino acid substitutions within the predicted active site imply that the FLNs have acquired a new function, which might be regulatory rather than metabolic. We were able to show that the FLN2 redox state changes in vivo during light/dark transitions and that this change is mediated by TRX z. Taken together, our data strongly suggest an important role for TRX z and both FLNs in the regulation of PEP-dependent transcription in chloroplasts.
DOI:10.1089/ars.2013.5817URLPMID:24483204 [本文引用: 1]

The post-translational modification of thiol groups stands out as a key strategy that cells employ for metabolic regulation and adaptation to changing environmental conditions. Nowhere is this more evident than in chloroplasts-the O2-evolving photosynthetic organelles of plant cells that are fitted with multiple redox systems, including the thioredoxin (Trx) family of oxidoreductases functional in the reversible modification of regulatory thiols of proteins in all types of cells. The best understood member of this family in chloroplasts is the ferredoxin-linked thioredoxin system (FTS) by which proteins are modified via light-dependent disulfide/dithiol (S-S/2SH) transitions.Discovered in the reductive activation of enzymes of the Calvin-Benson cycle in illuminated chloroplast preparations, recent studies have extended the role of the FTS far beyond its original boundaries to include a spectrum of cellular processes. Together with the NADP-linked thioredoxin reductase C-type (NTRC) and glutathione/glutaredoxin systems, the FTS also plays a central role in the response of chloroplasts to different types of stress.The comparisons of redox regulatory networks functional in chloroplasts of land plants with those of cyanobacteria-prokaryotes considered to be the ancestors of chloroplasts-and different types of algae summarized in this review have provided new insight into the evolutionary development of redox regulation, starting with the simplest O2-evolving organisms.The evolutionary appearance, mode of action, and specificity of the redox regulatory systems functional in chloroplasts, as well as the types of redox modification operating under diverse environmental conditions stand out as areas for future study.
DOI:10.1007/s00425-012-1803-yURLPMID:23223880 [本文引用: 2]

AbstractUncovered in studies on photosynthesis 3502years ago, redox regulation has been extended to all types of living cells. We understand a great deal about the occurrence, function, and mechanism of action of this mode of regulation, but we know little about its origin and its evolution. To help fill this gap, we have taken advantage of available genome sequences that make it possible to trace the phylogenetic roots of members of the system that was originally described for chloroplasts—ferredoxin, ferredoxin:thioredoxin reductase (FTR), and thioredoxin as well as target enzymes. The results suggest that: (1) the catalytic subunit, FTRc, originated in deeply rooted microaerophilic, chemoautotrophic bacteria where it appears to function in regulating CO fixation by the reverse citric acid cycle; (2) FTRc was incorporated into oxygenic photosynthetic organisms without significant structural change except for addition of a variable subunit (FTRv) seemingly to protect the Fe–S cluster against oxygen; (3) new Trxs and target enzymes were systematically added as evolution proceeded from bacteria through the different types of oxygenic photosynthetic organisms; (4) an oxygenic type of regulation preceded classical light–dark regulation in the regulation of enzymes of CO fixation by the Calvin–Benson cycle; (5) FTR is not universally present in oxygenic photosynthetic organisms, and in certain early representatives is seemingly functionally replaced by NADP-thioredoxin reductase; and (6) FTRc underwent structural diversification to meet the ecological needs of a variety of bacteria and archaea.
.
DOI:10.1111/pce.12276URLPMID:24428628 [本文引用: 2]

Abstract Thiol reduction proteins are key regulators of the redox state of the cell, managing development and stress response programs. In plants, thiol reduction proteins, namely thioredoxin (TRX), glutaredoxin (GRX), and their respective reducers glutathione reductase (GR) and thioredoxin reductase (TR), are organized in complex multigene families. In order to decipher the function of the different proteins, it is necessary to have a clear picture of their respective expression profiles. By collecting information from gene expression databases, we have performed a comprehensive in silico study of the expression of all members of different classes of thiol reduction genes (TRX, GRX) in A rabidopsis thaliana . Tissue expression profiles and response to many biotic and abiotic stress conditions have been studied systematically. Altogether, the significance of our data is discussed with respect to published biochemical and genetic studies.
DOI:10.1073/pnas.0808717106URLPMID:19218459 [本文引用: 1]

Cell-to-cell transport in plants occurs through cytoplasmic channels called "plasmodesmata" and is regulated by developmental and environmental factors. Callose deposition modulates plasmodesmal transport in vivo, but little is known about the mechanisms that regulate this process. Here we report a genetic approach to identify mutants affecting plasmodesmal transport. We isolated 5 mutants, named gfp arrested trafficking (gat), affected in GFP unloading from the phloem into the meristem. gat1 mutants were seedling lethal and carried lesions in an m-type thioredoxin that is expressed in non-green plastids of meristems and organ primordia. Callose and hydrogen peroxide accumulated in gat1 mutants, and WT plants subjected to oxidative conditions phenocopied the gat1 trafficking defects. Ectopic expression of GAT1 in mature leaves increased plasmodesmal permeability and led to a delay in senescence and flowering time. We propose a role for the GAT1 thioredoxin in the redox regulation of callose deposition and symplastic permeability that is essential for meristem maintenance in Arabidopsis.
DOI:10.1016/j.febslet.2014.09.044URLPMID:25448674 [本文引用: 1]

In addition to the standard NADPH thioredoxin reductases (NTRs), plants hold a plastidic NTR (NTRC), with a thioredoxin module fused at the C-terminus. NTRC is an efficient reductant of 2-Cys peroxiredoxins (2-Cys Prxs). The interaction of NTRC and chloroplastic thioredoxin x with 2-Cys Prxs has been confirmed in vivo, by bimolecular fluorescence complementation (BiFC) assays, and in vitro, by isothermal titration calorimetry (ITC) experiments. In comparison with thioredoxin x , NTRC interacts with 2-Cys Prx with higher affinity, both the thioredoxin and NTR domains of NTRC contributing significantly to this interaction, as demonstrated by using the NTR and thioredoxin modules of the enzyme expressed separately. The presence of the thioredoxin domain seems to prevent the interaction of NTRC with thioredoxin x . .overlined { text-decoration: overline; } .struck { text-decoration:line-through; } .underlined { text-decoration:underline; } .doubleUnderlined { text-decoration:underline;border-bottom:1px solid #000; }
DOI:10.1104/pp.114.252379URLPMID:25501945 [本文引用: 2]

Phosphorylation of the photosystem II antenna protein CP29 has been reported to be induced by excess light and further enhanced by low temperature, increasing resistance to these stressing factors. Moreover, high light-induced CP29 phosphorylation was specifically found in monocots, both C3 and C4, which include the large majority of food crops. Recently, knockout collections have become available in rice (Oryza sativa), a model organism for monocots. In this work, we have used reverse genetics coupled to biochemical and physiological analysis to elucidate the molecular basis of high light-induced phosphorylation of CP29 and the mechanisms by which it exerts a photoprotective effect. We found that kinases and phosphatases involved in CP29 phosphorylation are distinct from those reported to act in State 1-State 2 transitions. In addition, we elucidated the photoprotective role of CP29 phosphorylation in reducing singlet oxygen production and enhancing excess energy dissipation. We thus established, in monocots, a mechanistic connection between phosphorylation of CP29 and nonphotochemical quenching, two processes so far considered independent from one another.
DOI:10.1093/jxb/ers283URLPMID:23096001 [本文引用: 1]

In plants, thioredoxins (TRX) constitute a large protein disulphide oxidoreductase family comprising 10 plastidial members in Arabidopsis thaliana and subdivided in five types. The f- and m-types regulate enzymes involved mainly in carbon metabolism whereas the x, y, and z types have an antioxidant function. The reduction of TRXm and f in chloroplasts is performed in the light by ferredoxin:thioredoxin reductase (FTR) that uses photosynthetically reduced ferredoxin (Fd) as a reductant. The reduction system of Arabidopsis TRXx, y, and z has never been demonstrated. Recently, a gene encoding an atypical plastidial NADPH-dependent TRX reductase (NTRC) was found. In the present study, gene expression analysis revealed that both reductases are expressed in all organs of Arabidopsis and could potentially serve as electron donors to plastidial TRX. This ability was tested in vitro either with purified NTRC in presence of NADPH or with a light-driven reconstituted system comprising thylakoids and purified Fd and FTR. The results demonstrate that FTR reduces the x and y TRX isoforms but not the recently identified TRXz. Moreover, the results show that NTRC cannot be an efficient alternative reducing system, neither for TRXz nor for the other plastidial TRX. The data reveal that TRXf, m, x, and y, known as redox regulators in the chloroplast, have also the ability to reduce TRXz in vitro. Overall, the present study points out the unique properties of TRXz among plastidial TRX.
[本文引用: 1]
.
[本文引用: 2]
DOI:10.1146/annurev-arplant-043015-111949URLPMID:27128465 [本文引用: 1]

Abstract After a brief discussion of my graduate work at Duke University, I describe a series of investigations on redox proteins at the University of California, Berkeley. Starting with ferredoxin from fermentative bacteria, the Berkeley research fostered experiments that uncovered a pathway for fixing CO2 in bacterial photosynthesis. The carbon work, in turn, opened new vistas, including the discovery that thioredoxin functions universally in regulating the Calvin-Benson cycle in oxygenic photosynthesis. These experiments, which took place over a 50-year period, led to the formulation of a set of biological principles and set the stage for research demonstrating a role for redox in the regulation of previously unrecognized processes extending far beyond photosynthesis.
DOI:10.1016/j.bbagen.2012.07.006URLPMID:22863419 [本文引用: 1]

78 Commemoration of the 50th birthday of protein thioredoxin. 78 Development of field traced from discovery in 1964 to 2011. 78 Function expands from hydrogen carrier to regulatory protein acting throughout biology. 78 Stockholm, Berkeley and Saint Feliu de Guixols conferences serve as benchmarks.
DOI:10.1007/s11103-009-9471-4URLPMID:19259774 [本文引用: 1]

Thioredoxins play key regulatory roles in chloroplasts by linking photosynthetic light reactions to a series of plastid functions. In addition to the established groups of thioredoxins, f, m, x, and y, novel plant thioredoxins were also considered to include WCRKC motif proteins, CDSP32, the APR proteins, the lilium proteins and HCF164. Despite their important roles, the subcellular locations of many novel thioredoxins has remained unknown. Here, we report a study of their subcellular location using the cDNA clone resources of TAIR. In addition to filling all gaps in the subcellular map of the established chloroplast thioredoxins f, m, x and y, we show that the members of the WCRKC family are targeted to the stroma and provide evidence for a stromal location of the lilium proteins. The combined data from this and related studies indicate a consistent stromal location of the known Arabidopsis chloroplast thioredoxins except for thylakoid-bound HCF164.
DOI:10.1111/tpj.13226URLPMID:27233821 [本文引用: 1]

Summary The chloroplast ATP synthase is known to be regulated by redox modulation of a disulfide bridge on the γ-subunit through the ferredoxin–thioredoxin regulatory system. We show that a second enzyme, the recently identified chloroplast NADPH thioredoxin reductase C (NTRC), plays a role specifically at low irradiance. Arabidopsis mutants lacking NTRC ( ntrc ) displayed a striking photosynthetic phenotype in which feedback regulation of the light reactions was strongly activated at low light, but returned to wild-type levels as irradiance was increased. This effect was caused by an altered redox state of the γ-subunit under low, but not high, light. The low light-specific decrease in ATP synthase activity in ntrc resulted in a buildup of the thylakoid proton motive force with subsequent activation of non-photochemical quenching and downregulation of linear electron flow. We conclude that NTRC provides redox modulation at low light using the relatively oxidizing substrate NADPH, whereas the canonical ferredoxin–thioredoxin system can take over at higher light, when reduced ferredoxin can accumulate. Based on these results, we reassess previous models for ATP synthase regulation and propose that NTRC is most likely regulated by light. We also find that ntrc is highly sensitive to rapidly changing light intensities that probably do not involve the chloroplast ATP synthase, implicating this system in multiple photosynthetic processes, particularly under fluctuating environmental conditions.
DOI:10.1016/j.febslet.2012.07.003URLPMID:22796111 [本文引用: 1]

Protein disulphide ithiol interchange is a universal mechanism of redox regulation in which thioredoxins (Trxs) play an essential role. In heterotrophic organisms, and non-photosynthetic plant organs, NADPH provides the required reducing power in a reaction catalysed by NADPH-dependent thioredoxin reductase (NTR). It has been considered that chloroplasts constitute an exception because reducing equivalents for redox regulation in this organelle is provided by ferredoxin (Fd) reduced by the photosynthetic electron transport chain, not by NADPH. This view was modified by the discovery of a chloroplast-localised NTR, denoted NTRC, a bimodular enzyme formed by NTR and Trx domains with high affinity for NADPH. In this review, we will summarize the present knowledge of the biochemical properties of NTRC and discuss the implications of this enzyme on plastid redox regulation in plants.
DOI:10.1093/mp/sss105URLPMID:23024205 [本文引用: 2]

Genevestigator analysis has indicated heat shock induction of transcripts for NADPH-thioredoxin reductase, type C (NTRC) in the light. Here we show overexpression of NTRC in Arabidopsis (NTRCE) resulting in enhanced tolerance to heat shock, whereas NTRC knockout mutant plants (ntrc1) exhibit a temperature sensitive phenotype. To investigate the underlying mechanism of this phenotype, we analyzed the proteins biochemical properties and protein structure. NTRC assembles into homopolymeric structures of varying complexity with functions as a disulfide reductase, a foldase chaperone, and as a holdase chaperone. The multiple functions of NTRC are closely correlated with protein structure. Complexes of higher molecular weight (HMW) showed stronger activity as a holdase chaperone, while low molecular weight (LMW) species exhibited weaker holdase chaperone activity but stronger disulfide reductase and foldase chaperone activities. Heat shock converted LMW proteins into HMW complexes. Mutations of the two active site Cys residues of NTRC into Ser (C217/454S-NTRC) led to a complete inactivation of its disulfide reductase and foldase chaperone functions, but conferred only a slight decrease in its holdase chaperone function. The overexpression of the mutated C217/454S-NTRC provided Arabidopsis with a similar degree of thermotolerance compared with that of NTRCE plants. However, after prolonged incubation under heat shock, NTRCE plants tolerated the stress to a higher degree than C217/454S-NTRCE plants. The results suggest that the heat shock-mediated holdase chaperone function of NTRC is responsible for the increased thermotolerance of Arabidopsis and the activity is significantly supported by NADPH.
DOI:10.1093/jxb/eru207URLPMID:4112637 [本文引用: 1]

Chloroplast thioredoxins (TRXs) and glutathione function as redox messengers in the regulation of photosynthesis. In this work, the roles of chloroplast TRXs in brassinosteroids (BRs)-induced changes in cellular redox homeostasis and CO2 assimilation were studied in the leaves of tomato plants. BRs-deficient d (^im) plants showed decreased transcripts of TRX-f, TRX-m2, TRX-m1/4, and TRX-x, while exogenous BRs significantly induced CO2 assimilation and the expression of TRX-f, TRX-m2, TRX-m1/4, and TRX-x. Virus-induced gene silencing (VIGS) of the chloroplast TRX-f, TRX-m2, TRX-m1/4, and TRX-y genes individually increased membrane lipid peroxidation and accumulation of 2-Cys peroxiredoxin dimers, and decreased the activities of the ascorbate-glutathione cycle enzymes and the ratio of reduced glutathione to oxidized glutathione (GSH/GSSG) in the leaves. Furthermore, partial silencing of TRX-f, TRX-m2, TRX-m1/4, and TRX-y resulted in decreased expression of genes involved in the Benson-Calvin cycle and decreased activity of the associated enzymes. Importantly, the BRs-induced increase in CO2 assimilation and the increased expression and activities of antioxidant- and photosynthesis-related genes and enzymes were compromised in the partially TRX-f- and TRX-m1/4-silenced plants. All of these results suggest that TRX-f and TRX-m1/4 are involved in the BRs-induced changes in CO2 assimilation and cellular redox homeostasis in tomato.
DOI:10.1016/j.febslet.2011.03.006URLPMID:21385584 [本文引用: 2]

Trx-z is a chloroplastic thioredoxin, exhibiting a usual WCGPC active site, but whose biochemical properties are unknown. We demonstrate here that Trx-z supports the activity of several plastidial antioxidant enzymes, such as thiol-peroxidases and methionine sulfoxide reductases, using electrons provided by ferredoxin–thioredoxin reductase. Its disulfide reductase activity requires the presence of both active site cysteines forming a catalytic disulfide bridge with a midpoint redox potential of 61251mV at pH7. These in vitro biochemical data suggest that, besides its decisive role in the regulation of plastidial transcription, Trx-z might also be involved in stress response.
DOI:10.1089/ars.2012.4807URLPMID:23198756 [本文引用: 1]

Protein action in nature is largely controlled by the level of expression and by post-translational modifications. Post-translational modifications result in a proteome that is at least two orders of magnitude more diverse than the genome. There are three basic types of post-translational modifications: covalent modification of an amino acid side chain, hydrolytic cleavage or isomerization of a peptide bond, and reductive cleavage of a disulfide bond. This review addresses the modification of disulfide bonds. Protein disulfide bonds perform either a structural or a functional role, and there are two types of functional disulfide: the catalytic and allosteric bonds. The allosteric disulfide bonds control the function of the mature protein in which they reside by triggering a change when they are cleaved. The change can be in ligand binding, substrate hydrolysis, proteolysis, or oligomer formation. The allosteric disulfides are cleaved by oxidoreductases or by thiol/disulfide exchange, and the configurations of the disulfides and the secondary structures that they link share some recurring features. How these bonds are being identified using bioinformatics and experimental screens and what the future holds for this field of research are also discussed. Antioxid. Redox Signal. 18, 1987-2015.
DOI:10.1104/pp.112.207019URLPMID:23151348 [本文引用: 1]

In addition to the linear electron flow, a cyclic electron flow (CEF) around photosystem I occurs in chloroplasts. In CEF, electrons flow back from the donor site of photosystem I to the plastoquinone pool via two main routes: one that involves the Proton Gradient Regulation5 (PGR5)/PGRL1 complex (PGR) and one that is dependent of the NADH dehydrogenase-like complex. While the importance of CEF in photosynthesis and photoprotection has been clearly established, little is known about its regulation. We worked on the assumption of a redox regulation and surveyed the putative role of chloroplastic thioredoxins (TRX). Using Arabidopsis (Arabidopsis thaliana) mutants lacking different TRX isoforms, we demonstrated in vivo that TRXm4 specifically plays a role in the down-regulation of the NADH dehydrogenase-like complex-dependent plastoquinone reduction pathway. This result was confirmed in tobacco (Nicotiana tabacum) plants overexpressing the TRXm4 orthologous gene. In vitro assays performed with isolated chloroplasts and purified TRXm4 indicated that TRXm4 negatively controls the PGR pathway as well. The physiological significance of this regulation was investigated under steady-state photosynthesis and in the pgr5 mutant background. Lack of TRXm4 reversed the growth phenotype of the pgr5 mutant, but it did not compensate for the impaired photosynthesis and photoinhibition sensitivity. This suggests that the physiological role of TRXm4 occurs in vivo via a mechanism distinct from direct up-regulation of CEF.
DOI:10.3389/fpls.2013.00105URLPMID:23641245 [本文引用: 1]

Living organisms are subjected to oxidative stress conditions which are characterized by the production of reactive oxygen, nitrogen, and sulfur species. In plants as in other organisms, many of these compounds have a dual function as they damage different types of macromolecules but they also likely fulfil an important role as secondary messengers. Owing to the reactivity of their thiol groups, some protein cysteine residues are particularly prone to oxidation by these molecules. In the past years, besides their recognized catalytic and regulatory functions, the modification of cysteine thiol group was increasingly viewed as either protective or redox signaling mechanisms. The most physiologically relevant reversible redox post-translational modifications (PTMs) are disulfide bonds, sulfenic acids, S-glutathione adducts, S-nitrosothiols and to a lesser extent S-sulfenyl-amides, thiosulfinates and S-persulfides. These redox PTMs are mostly controlled by two oxidoreductase families, thioredoxins and glutaredoxins. This review focuses on recent advances highlighting the variety and physiological roles of these PTMs and the proteomic strategies used for their detection.
DOI:10.1104/pp.16.01500URLPMID:28827456 [本文引用: 1]

In chloroplasts, thioredoxin (TRX) isoforms and NADPH-dependent thioredoxin reductase C (NTRC) act as redox regulatory factors involved in multiple plastid biogenesis and metabolic processes. To date, less is known about the functional coordination between TRXs and NTRC in chlorophyll biosynthesis. In this study, we aimed to explore the potential functions of TRX m and NTRC in the regulation of the tetrapyrrole biosynthesis (TBS) pathway. Silencing of three genes, TRX m1, TRX m2, and TRX m4 (TRX ms), led to pale-green leaves, a significantly reduced 5-aminolevulinic acid (ALA)-synthesizing capacity, and reduced accumulation of chlorophyll and its metabolic intermediates in Arabidopsis (Arabidopsis thaliana). The contents of ALA dehydratase, protoporphyrinogen IX oxidase, the I subunit of Mg-chelatase, Mg-protoporphyrin IX methyltransferase (CHLM), and NADPH-protochlorophyllide oxidoreductase were decreased in triple TRX m-silenced seedlings compared with the wild type, although the transcript levels of the corresponding genes were not altered significantly. Protein-protein interaction analyses revealed a physical interaction between the TRX m isoforms and CHLM. 4-Acetoamido-4-maleimidylstilbene-2,2-disulfonate labeling showed the regulatory impact of TRX ms on the CHLM redox status. Since CHLM also is regulated by NTRC (Richter et al., 2013), we assessed the concurrent functions of TRX m and NTRC in the control of CHLM. Combined deficiencies of three TRX m isoforms and NTRC led to a cumulative decrease in leaf pigmentation, TBS intermediate contents, ALA synthesis rate, and CHLM activity. We discuss the coordinated roles of TRX m and NTRC in the redox control of CHLM stability with its corollary activity in the TBS pathway.
DOI:10.1104/pp.108.128314URLPMID:19109414 [本文引用: 1]

The reduction and the formation of regulatory disulfide bonds serve as a key signaling element in chloroplasts. Members of the thioredoxin (Trx) superfamily of oxidoreductases play a major role in these processes. We have characterized a small family of plant-specific Trxs in Arabidopsis (Arabidopsis thaliana) that are rich in cysteine and histidine residues and are typified by a variable noncanonical redox active site. We found that the redox midpoint potential of three selected family members is significantly less reducing than that of the classic Trxs. Assays of subcellular localization demonstrated that all proteins are localized to the chloroplast. Selected members showed high activity, contingent on a dithiol electron donor, toward the chloroplast 2-cysteine peroxiredoxin A and poor activity toward the chloroplast NADP-malate dehydrogenase. The expression profile of the family members suggests that they have distinct roles. The intermediate redox midpoint potential value of the atypical Trxs might imply adaptability to function in modulating the redox state of chloroplast proteins with regulatory disulfides.
DOI:10.1105/tpc.112.097139URLPMID:22570442 [本文引用: 2]

The transition from dark to light involves marked changes in the redox reactions of photosynthetic electron transport and in chloroplast stromal enzyme activity even under mild light and growth conditions. Thus, it is not surprising that redox regulation is used to dynamically adjust and coordinate the stromal and thylakoid compartments. While oxidation of regulatory proteins is necessary for the regulation, the identity and the mechanism of action of the oxidizing pathway are still unresolved. Here, we studied the oxidation of a thylakoid-associated atypical thioredoxin-type protein, ACHT1, in the Arabidopsis thaliana chloroplast. We found that after a brief period of net reduction in plants illuminated with moderate light intensity, a significant oxidation reaction of ACHT1 arises and counterbalances its reduction. Interestingly, ACHT1 oxidation is driven by 2-Cys peroxiredoxin (Prx), which in turn eliminates peroxides. The ACHT1 and 2-Cys Prx reaction characteristics in plants further indicated that ACHT1 oxidation is linked with changes in the photosynthetic production of peroxides. Our findings that plants with altered redox poise of the ACHT1 and 2-Cys Prx pathway show higher nonphotochemical quenching and lower photosynthetic electron transport infer a feedback regulatory role for this pathway.
.
[本文引用: 2]
DOI:10.1104/pp.110.170043URLPMID:21205617 [本文引用: 1]

Plant Physiol. 2011 Apr;155(4):1477-85. doi: 10.1104/pp.110.170043. Epub 2010 Dec 30. Research Support, Non-U.S. Gov't
DOI:10.1073/pnas.0912952107URLPMID:20018758 [本文引用: 1]

Blood coagulation in humans requires the activity of vitamin K epoxide reductase (VKOR), the target of the anticoagulant warfarin (Coumadin). Bacterial homologs of VKOR were recently found to participate in a pathway leading to disulfide bond formation in secreted proteins of many bacteria. Here we show that the VKOR homolog from the bacterium Mycobacterium tuberculosis, the causative agent of human tuberculosis, is inhibited by warfarin and that warfarin-resistant mutations of mycobacterial VKOR appear in similar locations to mutations found in human patients who require higher doses of warfarin. Deletion of VKOR results in a severe growth defect in mycobacteria, and the growth of M. tuberculosis is inhibited by warfarin. The bacterial VKOR homolog may represent a target for antibiotics and a model for genetic studies of human VKOR. We present a simple assay in Escherichia coli, based on a disulfide-sensitive galactosidase, which can be used to screen for stronger inhibitors of the M. tuberculosis VKOR homolog.
DOI:10.1073/pnas.1515513112URLPMID:26424450 [本文引用: 2]

The regulatory mechanisms that use signals of low levels of reactive oxygen species (ROS) could be obscured by ROS produced under stress and thus are better investigated under homeostatic conditions. Previous studies showed that the chloroplastic atypical thioredoxin ACHT1 is oxidized by 2-Cys peroxiredoxin (2-Cys Prx) in Arabidopsis plants illuminated with growth light and in turn transmits a disulfide-based signal via yet unknown target proteins in a feedback regulation of photosynthesis. Here, we studied the role of a second chloroplastic paralog, ACHT4, in plants subjected to low light conditions. Likewise, ACHT4 reacted in planta with 2-Cys Prx, indicating that it is oxidized by a similar disulfide exchange reaction. ACHT4 further reacted uniquely with the small subunit (APS1) of ADP-glucose pyrophosphorylase (AGPase), the first committed enzyme of the starch synthesis pathway, suggesting that it transfers the disulfides it receives from 2-Cys Prx to APS1 and turns off AGPase. In accordance, ACHT4 participated in an oxidative signal that quenched AGPase activity during the diurnal transition from day to night, and also in an attenuating oxidative signal of AGPase in a dynamic response to small fluctuations in light intensity during the day. Increasing the level of expressed ACHT4 or of ACHT4 C, a C terminus-deleted form that does not react with APS1, correspondingly decreased or increased the level of reduced APS1 and decreased or increased transitory starch content. These findings imply that oxidative control mechanisms act in concert with reductive signals to fine tune starch synthesis during daily homeostatic conditions.
DOI:10.1111/j.1742-4658.2011.08265.xURLPMID:21781282 [本文引用: 1]

In chloroplasts, thiol/disulfide-redox-regulated proteins have been linked to numerous metabolic pathways. However, the biochemical system for disulfide bond formation in chloroplasts remains undetermined. In the present study, we characterized an oxidoreductase, AtVKOR-DsbA, encoded by the gene At4g35760 as a potential disulfide bond oxidant in Arabidopsis. The gene product contains two distinct domains: an integral membrane domain homologous to the catalytic subunit of mammalian vitamin K epoxide reductase (VKOR) and a soluble DsbA-like domain. Transient expression of green fluorescent protein fusion in Arabidopsis protoplasts indicated that AtVKOR-DsbA is located in the chloroplast. The first 45 amino acids from the N-terminus were found to act as a transit peptide targeting the protein to the chloroplast. An immunoblot assay of chloroplast fractions revealed that AtVKOR-DsbA was localized in the thylakoid. A motility complementation assay showed that the full-length of AtVKOR-DsbA, if lacking its transit peptide, could catalyze the formation of disulfide bonds. Among the 10 cysteine residues present in the mature protein, eight cysteines (four in the AtVKOR domain and four in the AtDsbA domain) were found to be essential for promoting disulfide bond formation. The topological arrangement of AtVKOR-DsbA was assayed using alkaline phosphatase sandwich fusions. From these results, we developed a possible topology model of AtVKOR-DsbA in chloroplasts. We propose that the integral membrane domain of AtVKOR-DsbA contains four transmembrane helices, and that both termini and the cysteines involved in catalyzing the formation of disulfide bonds face the oxidative thylakoid lumen. These studies may help to resolve some of the issues surrounding the structure and function of AtVKOR-DsbA in Arabidopsis chloroplasts.Structured digital abstract AtVKOR-DsbA and D1 colocalize by cosedimentation in solution (View interaction)
.
DOI:10.1371/journal.pone.0035669URLPMID:3338714 [本文引用: 2]

Plants are often exposed to saturating light conditions, which can lead to oxidative stress. The carotenoid zeaxanthin, synthesized from violaxanthin by Violaxanthin De-Epoxidase (VDE) plays a major role in the protection from excess illumination. VDE activation is triggered by a pH reduction in the thylakoids lumen occurring under saturating light. In this work the mechanism of the VDE activation was investigated on a molecular level using multi conformer continuum electrostatic calculations, site directed mutagenesis and molecular dynamics. The pKa values of residues of the inactive VDE were determined to identify target residues that could be implicated in the activation. Five such target residues were investigated closer by site directed mutagenesis, whereas variants in four residues (D98, D117, H168 and D206) caused a reduction in enzymatic activity indicating a role in the activation of VDE while D86 mutants did not show any alteration. The analysis of the VDE sequence showed that the four putative activation residues are all conserved in plants but not in diatoms, explaining why VDE in these algae is already activated at higher pH. Molecular dynamics showed that the VDE structure was coherent at pH 7 with a low amount of water penetrating the hydrophobic barrel. Simulations carried out with the candidate residues locked into their protonated state showed instead an increased amount of water penetrating the barrel and the rupture of the H121 214 hydrogen bond at the end of the barrel, which is essential for VDE activation. These results suggest that VDE activation relies on a robust and redundant network, in which the four residues identified in this study play a major role.
DOI:10.1111/j.1365-313X.2010.04305.xURLPMID:20626653 [本文引用: 1]

Summary Top of page Summary Introduction Results Discussion Experimental Procedures Acknowledgements References Supporting Information Plants and certain species of cyanobacteria are the only organisms capable of synthesizing phylloquinone (vitaminK 1 for vertebrates), which they use as an electron carrier during photosynthesis. Recent studies, however, have identified a plastidial pool of non-photoactive phylloquinone that could be involved in additional cellular functions. Here, we characterized an Arabidopsis bimodular enzyme the At4g35760 gene product comprising an integral domain homologous to the catalytic subunit of mammalian vitaminK epoxide reductase (VKORC1, EC1.1.4.1) that is fused to a soluble thioredoxin-like moiety. GFP-fusion experiments in tobacco mesophyll cells established that the plant protein is targeted to plastids, and analyses of transcript and protein levels showed that expression is maximal in leaf tissues. The fused and individual VKORC1 domains were separately expressed in yeast, removing their chloroplast targeting pre-sequence and adding a C-terminal consensus signal for retention in the endoplasmic reticulum. The corresponding microsomal preparations were equally effective at mediating the dithiotreitol-dependent reduction of phylloquinone and menaquinone into their respective quinol forms. Strikingly, unlike mammalian VKORC1, the Arabidopsis enzyme did not reduce phylloquinone epoxide, and was resistant to inhibition by warfarin. The isoprenoid benzoquinone conjugates plastoquinone and ubiquinone were not substrates, establishing that the plant enzyme evolved strict specificity for the quinone form of naphthalenoid conjugates. Invitro reconstitution experiments established that the soluble thioredoxin-like domain can function as an electron donor for its integral VKORC1 partner.
DOI:10.1089/ars.2014.6018URLPMID:4158967 [本文引用: 2]

Reduction-oxidation (Redox) status operates as a major integrator of subcellular and extracellular metabolism and is simultaneously itself regulated by metabolic processes. Redox status not only dominates cellular metabolism due to the prominence of NAD(H) and NADP(H) couples in myriad metabolic reactions but also acts as an effective signal that informs the cell of the prevailing environmental conditions. After relay of this information, the cell is able to appropriately respond via a range of mechanisms, including directly affecting cellular functioning and reprogramming nuclear gene expression.The facile accession of Arabidopsis knockout mutants alongside the adoption of broad-scale post-genomic approaches, which are able to provide transcriptomic-, proteomic-, and metabolomic-level information alongside traditional biochemical and emerging cell biological techniques, has dramatically advanced our understanding of redox status control. This review summarizes redox status control of metabolism and the metabolic control of redox status at both cellular and subcellular levels.It is becoming apparent that plastid, mitochondria, and peroxisome functions influence a wide range of processes outside of the organelles themselves. While knowledge of the network of metabolic pathways and their intraorganellar redox status regulation has increased in the last years, little is known about the interorganellar redox signals coordinating these networks. A current challenge is, therefore, synthesizing our knowledge and planning experiments that tackle redox status regulation at both inter- and intracellular levels.Emerging tools are enabling ever-increasing spatiotemporal resolution of metabolism and imaging of redox status components. Broader application of these tools will likely greatly enhance our understanding of the interplay of redox status and metabolism as well as elucidating and characterizing signaling features thereof. We propose that such information will enable us to dissect the regulatory hierarchies that mediate the strict coupling of metabolism and redox status which, ultimately, determine plant growth and development.
[本文引用: 2]
DOI:10.1016/j.plantsci.2016.11.003URLPMID:28131337 [本文引用: 1]

Redox regulation of chloroplast enzymes via disulphide reduction is believed to control the rates of CO2fixation. The study of the thioredoxin reduction pathways and of various target enzymes lead to the following guidelines: i)Thioredoxin gene content is greatly higher in photosynthetic eukaryotes compared to prokaryotes;ii)Thioredoxin-reducing pathways have expanded in photosynthetic eukaryotes with four different thioredoxin reductases and the possibility to reduce some thioredoxins via glutaredoxins;iii)Some enzymes that were thought to be strictly linked to photosynthesis ferredoxin-thioredoxin reductase, phosphoribulokinase, ribulose-1,5-bisphosphate carboxylase/oxygenase, sedoheptulose-1,7-bisphosphatase are present in non-photosynthetic organisms;iv)Photosynthetic eukaryotes contain a genetic patchwork of sequences borrowed from prokaryotes including roteobacteria and archaea;v)The introduction of redox regulatory sequences did not occur at the same place for all targets. Some possess critical cysteines in cyanobacteria, for others the transition occurred rather at the green algae level;vi)Generally the regulatory sites of the target enzymes are distally located from the catalytic sites. The cysteine residues are generally not involved in catalysis. Following reduction, molecular movements open the active sites and make catalysis possible;vii)The regulatory sequences are located on surface-accessible loops. At least one instance they can be cut out and serve as signal peptides for inducing plant defence.
DOI:10.1073/pnas.1606241113URLPMID:27226308 [本文引用: 2]

The Calvin–Benson cycle of carbon dioxide fixation in chloroplasts is controlled by light-dependent redox reactions that target specific enzymes. Of the regulatory members of the cycle, our knowledge of sedoheptulose-1,7-bisphosphatase (SBPase) is particularly scanty, despite growing evidence for its importance and link to plant productivity. To help fill this gap,...
DOI:10.1002/pmic.200900654URLPMID:20049866 [本文引用: 2]

The light-dependent regulation of stromal enzymes by thioredoxin (Trx)-catalysed disulphide/dithiol exchange is known as a classical mechanism for control of chloroplast metabolism. Recent proteome studies show that Trx targets are present not only in the stroma but in all chloroplast compartments, from the envelope to the thylakoid lumen. Trx-mediated redox control appears to be a common feature of important pathways, such as the Calvin cycle, starch synthesis and tetrapyrrole biosynthesis. However, the extent of thiol-dependent redox regulation in the thylakoid lumen has not been previously systematically explored. In this study, we addressed Trx-linked redox control in the chloroplast lumen of Arabidopsis thaliana. Using complementary proteomics approaches, we identified 19 Trx target proteins, thus covering more than 40% of the currently known lumenal chloroplast proteome. We show that the redox state of thiols is decisive for degradation of the extrinsic PsbO1 and PsbO2 subunits of photosystem II. Moreover, disulphide reduction inhibits activity of the xanthophyll cycle enzyme violaxanthin de-epoxidase, which participates in thermal dissipation of excess absorbed light. Our results indicate that redox-controlled reactions in the chloroplast lumen play essential roles in the function of photosystem II and the regulation of adaptation to light intensity.
DOI:10.1007/s11120-015-0118-9URLPMID:25764016 [本文引用: 1]

Violaxanthin de-epoxidase (VDE) catalyses the conversion of violaxanthin to zeaxanthin at the lumen side of the thylakoids during exposure to intense light. VDE consists of a cysteine-rich N-terminal domain, a lipocalin-like domain and a negatively charged C-terminal domain. That the cysteines are important for the activity of VDE is well known, but in what way is less understood. In this study, wild-type spinach VDE was expressed in E. coli as inclusion bodies, refolded and purified to give a highly active and homogenous preparation. The metal content (Fe, Cu, Ni, Mn, Co and Zn) was lower than 1 mol% excluding a metal-binding function of the cysteines. To investigate which of the 13 cysteines that could be important for the function of VDE, we constructed mutants where the cysteines were replaced by serines, one by one. For 12 out of 13 mutants the activity dropped by more than 99.9%. A quantification of free cysteines showed that only the most N-terminal of these cysteines was in reduced form in the native VDE. A disulphide pattern in VDE of C9-C27, C14-C21, C33-C50, C37-C46, C65-C72 and C118-C284 was obtained after digestion of VDE with thermolysin followed by mass spectroscopy analysis of reduced versus non-reduced samples. The residual activity found for the mutants showed a variation that was consistent with the results obtained from mass spectroscopy. Reduction of the disulphides resulted in loss of a rigid structure and a decrease in thermal stability of 15 C.
DOI:10.1016/j.molcel.2012.11.030URLPMID:23290914 [本文引用: 1]

78 PGRL1 is the elusive FQR which has been sought for almost three decades 78 PGR5 is required for the transfer of electrons from ferredoxin to PGRL1 78 The six redox-active cysteine residues of PGRL1 can form intra- and intermolecular disulfide bridges 78 Thioredoxins destabilize PGRL1 homodimers
[本文引用: 1]
DOI:10.1016/j.tplants.2009.03.005URL [本文引用: 1]

http://linkinghub.elsevier.com/retrieve/pii/S1360138509001204
DOI:10.3389/fpls.2013.00434URLPMID:24198822 [本文引用: 1]

It has been known for a long time that the thylakoid lumen provides the environment for oxygen evolution, plastocyanin-mediated electron transfer, and photoprotection. More recently lumenal proteins have been revealed to play roles in numerous processes, most often linked with regulating thylakoid biogenesis and the activity and turnover of photosynthetic protein complexes, especially the photosystem II and NAD(P)H dehydrogenase-like complexes. Still, the functions of the majority of lumenal proteins inArabidopsis thalianaare unknown. Interestingly, while the thylakoid lumen proteome of at least 80 proteins contains several large protein families, individual members of many protein families have highly divergent roles. This is indicative of evolutionary pressure leading to neofunctionalization of lumenal proteins, emphasizing the important role of the thylakoid lumen for photosynthetic electron transfer and ultimately for plant fitness. Furthermore, the involvement of anterograde and retrograde signaling networks that regulate the expression and activity of lumen proteins is increasingly pertinent. Recent studies have also highlighted the importance of thiol/disulfide modulation in controlling the functions of many lumenal proteins and photosynthetic regulation pathways.
DOI:10.1016/j.jplph.2015.12.012URLPMID:26812087 [本文引用: 2]

Higher plants need to balance the efficiency of light energy absorption and dissipative photo-protection when exposed to fluctuations in light quantity and quality. This aim is partially realized through redox regulation within the chloroplast, which occurs in all chloroplast compartments except the envelope intermembrane space. In contrast to the chloroplast stroma, less attention has been paid to the thylakoid lumen, an inner, continuous space enclosed by the thylakoid membrane in which redox regulation is also essential for photosystem biogenesis and function. This sub-organelle compartment contains at least 80 lumenal proteins, more than 30 of which are known to contain disulfide bonds. Thioredoxins (Trx) in the chloroplast stroma are photo-reduced in the light, transferring reducing power to the proteins in the thylakoid membrane and ultimately the lumen through a trans-thylakoid membrane-reduced, equivalent pathway. The discovery of lumenal thiol oxidoreductase highlights the importance of the redox regulation network in the lumen for controlling disulfide bond formation, which is responsible for protein activity and folding and even plays a role in photo-protection. In addition, many lumenal members involved in photosystem assembly and non-photochemical quenching are likely required for reduction and/or oxidation to maintain their proper efficiency upon changes in light intensity. In light of recent findings, this review summarizes the multiple redox processes that occur in the thylakoid lumen in great detail, highlighting the essential auxiliary roles of lumenal proteins under fluctuating light conditions.
DOI:10.1105/tpc.111.089680URLPMID:22209765 [本文引用: 2]

Here, we identify Arabidopsis thaliana Lumen Thiol Oxidoreductase1 (LTO1) as a disulfide bond-forming enzyme in the thylakoid lumen. Using topological reporters in bacteria, we deduced a lumenal location for the redox active domains of the protein. LTO1 can partially substitute for the proteins catalyzing disulfide bond formation in the bacterial periplasm, which is topologically equivalent to the plastid lumen. An insertional mutation within the LTO1 promoter is associated with a severe photoautotrophic growth defect. Measurements of the photosynthetic activity indicate that the Ito1 mutant displays a limitation in the electron flow from photosystem II (PSII). In accordance with these measurements, we noted a severe depletion of the structural subunits of PSII but no change in the accumulation of the cytochrome b complex or photosystem I. In a yeast two-hybrid assay, the thioredoxin-like domain of LTO1 interacts with PsbO, a lumenal PSII subunit known to be disulfide bonded, and a recombinant form of the molecule can introduce a disulfide bond in PsbO in vitro. The documentation of a sulfhydryl-oxidizing activity in the thylakoid lumen further underscores the importance of catalyzed thiol-disulfide chemistry for the biogenesis of the thylakoid compartment.
.
[本文引用: 2]
[本文引用: 1]
.
[本文引用: 1]
[本文引用: 1]
.
[本文引用: 1]
DOI:10.1016/j.pbi.2011.12.002URLPMID:22226570 [本文引用: 1]

Plant cells sense, weigh and integrate various endogenous and exogenous cues in order to optimize acclimation and resource allocation. The thiol/disulfide redox network appears to be in the core of this versatile integration process. In plant cells its complexity exceeds by far that of other organisms. Recent research has elucidated the multiplicity of the diversified input elements, transmitters (thioredoxin, glutaredoxins), targets and sensors (peroxiredoxins and other peroxidases), controlled processes and final acceptors (reactive oxygen species). An additional level of thiol/disulfide regulation is achieved by introducing dynamics in time and subcompartment and complex association.
DOI:10.1111/pce.12005URLPMID:22943306 [本文引用: 1]

Methionine (Met) in proteins can be oxidized to two diastereoisomers of methionine sulfoxide, Met-S-O and Met-R-O, which are reduced back to Met by two types of methionine sulfoxide reductases (MSRs), A and B, respectively. MSRs are generally supplied with reducing power by thioredoxins. Plants are characterized by a large number of thioredoxin isoforms, but those providing electrons to MSRs in vivo are not known. Three MSR isoforms, MSRA4, MSRB1 and MSRB2, are present in Arabidopsis thaliana chloroplasts. Under conditions of high light and long photoperiod, plants knockdown for each plastidial MSR type or for both display reduced growth. In contrast, overexpression of plastidial MSRBs is not associated with beneficial effects in terms of growth under high light. To identify the physiological reductants for plastidial MSRs, we analyzed a series of mutants deficient for thioredoxins f, m, x or y. We show that mutant lines lacking both thioredoxins y1 and y2 or only thioredoxin y2 specifically display a significantly reduced leaf MSR capacity (25%) and growth characteristics under high light, related to those of plants lacking plastidial MSRs. We propose that thioredoxin y2 plays a physiological function in protein repair mechanisms as an electron donor to plastidial MSRs in photosynthetic organs.
DOI:10.4161/psb.4.6.8711URLPMID:19151130 [本文引用: 1]

The biogenesis and function of chloroplast are controlled both by anterograde mechanisms involving nuclear-encoded proteins targeted to chloroplast and by retrograde signals from plastid to nucleus contributing to regulation of nuclear gene expression. A number of experimental evidences support the implication of chlorophyll biosynthesis intermediates on the retrograde signaling, albeit an earlier-postulated direct link between accumulation of chlorophyll intermediates and changes in nuclear gene expression has recently been challenged. By characterization of Arabidopsis mutants lacking the chloroplast localized NADPH-thioredoxin reductase (NTRC) we have recently proposed that imbalanced activity of chlorophyll biosynthesis in developing cells modifies the chloroplast signals leading to alterations in nuclear gene expression. These signals appear to initiate from temporal perturbations in the flux through the pathway from protoporphyrin to protochlorophyllide rather than from the accumulation of a single intermediate of the tetrapyrrole pathway.
DOI:10.1016/j.jprot.2009.01.003URLPMID:19185068 [本文引用: 2]

Light-dependent disulphide/dithiol exchange catalysed by thioredoxin is a classical example of redox regulation of chloroplast enzymes. Recent proteome studies have mapped thioredoxin target proteins in all chloroplast compartments ranging from the envelope to the thylakoid lumen. Progress in the methodologies has made it possible to identify which cysteine residues interact with thioredoxin and to tackle membrane-bound thioredoxin targets. To date, more than hundred targets of thioredoxin and glutaredoxin have been found in plastids from Arabidopsis, spinach, poplar and Chlamydomonas reinhardtii. Thioredoxin-mediated redox control appears to be a feature of the central pathways for assimilation and storage of carbon, sulphur and nitrogen, as well as for translation and protein folding. Cyanobacteria are oxygenic photosynthetic prokaryotes, which presumably share a common ancestor with higher plant plastids. As in chloroplasts, cyanobacterial thioredoxins receive electrons from the photosynthetic electron transport, and thioredoxin-targeted proteins are therefore highly interesting in the context of acclimation of these organisms to their environment. Studies of the unicellular model cyanobacterium Synechocystis sp. PCC 6803 revealed 77 thioredoxin target proteins. Notably, the functions of all these thioredoxin targets highlight essentially the same processes as those described in chloroplasts suggesting that thioredoxin-mediated redox signalling is equally significant in oxygenic photosynthetic prokaryotes and eukaryotes.
DOI:10.1089/ars.2010.3551URLPMID:21275844 [本文引用: 1]

Ten years ago, proteomics techniques designed for large-scale investigations of redox-sensitive proteins started to emerge. The proteomes, defined as sets of proteins containing reactive cysteines that undergo oxidative post-translational modifications, have had a particular impact on research concerning the redox regulation of cellular processes. These proteomes, which are hereafter termed "disulfide proteomes," have been studied in nearly all kingdoms of life, including animals, plants, fungi, and bacteria. Disulfide proteomics has been applied to the identification of proteins modified by reactive oxygen and nitrogen species under stress conditions. Other studies involving disulfide proteomics have addressed the functions of thioredoxins and glutaredoxins. Hence, there is a steadily growing number of proteins containing reactive cysteines, which are probable targets for redox regulation. The disulfide proteomes have provided evidence that entire pathways, such as glycolysis, the tricarboxylic acid cycle, and the Calvin-Benson cycle, are controlled by mechanisms involving changes in the cysteine redox state of each enzyme implicated. Synthesis and degradation of proteins are processes highly represented in disulfide proteomes and additional biochemical data have established some mechanisms for their redox regulation. Thus, combined with biochemistry and genetics, disulfide proteomics has a significant potential to contribute to new discoveries on redox regulation and signaling.
DOI:10.1007/s00299-013-1455-9URLPMID:23689258 [本文引用: 1]

The role of LTO1/ At VKOR-DsbA in ROS homeostasis and in redox regulation of cysteine-containing proteins in chloroplast was studied in lto1 - 2 mutant, and a potential target of LTO1 was captured.A chloroplast membrane protein LTO1/AtVKOR-DsbA encoded by the gene At4g35760 was recently found to be an oxidoreductase and involved in assembly of PSII. Here, the growth of a mutant lto1-2 line of Arabidopsis was found to be severely stunted and transgenic complementation ultimately demonstrated the phenotype changes were due to this gene. A proteomic experiment identified 23 proteins presenting a differential abundance in lto1-2 compared with wild-type plants, including components in PSII and proteins scavenging active oxygen. Three scavengers of active oxygen, l-ascorbate peroxidase 1, peroxisomal catalase 2, dehydroascorbate reductase 1, are reduced in lto1-2 plants, corresponding to high levels of accumulation of reactive oxygen species (ROS). The photosynthetic activities of PSII and the quantity of core protein D1 decreased significantly in lto1-2. Further investigation showed the synthesis of D1 was not affected in mutants both at transcription and translation levels. The soluble DsbA-like domain of LTO1 was found to have reduction, oxidation and isomerization activities, and could promote the formation of disulfide bonds in a lumenal protein, FKBP13. A potential target of LTO1 was captured which was involving in chlorophyll degradation and photooxidative stress response. Experimental results imply that LTO1 plays important roles in redox regulation, ROS homeostasis and maintenance of PSII.
[本文引用: 1]
DOI:10.1089/ars.2011.4327URLPMID:22531002 [本文引用: 1]

Abstract Thioredoxins (Trx) and glutaredoxins (Grx) constitute families of thiol oxidoreductases. Our knowledge of Trx and Grx in plants has dramatically increased during the last decade. The release of the Arabidopsis genome sequence revealed an unexpectedly high number of Trx and Grx genes. The availability of several genomes of vascular and nonvascular plants allowed the establishment of a clear classification of the genes and the chronology of their appearance during plant evolution. Proteomic approaches have been developed that identified the putative Trx and Grx target proteins which are implicated in all aspects of plant growth, including basal metabolism, iron/sulfur cluster formation, development, adaptation to the environment, and stress responses. Analyses of the biochemical characteristics of specific Trx and Grx point to a strong specificity toward some target enzymes, particularly within plastidial Trx and Grx. In apparent contradiction with this specificity, genetic approaches show an absence of phenotype for most available Trx and Grx mutants, suggesting that redundancies also exist between Trx and Grx members. Despite this, the isolation of mutants inactivated in multiple genes and several genetic screens allowed the demonstration of the involvement of Trx and Grx in pathogen response, phytohormone pathways, and at several control points of plant development. Cytosolic Trxs are reduced by NADPH-thioredoxin reductase (NTR), while the reduction of Grx depends on reduced glutathione (GSH). Interestingly, recent development integrating biochemical analysis, proteomic data, and genetics have revealed an extensive crosstalk between the cytosolic NTR/Trx and GSH/Grx systems. This crosstalk, which occurs at multiple levels, reveals the high plasticity of the redox systems in plants.
[本文引用: 1]
DOI:10.1073/pnas.0903559106URL [本文引用: 1]

Plants have an unusual plastid-localized NADP-thioredoxin reductase C (NTRC) containing both an NADP-thioredoxin reductase (NTR) and a thioredoxin (Trx) domain in a single polypeptide. Although NTRC is known to supply reductant for detoxifying hydrogen peroxide in the dark, its other functions are unknown. We now report that NTRC plays a previously unrecognized role in the redox regulation of ADP-glucose pyrophosphorylase (AGPase), a central enzyme of starch synthesis. When supplied NADPH, NTRC activated AGPase in vitro in a redox reaction that required the active site cysteines of both domains of the enzyme. In leaves, AGPase was activated in planta either by light or external feeding of sucrose in the dark. Leaves of an Arabidopsis NTRC KO mutant showed a decrease both in the extent of redox activation of AGPase and in the enhancement of starch synthesis either in the light (by 40-60%) or in the dark after treatment with external sucrose (by almost 100%). The light-dependent activation of AGPase in isolated chloroplasts, by contrast was unaffected. In nonphotosynthetic tissue (roots), KO of NTRC decreased redox activation of AGPase and starch synthesis in response to light or external sucrose by almost 90%. The results provide biochemical and genetic evidence for a role of NTRC in regulating starch synthesis in response to either light or sucrose. The data also suggest that the Trx domain of NTRC and, to a lesser extent, free Trxs linked to ferredoxin enable amyloplasts of distant sink tissues to sense light used in photosynthesis by leaf chloroplasts and adjust heterotrophic starch synthesis accordingly.
DOI:10.3389/fpls.2013.00470URLPMID:24324475 [本文引用: 1]

Reversible redox post-translational modifications such as oxido-reduction of disulfide bonds, S-nitrosylation, and S-glutathionylation, play a prominent role in the regulation of cell metabolism and signaling in all organisms. These modifications are mainly controlled by members of the thioredoxin and glutaredoxin families. Early studies in photosynthetic organisms have identified the Calvin–Benson cycle, the photosynthetic pathway responsible for carbon assimilation, as a redox regulated process. Indeed, 4 out of 11 enzymes of the cycle were shown to have a low activity in the dark and to be activated in the light through thioredoxin-dependent reduction of regulatory disulfide bonds. The underlying molecular mechanisms were extensively studied at the biochemical and structural level. Unexpectedly, recent biochemical and proteomic studies have suggested that all enzymes of the cycle and several associated regulatory proteins may undergo redox regulation through multiple redox post-translational modifications including glutathionylation and nitrosylation. The aim of this review is to detail the well-established mechanisms of redox regulation of Calvin–Benson cycle enzymes as well as the most recent reports indicating that this pathway is tightly controlled by multiple interconnected redox post-translational modifications. This redox control is likely allowing fine tuning of the Calvin–Benson cycle required for adaptation to varying environmental conditions, especially during responses to biotic and abiotic stresses.
DOI:10.1016/j.jprot.2008.12.002URLPMID:19135183 [本文引用: 1]

The turn of the century welcomed major developments in redox biology. In plants, proteomics made possible the identification of proteins linked to thioredoxin (Trx), initially in chloroplasts and then other cell compartments. Two procedures, one based on thiol specific probes and the other on mutant Trx proteins, facilitated the labeling or isolation of potential Trx targets that were later identified with proteomic approaches. As a result, the number of targets in land plants increased 10-fold from fewer than 40 to more than 400. Additional targets have been identified in green algae and cyanobacteria, making a grand total of 500 in oxygenic photosynthetic organisms. Collectively these proteins have the potential to influence virtually every major process of the cell. A number of laboratories currently seek to confirm newly identified Trx targets by biochemical and genetic approaches. Almost certainly many new targets become redox active during oxidative stress, enabling the plant to cope with changing environments. Under these conditions, certain targets may be glutathionylated or nitrosylated such that reversion to the original reduced state is facilitated not only by Trx, but also, in some cases preferably, by glutaredoxin. When judging changes linked to Trx, it is prudent to recognize that effects transcend classical light/dark or oxidative regulation and fall in other arenas, in some cases yet to be defined. While future work will continue to give insight into functional details, it is clear that Trx plays a fundamental role in regulating diverse processes of the living cell.
DOI:10.1089/ars.2010.3138URLPMID:20214498 [本文引用: 4]

In order to transfer reducing equivalents into the thylakoid lumen, a specific thylakoid membrane transfer system is suggested that mediates the disulfide bond reduction of proteins in the thylakoid lumen of higher plant chloroplasts. In this system, although stromal thioredoxin can supply the reducing equivalents to a thioredoxin-like protein HCF164 in the thylakoid lumen, a mediator protein for electron transfer in the thylakoid membranes is proposed to be required to link the two suborganellar compartments. CcdA is a candidate protein as a component for this transfer system since CcdA- and HCF164-deficient mutants in Arabidopsis thaliana show the same phenotype. We now show that CcdA is localized in the thylakoid membrane and that its redox state, as well as that of HCF164, is modulated in thylakoids by stromal m-type thioredoxin. Our results strongly suggest that CcdA may act as a mediator in thylakoid membranes by transferring reducing equivalents from the stromal to the lumenal side of the thylakoid membrane in chloroplasts.
.
DOI:10.1093/jxb/erw017URLPMID:26842981 [本文引用: 1]

Arabidopsisplants lacking type-fthioredoxins show an impairment of photosynthetic performance indicating specific functions for these thioredoxins not compensated for by other redox systems.These functions only affect growth under short-day conditions. Redox regulation plays a central role in the adaptation of chloroplast metabolism to light. Extensive biochemical analysesin vitrohave identifiedf-type thioredoxins (Trxs) as the most important catalysts for light-dependent reduction and activation of the enzymes of the Calvin enson cycle. However, the precise function of typefTrxsin vivoand their impact on plant growth are still poorly known. To address this issue we have generated anArabidopsis thalianadouble knock-out mutant, termedtrxf1f2, devoid of bothf1 andf2 Trxs. Despite the essential function previously proposed forf-type Trxs, the visible phenotype of thetrxf1f2double mutant was virtually indistinguishable from the wild type when grown under a long-day photoperiod. However, the Trxf-deficient plants showed growth inhibition under a short-day photoperiod which was not rescued at high light intensity. The absence off-type Trxs led to significantly lower photosynthetic electron transport rates and higher levels of non-photochemical energy quenching. Notably, the Trxfnull mutant suffered from a shortage of photosystem I electron acceptors and delayed activation of carbon dioxide fixation following a dark ight transition. Two redox-regulated Calvin enson cycle enzymes, fructose 1,6-bisphosphatase (FBPase) and Rubisco activase, showed retarded and incomplete reduction in the double mutant upon illumination, compared with wild-type plants. These results show that the function off-type Trxs in the rapid activation of carbon metabolism in response to light is not entirely compensated for by additional plastid redox systems, and suggest that these Trxs have an important role in the light adjustment of photosynthetic metabolism.
DOI:10.1111/pce.12652URLPMID:26476233 [本文引用: 1]

High irradiances may lead to photo-oxidative stress in and non-photochemical quenching () contributes to protection against excess excitation. One of the mechanisms, qE, involves thermal dissipation of the light energy captured. Importantly, need to tune down qE under light-limiting conditions for efficient utilisation of the available quanta. Considering the possible redox control of responses to excess light implying enzymes, such as , we have studied the role of the NADPH C (). Whereas lacking tolerate high light intensities, these display drastically elevated qE, have larger trans- pH and ten-fold higher levels under low and medium light intensities, leading to extremely low linear rates. To test the impact of the high qE on plant , we generated an ntrc-psbs double knockout mutant, which is devoid of qE. This double mutant grows faster than the ntrc mutant and has higher content. The II activity is partially restored in the ntrc-psbs mutant and linear rates under low and medium light intensities are twice as high as compared to lacking ntrc alone. These data uncover a new role for in the control of photosynthetic yield. This article is protected by copyright. All rights reserved.
DOI:10.1016/j.febslet.2009.07.035URLPMID:19631646 [本文引用: 2]

Glucose-6-phosphate dehydrogenase (G6PDH) is the key enzyme of the oxidative pentose phosphate pathway supplying reducing power (as NADPH) in non-photosynthesizing cells. We have examined in detail the redox regulation of the plastidial isoform predominantly present in Arabidopsis green tissues (AtG6PDH1) and found that its oxidative activation is strictly dependent on plastidial thioredoxins (Trxs) that show differential efficiencies. Light/dark modulation of AtG6PDH1 was reproduced in vitro in a reconstituted ferredoxin/Trx system using f-type Trx allowing to propose a new function for this Trx isoform co-ordinating both reductive (Calvin cycle) and oxidative pentose phosphate pathways.
DOI:10.1098/rstb.2013.0224URLPMID:3949389 [本文引用: 1]

Plants have adopted a number of mechanisms to restore redox homeostasis in the chloroplast under fluctuating light conditions in nature. Chloroplast thioredoxin systems are crucial components of this redox network, mediating environmental signals to chloroplast proteins. In the reduced state, thioredoxins control the structure and function of proteins by reducing disulfide bridges in the redox active site of a protein. Subsequently, an oxidized thioredoxin is reduced by a thioredoxin reductase, the two enzymes together forming a thioredoxin system. Plant chloroplasts have versatile thioredoxin systems, including two reductases dependent on ferredoxin and NADPH as reducing power, respectively, several types of thioredoxins, and the system to deliver thiol redox signals to the thylakoid membrane and lumen. Light controls the activity of chloroplast thioredoxin systems in two ways. First, light reactions activate the thioredoxin systems via donation of electrons to oxidized ferredoxin and NADP+, and second, light induces production of reactive oxygen species in chloroplasts which deactivate the components of the thiol redox network. The diversity and partial redundancy of chloroplast thioredoxin systems enable chloroplast metabolism to rapidly respond to ever-changing environmental conditions and to raise plant fitness in natural growth conditions.
DOI:10.1098/rstb.2016.0474URLPMID:5566889 [本文引用: 2]

Thioredoxins (TRXs) are protein oxidoreductases that control the structure and function of cellular proteins by cleavage of a disulphide bond between the side chains of two cysteine residues. Oxidized thioredoxins are reactivated by thioredoxin reductases (TR) and a TR-dependent reduction of TRXs is called a thioredoxin system. Thiol-based redox regulation is an especially important mechanism to control chloroplast proteins involved in biogenesis, in regulation of light harvesting and distribution of light energy between photosystems, in photosynthetic carbon fixation and other biosynthetic pathways, and in stress responses of plants. Of the two plant plastid thioredoxin systems, the ferredoxin-dependent system relays reducing equivalents from photosystem I via ferredoxin and ferredoxin-thioredoxin reductase (FTR) to chloroplast proteins, while NADPH-dependent thioredoxin reductase (NTRC) forms a complete thioredoxin system including both reductase and thioredoxin domains in a single polypeptide. Chloroplast thioredoxins transmit environmental light signals to biochemical reactions, which allows fine tuning of photosynthetic processes in response to changing environmental conditions. In this paper we focus on the recent reports on specificity and networking of chloroplast thioredoxin systems and evaluate the prospect of improving photosynthetic performance by modifying the activity of thiol regulators in plants. This article is part of the themed issue nhancing photosynthesis in crop plants: targets for improvement'.
DOI:10.1111/pce.12718URLPMID:26831830 [本文引用: 4]

Thioredoxins (TRXs) mediate light‐dependent activation of primary photosynthetic reactions in plant chloroplasts by reducing disulphide bridges in redox‐regulated enzymes. Of the two plastid TRX systems, the ferredoxin‐TRX system consists of ferredoxin‐thioredoxin reductase (FTR) and multiple TRXs, while the NADPH‐dependent thioredoxin reductase (NTRC) contains a complete TRX system in a single polypeptide. Using Arabidopsis plants overexpressing or lacking a functional NTRC we have investigated the redundancy and interaction between the NTRC and Fd‐TRX systems in regulation of photosynthesis in vivo. Overexpression of NTRC raised the COfixation rate and lowered non‐photochemical quenching and acceptor side limitation of PSI in low light conditions by enhancing the activation of chloroplast ATP synthase and TRX‐regulated enzymes in Calvin‐Benson cycle (CBC). Overexpression of NTRC with an inactivated NTR or TRX domain partly recovered the phenotype of knockout plants, suggesting crosstalk between the plastid TRX systems. NTRC interacted in planta with fructose‐1,6‐bisphosphatase, phosphoribulokinase and CFγ subunit of the ATP synthase as well as with several chloroplast TRXs. These findings indicate that NTRC‐mediated regulation of the CBC and ATP synthesis occurs both directly and through interaction with the ferredoxin‐TRX system and is crucial when availability of light is limiting photosynthesis. This article is protected by copyright. All rights reserved.
DOI:10.1104/pp.17.00481URLPMID:28500266 [本文引用: 2]

Thiol-dependent redox regulation of enzyme activity plays a central role in the rapid acclimation of chloroplast metabolism to ever-fluctuating light availability. This regulatory mechanism relies on ferredoxin reduced by the photosynthetic electron transport chain, which fuels reducing power to thioredoxins (Trxs) via a ferredoxin-dependent Trx reductase. In addition, chloroplasts harbor an NADPH-dependent Trx reductase, which has a joint Trx domain at the carboxyl terminus, termed NTRC. Thus, a relevant issue concerning chloroplast function is to establish the relationship between these two redox systems and its impact on plant development. To address this issue, we generated Arabidopsis (Arabidopsis thaliana) mutants combining the deficiency of NTRC with those of Trxs f, which participate in metabolic redox regulation, and that of Trx x, which has antioxidant function. The ntrc-trxf1f2 and, to a lower extent, ntrc-trxx mutants showed severe growth-retarded phenotypes, decreased photosynthesis performance, and almost abolished light-dependent reduction of fructose-1,6-bisphosphatase. Moreover, the combined deficiency of both redox systems provokes aberrant chloroplast ultrastructure. Remarkably, both the ntrc-trxf1f2 and ntrc-trxx mutants showed high mortality at the seedling stage, which was overcome by the addition of an exogenous carbon source. Based on these results, we propose that NTRC plays a pivotal role in chloroplast redox regulation, being necessary for the activity of diverse Trxs with unrelated functions. The interaction between the two thiol redox systems is indispensable to sustain photosynthesis performed by cotyledons chloroplasts, which is essential for early plant development.
.
DOI:10.1111/tpj.13049URLPMID:26468055 [本文引用: 2]

Summary Thioredoxins (Trxs) regulate the activity of various chloroplastic proteins in a light-dependent manner. Five types of Trxs function in different physiological processes in the chloroplast of Arabidopsis thaliana . Previous in vitro experiments have suggested that the f -type Trx (Trx f ) is the main redox regulator of chloroplast enzymes, including Calvin cycle enzymes. To investigate the in vivo contribution of each Trx isoform to the redox regulatory system, we first quantified the protein concentration of each Trx isoform in the chloroplast stroma. The m -type Trx (Trx m ), which consists of four isoforms, was the most abundant type. Next, we analyzed several Arabidopsis Trx -m- deficient mutants to elucidate the physiological role of Trx m in vivo . Deficiency of Trx m impaired plant growth and decreased the CO2 assimilation rate. We also determined the redox state of Trx target enzymes to examine their photo-reduction, which is essential for enzyme activation. In the Trx- m -deficient mutants, the reduction level of fructose-1,6-bisphosphatase and sedoheptulose-1,7-bisphosphatase was lower than that in the wild type. Inconsistently with the historical view, our in vivo study suggested that Trx m plays a more important role than Trx f in the activation of Calvin cycle enzymes.
DOI:10.1371/journal.pone.0101470URLPMID:25007326 [本文引用: 1]

Photoautotrophic organisms efficiently regulate absorption of light energy to sustain photochemistry while promoting photoprotection. Photoprotection is achieved in part by triggering a series of dissipative processes termed non-photochemical quenching (NPQ), which depend on the re-organization of photosystem (PS) II supercomplexes in thylakoid membranes. Using atomic force microscopy, we characterized the structural attributes of grana thylakoids from Arabidopsis thaliana to correlate differences in PSII organization with the role of SOQ1, a recently discovered thylakoid protein that prevents formation of a slowly reversible NPQ state. We developed a statistical image analysis suite to discriminate disordered from crystalline particles and classify crystalline arrays according to their unit cell properties. Through detailed analysis of the local organization of PSII supercomplexes in ordered and disordered phases, we found evidence that interactions among light-harvesting antenna complexes are weakened in the absence of SOQ1, inducing protein rearrangements that favor larger separations between PSII complexes in the majority (disordered) phase and reshaping the PSII crystallization landscape. The features we observe are distinct from known protein rearrangements associated with NPQ, providing further support for a role of SOQ1 in a novel NPQ pathway. The particle clustering and unit cell methodology developed here is generalizable to multiple types of microscopy and will enable unbiased analysis and comparison of large data sets.
DOI:10.1093/mp/ssp011URLPMID:19825629 [本文引用: 1]

NADPH thioredoxin reductase C (NTRC) is a chloroplast enzyme able to conjugate NADPH thioredoxin reductase (NTR) and thioredoxin (TRX) activities for the efficient reduction of 2-Cys peroxiredoxin (2-Cys PRX). Because NADPH can be produced in chloroplasts during darkness, NTRC plays a key role for plant peroxide detoxification during the night. Here, it is shown that the quaternary structure of NTRC is highly dependent on its redox status. In vitro, most of the enzyme adopted an oligomeric state that disaggregated in dimers upon addition of NADPH, NADH, or DTT. Gel filtration and Western blot analysis of protein extracts from Arabidopsis chloroplast stroma showed that native NTRC forms aggregates, which are sensitive to NADPH and DTT, suggesting that the aggregation state might be a significant aspect of NTRC activity in vivo. Moreover, the enzyme is localized in clusters in Arabidopsis chloroplasts. NTRC triple and double mutants, A164G-V182E-R183F and A164G-R183F, replacing key residues of NADPH binding site, showed reduced activity but were still able to dimerize though with an increase in intermediary forms. Based on these results, we propose that the catalytically active form of NTRC is the dimer, which formation is induced by NADPH.
.
DOI:10.1093/mp/ssu032URLPMID:24658415 [本文引用: 1]
DOI:10.1073/pnas.1706003114URLPMID:29078290 [本文引用: 2]

Chloroplasts harbor a complex redox network formed by two systems, the FTR- thioredoxins (Trxs), which relies on photoreduced ferredoxin (Fd), and the NADPH-dependent Trx reductase C NTRC. Thus, an important issue in chloroplast biology is to establish the relationship between these redox pathways. Here we propose that the Fd-FTR-Trxs and NTRC redox systems are integrated via the redox balance of 2-Cys peroxiredoxins (Prxs), which therefore has a key role in chloroplast function. NTRC controls the redox balance of 2-Cys Prxs, which maintains the reducing capacity of the pool of chloroplast Trxs and, consequently, proper regulation of photosynthetic carbon assimilation enzymes. Therefore, redox regulation of chloroplast enzymes and hydrogen peroxide reduction are linked by the action of the NTRC-2-Cys Prxs system. Thiol-dependent redox regulation allows the rapid adaptation of chloroplast function to unpredictable changes in light intensity. Traditionally, it has been considered that chloroplast redox regulation relies on photosynthetically reduced ferredoxin (Fd), thioredoxins (Trxs), and an Fd-dependent Trx reductase (FTR), the Fd-FTR-Trxs system, which links redox regulation to light. More recently, a plastid-localized NADPH-dependent Trx reductase (NTR) with a joint Trx domain, termed NTRC, was identified. NTRC efficiently reduces 2-Cys peroxiredoxins (Prxs), thus having antioxidant function, but also participates in redox regulation of metabolic pathways previously established to be regulated by Trxs. Thus, the NTRC, 2-Cys Prxs, and Fd-FTR-Trxs redox systems may act concertedly, but the nature of the relationship between them is unknown. Here we show that decreased levels of 2-Cys Prxs suppress the phenotype of theArabidopsis thaliana ntrcKO mutant. The excess of oxidized 2-Cys Prxs in NTRC-deficient plants drains reducing power from chloroplast Trxs, which results in low efficiency of light energy utilization and impaired redox regulation of Calvin enson cycle enzymes. Moreover, the dramatic phenotype of thentrc-trxf1f2triple mutant, lacking NTRC andf-type Trxs, was also suppressed by decreased 2-Cys Prxs contents, as thentrc-trxf1f2-2cpmutant partially recovered the efficiency of light energy utilization and exhibited WT rate of CO2fixation and growth phenotype. The suppressor phenotype was not caused by compensatory effects of additional chloroplast antioxidant systems. It is proposed that the Fd-FTR-Trx and NTRC redox systems are linked by the redox balance of 2-Cys Prxs, which is crucial for chloroplast function.
.
DOI:10.1093/jxb/eru512URLPMID:4423512 [本文引用: 1]

This work shows the dominant effect of NADPH thioredoxin reductase C (NTRC) over sulfiredoxin on 2-Cys peroxiredoxin (2-Cys Prx) overoxidation, and uncovers an NTRC-independent, light-dependent component contributing to 2-Cys Prx overoxidation in Arabidopsis thaliana chloroplasts. Hydrogen peroxide is a harmful by-product of photosynthesis, which also has important signalling activity. Therefore, the level of hydrogen peroxide needs to be tightly controlled. Chloroplasts harbour different antioxidant systems including enzymes such as the 2-Cys peroxiredoxins (2-Cys Prxs). Under oxidizing conditions, 2-Cys Prxs are susceptible to inactivation by overoxidation of their peroxidatic cysteine, which is enzymatically reverted by sulfiredoxin (Srx). In chloroplasts, the redox status of 2-Cys Prxs is highly dependent on NADPH-thioredoxin reductase C (NTRC) and Srx; however, the relationship of these activities in determining the level of 2-Cys Prx overoxidation is unknown. Here we have addressed this question by a combination of genetic and biochemical approaches. An Arabidopsis thaliana double knockout mutant lacking NTRC and Srx shows a phenotype similar to the ntrc mutant, while the srx mutant resembles wild-type plants. The deficiency of NTRC causes reduced overoxidation of 2-Cys Prxs, whereas the deficiency of Srx has the opposite effect. Moreover, in vitro analyses show that the disulfide bond linking the resolving and peroxidatic cysteines protects the latter from overoxidation, thus explaining the dominant role of NTRC on the level of 2-Cys Prx overoxidation in vivo. The overoxidation of chloroplast 2-Cys Prxs shows no circadian oscillation, in agreement with the fact that neither the NTRC nor the SRX genes show circadian regulation of expression. Additionally, the low level of 2-Cys Prx overoxidation in the ntrc mutant is light dependent, suggesting that the redox status of 2-Cys Prxs in chloroplasts depends on light rather than the circadian clock.
.
DOI:10.1093/jxb/erq218URLPMID:20813724 [本文引用: 3]

Photosynthesis is a process that inevitably produces reactive oxygen species, such as hydrogen peroxide, which is reduced by chloroplast-localized detoxification mechanisms one of which involves 2-Cys peroxiredoxins (2-Cys Prxs). Arabidopsis chloroplasts contain two very similar 2-Cys Prxs (denoted A and B). These enzymes are reduced by two pathways: NADPH thioredoxin reductase C (NTRC), which uses NADPH as source of reducing power; and plastidial thioredoxins (Trxs) coupled to photosynthetically reduced ferredoxin of which Trx x is the most efficient reductant in vitro. With the aim of establishing the functional relationship between NTRC, Trx x, and 2-Cys Prxs in vivo, an Arabidopsis Trx x knock-out mutant has been identified and a double mutant (denoted delta 2cp) with < 5% of 2-Cys Prx content has been generated. The phenotypes of the three mutants, ntrc, trxx, and delta 2cp, were compared under standard growth conditions and in response to continuous light or prolonged darkness and oxidative stress. Though all mutants showed altered redox homeostasis, no difference was observed in response to oxidative stress treatment. Moreover, the redox status of the 2-Cys Prx was imbalanced in the ntrc mutant but not in the trxx mutant. These results show that NTRC is the most relevant pathway for chloroplast 2-Cys Prx reduction in vivo, but the antioxidant function of this system is not essential. The deficiency of NTRC caused a more severe phenotype than the deficiency of Trx x or 2-Cys Prxs as determined by growth, pigment content, CO2 fixation, and F-v/F-m, indicating additional functions of NTRC.
DOI:10.3389/fpls.2013.00390URLPMID:3797462 [本文引用: 1]

Plants display a remarkable diversity of thioredoxins (Trxs), reductases controlling the thiol redox status of proteins. The physiological function of many of them remains elusive, particularly for plastidial Trxs f and m, which are presumed based on biochemical data to regulate photosynthetic reactions and carbon metabolism. Recent reports revealed that Trxs f and m participate in vivo in the control of starch metabolism and cyclic photosynthetic electron transfer around photosystem I, respectively. To further delineate their in planta function, we compared the photosynthetic characteristics, the level and/or activity of various Trx targets and the responses to oxidative stress in transplastomic tobacco plants overexpressing either Trx f or Trx m. We found that plants overexpressing Trx m specifically exhibit altered growth, reduced chlorophyll content, impaired photosynthetic linear electron transfer and decreased pools of glutathione and ascorbate. In both transplastomic lines, activities of two enzymes involved in carbon metabolism, NADP-malate dehydrogenase and NADP-glyceraldehyde-3-phosphate dehydrogenase are markedly and similarly altered. In contrast, plants overexpressing Trx m specifically display increased capacity for methionine sulfoxide reductases, enzymes repairing damaged proteins by regenerating methionine from oxidized methionine. Finally, we also observed that transplastomic plants exhibit distinct responses when exposed to oxidative stress conditions generated by methyl viologen or exposure to high light combined with low temperature, the plants overexpressing Trx m being notably more tolerant than Wt and those overexpressing Trx f. Altogether, these data indicate that Trxs f and m fulfill distinct physiological functions. They prompt us to propose that the m type is involved in key processes linking photosynthetic activity, redox homeostasis and antioxidant mechanisms in the chloroplast.
DOI:10.3389/fpls.2013.00371URLPMID:3778395 [本文引用: 2]

The last decades of research brought substantial insights into tetrapyrrole biosynthetic pathway in photosynthetic organisms. Almost all genes have been identified and roles of seemingly all essential proteins, leading to the synthesis of heme, siroheme, phytochromobilin, and chlorophyll (Chl), have been characterized. Detailed studies revealed the existence of a complex network of transcriptional and post-translational control mechanisms for maintaining a well-adjusted tetrapyrrole biosynthesis during plant development and adequate responses to environmental changes. Among others one of the known post-translational modifications is regulation of enzyme activities by redox modulators. Thioredoxins and NADPH-dependent thioredoxin reductase C (NTRC) adjust the activity of tetrapyrrole synthesis to the redox status of plastids. Excessive excitation energy of Chls in both photosystems and accumulation of light-absorbing unbound tetrapyrrole intermediates generate reactive oxygen species, which interfere with the plastid redox poise. Recent reports highlight ferredoxin-thioredoxin and NTRC-dependent control of key steps in tetrapyrrole biosynthesis in plants. In this review we introduce the regulatory impact of these reductants on the stability and activity of enzymes involved in 5-aminolevulinic acid synthesis as well as in the Mg-branch of the tetrapyrrole biosynthetic pathway and we propose molecular mechanisms behind this redox control.
DOI:10.1016/j.bbagen.2015.01.023URLPMID:25676896 [本文引用: 3]

61Plant development is under the control of thiol-based mechanisms.61Regulation of cell redox homeostasis by GSH is essential for meristem functioning.61GRXs are particularly required for the reproductive development.61TRXs play numerous roles during the plant life cycle.61Thiol- and hormone-signaling pathways are intricately linked to carbon metabolism.
DOI:10.1111/pbi.12052URLPMID:23398733 [本文引用: 1]

Starch, the most abundant storage carbohydrate in plants, has been a major feedstock for first-generation biofuels. Growing fuel demands require, however, that the starch yields of energy crops be improved. Leaf starch is synthesised during the day and degraded at night to power nonphotosynthetic metabolism. Redox regulation has been associated with the coordination of the enzymes involved in starch metabolism, but neither the signals nor mechanisms that regulate this metabolism are entirely clear. In this work, the thioredoxin (Trx) f and m genes, which code for key enzymes in plastid redox regulation, were overexpressed from the plastid genome. Tobacco plants overexpressing Trx f, but not Trx m, showed an increase of up to 700% in leaf starch accumulation, accompanied by an increase in leaf sugars, specific leaf weight (SLW), and leaf biomass yield. To test the potential of these plants as a nonfood energy crop, tobacco leaves overexpressing Trx f were subjected to enzymatic hydrolysis, and around a 500% increase in the release of fermentable sugars was recorded. The results show that Trx f is a more effective regulator of photosynthetic carbon metabolism in planta than Trx m. The overexpression of Trx f might therefore provide a means of increasing the carbohydrate content of plants destined for use in biofuel production. It might also provide a means of improving the nutritional properties of staple food crops.
DOI:10.1093/jxb/err282URLPMID:21948853 [本文引用: 2]

Thioredoxins (Trxs) are ubiquitous disulphide reductases that play important roles in the redox regulation of many cellular processes. However, some redox-independent functions, such as chaperone activity, have also been attributed to Trxs in recent years. The focus of our study is on the putative chaperone function of the well-described plastid Trxs f and m. To that end, the cDNA of both Trxs, designated asNtTrxfandNtTrxm, was isolated fromNicotiana tabacumplants. It was found that bacterially expressed tobacco Trx f and Trx m, in addition to their disulphide reductase activity, possessed chaperone-like properties.In vitro, Trx f and Trx m could both facilitate the reactivation of the cysteine-free form of chemically denatured glucose-6 phosphate dehydrogenase (foldase chaperone activity) and prevent heat-induced malate dehydrogenase aggregation (holdase chaperone activity). Our results led us to infer that the disulphide reductase and foldase chaperone functions prevail when the proteins occur as monomers and the well-conserved non-active cysteine present in Trx f is critical for both functions. By contrast, the holdase chaperone activity of both Trxs depended on their oligomeric status: the proteins were functional only when they were associated with high molecular mass protein complexes. Because the oligomeric status of both Trxs was induced by salt and temperature, our data suggest that plastid Trxs could operate as molecular holdase chaperones upon oxidative stress, acting as a type of small stress protein.
DOI:10.3389/fpls.2013.00463URLPMID:3836485 [本文引用: 1]

The sessile nature of plants forces them to face an ever-changing environment instead of escape from hostile conditions as animals do. In order to overcome this survival challenge, a fine monitoring and controlling of the status of the photosynthetic electron transport chain and the general metabolism is vital for these organisms. Frequently, evolutionary plant adaptation has consisted in the appearance of multigenic families, comprising an array of enzymes, structural components, or sensing, and signaling elements, in numerous occasions with highly conserved primary sequences that sometimes make it difficult to discern between redundancy and specificity among the members of a same family. However, all this gene diversity is aimed to sort environment-derived plant signals to efficiently channel the external incoming information inducing a right physiological answer. Oxygenic photosynthesis is a powerful source of reactive oxygen species (ROS), molecules with a dual oxidative/signaling nature. In response to ROS, one of the most frequent post-translational modifications occurring in redox signaling proteins is the formation of disulfide bridges (from Cys oxidation). This review is focused on the role of plastid thioredoxins (pTRXs), proteins containing two Cys in their active site and largely known as part of the plant redox-signaling network. Several pTRXs types have been described so far, namely, TRX f, m, x, y, and z. In recent years, improvements in proteomic techniques and the study of loss-of-function mutants have enabled us to grasp the importance of TRXs for the plastid physiology. We will analyze the specific signaling function of each TRX type and discuss about the emerging role in non-photosynthetic plastids of these redox switchers.
DOI:10.1016/j.febslet.2015.02.033URLPMID:25747136 [本文引用: 2]

When exposed to saturating light conditions photosynthetic eukaryotes activate the xanthophyll cycle where the carotenoid violaxanthin is converted into zeaxanthin by the enzyme violaxanthin de-epoxidase (VDE). VDE protein sequence includes 13 cysteine residues, 12 of which are strongly conserved in both land plants and algae. Site directed mutagenesis of Arabidopsis thaliana VDE showed that all these 12 conserved cysteines have a major role in protein function and their mutation leads to a strong reduction of activity. VDE is also shown to be active in its completely oxidized form presenting six disulfide bonds. Redox titration showed that VDE activity is sensitive to variation in redox potential, suggesting the possibility that dithiol/disulfide exchange reactions may represent a mechanism for VDE regulation.
DOI:10.2307/3870056URLPMID:20417532 [本文引用: 1]

Abstract Chlorophyll captures and redirects light-energy and is thus essential for photosynthetic organisms. The demand for chlorophyll differs throughout the day and night and in response to changing light conditions. Moreover, the chlorophyll biosynthesis pathway is up to certain points shared between the different tetrapyrroles; chlorophyll, heme, siroheme and phytochromobilin, for which the cell has different requirements at different time points. Combined with the phototoxic properties of tetrapyrroles which, if not properly protected, can lead to formation of reactive oxygen species (ROS), the need for a strict regulation of the chlorophyll biosynthetic pathway is obvious. Here we describe the current knowledge on regulation of chlorophyll biosynthesis in plants by the chloroplast redox state with emphasis on the Mg-chelatase situated at the branch point between the heme and the chlorophyll pathway. We discuss the proposed role of the Mg-chelatase as a key regulator of the tetrapyrrole pathway by its effect on enzymes both up- and downstream in the pathway and we specifically describe how redox state might regulate the Mg-branch. Finally, we propose that a recently identified NADPH-dependent thioredoxin reductase (NTRC) could be involved in redox regulation or protection of chlorophyll biosynthetic enzymes and describe the possible modes of action by this enzyme. Copyright 2010 Elsevier Ltd. All rights reserved.
DOI:10.1016/j.bbabio.2015.07.012URLPMID:26235611 [本文引用: 1]

61Reduced DTT is required to maintain cyclic electron flow (CEF) in spinach thylakoid preparations.61The midpoint potential for activation/inactivation of CEF is approximately 61306mV.61Indicates redox modulation of thiol/disulfide couple for CEF plastoquinone reductase activity
DOI:10.1074/jbc.M110.108373URLPMID:2865344 [本文引用: 2]

Thioredoxins (Trxs) are ubiquitous enzymes catalyzing the reduction of disulfide bonds, thanks to a CXXC active site. Among their substrates, 2-Cys methionine sulfoxide reductases B (2-Cys MSRBs) reduce the R diastereoisomer of methionine sulfoxide (MetSO) and possess two redox-active Cys as follows: a catalytic Cys reducing MetSO and a resolving one, involved in disulfide bridge formation. The other MSRB type, 1-Cys MSRBs, possesses only the catalytic Cys, and their regeneration mechanisms by Trxs remain unclear. The plant plastidial Trx CDSP32 is able to provide 1-Cys MSRB with electrons. CDSP32 includes two Trx modules with one potential active site (219)CGPC(222) and three extra Cys. Here, we investigated the redox properties of recombinant Arabidopsis CDSP32 and delineated the
[本文引用: 3]
DOI:10.1111/j.1365-3040.2012.02549.xURLPMID:22646759 [本文引用: 1]

Chloroplast thioredoxin f (Trx f) is an important regulator of primary metabolic enzymes. However, genetic evidence for its physiological importance is largely lacking. To test the functional significance of Trx f in vivo, Arabidopsis mutants with insertions in the trx f1 gene were studied, showing a drastic decrease in Trx f leaf content. Knockout of Trx f1 led to strong attenuation in reductive light activation of ADP-glucose pyrophosphorylase (AGPase), the key enzyme of starch synthesis, in leaves during the day and in isolated chloroplasts, while sucrose-dependent redox activation of AGPase in darkened leaves was not affected. The decrease in light-activation of AGPase in leaves was accompanied by a decrease in starch accumulation, an increase in sucrose levels and a decrease in starch-to-sucrose ratio. Analysis of metabolite levels at the end of day shows that inhibition of starch synthesis was unlikely due to shortage of substrates or changes in allosteric effectors. Metabolite profiling by gas chromatography ass spectrometry pinpoints only a small number of metabolites affected, including sugars, organic acids and ethanolamine. Interestingly, metabolite data indicate carbon shortage in trx f1 mutant leaves at the end of night. Overall, results provide in planta evidence for the role played by Trx f in the light activation of AGPase and photosynthetic carbon partitioning in plants.
DOI:10.1016/j.molp.2016.11.012URL [本文引用: 1]
DOI:10.1104/pp.109.150250URL [本文引用: 1]

Several proteins of photosystem II (PSII) and its light-harvesting antenna (LHCII) are reversibly phosphorylated according to light quantity and quality. Nevertheless, the interdependence of protein phosphorylation, nonphotochemical quenching, and efficiency of electron transfer in the thylakoid membrane has remained elusive. These questions were addressed by investigating in parallel the wild type and the stn7, stn8, and stn7 stn8 kinase mutants of Arabidopsis (Arabidopsis thaliana), using the stn7 npq4, npq4, npq1, and pgr5 mutants as controls. Phosphorylation of PSII-LHCII proteins is strongly and dynamically regulated according to white light intensity. Yet, the changes in phosphorylation do not notably modify the relative excitation energy distribution between PSII and PSI, as typically occurs when phosphorylation is induced by "state 2" light that selectively excites PSII and induces the phosphorylation of both the PSII core and LHCII proteins. On the contrary, under low-light conditions, when excitation energy transfer from LHCII to reaction centers is efficient, the STN7-dependent LHCII protein phosphorylation guarantees a balanced distribution of excitation energy to both photosystems. The importance of this regulation diminishes at high light upon induction of thermal dissipation of excitation energy. Lack of the STN7 kinase, and thus the capacity for equal distribution of excitation energy to PSII and PSI, causes relative overexcitation of PSII under low light but not under high light, leading to disturbed maintenance of fluent electron flow under fluctuating light intensities. The physiological relevance of the STN7-dependent regulation is evidenced by severely stunted phenotypes of the stn7 and stn7 stn8 mutants under strongly fluctuating light conditions.
.
DOI:10.3389/fpls.2013.00389URLPMID:3792407 [本文引用: 3]

Plant chloroplasts have versatile thioredoxin systems including two thioredoxin reductases and multiple types of thioredoxins. Plastid-localized NADPH-dependent thioredoxin reductase (NTRC) contains both reductase (NTRd) and thioredoxin (TRXd) domains in a single polypeptide and forms homodimers. To study the action of NTRC and NTRC domains in vivo, we have complemented the ntrc knockout line of Arabidopsis with the wild type and full-length NTRC genes, in which 2-Cys motifs either in NTRd, or in TRXd were inactivated. The ntrc line was also transformed either with the truncated NTRd or TRXd alone. Overexpression of wild-type NTRC promoted plant growth by increasing leaf size and biomass yield of the rosettes. Complementation of the ntrc line with the full-length NTRC gene containing an active reductase but an inactive TRXd, or vice versa, recovered wild-type chloroplast phenotype and, partly, rosette biomass production, indicating that the NTRC domains are capable of interacting with other chloroplast thioredoxin systems. Overexpression of truncated NTRd or TRXd in ntrc background did not restore wild-type phenotype. Modeling of the three-dimensional structure of the NTRC dimer indicates extensive interactions between the NTR domains and the TRX domains further stabilize the dimeric structure. The long linker region between the NTRd and TRXd, however, allows flexibility for the position of the TRXd in the dimer. Supplementation of the TRXd in the NTRC homodimer model by free chloroplast thioredoxins indicated that TRXf is the most likely partner to interact with NTRC. We propose that overexpression of NTRC promotes plant biomass yield both directly by stimulation of chloroplast biosynthetic and protective pathways controlled by NTRC and indirectly via free chloroplast thioredoxins. Our data indicate that overexpression of chloroplast thiol redox-regulator has a potential to increase biofuel yield in plant and algal species suitable for sustainable bioenergy production.
.
DOI:10.1093/mp/ssu069URLPMID:24890758 [本文引用: 3]
DOI:10.1104/pp.113.228353URLPMID:24151299 [本文引用: 3]

Chloroplastic m-type thioredoxins (TRX m) are essential redox regulators in the light regulation of photosynthetic metabolism. However, recent genetic studies have revealed novel functions for TRX m in meristem development, chloroplast morphology, cyclic electron flow, and tetrapyrrole synthesis. The focus of this study is on the putative role of TRX m1, TRX m2, and TRX m4 in the biogenesis of the photosynthetic apparatus in Arabidopsis (Arabidopsis thaliana). To that end, we investigated the impact of single, double, and triple TRX m deficiency on chloroplast development and the accumulation of thylakoid protein complexes. Intriguingly, only inactivation of three TRX m genes led to pale-green leaves and specifically reduced stability of the photosystem II (PSII) complex, implying functional redundancy between three TRX m isoforms. In addition, plants silenced for three TRX m genes displayed elevated levels of reactive oxygen species, which in turn interrupted the transcription of photosynthesis-related nuclear genes but not the expression of chloroplast-encoded PSII core proteins. To dissect the function of TRX m in PSII biogenesis, we showed that TRX m1, TRX m2, and TRX m4 interact physically with minor PSII assembly intermediates as well as with PSII core subunits D1, D2, and CP47. Furthermore, silencing three TRX m genes disrupted the redox status of intermolecular disulfide bonds in PSII core proteins, most notably resulting in elevated accumulation of oxidized CP47 oligomers. Taken together, our results suggest an important role for TRX m1, TRX m2, and TRX m4 proteins in the biogenesis of PSII, and they appear to assist the assembly of CP47 into PSII.
.
DOI:10.1089/ars.2010.3558URLPMID:3061198 [本文引用: 1]

Abstract We have presented evidence that a homologue of vertebrate membrane protein vitamin K epoxide reductase (VKOR) is an important component of the protein disulfide bond-forming pathway in many bacteria. Bacterial VKOR appears to take the place of the nonhomologous DsbB found in Escherichia coli. We also determined the structure of a VKOR from a Cyanobacterium and showed that two or four conserved cysteines are required, according to different reductants for activity in an in vitro assay. Here we present evidence for the topologic arrangement in the cytoplasmic membrane of the VKOR from Mycobacterium tuberculosis (Mtb). The results show that Mtb VKOR is a membrane protein that spans the membrane 5 times with its N-terminus in the cytoplasm, C-terminus in the periplasm, and all four cysteines facing the periplasm. The essentiality of the four conserved cysteine residues has also been demonstrated in promoting disulfide bond formation in vivo and a mixed disulfide between a cysteine of DsbA of E. coli, and one of the cysteines (Cys(57)) of the VKOR homologue has been identified to be a likely intermediate in the disulfide bond-forming pathway. These studies may inform future resolution of issues surrounding the functioning of human VKOR.
DOI:10.1021/bi200058aURLPMID:21456578 [本文引用: 1]

Thioredoxin and thioredoxin reductase can regulate cell metabolism through redox regulation of disulfide bridges or through removal of H2O2. These two enzymatic functions are combined in NADPH-dependent thioredoxin reductase C (NTRC), which contains an N-terminal thioredoxin reductase domain fused with a C-terminal thioredoxin domain. Rice NTRC exists in different oligomeric states, depending on the absence or presence of its NADPH cofactor. It has been suggested that the different oligomeric states may have diverse activity. Thus, the redox status of the chloroplast could influence the oligomeric state of NTRC and thereby its activity. We have characterized the oligomeric states of NTRC from barley (Hordeum vulgare L.). This also includes a structural model of the tetrameric NTRC derived from cryo-electron microscopy and single-particle reconstruction. We conclude that the tetrameric NTRC is a dimeric arrangement of two NTRC homodimers. Unlike that of rice NTRC, the quaternary structure of barley NTRC co...
[本文引用: 2]
DOI:10.1073/pnas.1604101113URLPMID:27335455 [本文引用: 1]

The thiol-based redox regulation system is believed to adjust chloroplast functions in response to changes in light environments. A redox cascade via the ferredoxin-thioredoxin reductase (FTR)/thioredoxin (Trx) pathway has been traditionally considered to serve as a transmitter of light signals to target enzymes. However, emerging data indicate that chloroplasts have a complex redox network composed of diverse redox-mediator proteins and target enzymes. Despite extensive research addressing this system, two fundamental questions are still unresolved: How are redox pathways orchestrated within chloroplasts, and why are chloroplasts endowed with a complicated redox network? In this report, we show that NADPH-Trx reductase C (NTRC) is a key redox-mediator protein responsible for regulatory functions distinct from those of the classically known FTR/Trx system. Target screening and subsequent biochemical assays indicated that NTRC and the Trx family differentially recognize their target proteins. In addition, we found that NTRC is an electron donor to Trx-z, which is a key regulator of gene expression in chloroplasts. We further demonstrate that cooperative control of chloroplast functions via the FTR/Trx and NTRC pathways is essential for plant viability. Arabidopsis double mutants impaired in FTR and NTRC expression displayed lethal phenotypes under autotrophic growth conditions. This severe growth phenotype was related to a drastic loss of photosynthetic performance. These combined results provide an expanded map of the chloroplast redox network and its biological functions.
DOI:10.1042/bcj20161089URL [本文引用: 1]
.
DOI:10.1093/pcp/pcu066URLPMID:24850837 [本文引用: 1]

The thiol/disulfide redox network mediated by the thioredoxin (Trx) system in chloroplasts ensures light-responsive control of diverse crucial functions. Despite the suggested importance of this system, the working dynamics against changing light environments remains largely unknown. Thus, we directly assessed the in vivo redox behavior of chloroplast Trx-targeted thiol enzymes in Arabidopsis thaliana. In a time-course analysis throughout a day period that was artificially mimicked to natural light conditions, thiol enzymes showed a light-dependent shift in redox state, but the patterns were distinct among thiol enzymes. Notably, the ATP synthase CF1 subunit was rapidly reduced even under low-light conditions, whereas the stromal thiol enzymes fructose 1,6-bisphosphatase, sedoheptulose 1,7-bisphosphatase, and NADP-malate dehydrogenase were gradually reduced/re-oxidized along with the increase/decrease in light intensity. Photo-reduction of thiol enzymes was suppressed by the impairment of photosynthetic linear electron transport using DCMU and 2,5-dibromo-3-methyl-6-isopropyl-p-benzoquinone, but sensitivity to the impairment was uneven between CF1 and other stromal thiol enzymes. These different dependencies of photo-reduction on electron transport, rather than the redox state of Trx and the circadian clock, could readily explain the distinct diurnal redox behaviors of thiol enzymes. In addition, our results indicate that the cyclic electron transport around PSI is also involved in redox regulation of some thiol enzymes. Based on these findings, we propose an in vivo working model of the redox regulation system in chloroplasts.
植物硫氧还蛋白系统
1
2010
... 巯基基团氧化还原状态的变化(二硫键-巯基)是生物体内普遍存在的现象.在很多情况下, 二硫键的打开和闭合具有调节功能(
拟南芥叶绿体中DEG蛋白酶功能的研究进展
1
2009
... Trx系统对类囊体腔蛋白的氧化还原调控主要体现在植物PSII的装配以及对不同光强的适应.例如, PSII的外周蛋白PsbO的2个亚基(PsbO1和PsbO2)通过分子间二硫键与PSII反应中心蛋白共价连接.在PSII装配过程中, PsbO必须及时与PSII反应中心形成分子间二硫键, 如果长时间处于还原态, 则容易被蛋白酶降解(
A structural basis for the pH-dependent xanthophyll cycle in Arabidopsis thaliana
1
2009
... 依赖于叶黄素循环的热耗散被认为是植物光合机构免受过剩光能伤害的主要机制(
Plastidial thioredoxin z interacts with two fructokinase-like proteins in a thiol-dependent manner: evidence for an essential role in chloroplast development in Arabidopsis and Nicotiana benthamiana
1
2010
... Trx蛋白表面电荷分布及氧化还原电位(Em)的差异决定了它们的生化特性不同(
Evolutionary development of redox regulation in chloroplasts
1
2014
... 硫氧还蛋白(Thioredoxin, Trx)是一类保守的多功能酸性小蛋白, 广泛存在于植物、细菌、酵母和动物中, 分子量约为12 kDa (
Ferredoxin: thioredoxin reductase (FTR) links the regulation of oxygenic photosynthesis to deeply rooted bacteria
2
2013
... 植物含有2种类型的Trx系统, 分别由FTR和NTR扮演TR的角色.叶绿体Trx由FTR和NTRC两种TR还原, 是TR的下游电子受体.在拟南芥中, FTR是一种异二聚体铁-硫蛋白, 由1个催化亚基和1个可变亚基组成, 分别由2个单拷贝的基因编码(
... 在叶绿体中, FTR和NTRC并非两个相互独立的途径, 而是存在部分功能交叉.FTR并非在所有光能自养生物中都存在, 在某种程度上, 其功能可被NTRC所代替(
A comprehensive study of thiol reduction gene expression under stress conditions in Ara- bidopsis thaliana
2
2015
... 巯基基团氧化还原状态的变化(二硫键-巯基)是生物体内普遍存在的现象.在很多情况下, 二硫键的打开和闭合具有调节功能(
... Trx的靶点蛋白在整个叶绿体的亚细胞器结构中都有分布(
Control of Arabidopsis meristem development by thioredoxin-dependent regulation of intercellular transport
1
2009
... Trx蛋白表面电荷分布及氧化还原电位(Em)的差异决定了它们的生化特性不同(
Molecular recognition in the interaction of chloroplast 2-Cys peroxiredoxin with NADPH-thioredoxin reductase C (NTRC) and thioredoxin x
1
2014
... Trx蛋白表面电荷分布及氧化还原电位(Em)的差异决定了它们的生化特性不同(
High light-dependent phosphorylation of photosystem II inner antenna CP29 in monocots is STN7 independent and enhances nonphotochemical quenching
2
2015
... 叶绿体通过维持氧化还原平衡来优化PSI与PSII之间的能量分配.激酶STN7参与催化光捕获复合体LHCII的可逆磷酸化, 是控制低光照下PSI与PSII之间能量分配的重要机制.STN7激酶是1个跨类囊体膜蛋白, 催化位点位于叶绿体基质侧, 氧化还原活性位点在类囊体腔(
... 的Qo位点(
New insights into the reduction systems of plastidial thioredoxins point out the unique properties of thioredoxin z from Arabidopsis
1
2012
... Trx系统在维持叶绿体整体的氧化还原平衡中发挥重要作用(
Redox meets protein trafficking
1
2015
... 巯基基团氧化还原状态的变化(二硫键-巯基)是生物体内普遍存在的现象.在很多情况下, 二硫键的打开和闭合具有调节功能(
A thioredoxin-like/ β-propeller protein maintains the efficiency of light harvesting in Arabidopsis
2
2013
... Trx蛋白表面电荷分布及氧化还原电位(Em)的差异决定了它们的生化特性不同(
... HCF164参与PSI和Cyt b6f复合体的组装, 而淬灭抑制子1 (SOQ1)与PSII功能相关(
The path to thioredoxin and redox regulation in chloroplasts
1
2016
... 硫氧还蛋白(Thioredoxin, Trx)是一类保守的多功能酸性小蛋白, 广泛存在于植物、细菌、酵母和动物中, 分子量约为12 kDa (
Fifty years in the thioredoxin field and a bountiful harvest
1
2012
... 硫氧还蛋白(Thioredoxin, Trx)是一类保守的多功能酸性小蛋白, 广泛存在于植物、细菌、酵母和动物中, 分子量约为12 kDa (
A novel extended family of stromal thioredoxins
1
2009
... Trx蛋白表面电荷分布及氧化还原电位(Em)的差异决定了它们的生化特性不同(
Multi-level regulation of the chloroplast ATP synthase: the chloroplast NADPH thioredoxin reductase C (NTRC) is required for redox modulation specifically under low irradiance
1
2016
... Trx系统在叶绿体光合作用和胁迫应激调控中表现出多点交叉及调控循环网络的特征(
The function of the NADPH thioredoxin reductase C-2-Cys peroxiredoxin system in plastid redox regulation and signaling
1
2012
... 巯基基团氧化还原状态的变化(二硫键-巯基)是生物体内普遍存在的现象.在很多情况下, 二硫键的打开和闭合具有调节功能(
Thioredoxin reductase type C (NTRC) orchestrates enhanced thermotolerance to Arabidopsis by its redox-dependent holdase chaperone function
2
2013
... 巯基基团氧化还原状态的变化(二硫键-巯基)是生物体内普遍存在的现象.在很多情况下, 二硫键的打开和闭合具有调节功能(
... 叶绿体Trx基因的过表达研究显示, Trx对提高植物适应性有实际应用意义.过表达Trx f的转基因烟草(Nicotiana tabacum)株系表现出生物量和淀粉含量显著增加(
Chloroplastic thioredoxin-f and thioredoxin-m1/4 play important roles in brassinosteroids-induced changes in CO2 assimilation and cellular redox homeostasis in tomato
1
2014
... Trx系统在维持叶绿体整体的氧化还原平衡中发挥重要作用(
Biochemical properties of poplar thioredoxin z
2
2011
... Trx蛋白表面电荷分布及氧化还原电位(Em)的差异决定了它们的生化特性不同(
... )及2种非典型性WCRKC型蛋白(
Post-translational control of protein function by disulfide bond cleavage
1
2013
... 巯基基团氧化还原状态的变化(二硫键-巯基)是生物体内普遍存在的现象.在很多情况下, 二硫键的打开和闭合具有调节功能(
Thioredoxin m4 controls photosynthetic alternative electron pathways in Arabidopsis
1
2013
... Trx蛋白表面电荷分布及氧化还原电位(Em)的差异决定了它们的生化特性不同(
Cysteine-based redox regulation and signaling in plants
1
2013
... 硫氧还蛋白(Thioredoxin, Trx)是一类保守的多功能酸性小蛋白, 广泛存在于植物、细菌、酵母和动物中, 分子量约为12 kDa (
Thioredoxin and NADPH- dependent thioredoxin reductase C regulation of tetrapyrrole biosynthesis
1
2017
... 在叶绿体所含5种Trx中, 只有Trx f来源于真核生物, 其它4种均属于原核起源(
A small family of chloroplast atypical thioredoxins
1
2009
... Trx蛋白表面电荷分布及氧化还原电位(Em)的差异决定了它们的生化特性不同(
A chloroplast light-regulated oxidative sensor for moderate light intensity in Arabidopsis
2
2012
... Trx蛋白表面电荷分布及氧化还原电位(Em)的差异决定了它们的生化特性不同(
... ).ACHT1蛋白在光照下处于还原态, 可还原2-Cys Prx, 但电子供体未被鉴定(
Redox regulation of PEP activity during seedling establishment in Arabidopsis tha- liana
2
2018
... 拟南芥(Arabidopsis thaliana)基因组编码20个Trx异构体.根据氨基酸序列的不同, Trx可分为7个亚家族: h、f、m、z、x、y和o型(
... Trx蛋白表面电荷分布及氧化还原电位(Em)的差异决定了它们的生化特性不同(
Novel regulators in photosynthetic redox control of plant metabolism and gene expression
1
2011
... 巯基基团氧化还原状态的变化(二硫键-巯基)是生物体内普遍存在的现象.在很多情况下, 二硫键的打开和闭合具有调节功能(
Inhibition of bacterial disulfide bond formation by the anticoagulant warfarin
1
2010
... LTO1 (Lumen Thiol Oxidoreductase 1)是1个类囊体膜蛋白, 由2个结构域组成.N端结构域是1个与哺乳动物维生素K还原酶同源的VKOR结构域, 与细菌DsbB功能类似; 另一个是Trx类似结构域, 具有与细菌巯基氧化还原酶蛋白DsbA同源的Trx类似序列(
ACHT4-driven oxidation of APS1 attenuates starch synthesis under low light intensity in Arabidopsis plants
2
2015
... Trx蛋白表面电荷分布及氧化还原电位(Em)的差异决定了它们的生化特性不同(
... ).ACHT4除了可以还原2-Cys Prx外, 还直接为腺苷二磷酸葡萄糖焦磷酸化酶(ADP-glucose pyrophosphorylase, AGPase)的小亚基ASP1提供电子(
A protein oxidase catalysing disulfide bond formation is localized to the chloroplast thylakoids
1
2011
... LTO1 (Lumen Thiol Oxidoreductase 1)是1个类囊体膜蛋白, 由2个结构域组成.N端结构域是1个与哺乳动物维生素K还原酶同源的VKOR结构域, 与细菌DsbB功能类似; 另一个是Trx类似结构域, 具有与细菌巯基氧化还原酶蛋白DsbA同源的Trx类似序列(
Identification of key residues for pH dependent activation of violaxanthin de-epoxidase from Arabidopsis thaliana
2
2012
... Trx系统对类囊体腔蛋白的氧化还原调控主要体现在植物PSII的装配以及对不同光强的适应.例如, PSII的外周蛋白PsbO的2个亚基(PsbO1和PsbO2)通过分子间二硫键与PSII反应中心蛋白共价连接.在PSII装配过程中, PsbO必须及时与PSII反应中心形成分子间二硫键, 如果长时间处于还原态, 则容易被蛋白酶降解(
... 依赖于叶黄素循环的热耗散被认为是植物光合机构免受过剩光能伤害的主要机制(
A bimodular oxidoreductase mediates the specific reduction of phylloquinone (vitamin K1) in chloroplasts
1
2010
... LTO1与PSII亚基PsbO互作.在体外, LTO1的Trx类似结构域能够向PsbO中导入1个二硫键.PsbO巯基的氧化还原状态对其稳定性和PSII的装配起决定性作用.由此可见, lto1突变体中PSII的累积受损是由于PsbO与PSII之间的二硫键未能及时形成引起.但LTO1是否可以协助PsbO转入类囊体腔还需实验验证.目前, 普遍认为叶绿醌可能是LTO1依赖的二硫键形成通路的电子受体(
Metabolic control of redox and redox control of metabolism in plants
2
2014
... 拟南芥(Arabidopsis thaliana)基因组编码20个Trx异构体.根据氨基酸序列的不同, Trx可分为7个亚家族: h、f、m、z、x、y和o型(
... 巯基基团氧化还原状态的变化(二硫键-巯基)是生物体内普遍存在的现象.在很多情况下, 二硫键的打开和闭合具有调节功能(
Biodiversity of NPQ
2
2015
... Trx系统对类囊体腔蛋白的氧化还原调控主要体现在植物PSII的装配以及对不同光强的适应.例如, PSII的外周蛋白PsbO的2个亚基(PsbO1和PsbO2)通过分子间二硫键与PSII反应中心蛋白共价连接.在PSII装配过程中, PsbO必须及时与PSII反应中心形成分子间二硫键, 如果长时间处于还原态, 则容易被蛋白酶降解(
... 当入射光强超过一定限度时, 光合作用会达到一个饱和点.超过饱和点的入射光强在光合膜上引起多余的能量吸收而使叶绿素分子产生过多的激发态, 如不能通过转化成化学能及时将能量传递出去, 则会传递给周围的氧气, 产生活性氧(ROS)自由基, 而ROS会氧化光合膜, 造成光合膜被破坏, 反而抑制光合作用(
Dithiol disulphide exchange in redox regulation of chloroplast enzymes in response to evolutionary and structural constraints
1
2017
... 巯基基团氧化还原状态的变化(二硫键-巯基)是生物体内普遍存在的现象.在很多情况下, 二硫键的打开和闭合具有调节功能(
Chloroplast FBPase and SBPase are thioredoxin-linked enzymes with similar architecture but different evolutionary histories
2
2016
... Calvin-Benson循环中关键酶的光诱导活化是叶绿体Trx系统的标志性作用.Trx缺失会对酶活性产生直接影响.Trx系统可激活甘油醛-3-磷酸脱氢酶(glyce raldehyde-3-phosphate dehydrogenase, GA- PDH) (
... )、景天庚酮糖-二磷酸酶(sedoheptulose- 1,7-bisphosphatase, SBPase) (
Thioredoxin targets of the plant chloroplast lumen and their implications for plastid function
2
2010
... 巯基基团氧化还原状态的变化(二硫键-巯基)是生物体内普遍存在的现象.在很多情况下, 二硫键的打开和闭合具有调节功能(
... Trx的靶点蛋白在整个叶绿体的亚细胞器结构中都有分布(
Violaxanthin de-epo- xidase disulphides and their role in activity and thermal stability
1
2015
... 依赖于叶黄素循环的热耗散被认为是植物光合机构免受过剩光能伤害的主要机制(
PGRL1 is the elusive ferredoxin-plastoquinone reductase in photosynthetic cyclic electron flow
1
2013
... Trx系统在叶绿体光合作用和胁迫应激调控中表现出多点交叉及调控循环网络的特征(
The chloroplast ATP synthase features the characteristic redox regulation machinery
1
2013
... Trx系统在叶绿体光合作用和胁迫应激调控中表现出多点交叉及调控循环网络的特征(
Structural and evolutionary aspects of thioredoxin reductases in photosynthetic organisms
1
2009
... 硫氧还蛋白(Thioredoxin, Trx)是一类保守的多功能酸性小蛋白, 广泛存在于植物、细菌、酵母和动物中, 分子量约为12 kDa (
Understanding the roles of the thylakoid lumen in photosynthesis regulation
1
2013
... 植物类囊体腔是光合电子传递和跨膜质子梯度形成的场所(
Redox regulation in the thylakoid lumen
2
2016
... 巯基基团氧化还原状态的变化(二硫键-巯基)是生物体内普遍存在的现象.在很多情况下, 二硫键的打开和闭合具有调节功能(
... 植物类囊体腔起源于细菌周质.在叶绿体基质和类囊体腔之间, 人们发现了类似细菌周质的巯基代谢通路(
Lumen Thiol Oxidoreductase 1, a disulfide bond- forming catalyst, is required for the assembly of photosystem II in Arabidopsis
2
2011
... Trx蛋白表面电荷分布及氧化还原电位(Em)的差异决定了它们的生化特性不同(
... LTO1 (Lumen Thiol Oxidoreductase 1)是1个类囊体膜蛋白, 由2个结构域组成.N端结构域是1个与哺乳动物维生素K还原酶同源的VKOR结构域, 与细菌DsbB功能类似; 另一个是Trx类似结构域, 具有与细菌巯基氧化还原酶蛋白DsbA同源的Trx类似序列(
Operation of trans-thylakoid thiol-metabolizing pathways in photosynthesis
2
2013
... 巯基基团氧化还原状态的变化(二硫键-巯基)是生物体内普遍存在的现象.在很多情况下, 二硫键的打开和闭合具有调节功能(
... LTO1 (Lumen Thiol Oxidoreductase 1)是1个类囊体膜蛋白, 由2个结构域组成.N端结构域是1个与哺乳动物维生素K还原酶同源的VKOR结构域, 与细菌DsbB功能类似; 另一个是Trx类似结构域, 具有与细菌巯基氧化还原酶蛋白DsbA同源的Trx类似序列(
Oxidative folding in chloroplasts
1
2013
... LTO1可以催化PsbO二硫键的形成, 把类囊体腔蛋白转运与PSII装配紧密联系起来.刚转运至类囊体膜的PsbO, 由于处于过渡态很容易被蛋白酶解(
Overexpression of chloroplast-localized NAD- PH-dependent thioredoxin reductase C (NTRC) enhances tolerance to photo-oxidative and drought stresses in Ara- bidopsis thaliana
1
2017
... 叶绿体Trx基因的过表达研究显示, Trx对提高植物适应性有实际应用意义.过表达Trx f的转基因烟草(Nicotiana tabacum)株系表现出生物量和淀粉含量显著增加(
NADPH thioredoxin reductase C is localized in plastids of photosynthetic and nonphotosynthetic tissues and is involved in lateral root formation in Arabidopsis
1
2012
... 在叶绿体中, FTR和NTRC并非两个相互独立的途径, 而是存在部分功能交叉.FTR并非在所有光能自养生物中都存在, 在某种程度上, 其功能可被NTRC所代替(
NADPH thioredoxin reductase C controls the redox status of chloroplast 2-Cys peroxiredoxins in Arabidopsis thalia- na
1
2009
... 巯基基团氧化还原状态的变化(二硫键-巯基)是生物体内普遍存在的现象.在很多情况下, 二硫键的打开和闭合具有调节功能(
Mechanisms and dynamics in the thiol/disulfide redox regulatory network: transmitters, sensors and targets
1
2012
... 硫氧还蛋白(Thioredoxin, Trx)是一类保守的多功能酸性小蛋白, 广泛存在于植物、细菌、酵母和动物中, 分子量约为12 kDa (
Involvement of thioredoxin y2 in the preservation of leaf methionine sulfoxide reductase capacity and growth under high light
1
2013
... Trx蛋白表面电荷分布及氧化还原电位(Em)的差异决定了它们的生化特性不同(
Chlo- roplast NADPH-thioredoxin reductase interacts with photoperiodic development in Arabidopsis
1
2009
... Trx系统可以响应不同的光照条件以调节FBP- ase活性和Calvin-Benson循环.拟南芥ntrc突变体生长发育延迟, 叶片呈灰绿色, 并且在短日照下表型更加明显, 暗示了NTRC在光周期调控中的重要作用(
Disulphide proteomes and interactions with thioredoxin on the track towards understanding redox regulation in chloroplasts and cyanobacteria
2
2009
... 硫氧还蛋白(Thioredoxin, Trx)是一类保守的多功能酸性小蛋白, 广泛存在于植物、细菌、酵母和动物中, 分子量约为12 kDa (
... Trx系统对类囊体腔蛋白的氧化还原调控主要体现在植物PSII的装配以及对不同光强的适应.例如, PSII的外周蛋白PsbO的2个亚基(PsbO1和PsbO2)通过分子间二硫键与PSII反应中心蛋白共价连接.在PSII装配过程中, PsbO必须及时与PSII反应中心形成分子间二硫键, 如果长时间处于还原态, 则容易被蛋白酶降解(
The disulfide proteome and other reactive cysteine proteomes: analysis and functional significance
1
2011
... 植物类囊体腔起源于细菌周质.在叶绿体基质和类囊体腔之间, 人们发现了类似细菌周质的巯基代谢通路(
A chloroplast membrane protein LTO1/AtVKOR involving in redox regulation and ROS homeostasis
1
2013
... LTO1与PSII亚基PsbO互作.在体外, LTO1的Trx类似结构域能够向PsbO中导入1个二硫键.PsbO巯基的氧化还原状态对其稳定性和PSII的装配起决定性作用.由此可见, lto1突变体中PSII的累积受损是由于PsbO与PSII之间的二硫键未能及时形成引起.但LTO1是否可以协助PsbO转入类囊体腔还需实验验证.目前, 普遍认为叶绿醌可能是LTO1依赖的二硫键形成通路的电子受体(
Thioredoxin redox regulates ATPase activity of magnesium chelatase CHLI subunit and modulates redox-mediated signaling in tetrapyrrole biosynthesis and homeostasis of reactive oxygen species in pea plants
1
2012
... Trx系统在叶绿体光合作用和胁迫应激调控中表现出多点交叉及调控循环网络的特征(
Thioredoxin and glutaredoxin systems in plants: molecular mechanisms, crosstalks, and functional significance
1
2012
... 拟南芥(Arabidopsis thaliana)基因组编码20个Trx异构体.根据氨基酸序列的不同, Trx可分为7个亚家族: h、f、m、z、x、y和o型(
Thioredoxins and glutaredoxins: unifying elements in redox biology
1
2009
... 硫氧还蛋白(Thioredoxin, Trx)是一类保守的多功能酸性小蛋白, 广泛存在于植物、细菌、酵母和动物中, 分子量约为12 kDa (
NTRC links built-in thioredoxin to light and sucrose in regulating starch synthesis in chloroplasts and amyloplasts
1
2009
... Calvin-Benson循环中关键酶的光诱导活化是叶绿体Trx系统的标志性作用.Trx缺失会对酶活性产生直接影响.Trx系统可激活甘油醛-3-磷酸脱氢酶(glyce raldehyde-3-phosphate dehydrogenase, GA- PDH) (
Redox regulation of the Calvin-Benson cycle: something old, something new
1
2013
... 硫氧还蛋白(Thioredoxin, Trx)是一类保守的多功能酸性小蛋白, 广泛存在于植物、细菌、酵母和动物中, 分子量约为12 kDa (
Thioredoxin targets in plants: the first 30 years
1
2009
... 硫氧还蛋白(Thioredoxin, Trx)是一类保守的多功能酸性小蛋白, 广泛存在于植物、细菌、酵母和动物中, 分子量约为12 kDa (
CcdA is a thylakoid membrane protein required for the transfer of reducing equivalents from stroma to thylakoid lumen in the higher plant chloroplast
4
2010
... Trx蛋白表面电荷分布及氧化还原电位(Em)的差异决定了它们的生化特性不同(
... 植物类囊体腔是光合电子传递和跨膜质子梯度形成的场所(
... 然而, 在类囊体腔中并没有发现可溶性Trx.越来越多的研究表明, 还原势是从叶绿体基质转入到类囊体腔.高等植物叶绿体具有一套跨类囊体膜从基质侧到类囊体腔侧的还原势传递系统.相比细菌跨质膜DsbA-C/G-D氧化还原通路参与跨膜蛋白转运, 类囊体腔的这套系统则由LTO1、HCF164/SOQ1和CCDA组成, 参与跨膜还原势的传递.HCF164的拓扑学结构特征、氧化还原活性及电子势表明, 其具有从叶绿体基质向类囊体腔传递还原电子的能力(
... 拟南芥ccda突变体表现出与hcf164突变体类似的表型, 原因是它们都不能合成Cyt b6f复合体.蛋白序列分析显示, 植物CCDA与一个原核生物中巯基二硫键转运子蛋白同源.CCDA属于类囊体膜蛋白, 含有6个跨膜区域, 可被Trx m还原, 作为介导因子参与还原势从基质到类囊体腔的传递(
Type- f thioredoxins have a role in the short-term activation of carbon metabolism and their loss affects growth under short-day conditions in Arabidopsis thaliana
1
2016
... Trx系统可以响应不同的光照条件以调节FBP- ase活性和Calvin-Benson循环.拟南芥ntrc突变体生长发育延迟, 叶片呈灰绿色, 并且在短日照下表型更加明显, 暗示了NTRC在光周期调控中的重要作用(
The chloroplast NADPH thioredoxin reductase C, NTRC, controls non-photochemical quenching of light energy and photosynthetic electron transport in Arabidopsis
1
2016
... Trx系统在叶绿体光合作用和胁迫应激调控中表现出多点交叉及调控循环网络的特征(
Redox regulation of chloroplastic glucose-6-phosphate dehydrogenase: a new role for f-type thioredoxin
2
2009
... Trx蛋白表面电荷分布及氧化还原电位(Em)的差异决定了它们的生化特性不同(
... Calvin-Benson循环中关键酶的光诱导活化是叶绿体Trx系统的标志性作用.Trx缺失会对酶活性产生直接影响.Trx系统可激活甘油醛-3-磷酸脱氢酶(glyce raldehyde-3-phosphate dehydrogenase, GA- PDH) (
Thioredoxin-dependent regulatory networks in chloroplasts under fluctuating light conditions
1
2014
... Trx系统对类囊体腔蛋白的氧化还原调控主要体现在植物PSII的装配以及对不同光强的适应.例如, PSII的外周蛋白PsbO的2个亚基(PsbO1和PsbO2)通过分子间二硫键与PSII反应中心蛋白共价连接.在PSII装配过程中, PsbO必须及时与PSII反应中心形成分子间二硫键, 如果长时间处于还原态, 则容易被蛋白酶降解(
Chloroplast thioredoxin systems: prospects for improving photosynthesis
2
2017
... Calvin-Benson循环中关键酶的光诱导活化是叶绿体Trx系统的标志性作用.Trx缺失会对酶活性产生直接影响.Trx系统可激活甘油醛-3-磷酸脱氢酶(glyce raldehyde-3-phosphate dehydrogenase, GA- PDH) (
... )、磷酸核糖激酶(phosphoribulokinase, PRK) (
Crosstalk between chloroplast thioredoxin systems in regulation of photosynthesis
4
2016
... 巯基基团氧化还原状态的变化(二硫键-巯基)是生物体内普遍存在的现象.在很多情况下, 二硫键的打开和闭合具有调节功能(
... Trx系统在维持叶绿体整体的氧化还原平衡中发挥重要作用(
... 在叶绿体中, FTR和NTRC并非两个相互独立的途径, 而是存在部分功能交叉.FTR并非在所有光能自养生物中都存在, 在某种程度上, 其功能可被NTRC所代替(
... 由于不同Trx以异构体的形式存在, 因此需构建多重突变体来研究它们之间的相互作用, 如trx f1/f2双突变体和trx m1/m2/m4三突变体.这些研究进一步丰富了Trx系统在光系统功能及光合作用相关酶类的活性调节中的作用.拟南芥trx m1和trx m2单突变体以及trx m1/m2双突变体在正常光照下并无表型差异, 只有在Trx m4基因进一步突变或沉默后才表现出生长迟缓的表型, trx m1/m2/m4三突变体光合能力下降50% (
NADPH thioredoxin reductase C and thioredoxins act concertedly in seedling development
2
2017
... 拟南芥(Arabidopsis thaliana)基因组编码20个Trx异构体.根据氨基酸序列的不同, Trx可分为7个亚家族: h、f、m、z、x、y和o型(
... Trx系统在维持叶绿体整体的氧化还原平衡中发挥重要作用(
Chloroplastic thioredoxin m functions as a major regulator of Calvin cycle enzymes during photosynthesis in vivo
2
2015
... 在叶绿体所含5种Trx中, 只有Trx f来源于真核生物, 其它4种均属于原核起源(
... Trx蛋白表面电荷分布及氧化还原电位(Em)的差异决定了它们的生化特性不同(
Atomic force microscopy of photosystem II and its unit cell clustering quantitatively delineate the mesoscale variability in Arabidopsis thylakoids
1
2014
... HCF164参与PSI和Cyt b6f复合体的组装, 而淬灭抑制子1 (SOQ1)与PSII功能相关(
The quaternary structure of NADPH thioredoxin reductase C is redox-sensitive
1
2009
... 植物含有2种类型的Trx系统, 分别由FTR和NTR扮演TR的角色.叶绿体Trx由FTR和NTRC两种TR还原, 是TR的下游电子受体.在拟南芥中, FTR是一种异二聚体铁-硫蛋白, 由1个催化亚基和1个可变亚基组成, 分别由2个单拷贝的基因编码(
NADPH thioredoxin reductase C is involved in redox regulation of the Mg-chelatase I subunit in Arabidopsis thaliana chloroplasts
1
2014
... 巯基基团氧化还原状态的变化(二硫键-巯基)是生物体内普遍存在的现象.在很多情况下, 二硫键的打开和闭合具有调节功能(
NTRC-dependent redox balance of 2-Cys peroxiredoxins is needed for optimal function of the photosynthetic apparatus
2
2017
... Trx系统在维持叶绿体整体的氧化还原平衡中发挥重要作用(
... ), 后者又进一步影响叶绿体内某些酶类的氧化还原状态(
The contribution of NADPH thioredoxin reductase C (NTRC) and sulfiredoxin to 2-Cys peroxiredoxin overoxidation in Arabidopsis thaliana chloroplasts
1
2015
... 在叶绿体中, FTR和NTRC并非两个相互独立的途径, 而是存在部分功能交叉.FTR并非在所有光能自养生物中都存在, 在某种程度上, 其功能可被NTRC所代替(
Functional analysis of the pathways for 2-Cys peroxiredoxin reduction in Arabidopsis thaliana chloroplasts
3
2010
... Trx蛋白表面电荷分布及氧化还原电位(Em)的差异决定了它们的生化特性不同(
... Trx系统在维持叶绿体整体的氧化还原平衡中发挥重要作用(
... 单突变体(
Overexpression of plastidial thioredoxins f and m differentially alters photosynthetic activity and response to oxidative stress in tobacco plants
1
2013
... 叶绿体Trx基因的过表达研究显示, Trx对提高植物适应性有实际应用意义.过表达Trx f的转基因烟草(Nicotiana tabacum)株系表现出生物量和淀粉含量显著增加(
Thiol-based redox control of enzymes involved in the tetrapyrrole biosynthesis pathway in plants
2
2013
... Trx系统在叶绿体光合作用和胁迫应激调控中表现出多点交叉及调控循环网络的特征(
... ;
Posttranslational influence of NADPH-dependent thioredoxin reductase C on enzymes in tetrapyrrole synthesis
0
2013
Involvement of thiol-based mechanisms in plant development
3
2015
... 在叶绿体所含5种Trx中, 只有Trx f来源于真核生物, 其它4种均属于原核起源(
... Trx系统在叶绿体光合作用和胁迫应激调控中表现出多点交叉及调控循环网络的特征(
... 植物类囊体腔是光合电子传递和跨膜质子梯度形成的场所(
Overexpression of plastidial thioredoxin f leads to enhanced starch accumulation in tobacco leaves
1
2013
... 叶绿体Trx基因的过表达研究显示, Trx对提高植物适应性有实际应用意义.过表达Trx f的转基因烟草(Nicotiana tabacum)株系表现出生物量和淀粉含量显著增加(
Chaperone-like properties of tobacco plastid thioredoxins f and m
2
2012
... Trx系统在维持叶绿体整体的氧化还原平衡中发挥重要作用(
... 叶绿体Trx基因的过表达研究显示, Trx对提高植物适应性有实际应用意义.过表达Trx f的转基因烟草(Nicotiana tabacum)株系表现出生物量和淀粉含量显著增加(
Plastid thioredoxins: a “one-for-all” redox-signaling system in plants
1
2013
... 在自然界中, 植物生长环境的光照情况不断变化, 包括日照时数的季节性变化以及由于云量等环境因素导致的光照强度的日变化.不同光照条件下光合作用的优化需要维持其光能吸收和热耗散之间的平衡, 该过程在很大程度上通过叶绿体中氧化还原调控实现.由于FTR是在光照条件下从Fd接收电子, 然后通过还原Trx向Trx传递电子, 在叶绿体中, Trx是很多酶类的电子供体(
Protein redox regulation in the thylakoid lumen: the importance of disulfide bonds for violaxanthin de-epoxidase
2
2015
... 植物类囊体腔是光合电子传递和跨膜质子梯度形成的场所(
... 依赖于叶黄素循环的热耗散被认为是植物光合机构免受过剩光能伤害的主要机制(
Redox regulation of chlorophyll biosynthesis
1
2010
... 巯基基团氧化还原状态的变化(二硫键-巯基)是生物体内普遍存在的现象.在很多情况下, 二硫键的打开和闭合具有调节功能(
Redox regulation of the antimycin A sensitive pathway of cyclic electron flow around photosystem I in higher plant thylakoids
1
2016
... Trx系统在叶绿体光合作用和胁迫应激调控中表现出多点交叉及调控循环网络的特征(
Plant thioredoxin CDSP32 regenerates 1-cys methionine sulfoxide reductase B activity through the direct reduction of sulfenic acid
2
2010
... Trx蛋白表面电荷分布及氧化还原电位(Em)的差异决定了它们的生化特性不同(
... ).它们均属于Trx类似蛋白, 在活性位点上含有非典型氧化还原活性基序.其中, CDSP32受干旱和高光诱导, 并可调节MSRB1的活性(
Thioredoxin f1 and NADPH-dependent thioredoxin reductase C have overlapping functions in regulating photosynthetic metabolism and plant growth in response to varying light conditions
3
2015
... Trx系统在维持叶绿体整体的氧化还原平衡中发挥重要作用(
... Trx系统可以响应不同的光照条件以调节FBP- ase活性和Calvin-Benson循环.拟南芥ntrc突变体生长发育延迟, 叶片呈灰绿色, 并且在短日照下表型更加明显, 暗示了NTRC在光周期调控中的重要作用(
... 由于不同Trx以异构体的形式存在, 因此需构建多重突变体来研究它们之间的相互作用, 如trx f1/f2双突变体和trx m1/m2/m4三突变体.这些研究进一步丰富了Trx系统在光系统功能及光合作用相关酶类的活性调节中的作用.拟南芥trx m1和trx m2单突变体以及trx m1/m2双突变体在正常光照下并无表型差异, 只有在Trx m4基因进一步突变或沉默后才表现出生长迟缓的表型, trx m1/m2/m4三突变体光合能力下降50% (
Inactivation of thioredoxin f1 leads to decreased light activation of ADP- glucose pyrophosphorylase and altered diurnal starch turnover in leaves of Arabidopsis plants
1
2013
... Trx系统可以响应不同的光照条件以调节FBP- ase活性和Calvin-Benson循环.拟南芥ntrc突变体生长发育延迟, 叶片呈灰绿色, 并且在短日照下表型更加明显, 暗示了NTRC在光周期调控中的重要作用(
Thioredoxins play a crucial role in dynamic acclimation of photosynthesis in fluctuating light
1
2017
... 在叶绿体中, FTR和NTRC并非两个相互独立的途径, 而是存在部分功能交叉.FTR并非在所有光能自养生物中都存在, 在某种程度上, 其功能可被NTRC所代替(
Thylakoid protein phosphorylation in higher plant chloroplasts optimizes electron transfer under fluctuating light
1
2010
... 叶绿体通过维持氧化还原平衡来优化PSI与PSII之间的能量分配.激酶STN7参与催化光捕获复合体LHCII的可逆磷酸化, 是控制低光照下PSI与PSII之间能量分配的重要机制.STN7激酶是1个跨类囊体膜蛋白, 催化位点位于叶绿体基质侧, 氧化还原活性位点在类囊体腔(
Overexpression of chloroplast NADPH-dependent thioredoxin reductase in Arabidopsis enhances leaf growth and elucidates in vivo function of reductase and thioredoxin domains
3
2013
... Trx系统在维持叶绿体整体的氧化还原平衡中发挥重要作用(
... 在叶绿体中, FTR和NTRC并非两个相互独立的途径, 而是存在部分功能交叉.FTR并非在所有光能自养生物中都存在, 在某种程度上, 其功能可被NTRC所代替(
... 叶绿体Trx基因的过表达研究显示, Trx对提高植物适应性有实际应用意义.过表达Trx f的转基因烟草(Nicotiana tabacum)株系表现出生物量和淀粉含量显著增加(
Ferredoxin: thioredoxin reductase is required for proper chloroplast development and is involved in the regulation of plastid gene expression in Arabidopsis thaliana
3
2014
... 巯基基团氧化还原状态的变化(二硫键-巯基)是生物体内普遍存在的现象.在很多情况下, 二硫键的打开和闭合具有调节功能(
... ).FTR除了通过还原Trx激活与Calvin- Benson循环直接相关的酶类, 如果糖1, 6二磷酸酶(Fructose-1,6-bisphosphatase, FBPase) (
... 植物含有2种类型的Trx系统, 分别由FTR和NTR扮演TR的角色.叶绿体Trx由FTR和NTRC两种TR还原, 是TR的下游电子受体.在拟南芥中, FTR是一种异二聚体铁-硫蛋白, 由1个催化亚基和1个可变亚基组成, 分别由2个单拷贝的基因编码(
Evidence for a role of chloroplastic m-type thioredoxins in the biogenesis of photosystem II in Arabidopsis
3
2013
... Trx蛋白表面电荷分布及氧化还原电位(Em)的差异决定了它们的生化特性不同(
... Trx系统在维持叶绿体整体的氧化还原平衡中发挥重要作用(
... 由于不同Trx以异构体的形式存在, 因此需构建多重突变体来研究它们之间的相互作用, 如trx f1/f2双突变体和trx m1/m2/m4三突变体.这些研究进一步丰富了Trx系统在光系统功能及光合作用相关酶类的活性调节中的作用.拟南芥trx m1和trx m2单突变体以及trx m1/m2双突变体在正常光照下并无表型差异, 只有在Trx m4基因进一步突变或沉默后才表现出生长迟缓的表型, trx m1/m2/m4三突变体光合能力下降50% (
Membrane topology and mutational analysis of Mycobacterium tuberculosis VKOR, a protein involved in disulfide bond formation and a homologue of human vitamin K epoxide reductase
1
2011
... LTO1 (Lumen Thiol Oxidoreductase 1)是1个类囊体膜蛋白, 由2个结构域组成.N端结构域是1个与哺乳动物维生素K还原酶同源的VKOR结构域, 与细菌DsbB功能类似; 另一个是Trx类似结构域, 具有与细菌巯基氧化还原酶蛋白DsbA同源的Trx类似序列(
The activity of barley NADPH-dependent thioredoxin reductase C is independent of the oligomeric state of the protein: tetrameric structure determined by cryoelec- tron microscopy
1
2011
... 植物含有2种类型的Trx系统, 分别由FTR和NTR扮演TR的角色.叶绿体Trx由FTR和NTRC两种TR还原, 是TR的下游电子受体.在拟南芥中, FTR是一种异二聚体铁-硫蛋白, 由1个催化亚基和1个可变亚基组成, 分别由2个单拷贝的基因编码(
Thioredoxin selecti- vity for thiol-based redox regulation of target proteins in chloroplasts
2
2015
... Trx系统在维持叶绿体整体的氧化还原平衡中发挥重要作用(
... Trx的靶点蛋白在整个叶绿体的亚细胞器结构中都有分布(
Two distinct redox cascades cooperatively regulate chloroplast functions and sustain plant viability
1
2016
... 植物含有2种类型的Trx系统, 分别由FTR和NTR扮演TR的角色.叶绿体Trx由FTR和NTRC两种TR还原, 是TR的下游电子受体.在拟南芥中, FTR是一种异二聚体铁-硫蛋白, 由1个催化亚基和1个可变亚基组成, 分别由2个单拷贝的基因编码(
Distinct electron transfer from ferredoxin-thioredoxin reductase to multiple thioredoxin isoforms in chloroplasts
1
2017
... 在叶绿体所含5种Trx中, 只有Trx f来源于真核生物, 其它4种均属于原核起源(
Distinct redox behaviors of chloroplast thiol enzymes and their relationships with photosynthetic electron transport in Arabidopsis thaliana
1
2014
... Calvin-Benson循环中关键酶的光诱导活化是叶绿体Trx系统的标志性作用.Trx缺失会对酶活性产生直接影响.Trx系统可激活甘油醛-3-磷酸脱氢酶(glyce raldehyde-3-phosphate dehydrogenase, GA- PDH) (
备案号: 京ICP备16067583号-21
版权所有 © 2021 《植物学报》编辑部
地址:北京香山南辛村20号 邮编:100093
电话:010-62836135 010-62836131 E-mail:cbb@ibcas.ac.cn
本系统由北京玛格泰克科技发展有限公司设计开发