
13C pulse labeling reveals the effects of grazing on partitioning of assimilated carbon in an alpine meadow
Jin CHEN1,2, Ming-Hua SONG
通讯作者: *songmh@igsnrr.ac.cn
编委: 陈世苹
责任编辑: 李敏, 赵航(实习)
收稿日期:2019-01-10接受日期:2019-06-25网络出版日期:2019-07-20
基金资助: |
Received:2019-01-10Accepted:2019-06-25Online:2019-07-20
Fund supported: |

摘要
放牧是人类对草地进行利用的重要方式之一, 放牧影响草地生态系统的结构和功能, 改变植物光合碳(C)分配, 进而改变土壤有机碳的储存。青藏高原的高寒草甸是世界上海拔最高的草地生态系统, 寒冷季节长等独特的环境特点使其具有高的土壤有机碳含量。为了揭示长期轻度放牧对植物光合碳分配及植物光合碳在各库之间运移的影响, 基于在青藏高原矮嵩草草甸开展的长期冬季轻度放牧和围栏封育实验, 利用 13C示踪方法揭示了放牧对光合碳在植物地上、地下组织的分配以及光合碳在植物、土壤各碳库中的运移和滞留。研究结果发现, 在 13C标记之后第30天, 冬季轻度放牧样地的植物地上部分内 13C约占开始时 13C含量的32%, 根和土壤中的 13C约占22%, 植物地上部分呼吸中的 13C量约占30%。在放牧和围封这两个不同处理中, 土壤中光合碳的滞留以及光合碳随土壤呼吸释放的速率存在显著差异。长期冬季轻度放牧促使植物将更多的光合碳输入到根和土壤碳库中。与围栏封育处理相比较, 放牧处理下的 13C从植物地上部分输入到地下的速率较快, 通过土壤呼吸释放的速率也快, 而植物地上部分和植物地上部分呼吸中 13C的量较低。另外, 高寒矮嵩草草甸土壤C储量在冬季轻度放牧和围栏封育处理下没有显著差异。我们的研究表明, 尽管冬季轻度放牧改变了植物光合碳分配在地上和地下碳库中的分配, 但是没有显著影响土壤碳库储量。
关键词:
Abstract
Aims In this study, we aim to understand how grazing would influence the partitioning of assimilated carbon in an alpine meadow on the Qinghai-Xizang Plateau.
Methods Measurements on carbon partitioning were made in a long-term grazing experiment consisting of light winter grazing and enclosure treatments. The 13C tracer method was used to determine the partitioning and transportation of assimilated carbon into different carbon pools.
Important findings On the 30th day following the labeling, shoots retained 32% of the initial 13C, and roots and soil together retained 22%; about 30% of the initial 13C were lost through shoot respiration. There were significant differences in the retention in soil, and the respiratory emission from soil, of assimilated carbon between the light grazing and enclosure treatments. Under light grazing, plants invested more assimilated carbon into the root and soil carbon pools. The rate of 13C transportation from shoots to soil and the rate of respiratory 13C release from soil were both greater, and the retention of 13C in and respiratory release from shoots were lower, under light grazing than under enclosure. Our results suggest that grazing is an important mechanism for maintenance of grassland. Grazing may cause changes in the structure and functioning of ecosystems, and induce large variations in soil carbon storage. Alpine meadow in the Qinghai-Xizang Plateau is amongst the grasslands with highest elevation in the world, and has large soil carbon storage due to low temperatures. We found no difference in soil C stocks between light grazing and enclosure treatments, indicating that light grazing would have no significant impact on soil carbon stocks.
Keywords:
PDF (1254KB)元数据多维度评价相关文章导出EndNote|Ris|Bibtex收藏本文
引用本文
陈锦, 宋明华, 李以康. 13C脉冲标记揭示放牧对高寒草甸同化碳分配的 影响 . 植物生态学报, 2019, 43(7): 576-584. DOI: 10.17521/cjpe.2019.0009
CHEN Jin, SONG Ming-Hua, LI Yi-Kang.
草地覆盖了地球陆地表面的25.4% (IPCC, 2001), 而在亚洲近890万km2的草地中, 中国就占了约400万km2 (White et al., 2000)。同时草地有着较大的碳(C)储存能力(200-300 Pg C)(Scurlock & Hall, 1998)。广泛的分布和较高的C储存能力使得草原在全球陆地碳循环和碳固持等方面起着重要作用。草原C库的微小变化会对大气CO2产生较大影响(Menke & Bradford, 1992; Haferkamp & MacNeil, 2004)。青藏高原是世界上海拔最高、面积最大的高原(Herzschuh et al., 2010), 分布于青藏高原的草地约占青藏高原面积的35%。草地是高原人民赖以生存的主要自然资源(Zheng et al., 2000), 在调节区域和周边邻域地区气候方面起着重要的作用。青藏高原具有夏季干旱、冬季寒冷时期长等环境特点, 土壤有机质分解慢, 大量有机质滞留在土壤中(Zhou, 2001)。在不同的草地类型中, 高寒草地具有向地下分配较多光合产物的特点(Kuzyakov & Domanski, 2000)。因此, 高寒草甸土壤的C储量也是最高的(Ni, 2002)。然而, 高寒草甸生态系统土壤的C库和碳循环过程对全球变化极为敏感, 全球气候变暖、土地利用方式改变以及不同的管理方式均会对其有很大的干扰(UNEP, 1993)。
放牧是人类对草地最普遍的一种利用方式, 放牧能改变地上净初级生产力或物种组成(Milchunas & Lauenroth, 1993; Oesterheld et al., 1999; Cingolani et al., 2005)。许多研究表明了不同程度的放牧、放牧历史以及草原类型对草地净生态系统碳交换量(NEE)有影响(Wilsey et al., 2002; Rogiers et al., 2005; Ward et al., 2007; Thomas, 2012)。研究表明, 在未放牧区域, 植物将更多的光合产物分配到地上部分, 禾草类植物的丰度增加, 地上生物量增加(Song et al., 2018), 凋落物累积。与放牧区相比, 未放牧区域有较高的地上生物量和植物覆盖度(Wu et al., 2010)。在停止放牧5年、9年之后, 表层土壤中有机碳的含量分别是放牧地区的1.4和2倍(Gao et al., 2011)。而轻度或者适度地放牧有助于维持生物多样性和地上生物量(McNaughton, 1979)。可能机制之一是减少了植物遮阴面积, 促进了植物剩余组织的光合作用(Doescher et al., 1997)。也有研究认为在适度放牧的情况下, 植物将更多的光合产物向地下分配, 增加了根的生物量(Gao et al., 2008)。根系将分泌更多作为C源的根系分泌物, 有利于土壤微生物快速生长。在土壤养分受限的情况下, 土壤微生物分泌胞外酶用于分解土壤中更难以利用的土壤有机质, 加快土壤有机质的周转, 释放土壤中的养分供植物和微生物利用(孙悦等, 2014)。过度放牧则是引起草地退化的主要原因, 它改变了草原生态系统结构、养分循环以及能量流动过程(McNaughton et al., 1988), 影响了生态系统的稳定性和生产力(McNaughton, 1985, 1993)。
放牧会影响草原的植物-土壤系统中的光合碳的分配。青藏高原冬夏季轮牧是维持草地可持续利用的科学管理方式, 因此高寒草甸有冬季轻度放牧和夏季放牧两种不同的利用方式。但是在高寒草甸, 关于冬季轻度放牧对土壤有机碳的影响的研究较少。为了深入理解长期冬季轻度放牧对植物光合碳分配模式及土壤碳库的影响及其作用机制, 我们运用13C标记法对比在冬季轻度放牧和围栏封育两种处理下植物光合碳在各碳库间的运移和分配模式, 以期回答以下科学问题: (1)冬季轻度放牧对植物光合碳在各个碳库中运移的影响如何?(2)冬季轻度放牧对各碳库光合碳分配模式的影响如何?本研究的实验区域属于冬季轻度放牧区。我们运用13C脉冲标记示踪30天内的光合碳分配的动态变化依次揭示冬季轻度放牧对植物光合碳分配的影响。借助此短期实验能够更好地理解放牧通过影响植物光合碳分配格局进而作用于土壤碳储量。
1 材料和方法
1.1 研究地概况
本研究样点位于中国青海省青藏高原东北部的中国科学院海北高寒草甸生态系统定位研究站(简称海北站, 37.62° N, 101.32° E), 海北站的植被类型是高寒矮嵩草草甸。该地区的海拔高度为3 240 m, 年平均气温为-1.7 ℃, 年降水量约580 mm。降水主要集中在5到9月份(生长季节), 有利于植物生长。植物的生长在8月达到顶峰(Zhou, 2001), 研究区域是矮嵩草草甸, 优势物种为矮生嵩草(Kobresia humilis)和异针茅(Stipa aliena)、垂穗披碱草(Elymus nutans)。植被覆盖率超过95% (Song et al., 2012)。该地区土壤为高山草甸土、高山灌丛草甸土和沼泽土(中国土壤分类学研究组, 1995)。1.2 研究方法
1.2.1 实验设计自2005年, 我们在青海海北实验站建立了80 m × 60 m的研究样地。此样地用围栏围封, 不存在大型动物(牦牛和绵羊等)的采食(Song et al., 2012)。围封区域内植物、土壤的环境一致, 减少或排除了其他异质性因素对实验的影响。在围封区内, 采用完全随机化区组设计, 设置3个裂区, 每个裂区1个样方, 样方大小为2 m × 2 m, 相邻2个样方间隔1 m。样方内与样方外植被的分割采用高为30 cm的铁皮打入样方四周地下, 地上部分保留5 cm。在围封区域旁边是冬季轻度放牧草场, 冬季轻度放牧草场的面积远超于围封样地的面积。围封与放牧样地是用围栏分离开来的, 与围栏的距离是3 m。我们一共设置了5个样方, 每个样方大小为2 m × 2 m, 相邻2个样方间隔1 m。在5个样方中随机选择3个样方, 作为本次13C标记实验中的放牧处理组。实验于2014年8月1日开始, 于8月31日结束。
1.2.2 13C脉冲示踪实验
实验于8月1日至31日期间进行, 在2个研究区内各选3个2 m × 2 m样方用于标记实验。在每个实验样方内选取2个40 cm × 40 cm的小样方。其中一个小样方用于13C示踪实验, 沿着小样方的4个边外侧挖开至2 cm左右深, 将立体金属架架在槽内, 金属架大小为40 cm × 40 cm × 30 cm (长×宽×高); 另一个小样方作为对照样地, 在小样方其4个角用木棍或金属做好标记。用于标记的小样方, 在实验开始的前一天下午, 将塑料薄膜包裹在立体架外面, 并将塑料薄膜沿底部四个边埋入槽内, 用湿土把薄膜盖好, 避免漏气。箱壁插入地面5 cm深, 用孔径为45 μm的尼龙网裹住, 尼龙网延伸至地面下10 cm深, 以便隔断来自标记箱外的植物根系, 而养分和水分可以相互交换。箱体的内表面抹上防雾剂来减少标记过程中箱体表面的水蒸气凝结, 从而增加光强和降低13CO2溶解在箱体内表面水滴中的量。
标记实验选在晴朗天气10:00进行, CO2 (13C原子百分数99%)被储藏在高压瓶内, 通过降压阀和直径4 mm的进气管输入箱内, 流速为0.125 L·min-1, 并用流量计测量流量。密闭箱上部侧面安装了两个风扇, 用于降低内部温度。为了消除CO2浓度对植物光合过程的影响, 需将实验中的CO2浓度控制在大气CO2浓度或者略高于大气中的CO2浓度范围内。13CO2输入1 h后停止, 箱体保持密闭状态再持续5 h (Klumpp & Soussana, 2009)。气体输入流量和标记时间根据以往观测的光合速率和地下生产力来确定, 确保植物地上部分和根系样品有足够高的13C丰度 (Rangel-Castro et al., 2004)。
1.3 样品取样
1.3.1 土壤呼吸气体取样预计在标记后第1、6、12、24 h和第4、7、15和30天收集土壤呼吸释放的CO2。在13C标记后的实际取样过程中, 第1、6、12、24 h进行了呼吸取样, 而13C标记后的第4天发生降雨, 为了减少降雨的干扰, 我们将取样时间推迟到第6天, 随后的取样时间大体按照14、21、30天进行, 推后的时间也控制在1天之内。由于根系取样是在第1、6、14、21、30天, 所以土壤呼吸样品13C的测定也抽取相应时间样品进行了分析。样品收集采用2种不同型号的透明PVC箱, 大箱高15 cm, 直径20 cm, 用于收集植物-土壤系统呼吸释放的CO2, 这些箱内地上植被被保留。小箱高25 cm, 直径5 cm, 用于测定植物根与微生物呼吸, 这些小箱内地上植被被收获。每个箱的顶部有一个用橡皮塞密封的孔用于针头取样。每个箱内抽取70 mL气体立即注射进铝-塑料组合的气袋内(TPV-005, 中国大连德林气体包装有限公司)用于分析呼吸释放的CO2-13C。每一次取样同时在距地面2 m高用注射器取大气样品作为13C的对照测定。
1.3.2 植物取样
在标记后第1、6、14、21、30天, 我们在气体取样同时收获植物样品, 将植物地上部分贴地面剪掉, 仅取绿色枝叶用于13C分析。植物取样时将植物地上部分混匀后随机取样, 用以代表整个群落水平的物种组成。植物根系在采集土壤样品时采集, 根系放在孔径为0.5 mm的筛子内仔细清洗, 去除吸附的土壤和暗棕色/黑色碎屑物质。枝叶和根样品在70 ℃下48 h烘干, 用于测量植物生物量、植物样品中13C、C含量指标。
1.3.3 土壤样品取样
分别在标记后的第1、6、14、21、30天, 用直径5 cm、深度20 cm的土钻在每个样方内的2个小样方取6份土, 将同一样地收集的土壤样本混合成一个复合样本, 并通过一个2 mm的筛子将根系和粗石清除后, 筛选出的土壤在4 ℃下保存, 并将其转移到实验室用于后续的测量, 风干后分析总C和13C含量。此外, 用环刀在每个样方内取得土壤样品, 测得土壤容重指标。
1.4 实验指标测定
1.4.1 植物、土壤样品、呼吸样品的碳稳定同位素比率(δ13C)分析的基本原理和测定过程: 样品经高温燃烧后(氧化/还原炉温度为950 ℃), 通过热导检测器(TCD)测定有机碳含量, 剩余气体导入稳定同位素质谱仪(Delta Plus, Thermo Fisher Scientific, Bremen, Germany), 在质谱仪上测定δ13C值。另外, 用NaOH吸收气体中的CO2, 加入4 mL 0.5 mol·L-1的SrCl2以沉淀碳酸盐。用0.2 mol·L-1 HCl中和未反应的NaOH (Zibilske, 1994)。将沉淀的SrCO 3在1 200 g离心3次, 用蒸馏水冲洗, SrCO3放入105 ℃的烘箱干燥并称质量, 转入锡胶囊中, 导入稳定同位素质谱仪, 在质谱仪上测定δ13C值。植株(根系和地上部)、植物地上部分呼吸和土壤中13C含量的计算:
式中, 13C样品 (kg·m-2)表示样品中13C含量, at%13C (%)表示样品中13C的丰度; labeled和unlabeled分别表示标记和非标记; TC (kg·m-2)表示样品中总有机碳的含量。
1.4.2 植物、土壤C浓度、土壤容重
用于C浓度分析的土壤子样品风干后, 过2 mm筛, 然后在球磨机中研磨。采用改良的Walkley-?Black法, 在175 ℃下煮沸土壤-硫酸混合物5 min (Walkley & Black, 1934; Lettens et al., 2005)。采用1.1逆变换因子对Walkley-Black方法获得的土壤有机碳测量结果进行校正(Lettens et al., 2005), 修正了含水率和干质量对有机物含量的影响。对植物样品烘干称质量后, 利用连续流动同位素质谱仪(EA 1110; CE Instruments, Milan, Italy)(EA-IRMS)和连接到IRMS的ConFlo II设备(FinniganMAT 253, Bremen, Germany)进行分析。测量土壤水分的质量, 并用已知体积的土壤环刀测定土壤容重。
1.4.3 C储量的测定值
植物C储量公式如下:
式中, Cpool (kg·m-2)为植物样品的C库, B样品 (kg·m-2)为植物样品干质量, C样品%为样品C含量。
土壤有机碳储量(解宪丽等, 2004; Yang et al., 2007)公式如下:
式中, SOC (kg·m-2)为土壤有机碳储量, Cs、D、E、G分别为土壤有机C含量(%), 土壤容重(g·cm-3), 土壤层厚度(cm)和大于2 mm的石砾所占的体积百分比(%)。
1.5 数据分析与统计
采用独立样本t-test分析方法, 比较了围封和放牧处理下植物地上部分、根、植物地上部分呼吸、土壤碳库和土壤呼吸速率的差异, 检验了围封和放牧处理对光合碳在植物地上部分、根、植物地上部分呼吸、土壤以及土壤呼吸中的分配比例及随时间变化动态。数据统计分析在SPSS 16软件中进行。2 结果
2.1 围封与放牧对植物生物量、土壤碳库及土壤呼吸的影响
围封样地植物地上部分的C贮存量显著高于冬季轻度放牧样地, 而围封样地植物根的C贮存量则显著低于冬季轻度放牧样地(图1C、1D)。虽然放牧和围封样地的植物地上部分和根的C贮存量有显著差异, 但是对于植物整体的C贮存量来说, 两者之间的差距不显著(图1B)。对于土壤C库来说, 冬季轻度放牧和围封样地之间并没有显著差异(图1A)。在13C脉冲标记实验结束30天后, 围封样地累积的土壤CO2释放量显著高于放牧样地(图2)。图1
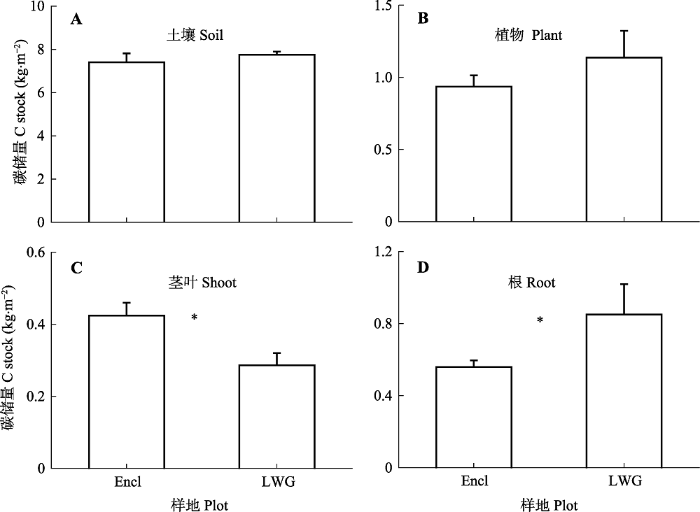
图1冬季轻度放牧(LWG)和围封(Encl)的高寒草甸土壤(A)和植物(B)碳库及植物植物地上部分(C)和根(D)碳储量(平均值±标准误差)。*表示放牧与未放牧样地差异显著(p < 0.05)。
Fig. 1Carbon (C) stock in soil (A) and plants (B) in light winter grazed (LWG) and fence-enclosed (Encl) alpine meadows and in plant shoot (C) and root (D) (mean ± SE). * indicates significant difference between grazed and ungrazed plots (p < 0.05).
图2
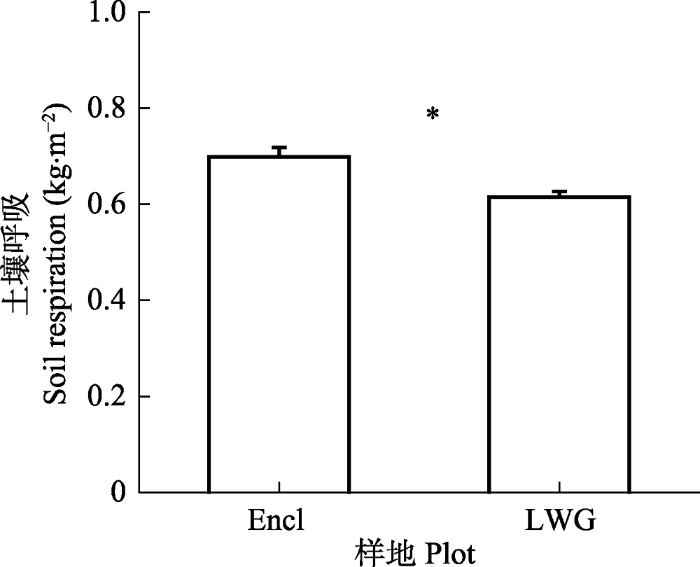
图2冬季轻度放牧(LWG)和围封(Encl)高寒草甸13C标记后30天的累积土壤CO2-C排放情况(平均值±标准误差)。*表示放牧与未放牧样地差异显著(p < 0.05)。
Fig. 2Cumulative soil CO2-C efflux over 30 days following 13C labelling in light winter grazed (LWG) and fence-enclosed (Encl) alpine meadows(mean ± SE). * indicates significant difference between grazed and ungrazed plots (p < 0.05).
2.2 围封与放牧对植物光合碳在植物地上部分、根和土壤、植物地上部分呼吸中分配比例的影响
从总趋势看, 实验期间的不同C库的动态变化不一样。13C标记之后的第一天, 植物地上部分13C约占刚标记的58%, 随着光合固碳的不断进行,植物地上部分13C呈现下降趋势。围封样地的植物地上部分中13C在第6天有上升趋势, 之后再减少, 且在第6、14、21天显著高于放牧样地。到第30天, 植物地上部分内13C约占开始时13C含量的32%, 此时围封样地的值略高于放牧样地, 但二者之间没有显著差异(图3A)。植物地上部分呼吸中13C随着时间的延长总体趋势是增加的, 在13C标记之后第1、6天, 围封样地中的13C显著高于放牧样地, 其他的取样时间内没有显著差异。在标记之后的第30天, 植物地上部分呼吸中的13C约占30% (图3C)。随着实验的进行, 光合产物不断输入到地下, 在13C标记实验结束后第30天, 根和土壤中的13C约占22%。放牧样地中根和土壤中的13C在第1、6、21天显著高于围封样地(图3B)。图3
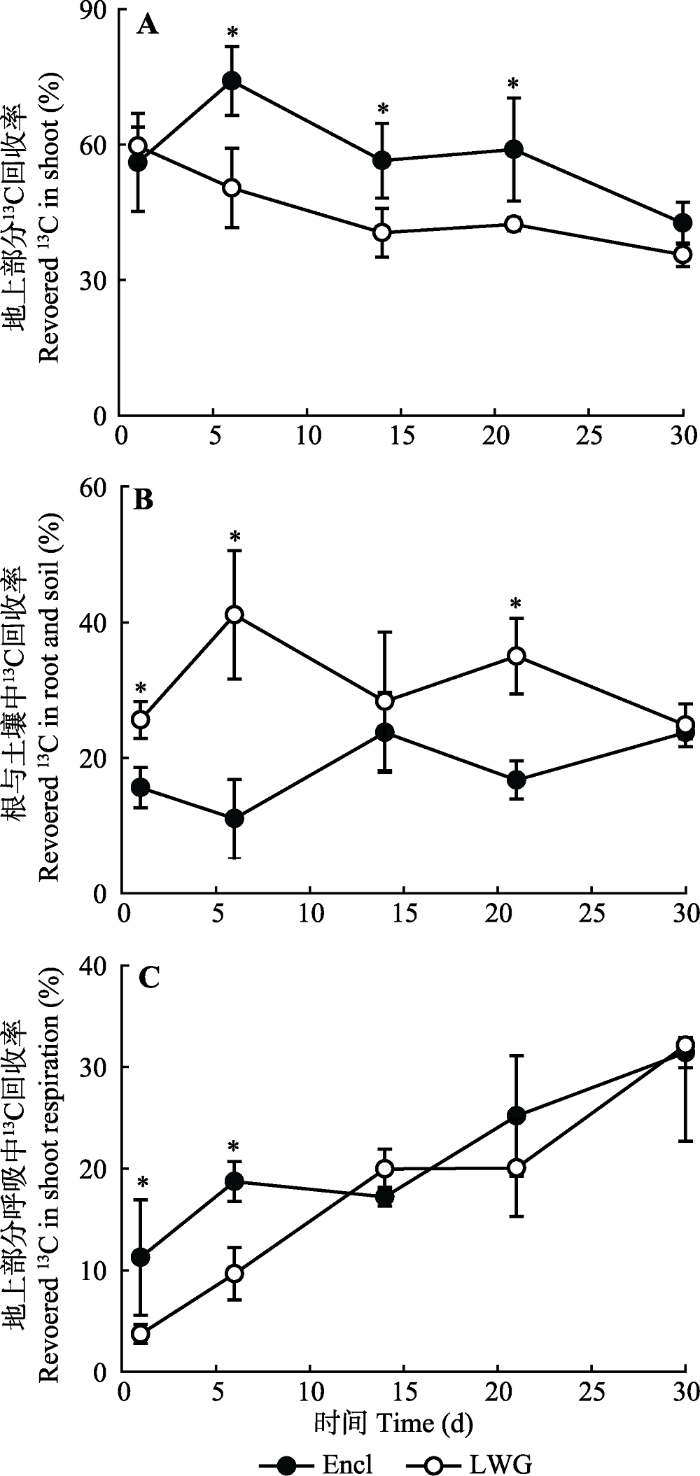
图3在13C标记后的30天期间, 冬季轻度放牧(LWG)和围封(Encl)的高寒草甸的植物地上部分、根和植物地上部分呼吸作用下损失的13C动态(平均值±标准误差)。*表示放牧与未放牧样地差异显著(p < 0.05)。
Fig. 313C dynamics in shoots and roots and 13C losses by shoot respiration during a 30 day period following 13C labelling in light winter grazed (LWG) and fence-enclosed (Encl) alpine meadows (mean ± SE). * indicates significant difference between grazed and ungrazed plots (p < 0.05).
2.3 围封与放牧对植物光合碳在根、土壤中的动态变化及对土壤呼吸释放CO2速率的影响
地下部分进一步划分为根和土壤两部分之后, 我们发现围封样地和放牧样地中根的13C没有显著差异(图4A), 13C分配到土壤中的量随着时间的变化是增加的, 且放牧样地的值高于围封样地的值, 并在第6、14、21天显著不同(图4B)。而从土壤呼吸速率上看, 围封和放牧样地都是呈衰减的趋势, 其中放牧样地土壤中13CO2-C的排放速率下降最快, 除去开始的几天, 围封样地的释放速率高于放牧样地(图5)。图4
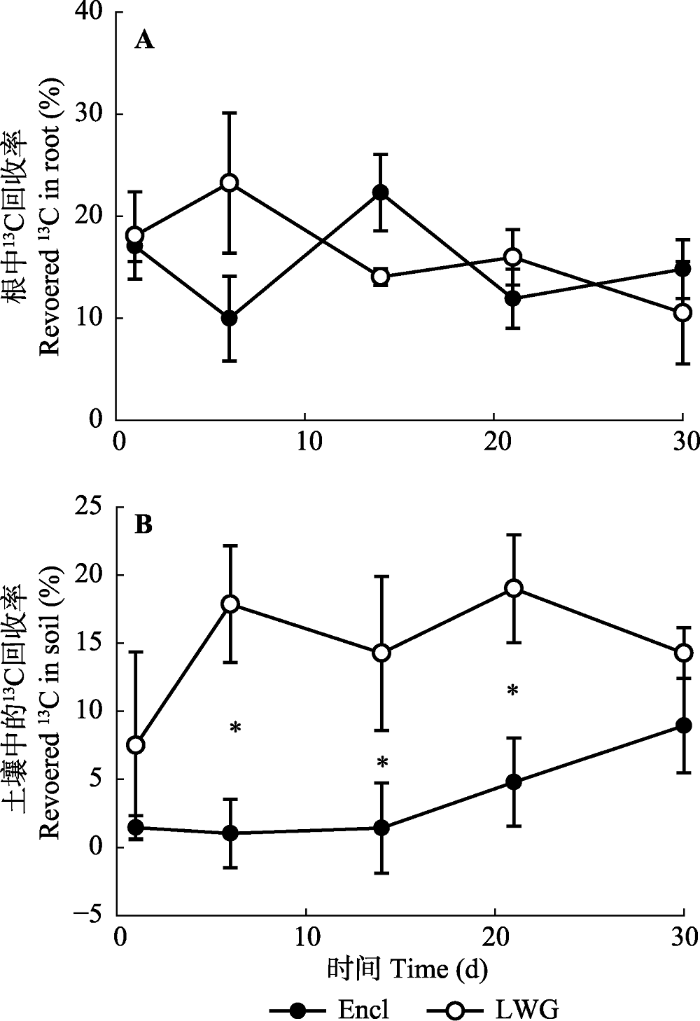
图413C标记后30天, 冬季轻度放牧(LWG)和围封(Encl)的高寒草甸的根和土壤13C动态(平均值±标准误差)。*表示放牧与未放牧样地差异显著(p < 0.05)。
Fig. 413C dynamics in roots and soil during a 30 day period following 13C labelling in light winter grazed (LWG) and fence- enclosed (Encl) alpine meadows (mean ± SE). * indicates significant difference between grazed and ungrazed plots (p < 0.05).
图5
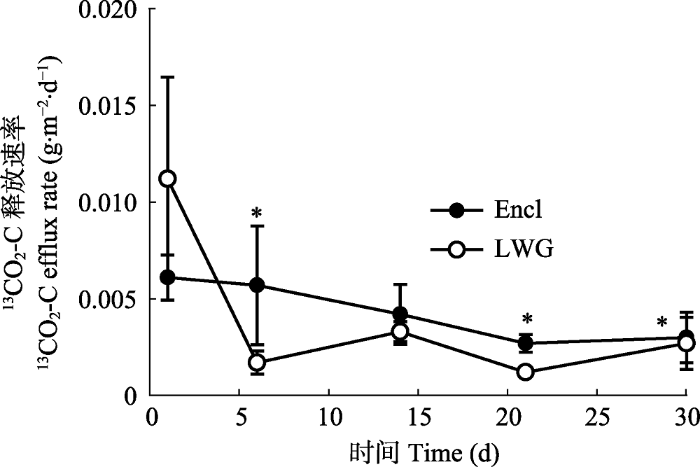
图513CO2-C标记后30天, 冬季轻度放牧(LWG)和围封(Encl)的高寒草甸的土壤中13CO2-C的排放速率(平均值±标准误差)。*表示放牧与未放牧样地差异显著(p < 0.05)。
Fig. 513CO2-C efflux rate in soil of light winter grazed (LWG) and fence-enclosed (Encl) alpine meadows during a 30 day period following 13C labelling (mean ± SE). * indicates significant difference between grazed and ungrazed plots (p < 0.05).
3 讨论
本研究基于13C脉冲标记标记了长期冬季轻度放牧和围封处理下高寒矮嵩草草甸生长旺季植物光合碳在各碳库间的运移和分配模式。结果表明冬季轻度放牧改变了光合碳向地下运移的速度, 也对光合碳在各库中的分配格局产生了显著影响。这些结果能够使我们从机理上更好地理解放牧通过影响植物光合碳分配格局进而影响土壤碳储量。在草地生态系统, 放牧是重要的草地利用方式, 特定区域形成不同的放牧方式。在青藏高原地区, 牧民们主要采用了连牧和轮牧两种方式。冬季放牧的时间是从每年的11月初开始至次年的4月底结束。有研究结果表明, 海北的不同季节的牧草利用率基本都处在35%-60%之间, 属于适度放牧强度。因此, 实验中的冬季轻度放牧处理属于轻度放牧程度(布仁巴音, 2012)。另外, 放牧强度对草原生态系统的影响也存在显著差异。过度放牧之后, 地上生物量减少, 从而减少植物凋落物等植物残体作为有机物质向地下的输入。这可能会减少土壤的C固持量(Morris & Jensen, 1998)。而在轻度或适度放牧情况下, 植物存在补偿机制, 对植物生产力有正向效应(McNaughton, 1985)。适度地放牧会适当抑制禾草植物的丰富度, 从而增加杂类草植物的丰富度, 进而可以维持较高的物种丰富度和均匀度, 有利于群落水平生物量的维持(McNaughton, 1979)。
3.1 放牧和围封下植物光合碳在地上地下的分配
我们发现, 在长期放牧之后, 围封样地的植物地上部分中C库显著高于放牧样地(图1C), 这可能是因为放牧之后, 地上生物量减少。围封样地后, 会增加禾草类植物的丰度, 抑制杂类草植物的生长, 禾草类植物对于群落水平的地上生物量有较高的贡献量(Song et al., 2018)。而放牧样地分配到根中的C显著高于围封样地(图1D), 放牧后植物将更多的光合产物分配到地下部分。这可能是由于在受养分限制的青藏高原中, 植物通过增加根系获取土壤中更多的养分, 用以维持植物本身的生长。有研究认为, 在养分受限的生态系统中, 植物根系分泌的有机物质进入土壤后, 土壤中的微生物活性增强, 分泌胞外酶来分解土壤有机质, 释放养分(Kuzyakov & Domanski, 2000)。土壤呼吸包括了植物根系呼吸和土壤生物呼吸两部分, 但受目前研究技术的限制, 并不能将二者分开来(Kuzyakov & Domanski, 2000)。在实验期间, 围封样地内土壤累积的CO2释放量显著高于放牧样地(图2), 这与在高寒草甸的研究发现一致(Cao et al., 2004)。在短期的13C示踪实验中, 我们发现围封样地和放牧样地中光合产物分配到根的13C没有显著差异(图4A), 因此, 短期实验结果中根系13C含量的差异没有指示出长期放牧处理下根生物量的增加。同时, 土壤和整株植物C储量之间没有显著差异(图1A、1B)。我们的研究发现轻度放牧和围栏封育对草原生态系统的土壤C库的C储量没有差异。这可能是因为长期放牧之后, 虽然植物将更多的光合碳分配到根系中, 但根系的分泌物促进土壤微生物的增长, 加快对土壤有机质的分解(Kuzyakov & Domanski, 2000)。3.2 放牧和围封下植物光合碳在植物、土壤各碳库中的运移
13C标记之后的第一天, 植物地上部分13C约占刚标记的58%, 随着植物光合碳的不断输入, 植物地上部分13C呈现下降趋势(图3A), 光合产物输入到地下, 放牧样地的根和地下碳库中的13C约占24%, 而围封样地占16%左右(图3B)。这说明在放牧样地中13C从植物地上部分输入到地下的速率较快。13C标记后的第一天, 放牧样地土壤中的13C含量约为8%, 而围封样地土壤中的含量只有1%左右, 13C分配到土壤中的量随着时间的变化是逐渐增加的(图4B)。在两种处理中, 我们发现在短期13C示踪实验中, 并没有观测到围封样地和放牧样地中光合产物分配到根的13C的显著差异(图4A), 而在冬季长期轻度放牧的情况下, 放牧样地分配到根中的C储量显著高于围封样地(图1D)。13C脉冲的精度还不能区分出30天以来光合碳分配的动态变化对长期放牧处理下碳分配的累积作用。Hafner等(2012)在高寒草地研究中发现, 进行13C标记之后, 植物活根中的13C的含量最小, 这与我们的研究结果一致。13C主要是通过植物根系分泌物进入土壤, 而植物根系中的13C含量最小, 说明根系中13C转移速度较快。在短期的13C示踪实验中, 我们发现两个处理下的根的光合碳分配没有显著差异(图1D), 而在较长的时间尺度上, 放牧样地的根系生物量显著高于围封样地(图4A)。Hamblin等(1990)认为在养分受限的情况下, 植物会增加根中13C的分配量, 从而可以从更大的空间获取养分。同时, 植物的根系分泌物增加, 土壤中正向激发效应增强, 土壤有机质分解加快, 释放更多的养分用于植物吸收利用。在13C标记之后第30天, 冬季轻度放牧样地的植物地上部分内13C约占开始时13C含量的32% (图3A), 根和土壤中的13C约占22% (图3B)。在标记之后的第30天, 植物地上部分呼吸中的13C量约占30% (图3C), 剩余的则是通过土壤呼吸的途径流失。从土壤呼吸速率上看, 放牧样地土壤中13CO2-C的排放速率下降最快(图5)。土壤呼吸过程中13CO2的排放速率随时间呈现下降趋势, 这可能是对植物地上部分中13C随时间分配情况的响应。在13C标记之后的第1天, 围封样地中植物地上部分呼吸13C含量显著大于放牧样地(图3C), 这跟围封样地的地上植物生物量更多的研究结果相一致, 植物地上部分呼吸中13C含量随着时间总体趋势是增加的。4 结论
研究结果表明, 放牧会引起光合碳在植物地上、地下组织分配的差异。放牧之后, 地上生物量减少, 植物将更多的光合碳输入到根碳库中, 通过增加根系来获取更多的营养。围封样地内土壤累积的CO2释放量显著高于放牧样地(图2)。我们的研究发现, 在冬季轻度放牧情况下, 轻度放牧和围栏封育对草原生态系统的土壤C库的C储量没有差异。土壤中光合碳的滞留以及光合碳随土壤呼吸释放的速率存在显著差异, 与围栏封育处理相比较, 放牧情况下的13C从植物地上部分输入到地下的速率较快。围封样地内土壤累积的CO2释放量显著高于放牧样地(图2), 但放牧处理下的土壤呼吸的释放速率在实验初期高于围封样地。从土壤呼吸速率上看, 放牧样地土壤中13CO2-C的排放速率下降最快 (图5)。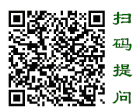
参考文献 原文顺序
文献年度倒序
文中引用次数倒序
被引期刊影响因子
[本文引用: 1]
[本文引用: 1]
DOI:10.1111/gcb.14962URLPMID:31838767 [本文引用: 1]

Numerous studies have demonstrated that fertilization with nutrients such as nitrogen, phosphorus, and potassium increase plant productivity in both natural and managed ecosystems, demonstrating that primary productivity is nutrient limited in most terrestrial ecosystems. In contrast, it has been demonstrated that heterotrophic microbial communities in soil are primarily limited by organic carbon or energy. While this concept of contrasting limitations, i.e., microbial carbon and plant nutrient limitation, is based on strong evidence that we review in this paper, it is often ignored in discussions of ecosystem response to global environment changes. The plant-centric perspective has equated plant-nutrient limitations with those of whole ecosystems, thereby ignoring the important role of the heterotrophs responsible for soil decomposition in driving ecosystem carbon storage. In order to truly integrate carbon and nutrient cycles in ecosystem science, we must account for the fact that while plant productivity may be nutrient- limited, the secondary productivity by heterotrophic communities is inherently carbon-limited. Ecosystem carbon cycling integrates the independent physiological responses of its individual components, as well as tightly coupled exchanges between autotrophs and heterotrophs. To the extent that the interacting autotrophic and heterotrophic processes are controlled by organisms that are limited by nutrient versus carbon accessibility, respectively, we propose that ecosystems by definition cannot be 'limited' by nutrients or carbon alone. Here, we outline how models aimed at predicting non-steady state ecosystem responses over time can benefit from dissecting ecosystems into the organismal components and their inherent limitations to better represent plant-microbe interactions in coupled carbon and nutrient models.
[本文引用: 1]
[本文引用: 1]
DOI:10.1890/03-5272URL [本文引用: 1]
DOI:10.2307/4003731URL [本文引用: 1]
DOI:10.1080/15324982.2011.554954URL [本文引用: 1]
DOI:10.1007/s11104-008-9579-3URL [本文引用: 1]
[本文引用: 1]
DOI:10.1111/j.1365-2486.2011.02557.xURL [本文引用: 1]
[本文引用: 1]
DOI:10.1177/0959683609348882URL [本文引用: 1]
[本文引用: 2]
DOI:10.1111/gcb.2009.15.issue-12URL [本文引用: 4]
DOI:10.1016/j.tplants.2019.07.008URLPMID:31488354 [本文引用: 2]

Increasing the photosynthesis rate of plants has been recently revitalized as an approach for increasing grain crop yields and solving world food crises. The idea that photosynthesis is the key to increasing grain crop yields is not new. Considerable research in the 1970s and 1980s showed that carbon input was not limiting for crop growth and yield. Instead, the availability and uptake of water and nutrients were found to be critical for increasing grain yield, and that conclusion still applies today. In this Opinion article, nitrogen limitation is given particular attention because of its quantitative linkage with vegetative and reproductive growth and its essential role as a quantitative component of seeds.
DOI:10.1111/gcb.2005.11.issue-12URL [本文引用: 2]
DOI:10.1086/283426URL [本文引用: 2]
DOI:10.1111/j.1365-2656.2011.01885.xURLPMID:21801174 [本文引用: 1]

1. Theory predicts that small grazers are regulated by the digestive quality of grass, while large grazers extract sufficient nutrients from low-quality forage and are regulated by its abundance instead. In addition, predation potentially affects populations of small grazers more than large grazers, because predators have difficulty capturing and handling large prey. 2. We analyse the spatial distribution of five grazer species of different body size in relation to gradients of food availability and predation risk. Specifically, we investigate how the quality of grass, the abundance of grass biomass and the associated risks of predation affect the habitat use of small, intermediate and large savanna grazers at a landscape level. 3. Resource selection functions of five mammalian grazer species surveyed over a 21-year period in Serengeti are calculated using logistic regressions. Variables included in the analyses are grass nitrogen, rainfall, topographic wetness index, woody cover, drainage lines, landscape curvature, water and human habitation. Structural equation modelling (SEM) is used to aggregate predictor variables into 'composites' representing food quality, food abundance and predation risk. Subsequently, SEM is used to investigate species' habitat use, defined as their recurrence in 5 × 5 km cells across repeated censuses. 4. The distribution of small grazers is constrained by predation and food quality, whereas the distribution of large grazers is relatively unconstrained. The distribution of the largest grazer (African buffalo) is primarily associated with forage abundance but not predation risk, while the distributions of the smallest grazers (Thomson's gazelle and Grant's gazelle) are associated with high grass quality and negatively with the risk of predation. The distributions of intermediate sized grazers (Coke's hartebeest and topi) suggest they optimize access to grass biomass of sufficient quality in relatively predator-safe areas. 5. The results illustrate how top-down (vegetation-mediated predation risk) and bottom-up factors (biomass and nutrient content of vegetation) predictably contribute to the division of niche space for herbivores that vary in body size. Furthermore, diverse grazing assemblages are composed of herbivores of many body sizes (rather than similar body sizes), because these herbivores best exploit the resources of different habitat types.
DOI:10.1007/s00442-016-3627-0URLPMID:27094543 [本文引用: 1]

The scale of resource heterogeneity may influence how resources are locally partitioned between co-existing large and small organisms such as trees and grasses in savannas. Scale-related plant responses may, in turn, influence herbivore use of the vegetation. To examine these scale-dependent bi-trophic interactions, we varied fertilizer [(nitrogen (N)/phosphorus (P)/potassium (K)] applications to patches to create different scales of nutrient patchiness (patch size 2?×?2?m, 10?×?10?m, or whole-plot 50?×?50?m) in a large field experiment in intact African savanna. Within-patch fertilizer concentration and the total fertilizer load per plot were independently varied. We found that fertilization increased the leaf N and P concentrations of trees and grasses, resulting in elevated utilization by browsers and grazers. Herbivory off-take was particularly considerable at higher nutrient concentrations. Scale-dependent effects were weak. The net effect of fertilization and herbivory was that plants in fertilized areas tended to grow less and develop smaller rather than larger standing biomass compared to plants growing in areas that remained unfertilized. When all of these effects were considered together at the community (plot) level, herbivory completely eliminated the positive effects of fertilization on the plant community. While this was true for all scales of fertilization, grasses tended to profit more from coarse-grained fertilization and trees from fine-grained fertilization. We conclude that in herbivore-dominated communities, such as the African savanna, nutrient patchiness results in the herbivore community profiting rather more than the plant community, irrespective of the scale of patchiness. At the community level, the allometric scaling theory's prediction of plant-and probably also animal-production does not hold or may even be reversed as a result of complex bi-trophic interactions.
DOI:10.1159/000492998URLPMID:30799412 [本文引用: 1]

To improve our understanding of the evolutionary origins of culture and technology in humans, it is vital that we document the full extent of behavioural diversity in our great ape relatives. About half of the world's remaining chimpanzees (Pan spp.) live in the Democratic Republic of Congo (DRC), yet until now we have known almost nothing about their behaviour. Here we describe the insect-related tool technology of Bili-Uéré chimpanzees (Pan troglodytes schweinfurthii) inhabiting an area of at least a 50,000-km2 area in northern DRC, as well as their percussive technology associated with food processing. Over a 12-year period, we documented chimpanzee tools and artefacts at 20 survey areas and gathered data on dung, feeding remains and sleeping nests. We describe a new chimpanzee tool kit: long probes used to harvest epigaeic driver ants (Dorylus spp.), short probes used to extract ponerine ants and the arboreal nests of stingless bees, wands to dip for D. kohli and stout digging sticks used to access underground meliponine bee nests. Epigaeic Dorylus tools were significantly longer than the other tool types, and D. kohli tools were significantly thinner. Tools classified as terrestrial honey-digging sticks were a significant predictor for brushed and blunted tool ends, consistent with their presumed use. We describe two potential new tool types, an &quot;ant scoop&quot; and a &quot;fruit hammer.&quot; We document an extensive percussive technology used to process termite mounds of Cubitermes sp. and Thoracotermes macrothorax and hard-shelled fruits such as Strychnos, along with evidence of the pounding open of African giant snails and tortoises. We encountered some geographic variation in behaviour: we found honey-digging tools, long driver ant probes and fruit-pounding sites only to the north of the Uele River; there were more epigaeic Dorylus tools to the north and more ponerine ant tools to the south. We found no evidence of termite-fishing, despite the availability of Macrotermes muelleri mounds throughout the region. This lack of evidence is consistent with the results of dung washes, which revealed a substantial proportion of driver ants, but no evidence of Macrotermes or other termites. Our results allow us to describe a new chimpanzee behavioural complex, characterised by a general similarity of multiple behaviours across a large, ecologically diverse region but with subtle differences in prey choice and techniques. We propose that this widespread and related suite of behaviours be referred to as the Bili-Uéré Chimpanzee Behavioural Realm. Possible explanations for this pattern are a recent chimpanzee expansion across the region and the interconnectedness of this population across at least the entirety of northern DRC.
DOI:10.1007/s11356-019-06538-4URLPMID:31838703 [本文引用: 1]

Valuation of environmental goods and services are frequently spatially heterogeneous, the significance of this heterogeneity for policy analysis is gradually recognized. For the valuation of environmental goods and services in the context of attaining a better environmental status across Heihe River basin (HRB), Northwest of China by 2020, a survey was conducted employing choice experiment approach across the entire river basin. In this research we estimate the impact of distance from inland rivers' origin (spatial attribute) on the inhabitants' willingness to pay for restoration of ecological attributes. A total of 11 ecological attributes were selected including 6 land related attributes and 5 water related attributes. The present study displays the outcomes of the research planned to examine differences in willingness to pay across different locations/distances. A total of five cities and 33 surround villages/townships were included to examine for location effect, while four ad hoc base distances split samples were recognized for distance effect i.e. ≤100?km, ≤200?km, ≤300 and?&gt;?300?km from rivers' origin. The results of mixed logit model recognized that people living at different locations value the attributes differently. For instance the people of Zhangye region are willing to pay RMB 95.66 annually for improvements in biodiversity while in Gaotai the individuals' willingness to pay (WTP) for the same attributes was RMB 45.68. Similarly, the respondents' living nearer to the origin of river were willing to pay relatively higher amount for the upgradation in the degraded ecosystem services than the remainders. The results of willingness to pay obtained by Krinsky Robb method confirmed that the attributes quality of agricultural products and water quality were the most preferred attributes with the willingness to pay RMB 91.09 and 122.89 respectively. The significant results of willingness to pay may serve as a reference for sustainable improvements and uplifting of the degraded ecological attributes.
DOI:10.2307/2937150URL [本文引用: 1]
DOI:10.1046/j.1365-2745.1998.00251.xURL [本文引用: 1]
DOI:10.1371/journal.pone.0225952URLPMID:31805113

The grassland soils of the Qinghai-Tibet Plateau (QTP) store a large amount of organic carbon because of the cold, humid climate, and topsoil organic carbon is quite sensitive to global climate changes. However, the spatio-temporal dynamics and factors that influence the soil organic carbon (SOC) on the QTP's grassland are not understood well. Moreover, there are few comparative analyses of different approaches to estimate the QTP' SOC. In this study, we estimated the storage and patterns of SOC density (SOCD) using several methods, including MODIS (moderate-resolution imaging spectroradiometer) retrieval, field data and previous empirical models (Models1-4, and soil organic matter (SOM)). And their relations with aboveground biomass, soil moisture, temperature, elevation, and soil conductivity were further explored. The results showed that SOC showed a similar variation trend in the different models, in which it decreased with increasing bulk density (BD) in the topsoil at 30 cm. For meadow and steppe grasslands, Models 1, 2, and 4 showed similar estimated values of SOCD, while Model3 had a lower value than them. SOC storage in the BD 3 and SOM methods had abnormal values, while the MODIS-NDVI, BD 1, 2, and 4 methods had similar SOC stock values for meadow and steppe grassland. Moreover, meadow grassland had a higher SOC storage than did steppe grassland, with means values of 397.9×1010 kg and 242.2×1010 kg, respectively. SOCD's spatial distribution using MODIS-NDVI method differed clearly from the empirical models, with a significant tendency for spatial variation that increased from the northwestern to southeastern regions on the QTP. Therefore, based on the values estimated and spatial variation features, the MODIS-NDVI method may be a more feasible and valid model to estimate SOC. Moreover, the mean annual SOCD values during 2000-2015 showed an increasing trend, with a higher mean value in meadow and a lower mean value in steppe. Further, SOCD was correlated significantly and positively with aboveground biomass and soil moisture, and negatively correlated with elevation and soil conductivity. Increasing temperature had negative effects on SOCD, which was consistent with the global trend. These results indicated that topsoil moisture plays a key role in SOCD spatial patterns. Our results provide valuable support for the long-term estimation of SOCD in future research on the QTP.
[本文引用: 2]
DOI:10.1111/gcb.2004.10.issue-12URL [本文引用: 1]
DOI:10.1007/s00704-004-0099-7URL [本文引用: 1]
DOI:10.1016/j.scitotenv.2018.08.140URLPMID:30118941 [本文引用: 2]

With the proliferation of woody plant species in much of the world's grasslands, human has manipulated landscape fire to return their forage provisioning service. Yet other ecosystem services (e.g., carbon sequestration, biodiversity conservation, erosion control) in the post-managed areas compared to those previously available in the shrub-encroached area are largely unknown, including trade-offs between ecosystem services. Using data from previous publications, we quantitatively synthesized the sustainability of fire as shrub management practice, expressed as its efficacy to control shrubs and its capacity to maintain different ecosystem services. A simple indicator (δ), defined as the ratio of an observed ecological attribute between area experiencing shrub management and untreated control, was used to quantify the changes. Our results showed that fire could be an effective strategy to control shrubs and to increase forage provisioning service (δherbaceous biomass?=?1.39). However, there are possible trade-offs with other ecosystem services (e.g., erosion control, nutrient cycling) when a 54% increase in bare soil cover (δbare soil?=?1.54) and ~74% loss of biological soil crusts cover (δbiological crust?=?0.26) were found. Because increasing forage provisioning at the cost of other ecosystem services might not be sustainable, management should focus on strategies to minimize such trade-offs, which may include but not limited to rotational grazing, adjustment in stocking rate, or supplementary external inputs (e.g., fertilizer). Unless those measures are employed, there is possible emergence of a novel crash (i.e., vegetation- and resource-poor scabland) resulting from a combination of soil erosion and high vulnerability of burnt landscape to exotic species invasion.
DOI:10.1111/gcb.14304URLPMID:29748989 [本文引用: 2]

Input of labile organic carbon can enhance decomposition of extant soil organic carbon (SOC) through priming. We hypothesized that long-term nitrogen (N) input in different chemical forms alters SOC pools by altering priming effects associated with N-mediated changes in plants and soil microbes. The hypothesis was tested by integrating field experimental data of plants, soil microbes and two incubation experiments with soils that had experienced 10?years of N enrichment with three chemical forms (ammonium, nitrate and both ammonium and nitrate) in an alpine meadow on the Tibetan Plateau. Incubations with glucose-13 C addition at three rates were used to quantify effects of exogenous organic carbon input on the priming of SOC. Incubations with microbial inocula extracted from soils that had experienced different long-term N treatments were conducted to detect effects of N-mediated changes in soil microbes on priming effects. We found strong evidence and a mechanistic explanation for alteration of SOC pools following 10?years of N enrichment with different chemical forms. We detected significant negative priming effects both in soils collected from ammonium-addition plots and in sterilized soils inoculated with soil microbes extracted from ammonium-addition plots. In contrast, significant positive priming effects were found both in soils collected from nitrate-addition plots and in sterilized soils inoculated with soil microbes extracted from nitrate-addition plots. Meanwhile, the abundance and richness of graminoids were higher and the abundance of soil microbes was lower in ammonium-addition than in nitrate-addition plots. Our findings provide evidence that shifts toward higher graminoid abundance and changes in soil microbial abundance mediated by N chemical forms are key drivers for priming effects and SOC pool changes, thereby linking human interference with the N cycle to climate change.
DOI:10.1111/j.1365-2486.2012.02738.xURLPMID:28741827 [本文引用: 1]

Plant species and functional groups in nitrogen (N) limited communities may coexist through strong eco-physiological niche differentiation, leading to idiosyncratic responses to multiple nutrition and disturbance regimes. Very little is known about how such responses depend on the availability of N in different chemical forms. Here we hypothesize that idiosyncratic year-to-year responses of plant functional groups to availability and form of nitrogen explain species coexistence in an alpine meadow community after release from grazing. We conducted a 6?year N addition experiment in an alpine meadow on the Tibetan Plateau released from grazing by livestock. The experimental design featured three N forms (ammonium, nitrate, and ammonium nitrate), crossed with three levels of N supply rates (0.375, 1.500 and 7.500?g?N?m-2 ?yr-1 ), with unfertilized treatments without and with light grazing as controls. All treatments showed increasing productivity and decreasing species richness after cessation of grazing and these responses were stronger at higher N rates. Although N forms did not affect aboveground biomass at community level, different functional groups did show different responses to N chemical form and supply rate and these responses varied from year to year. In support of our hypothesis, these idiosyncratic responses seemed to enable a substantial diversity and biomass of sedges, forbs, and legumes to still coexist with the increasingly productive grasses in the absence of grazing, at least at low and intermediate N availability regimes. This study provides direct field-based evidence in support of the hypothesis that idiosyncratic and annually varying responses to both N quantity and quality may be a key driver of community structure and species coexistence. This finding has important implications for the diversity and functioning of other ecosystems with spatial and temporal variation in available N quantity and quality as related to changing atmospheric N deposition, land-use, and climate-induced soil warming.
DOI:10.3724/SP.J.1258.2014.00007URL [本文引用: 1]
DOI:10.3724/SP.J.1258.2014.00007URL [本文引用: 1]
DOI:10.1098/rstb.2012.0102URLPMID:23045706

Biological soil crusts (BSCs) are an important source of organic carbon, and affect a range of ecosystem functions in arid and semiarid environments. Yet the impact of grazing disturbance on crust properties and soil CO(2) efflux remain poorly studied, particularly in African ecosystems. The effects of burial under wind-blown sand, disaggregation and removal of BSCs on seasonal variations in soil CO(2) efflux, soil organic carbon, chlorophyll a and scytonemin were investigated at two sites in the Kalahari of southern Botswana. Field experiments were employed to isolate CO(2) efflux originating from BSCs in order to estimate the C exchange within the crust. Organic carbon was not evenly distributed through the soil profile but concentrated in the BSC. Soil CO(2) efflux was higher in Kalahari Sand than in calcrete soils, but rates varied significantly with seasonal changes in moisture and temperature. BSCs at both sites were a small net sink of C to the soil. Soil CO(2) efflux was significantly higher in sand soils where the BSC was removed, and on calcrete where the BSC was buried under sand. The BSC removal and burial under sand also significantly reduced chlorophyll a, organic carbon and scytonemin. Disaggregation of the soil crust, however, led to increases in chlorophyll a and organic carbon. The data confirm the importance of BSCs for C cycling in drylands and indicate intensive grazing, which destroys BSCs through trampling and burial, will adversely affect C sequestration and storage. Managed grazing, where soil surfaces are only lightly disturbed, would help maintain a positive carbon balance in African drylands.
[本文引用: 2]
DOI:10.1097/00010694-193401000-00003URL [本文引用: 1]
DOI:10.1007/s10021-007-9080-5URL [本文引用: 1]
[本文引用: 1]
DOI:10.1046/j.1461-0248.2002.00322.xURL [本文引用: 1]
DOI:10.1007/s11104-010-0299-0URL [本文引用: 1]
[本文引用: 1]
[本文引用: 1]
DOI:10.1111/gcb.14132URLPMID:29573504 [本文引用: 1]

Forest soils store large amounts of carbon (C) and nitrogen (N), yet how predicted shifts in forest composition will impact long-term C and N persistence remains poorly understood. A recent hypothesis predicts that soils under trees associated with arbuscular mycorrhizas (AM) store less C than soils dominated by trees associated with ectomycorrhizas (ECM), due to slower decomposition in ECM-dominated forests. However, an incipient hypothesis predicts that systems with rapid decomposition-e.g. most AM-dominated forests-enhance soil organic matter (SOM) stabilization by accelerating the production of microbial residues. To address these contrasting predictions, we quantified soil C and N to 1?m depth across gradients of ECM-dominance in three temperate forests. By focusing on sites where AM- and ECM-plants co-occur, our analysis controls for climatic factors that covary with mycorrhizal dominance across broad scales. We found that while ECM stands contain more SOM in topsoil, AM stands contain more SOM when subsoil to 1?m depth is included. Biomarkers and soil fractionations reveal that these patterns are driven by an accumulation of microbial residues in AM-dominated soils. Collectively, our results support emerging theory on SOM formation, demonstrate the importance of subsurface soils in mediating plant effects on soil C and N, and indicate that shifts in the mycorrhizal composition of temperate forests may alter the stabilization of SOM.
[本文引用: 3]
[本文引用: 3]
DOI:10.1016/j.scitotenv.2019.135969URLPMID:31838422

Future climate change-driven alterations in precipitation patterns, increases in temperature, and rises in atmospheric carbon dioxide concentration ([CO2]atm) are expected to alter agricultural productivity and environmental quality, while high latitude countries like Canada are likely to face more challenges from global climate change. However, potential climate change impact on GHG emissions from tile-drained fields is poorly documented. Accordingly, climate change impacts on GHG emissions, N losses to drainage and crop production in a subsurface-drained field in Southern Quebec, Canada were assessed using calibrated and validated RZWQM2 model. The RZWQM2 model was run for a historical period (1971-2000) and for a future period (2038 to 2070) using data generated from 11 different GCM-RCMs (global climate models coupled with regional climate models). Under the projected warmer and higher rainfall conditions mean drainage flow was predicted to increase by 17%, and the N losses through subsurface drains increase by 47%. Despite the negative effect of warming temperature on crop yield, soybean yield was predicted to increase by 31% due to increased photosynthesis rates and improved crop water use efficiency (WUE) under elevated [CO2]atm, while corn yield was reduced by 7% even with elevated [CO2]atm because of a shorter life cycle from seedling to maturity resulted from higher temperature. The N2O emissions would be enhanced by 21% due to greater denitrification and mineralization, while CO2 emissions would increase by 16% because of more crop biomass accumulation, higher crop residue decomposition, and greater soil microbial activities. Soil organic carbon storage was predicted to decrease 22% faster in the future, which would result in higher global warming potential in turn. This study demonstrates the potential of exacerbating GHG emissions and water quality problems and reduced corn yield under climate change impact in subsurface drained fields in southern Quebec.
不同季节放牧对高寒草甸植物群落多样性和生产力的影响
1
2012
... 在草地生态系统, 放牧是重要的草地利用方式, 特定区域形成不同的放牧方式.在青藏高原地区, 牧民们主要采用了连牧和轮牧两种方式.冬季放牧的时间是从每年的11月初开始至次年的4月底结束.有研究结果表明, 海北的不同季节的牧草利用率基本都处在35%-60%之间, 属于适度放牧强度.因此, 实验中的冬季轻度放牧处理属于轻度放牧程度(
不同季节放牧对高寒草甸植物群落多样性和生产力的影响
1
2012
... 在草地生态系统, 放牧是重要的草地利用方式, 特定区域形成不同的放牧方式.在青藏高原地区, 牧民们主要采用了连牧和轮牧两种方式.冬季放牧的时间是从每年的11月初开始至次年的4月底结束.有研究结果表明, 海北的不同季节的牧草利用率基本都处在35%-60%之间, 属于适度放牧强度.因此, 实验中的冬季轻度放牧处理属于轻度放牧程度(
Grazing intensity alters soil respiration in an alpine meadow on the Tibetan Plateau
1
2004
... 我们发现, 在长期放牧之后, 围封样地的植物地上部分中C库显著高于放牧样地(
1
1995
... 本研究样点位于中国青海省青藏高原东北部的中国科学院海北高寒草甸生态系统定位研究站(简称海北站, 37.62° N, 101.32° E), 海北站的植被类型是高寒矮嵩草草甸.该地区的海拔高度为3 240 m, 年平均气温为-1.7 ℃, 年降水量约580 mm.降水主要集中在5到9月份(生长季节), 有利于植物生长.植物的生长在8月达到顶峰(
1
1995
... 本研究样点位于中国青海省青藏高原东北部的中国科学院海北高寒草甸生态系统定位研究站(简称海北站, 37.62° N, 101.32° E), 海北站的植被类型是高寒矮嵩草草甸.该地区的海拔高度为3 240 m, 年平均气温为-1.7 ℃, 年降水量约580 mm.降水主要集中在5到9月份(生长季节), 有利于植物生长.植物的生长在8月达到顶峰(
Grazing effects on rangeland diversity: A synthesis of contemporary models
1
2005
... 放牧是人类对草地最普遍的一种利用方式, 放牧能改变地上净初级生产力或物种组成(
Gas exchange of Idaho fescue in response to defoliation and grazing history
1
1997
... 放牧是人类对草地最普遍的一种利用方式, 放牧能改变地上净初级生产力或物种组成(
Effectiveness of exclosures on restoration of degraded alpine meadow in the eastern Tibetan Plateau
1
2011
... 放牧是人类对草地最普遍的一种利用方式, 放牧能改变地上净初级生产力或物种组成(
Belowground net primary productivity and biomass allocation of a grassland in Inner Mongolia is affected by grazing intensity
1
2008
... 放牧是人类对草地最普遍的一种利用方式, 放牧能改变地上净初级生产力或物种组成(
Grazing effects on carbon dynamics in the northern mixed-grass prairie
1
2004
... 草地覆盖了地球陆地表面的25.4% (
Effect of grazing on carbon stocks and assimilate partitioning in a Tibetan montane pasture revealed by 13CO2 pulse labeling
1
2012
... 13C标记之后的第一天, 植物地上部分13C约占刚标记的58%, 随着植物光合碳的不断输入, 植物地上部分13C呈现下降趋势(
The cost of stress: Dry matter partitioning changes with seasonal supply of water and nitrogen to dryland wheat
1
1990
... 13C标记之后的第一天, 植物地上部分13C约占刚标记的58%, 随着植物光合碳的不断输入, 植物地上部分13C呈现下降趋势(
Holocene land-cover changes on the Tibetan Plateau. The
1
2010
... 草地覆盖了地球陆地表面的25.4% (
2
2001
... 草地覆盖了地球陆地表面的25.4% (
... 标记实验选在晴朗天气10:00进行, CO2 (13C原子百分数99%)被储藏在高压瓶内, 通过降压阀和直径4 mm的进气管输入箱内, 流速为0.125 L·min-1, 并用流量计测量流量.密闭箱上部侧面安装了两个风扇, 用于降低内部温度.为了消除CO2浓度对植物光合过程的影响, 需将实验中的CO2浓度控制在大气CO2浓度或者略高于大气中的CO2浓度范围内.13CO2输入1 h后停止, 箱体保持密闭状态再持续5 h (
Using functional traits to predict grassland ecosystem change: A mathematical test of the response-and-effect trait approach
4
2009
... 草地覆盖了地球陆地表面的25.4% (
... 我们发现, 在长期放牧之后, 围封样地的植物地上部分中C库显著高于放牧样地(
... ).土壤呼吸包括了植物根系呼吸和土壤生物呼吸两部分, 但受目前研究技术的限制, 并不能将二者分开来(
... 、1B).我们的研究发现轻度放牧和围栏封育对草原生态系统的土壤C库的C储量没有差异.这可能是因为长期放牧之后, 虽然植物将更多的光合碳分配到根系中, 但根系的分泌物促进土壤微生物的增长, 加快对土壤有机质的分解(
Carbon input by plants into the soil. Review
2
2000
... 用于C浓度分析的土壤子样品风干后, 过2 mm筛, 然后在球磨机中研磨.采用改良的Walkley-?Black法, 在175 ℃下煮沸土壤-硫酸混合物5 min (
... ).采用1.1逆变换因子对Walkley-Black方法获得的土壤有机碳测量结果进行校正(
Soil organic carbon changes in landscape units of Belgium between 1960 and 2000 with reference to 1990
2
2005
... 放牧是人类对草地最普遍的一种利用方式, 放牧能改变地上净初级生产力或物种组成(
... 在草地生态系统, 放牧是重要的草地利用方式, 特定区域形成不同的放牧方式.在青藏高原地区, 牧民们主要采用了连牧和轮牧两种方式.冬季放牧的时间是从每年的11月初开始至次年的4月底结束.有研究结果表明, 海北的不同季节的牧草利用率基本都处在35%-60%之间, 属于适度放牧强度.因此, 实验中的冬季轻度放牧处理属于轻度放牧程度(
Grazing as an optimization process: Grass-ungulate relationships in the Serengeti
2
1979
... 放牧是人类对草地最普遍的一种利用方式, 放牧能改变地上净初级生产力或物种组成(
... 在草地生态系统, 放牧是重要的草地利用方式, 特定区域形成不同的放牧方式.在青藏高原地区, 牧民们主要采用了连牧和轮牧两种方式.冬季放牧的时间是从每年的11月初开始至次年的4月底结束.有研究结果表明, 海北的不同季节的牧草利用率基本都处在35%-60%之间, 属于适度放牧强度.因此, 实验中的冬季轻度放牧处理属于轻度放牧程度(
Ecology of a grazing ecosystem: The Serengeti
1
1985
... 放牧是人类对草地最普遍的一种利用方式, 放牧能改变地上净初级生产力或物种组成(
Grasses and grazers, science and management
1
1993
... 放牧是人类对草地最普遍的一种利用方式, 放牧能改变地上净初级生产力或物种组成(
Large mammals and process dynamics in African ecosystems
1
1988
... 草地覆盖了地球陆地表面的25.4% (
Rangelands
1
1992
... 放牧是人类对草地最普遍的一种利用方式, 放牧能改变地上净初级生产力或物种组成(
Quantitative effects of grazing on vegetation and soils over a global range of environments
1
1993
... 在草地生态系统, 放牧是重要的草地利用方式, 特定区域形成不同的放牧方式.在青藏高原地区, 牧民们主要采用了连牧和轮牧两种方式.冬季放牧的时间是从每年的11月初开始至次年的4月底结束.有研究结果表明, 海北的不同季节的牧草利用率基本都处在35%-60%之间, 属于适度放牧强度.因此, 实验中的冬季轻度放牧处理属于轻度放牧程度(
The carbon balance of grazed and non-grazed Spartina anglica saltmarshes at Skallingen, Denmark
1
1998
... 草地覆盖了地球陆地表面的25.4% (
Carbon storage in grasslands of China
2002
Grazing, fire, and climate effects on primary productivity of grasslands and savannas
2
1999
... 放牧是人类对草地最普遍的一种利用方式, 放牧能改变地上净初级生产力或物种组成(
... 标记实验选在晴朗天气10:00进行, CO2 (13C原子百分数99%)被储藏在高压瓶内, 通过降压阀和直径4 mm的进气管输入箱内, 流速为0.125 L·min-1, 并用流量计测量流量.密闭箱上部侧面安装了两个风扇, 用于降低内部温度.为了消除CO2浓度对植物光合过程的影响, 需将实验中的CO2浓度控制在大气CO2浓度或者略高于大气中的CO2浓度范围内.13CO2输入1 h后停止, 箱体保持密闭状态再持续5 h (
Carbon flow in an upland grassland: Effect of liming on the flux of recently photosynthesized carbon to rhizosphere soil
1
2004
... 放牧是人类对草地最普遍的一种利用方式, 放牧能改变地上净初级生产力或物种组成(
Effect of land management on ecosystem carbon fluxes at a subalpine grassland site in the Swiss Alps
1
2005
... 草地覆盖了地球陆地表面的25.4% (
The global carbon sink: A grassland perspective
2
1998
... 放牧是人类对草地最普遍的一种利用方式, 放牧能改变地上净初级生产力或物种组成(
... 我们发现, 在长期放牧之后, 围封样地的植物地上部分中C库显著高于放牧样地(
Shifts in priming partly explain impacts of long-term nitrogen input in different chemical forms on soil organic carbon storage
2
2018
... 本研究样点位于中国青海省青藏高原东北部的中国科学院海北高寒草甸生态系统定位研究站(简称海北站, 37.62° N, 101.32° E), 海北站的植被类型是高寒矮嵩草草甸.该地区的海拔高度为3 240 m, 年平均气温为-1.7 ℃, 年降水量约580 mm.降水主要集中在5到9月份(生长季节), 有利于植物生长.植物的生长在8月达到顶峰(
... 自2005年, 我们在青海海北实验站建立了80 m × 60 m的研究样地.此样地用围栏围封, 不存在大型动物(牦牛和绵羊等)的采食(
Different inter-annual responses to availability and form of nitrogen explain species coexistence in an alpine meadow community after release from grazing
1
2012
... 放牧是人类对草地最普遍的一种利用方式, 放牧能改变地上净初级生产力或物种组成(
根际激发效应的发生机制及其生态重要性
1
2014
... 放牧是人类对草地最普遍的一种利用方式, 放牧能改变地上净初级生产力或物种组成(
根际激发效应的发生机制及其生态重要性
1
2014
... 放牧是人类对草地最普遍的一种利用方式, 放牧能改变地上净初级生产力或物种组成(
Impact of grazing intensity on seasonal variations in soil organic carbon and soil CO2 efflux in two semiarid grasslands in southern Botswana
2012
Agenda 21 Rio Declaration
2
1993
... 草地覆盖了地球陆地表面的25.4% (
... 用于C浓度分析的土壤子样品风干后, 过2 mm筛, 然后在球磨机中研磨.采用改良的Walkley-?Black法, 在175 ℃下煮沸土壤-硫酸混合物5 min (
An examination of the Degtjareff method for determining soil organic matter, and a proposed modification of the chromic acid titration method
1
1934
... 放牧是人类对草地最普遍的一种利用方式, 放牧能改变地上净初级生产力或物种组成(
Long-term consequences of grazing and burning on northern peatland carbon dynamics
1
2007
... 草地覆盖了地球陆地表面的25.4% (
Pilot Analysis of Global Ecosystems: Grassland Ecosystems
1
2000
... 放牧是人类对草地最普遍的一种利用方式, 放牧能改变地上净初级生产力或物种组成(
Tropical pasture carbon cycling: Relationships between C source/sink strength, above-ground biomass and grazing
1
2002
... 放牧是人类对草地最普遍的一种利用方式, 放牧能改变地上净初级生产力或物种组成(
Long-term fencing improved soil properties and soil organic carbon storage in an alpine swamp meadow of western China
1
2010
... 土壤有机碳储量(
中国土壤有机碳密度和储量的估算与空间分布分析
1
2004
... 土壤有机碳储量(
中国土壤有机碳密度和储量的估算与空间分布分析
1
2004
... 土壤有机碳储量(
Storage, patterns and environmental controls of soil organic carbon in China
1
2007
... 草地覆盖了地球陆地表面的25.4% (
Mountain Geoecology and Sustainable Development of the Tibetan Plateau
2000
3
2001
... 草地覆盖了地球陆地表面的25.4% (
... 本研究样点位于中国青海省青藏高原东北部的中国科学院海北高寒草甸生态系统定位研究站(简称海北站, 37.62° N, 101.32° E), 海北站的植被类型是高寒矮嵩草草甸.该地区的海拔高度为3 240 m, 年平均气温为-1.7 ℃, 年降水量约580 mm.降水主要集中在5到9月份(生长季节), 有利于植物生长.植物的生长在8月达到顶峰(
... 分析的基本原理和测定过程: 样品经高温燃烧后(氧化/还原炉温度为950 ℃), 通过热导检测器(TCD)测定有机碳含量, 剩余气体导入稳定同位素质谱仪(Delta Plus, Thermo Fisher Scientific, Bremen, Germany), 在质谱仪上测定δ13C值.另外, 用NaOH吸收气体中的CO2, 加入4 mL 0.5 mol·L-1的SrCl2以沉淀碳酸盐.用0.2 mol·L-1 HCl中和未反应的NaOH (
3
2001
... 草地覆盖了地球陆地表面的25.4% (
... 本研究样点位于中国青海省青藏高原东北部的中国科学院海北高寒草甸生态系统定位研究站(简称海北站, 37.62° N, 101.32° E), 海北站的植被类型是高寒矮嵩草草甸.该地区的海拔高度为3 240 m, 年平均气温为-1.7 ℃, 年降水量约580 mm.降水主要集中在5到9月份(生长季节), 有利于植物生长.植物的生长在8月达到顶峰(
... 分析的基本原理和测定过程: 样品经高温燃烧后(氧化/还原炉温度为950 ℃), 通过热导检测器(TCD)测定有机碳含量, 剩余气体导入稳定同位素质谱仪(Delta Plus, Thermo Fisher Scientific, Bremen, Germany), 在质谱仪上测定δ13C值.另外, 用NaOH吸收气体中的CO2, 加入4 mL 0.5 mol·L-1的SrCl2以沉淀碳酸盐.用0.2 mol·L-1 HCl中和未反应的NaOH (
Carbon mineralization
1994