
Responses of soil N2O emissions to experimental warming regulated by soil moisture in an alpine steppe
WANG Guan-Qin1,2, LI Fei1,2, PENG Yun-Feng1, CHEN Yong-Liang1, HAN Tian-Feng1, YANG Gui-Biao1,2, LIU Li1,2, ZHOU Guo-Ying3, YANG Yuan-He
通讯作者:
基金资助: |
Online:2018-01-20
Fund supported: |

摘要
关键词:
Abstract
Keywords:
PDF (1876KB)元数据多维度评价相关文章导出EndNote|Ris|Bibtex收藏本文
本文引用格式
王冠钦, 李飞, 彭云峰, 陈永亮, 韩天丰, 杨贵彪, 刘莉, 周国英, 杨元合. 土壤含水量调控高寒草原生态系统N2O排放对增温的响应. 植物生态学报[J], 2018, 42(1): 105-115 doi:10.17521/cjpe.2017.0164
WANG Guan-Qin.
氧化亚氮(N2O)是大气中一种重要的温室气体, 其温室效应是CO2的298倍(IPCC, 2013)。此外, N2O还是导致臭氧层被破坏的主要气体(Ravishankara et al., 2009), 因而受到学术界的广泛关注。自然生态系统中土壤N2O排放已成为大气N2O不可忽视的来源, 尤其是气候变暖等全球变化要素加剧了土壤N2O的排放(Flechard et al., 2007)。按照目前的估计, 陆地生态系统中土壤向大气排放的N2O已达到6.6 Tg N·a-1, 占自然界N2O排放总量的60% (IPCC, 2013)。因此, 明确土壤N2O排放对气候变暖的响应及其调控因素对预测未来大气N2O浓度、制定相关减排政策至关重要。
近10年来, 国内外****相继开展了土壤N2O对增温响应方面的研究。多数研究报道增温会促进不同生态系统中土壤N2O的排放(Mosier, 2008; Shi et al., 2012; Voigt et al., 2016), 然而, 也有少数研究发现增温会抑制土壤N2O的排放(Liu et al., 2016)或者未造成显著影响(Ward et al., 2013; 徐冰鑫等, 2014), 甚至可能会造成N2O的吸收(Chapuis-Lardy et al., 2007)。不同研究结果之间的差异可能与以下3个方面的因素有关: 首先, 不同研究结果之间的差异可能与底物差异有关。土壤N2O的产生由NO3-还原的反硝化过程和NH4+氧化为NO3-的硝化作用主导(Butterbach-Bahl et al., 2013)。土壤NO3-含量和有机碳含量是调控反硝化作用最重要的底物因素, 而土壤NH4+含量和通气性是调控硝化作用的两个基本因素, 其他因素主要起间接作用进而影响土壤N2O排放(Robertson, 1982; Chapin III et al., 2011)。因此, 底物的差异在一定程度上能够解释土壤N2O通量对增温的多元化响应。其次, 不同研究结果之间的差异可能与环境条件差异有关。例如, 增温对温带地区土壤N2O通量的影响较小(Xu et al., 2017), 但增温会显著促进高寒地区土壤N2O排放(Shi et al., 2012)。除了温度, 水分的差异也会影响N2O通量对增温的响应: 增温会促进湿润地区土壤N2O的排放(Yan et al., 2014), 但会导致土壤含水量的显著下降(Hu et al., 2010), 进而可能会抑制增温对干旱地区土壤N2O排放的影响。再次, 不同研究结果之间的差异还可能与微生物功能基因丰度的差异有关。以往的研究表明, 氨氧化古菌(AOA)和氨氧化细菌(AOB)是主导硝化作用产生N2O的功能微生物(Chen et al., 2013b; 刘正辉和李德豪, 2015), 反硝化细菌是主导反硝化作用产生N2O的功能微生物(Rotthauwe et al., 1997; 郭丽芸等, 2011)。然而, 在高寒干旱的环境中, 增温如何影响AOA和AOB, 进而如何影响土壤N2O通量还不是很清楚。
高寒草原是青藏高原面积最大的草地生态系统, 其分布面积达6.4 × 105 km2 (Ding et al., 2016)。近几十年来, 高寒草原分布区经历了显著的气候变暖(Chen et al., 2013a), 同时, 由于海拔高、温度低, 该地区对气候变暖十分敏感(Zhang et al., 2015)。此外, 高寒草原分布区降水量少, 生态系统功能受到水分的限制(Liu et al., 2008), 进而为研究干旱和半干旱生态系统中N2O通量对增温的响应提供了理想平台。为此, 本研究依托在青海省三角城种羊场建立的高寒草原增温控制实验平台, 基于静态箱-气相色谱法测定了土壤N2O通量, 并利用定量PCR技术测定了相关的功能基因丰度。在此基础上试图揭示: 1)高寒草原生态系统土壤N2O通量对增温的响应; 2)调控土壤N2O通量的生物与非生物因素。
1 材料和方法
1.1 研究区域概况
本研究在青海省刚察县三角城种羊场(地理位置37.30° N, 100.25° E, 海拔3β290 m)进行。该地区属高原大陆性气候, 1980-2012年年平均气温为0.08 ℃, 年降水量为387 mm (Peng et al., 2017)。研究区域的植被类型为典型的高寒草原, 优势种为紫花针茅(Stipa purpurea)和细叶薹草(Carex duriuscula subsp. stenophylloides), 其他物种包括冰草(Agropyron cristatum)、冷地早熟禾(Poa crymophila)及阿尔泰狗娃花(Heteropappus altaicus)等。实验地的初始土壤基本理化性质为: 黏粒含量4.9%, 粉粒含量33.4%, 砂粒含量61.7%, 有机碳含量20.5 g·kg-1, 总氮含量2.5 g·kg-1 (Peng et al., 2017)。1.2 实验设计
我们于2013年6月在研究区建立了高寒草原增温控制实验平台(图1), 设置了立地条件基本一致的50 m × 50 m的围封区, 并在围封区内随机布置10个4 m × 4 m的样方。增温-对照处理采用配对设置, 即每个样方的对角分别设置对照(C)与增温(W)处理, 共10个重复。参照国际苔原计划中的经典设计, 利用六边形的开顶箱增温装置对地表大气及表层土壤进行增温处理, 每个开顶箱的顶边长为80 cm, 底边长为120 cm, 高度为50 cm (Chapin III & Shaver, 1985)。开顶箱增温装置由透明亚克力材质构成, 透光率在92%以上, 并全年放置在野外实现整年增温。为了揭示增温对土壤温湿度的影响, 我们在每个处理内安装了EM50数据采集器(Decagon Devices, Pullman, USA)测定土壤温湿度。EM50能全年、全天候采集数据, 即每30 min自动测定并储存0- 5 cm土壤温度和湿度。图1
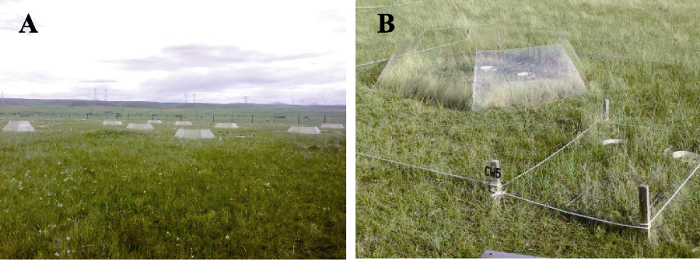
图1高寒草原样地及增温控制实验平台照片。A, 样地照片。B, 开顶箱增温装置。
Fig. 1Photos of the study site and warming experiment. A, Photo of the study site. B, Open-top chamber (OTC) warming facility.
1.3 研究方法
1.3.1 温室气体通量的测定与计算我们于2013年6月在对照和增温处理中分别布设了一个40 cm × 40 cm的不锈钢底座, 底座打入土壤约6 cm。在2014和2015年的生长季(5-10月)利用静态箱-气相色谱法(Kaufman, 1994; Tang et al., 2006)测定了土壤N2O通量。静态箱为不锈钢暗箱(40 cm × 40 cm × 30 cm), 外层用泡沫保温材料覆盖, 内部安装风扇混匀箱内气体。每月采集3次气体样品, 每次采集在9:00-12:00进行。采样前向底座的凹槽中注水, 将静态箱覆盖到基座形成密闭空间。利用100 mL医用注射器采集样品, 针头与接入暗箱的乳胶管经三通阀连接, 以确保气密性。分别在0、10、20、30和40 min抽取一次气体样品, 气体样品注射到100 mL的铝箔气袋中保存, 利用气相色谱仪(Agilent 7890A, Agilent Technologies, Santa Clara, USA)测定样品中的N2O浓度, 通过拟合N2O浓度与采样时间的线性变化关系, 得到土壤N2O通量。此外, 野外采集气体样品的同时, 使用探针式温度计测定箱内气体温度。
土壤N2O排放通量用以下公式(Tang et al., 2006)计算:
$F=\frac{M}{{{V}_{0}}}\times \frac{P}{{{P}_{0}}}\times \frac{{{T}_{0}}}{T}\times H\times \frac{dc}{dt}$
式中: F为N2O通量(μg·m-2·h-1); M为N2O的摩尔质量(g·mol-1); P0和T0为理想气体在标准状态下的空气压力和温度(分别为101.3 kPa和273.2 K); V0为气体在标准状态下的摩尔体积(22.4 L·mol-1); H为采样箱内气室高度(30 cm); P和T为采样时箱内的实际气压(kPa)和气温(K); dc/dt为箱体上部N2O气体浓度随时间变化的斜率(10-6·h-1)。F > 0表示排放, F < 0表示吸收。
1.3.2 土壤样品采集与测定
为了揭示土壤N2O通量对增温响应的调控因素, 我们于2014和2015年的生长季旺盛期(8月)采集了土壤样品。分别在各个样方的对照与增温处理中随机选取3个点, 去除表层凋落物后, 用直径3 cm的土钻采集0-10 cm的土壤样品, 将3个土钻的样品混合均匀后装入自封袋中。新鲜样品立即运回实验室, 挑除可见的根系后, 过2 mm筛并在-20 ℃条件下保存。
土壤样品采用1 mol·L-1 KCl溶液浸提, 之后采用连续流动分析仪(Autoanalyzer 3 SEAL, Bran and Luebbe, Norderstedt, Germany)测定浸提液中的NH4+-N和NO3--N含量, 二者相加得到土壤总无机氮(SIN)含量。土壤微生物碳(MBC)和微生物氮(MBN)采用氯仿熏蒸法(Vance et al., 1987)测定。首先取20 g鲜土放入真空干燥器, 同时放入氯仿并抽真空使氯仿沸腾5 min, 25 ℃下放置24 h后用0.5 mol·L-1 K2SO4浸提; 同时另取20 g未熏蒸鲜土直接用K2SO4浸提(吴金水等, 2006)。采用C/N分析仪(multi-N/C 3100, Analytik Jena AG, Jena, Germany)测定熏蒸与未熏蒸样品浸提液的C、N含量, 计算二者之间的差值得到MBC和MBN, 转换系数分别为0.45和0.54 (Brookes et al., 1985; Tischer et al., 2014)。
1.3.3 DNA提取与功能基因定量PCR测定
为了揭示土壤N2O排放的微生物机制, 我们基于2014和2015年生长季旺盛期(8月)的土壤样品, 称取0.5 g鲜土, 采用FastDNA SPIN Kit for soil (MP Biomedical, Carlsbad, USA)试剂盒提取了土壤微生物的总DNA。提取的DNA原液采用NanoDrop? ND-2000c UV-Vis分光光度计(Thermo Fisher Scientific, Wilmington, USA)测定浓度与质量。在此基础上测定了AOA和AOB的amoA基因丰度。
土壤样品AOA和AOB的amoA基因丰度通过定量PCR反应检测。反应在StepOne Plus PCR System (Applied Biosystems, Carlsbad, USA)中进行。反应使用的引物分别为AOA: amoAF(5’-STAATGGTCTGGCTTAGACG-3’)/amoAR(5’-GCGGCCATCCATCTGTATGT-3’); AOB: amoA1F(5’-GGGGTTTCTACTGGTGGT-3’)/amoA2R(5’-CCCCTCKGSAAAGCCTTCTTC-3’)(Rotthauwe et al., 1997)。试剂选用SYBR? Premix ExTaqTM (Tli RNaseH Plus) (宝生物工程有限公司, 大连), 反应体系20 μL。对于AOA, 具体反应程序为: 95 ℃预变性30 s; 95 ℃变性10 s, 55 ℃退火30 s, 72 ℃延伸1 min, 40个循环。对于AOB, 具体反应程序为: 95 ℃预变性1 min; 94 ℃变性30 s, 57 ℃退火30 s, 72 ℃延伸1 min, 40个循环。标准曲线为分别含有AOA和AOB amoA基因质粒的10倍梯度稀释液, 曲线的决定系数(R2)在0.97以上, 反应的扩增效率在90%-110%之间。
1.4 统计分析
首先, 采用重复测量方差分析检验增温处理对土壤温度、土壤湿度以及N2O通量的效应, 其中利用主体间效应检验增温处理本身的影响, 利用主体内效应检验气体测定时间及测定时间与增温的交互效应的影响。其次, 分别计算土壤温度、含水量、土壤理化性质及功能基因和N2O通量平均值在增温处理与对照之间的差值, 并采用混合线性模型分析三者之间的关系。最后, 将AOA和AOB的amoA基因拷贝数进行log转化后进行配对t检验。上述统计分析利用软件R 3.4.0 (R Development Core Team, 2017)完成。2 研究结果
2.1 增温对土壤微环境的影响
实验期间研究地点的气候出现明显的年际变异(附件I)。2014和2015两年间气温相差不大, 两个生长季平均气温分别为7.4和7.3 ℃。但两年的降水量差异明显, 2014年降水量为556.8 mm, 超过1980- 2012年的平均值(387 mm), 而2015年降水量为416.5 mm, 接近平均值。两年的降水均集中在生长季, 其中2014和2015年生长季降水量分别为534.7和371.7 mm, 占全年降水量的96%和89%。此外, 两年的降水量表现出明显的季节动态(附件I)。增温处理显著改变了表层土壤的温湿度(图2)。其中, 增温处理导致表层0-10 cm土壤温度显著上升, 2014、2015年生长季的平均温度分别为15.4和15.0 ℃, 相对于对照分别增加了1.7 (p < 0.001)和1.6 ℃ (p < 0.01; 图2A), 两年非生长季的土壤温度分别增加了1.4 (p < 0.01)和1.2 ℃ (p < 0.01)。实验期间, 增温幅度存在昼夜变化: 白天的增温幅度为2.1 ℃, 夜间为1.1 ℃。同时, 增温处理导致土壤湿度显著下降, 2014、2015年生长季土壤的体积含水量分别为14.8%和10.3%, 相较于对照下降了2.5% (p < 0.001)和3.3% (p < 0.001; 图2B)。
图2
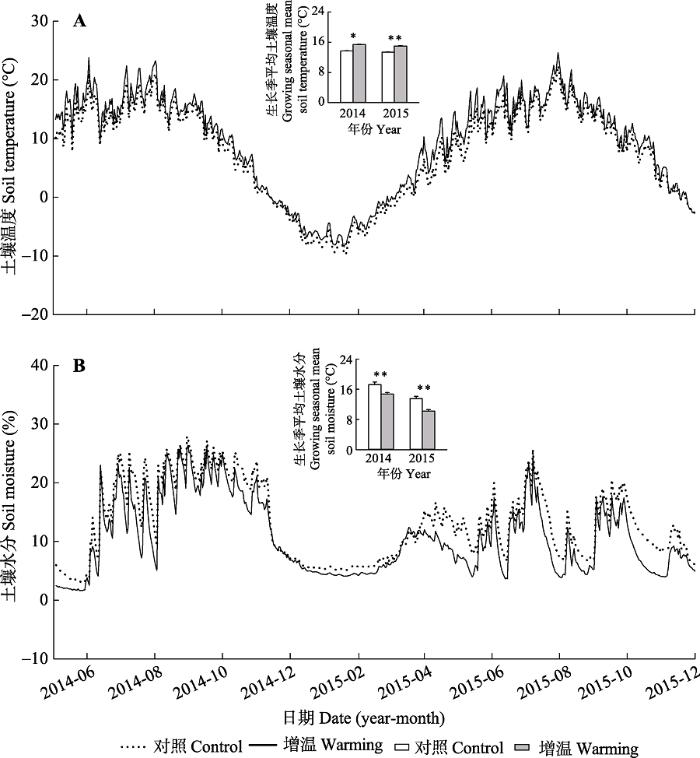
图2实验期间增温对0-10 cm土壤温度(A)和土壤含水率(B)的影响(平均值±标准误差)。*, p < 0.05; **, p < 0.01。
Fig. 2Warming effects on soil temperature (A) and soil moisture (B) at 0-10 cm depth during 2014-2015 (mean ± SE). *, p < 0.05; **, p < 0.01.
除了土壤温湿度以外, 开顶箱增温处理还显著改变了土壤的其他理化性质。增温导致两个生长季旺期的NH4+-N均显著增加(2014: p = 0.01; 2015: p < 0.001), NO3--N和SIN在2015年显著下降(NO3--N: p = 0.001; SIN: p = 0.04), MBC和MBN在2014年显著下降(MBC: p < 0.05; MBN: p < 0.001)。此外, 土壤无机氮与微生物碳氮含量存在较大的年际差异。除了NH4+-N之外, 2015年土壤NO3--N、SIN、MBC、MBN含量均低于2014年(表1)。
Table 1
Table 1Warming effects on soil physicochemical properties and microbial biomass (mean ± SE)
年份 Year | 处理 Treatment | 氨态氮 NH4+-N (mg·kg-1) | 硝态氮 NO3- -N (mg·kg-1) | 土壤无机氮 SIN (mg·kg-1) | 微生物生物量碳 MBC (mg·kg-1) | 微生物生物量氮 MBN (mg·kg-1) |
---|---|---|---|---|---|---|
2014 | 对照 Control | 1.8 ± 0.38 | 25.0 ± 1.6 | 26.8 ± 1.7 | 890.4 ± 23.7 | 91.6 ± 4.0 |
增温 Warming | 3.0 ± 0.60* | 23.5 ± 1.3 | 26.5 ± 1.5 | 801.9 ± 40.8* | 68.2 ± 5.8** | |
2015 | 对照 Control | 2.2 ± 0.25 | 5.96 ± 0.4 | 8.20 ± 0.5 | 732.6 ± 11.3 | 42.2 ± 1.5 |
增温 Warming | 3.0 ± 0.19* | 4.04 ± 0.4** | 7.02 ± 0.3* | 720.1 ± 18.6 | 41.3 ± 2.4 |
MBC, soil microbial biomass carbon; MBN, soil microbial biomass nitrogen; SIN, soil inorganic nitrogen. *, p < 0.05; **, p < 0.01.
新窗口打开|下载CSV
2.2 土壤N2O通量及功能基因对增温的响应
高寒草原生态系统生长季表现为N2O源, 2014和2015年对照内土壤N2O的生长季平均通量分别为3.23和1.47 μg·m-2·h-1。并且, 2014年生长季的土壤N2O通量表现出与土壤温度和水分状况相似的波动, 即呈现先上升后下降的趋势(图3A), 而在2015年生长季N2O通量没有明显的变化规律(图3B)。图3
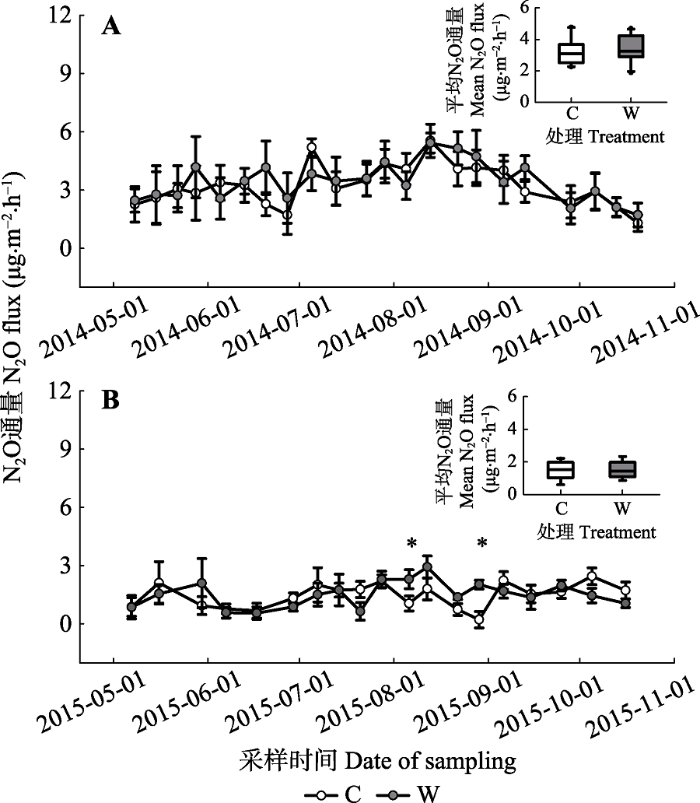
图32014(A)和2015(B)年生长季期间对照与增温处理下土壤的N2O通量(平均值±标准误差)。*, p < 0.05。C, 对照; W, 增温。
Fig. 3N2O fluxes under control and warming treatments during the growing seasons of 2014(A) and 2015 (B), (mean ± SE). *, p < 0.05. C, control; W, warming.
增温处理并没有显著改变两个生长季的土壤N2O通量(2014: p = 0.53; 2015: p = 0.83)。并且, 两个生长季中增温及其与采样日期的交互效应对土壤N2O通量影响也不显著(2014: p = 0.93; 2015: p = 0.25)。然而, 采样日期对土壤N2O通量存在显著影响(2014: p < 0.01; 2015: p < 0.01, 表2)。
Table 2
表2
表2基于重复测量方差分析得到的增温(W)、测定时间(T)及其交互作用(W × T)对土壤N2O通量影响
Table 2
来源 Source | 2014 | 2015 | ||||
---|---|---|---|---|---|---|
df | F | p | df | F | p | |
增温 Warming (W) | 1 | 0.41 | 0.53 | 1 | 0.05 | 0.83 |
日期 Date (T) | 22 | 2.16 | 0.00** | 18 | 2.05 | 0.00** |
T × W | 22 | 0.40 | 0.99 | 18 | 1.32 | 0.17 |
新窗口打开|下载CSV
2014年生长季, 增温处理表层土壤中AOA和AOB amoA基因的拷贝数分别为5.0 × 107和4.7 × 105拷贝·g-1, 2015年生长季对应的数值分别为15.2 × 107和10.0 × 105拷贝·g-1 (图4)。AOA高出AOB两个数量级, 但AOA和AOB的基因丰度在增温处理与对照之间没有显著差异(2014年, AOA: p = 0.87; AOB: p = 0.38; 2015年, AOA: p = 0.55; AOB: p = 0.61)。
图4
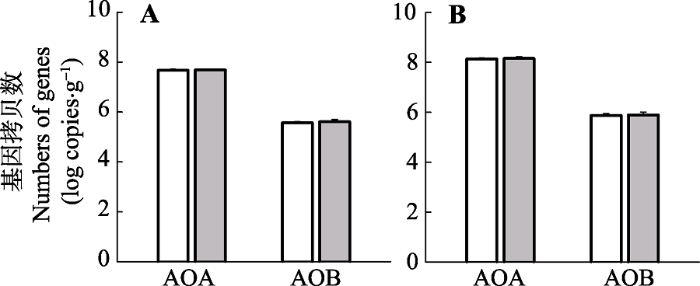
图42014 (A)和2015 (B)年生长季期间增温对AOA与AOB的amoA基因丰度的影响(平均值±标准误差)。AOA, 氨氧化古菌; AOB, 氨氧化细菌。
Fig. 4Warming effects on the abundance of AOA-amoA and AOB-amoA during the growing seasons of 2014 (A) and 2015 (B) (mean ± SE). AOA, ammonia-oxidizing archaea; AOB, ammonia-oxidizing bacteria.
2.3 土壤N2O通量的变化与土壤温度、水分变化之间的关系
增温引起的土壤温度变化量与土壤水分变化量之间呈显著负相关关系(r = -0.37, p < 0.05; 图5A), 说明土壤温度增加幅度越大, 相应的土壤含水量下降越大, 也意味着增温的确导致了土壤干旱。增温导致的土壤N2O通量变化量与土壤温度变化量之间没有显著相关关系(p = 0.69, 图5B), 但土壤N2O通量的变化量与土壤水分变化量之间显著正相关(r = 0.46, p < 0.01; 图5C)。图5
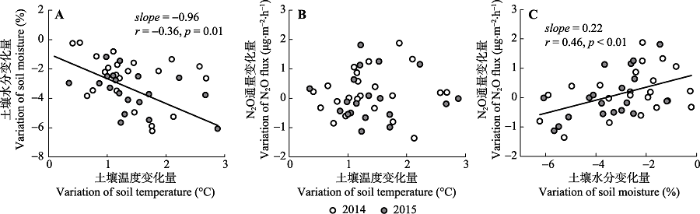
图5增温引起的土壤N2O通量的变化量(增温-对照)与土壤温度的变化量(增温-对照)、土壤水分的变化量(增温-对照)之间的关系。A, 土壤水分与土壤温度。B, N2O与土壤温度。C, N2O与土壤水分。
Fig. 5Relationships among warming induced changes (warming-control) in soil N2O fluxes, soil temperature and soil moisture. A, soil moisture and temperature; B, N2O and soil temperature; C, N2O and soil moisture.
3 讨论
本研究结果表明, 增温并没有显著改变青藏高原高寒草原土壤N2O通量。一般来讲, 作为硝化和反硝化等生物地球化学过程的产物, 土壤N2O通量会受到土壤温度变化的影响。以往的研究显示, 土壤温度的上升会促进土壤N矿化, 提高土壤无机氮的含量, 为硝化和反硝化过程提供更充足的底物(Shi et al., 2012)。同时, 增温会提高硝化和反硝化酶及相关微生物的活性, 尤其是硝化作用的酶系, 进而促进土壤N循环(Cantarel et al., 2012), 提高N2O的产生量, 此效应在高寒地区更加明显(Brzostek et al., 2012; Wang et al., 2016)。然而, 本文的结果显示, 尽管增温后土壤NH4+-N含量增加, 但增温并没有显著改变N2O通量(表2), 且土壤N2O通量的变化量与土壤温度的变化量之间也没有显著的相关关系(图5A), 这可能是因为增温对土壤性质和地上植被的影响不利于N2O排放(Ward et al., 2013; Maljanen et al., 2017)。增温会导致土壤有机碳含量与质量下降(黄锦学等, 2017), 进而影响硝化和反硝化微生物的能量获取, 导致N2O的产生受到限制(Gu et al., 2017)。同时, 增温引起植物群落中浅根系物种减少, 深根系物种增多, 进而对土壤无机氮的吸收量增加(Stewart et al., 2012), 与微生物竞争N素底物(Chapin III et al., 2011), 进而抑制了土壤N2O的产生。本研究的结果显示, 增温处理引起土壤NH4+增加及NO3-、SIN、MBC、MBN等因素下降, 然而土壤N2O通量与NH4+、NO3-、SIN、MBC、MBN等因素之间并没有显著的相关关系(附件III)。由此可见, 虽然氨氮和硝氮的含量在增温处理与对照间存在显著差异, 但这些因素并没有直接影响高寒草原生态系统土壤N2O通量。本研究结果显示, 土壤含水量对高寒草原土壤N2O通量起着明显的调控作用。增温导致了土壤含水量的显著下降(图2B), 且土壤干旱越严重的小区内增温引起的土壤N2O通量增量越小, 甚至转为负增长, 表明水分对土壤N2O排放有直接影响。那么, 土壤含水量调控N2O排放的可能途径是什么呢?土壤干旱主要通过影响硝化和反硝化过程的底物和相关微生物作用于对N2O的产生和排放。一方面, 土壤水分减少会直接降低NH4+溶解量, 进而限制其扩散, 导致N可利用性下降, 影响硝化作用微生物对N的正常获取(Bijoor et al., 2008), 因此N2O的产生会间接受到干旱效应的抑制。另一方面, 当土壤环境中NO3-含量较低时, N2O会取代NO3-作为电子受体, 参与到反硝化作用中还原为N2 (Stewart et al., 2012), 而含水量的降低会提高土壤通气性, 促进N2O扩散, 增强了N2O被还原为N2的过程(Lydie et al., 2007)。此外, 土壤含水量的下降还会抑制主导N循环过程的功能微生物对增温响应, 进而削弱土壤N2O排放对增温的响应(Szukics et al., 2010; Daebeler et al., 2017)。与这个假说一致, 我们的结果也显示增温并没有改变AOA和AOB的amoA基因丰度, 可能是因为土壤干旱限制了功能微生物的活性(Maestre et al., 2015)。一般来讲, 温度上升对微生物的活性有促进作用(Sistla et al., 2014; 王文立等, 2015), 然而当土壤含水量过低时增温的促进效应会被掩盖。野外观测和室内培养实验的证据均支持这一推论。对半干旱生态系统的研究表明, 土壤含水量对微生物活性的影响比土壤温度更强, 干旱会抵消温度上升对微生物活性的促进效应(Hu et al., 2017)。室内培养实验的结果也进一步证实, 当土壤含水量低于30%土壤孔隙水含量(WFPS)时AOA和AOB对增温的响应会受到明显限制, 表现为不同增温水平的amoA基因丰度没有发生显著改变, 而相应的N2O产生量也没有显著差异(Szukics et al., 2010)。
本研究结果表明, 高寒草原土壤N2O通量呈现明显的年际变化。在两年观测期间, 2014年生长季降水量较为丰沛(534.7 mm), 而2015年生长季降水量明显偏低(371.7 mm), 导致土壤含水量明显低于2014年(图2B)。虽然两个生长季中土壤温度没有差异(图2A), 但2015年生长季的土壤N2O通量依然出现明显下降(1.47 μg·m-2·h-1), 显著低于2014年生长季的对应值(3.23 μg·m-2·h-1)。按照以往的研究, 降雨量和土壤含水量对土壤性质和微生物影响较大, 土壤水分改善后有利于土壤的矿化作用, 提高土壤无机氮的含量(Chapin III et al., 2011), 并且提高微生物的生物量(王文立等, 2015)。与以往的研究一致, 本研究中同样发现降水丰沛的2014年土壤NO3- -N和SIN含量高于2015年的对应值(NO3- -N: 25.0 vs. 6.0 mg·kg-1; SIN: 26.8 vs. 8.2 mg·kg-1, 表1), 且土壤微生物的生物量也呈现相似的现象(MBC: 890.4 vs. 732.6 mg·kg-1; MBN: 91.6 vs. 42.2 mg·kg-1, 表1), 可以部分解释N2O的年际差异。然而, 功能基因丰度并不能很好地解释土壤N2O通量的年际变异。土壤微生物功能基因的结果显示, 2014年AOA和AOB的基因丰度显著低于2015年(AOA: 5.0 × 107vs. 15.2 × 105 拷贝·g-1; AOB: 15.2 × 107vs. 10.0 × 105 拷贝·g-1, 图4), 这一差异与土壤N2O通量的年际差异趋势相反。总体而言, 环境条件差异, 尤其是降水波动是导致N2O通量年际变异的主要因素。
4 结论
本研究利用野外增温控制实验, 通过连续两个生长季的测定, 发现增温并没有改变高寒草原生态系统N2O的通量。原因是增温会引发显著的土壤干旱, 使得土壤含水量成为影响硝化作用底物与功能微生物的主要因素, 抵消增温对土壤N2O排放的促进作用。因此, 未来评估全球变暖背景下土壤N2O排放量时需要考虑增温引发的土壤干旱等间接效应。特邀编委: 陈 槐 责任编辑: 李 敏
附件
附件I
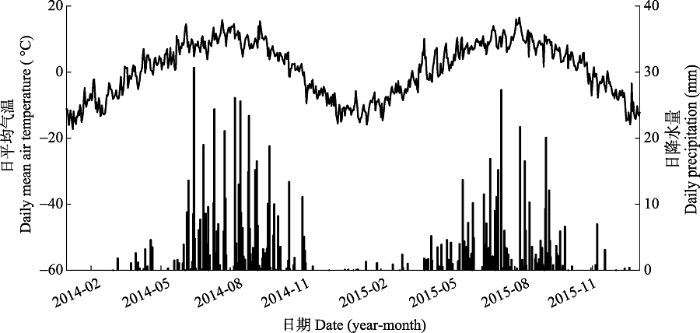
附件I2014-2015年间生长季日平均气温(折线图)和日降水量(柱状图)
Appendix IDaily mean air temperature (lines) and daily precipitation (bars) during 2014-2015 at our experiment site
附件II
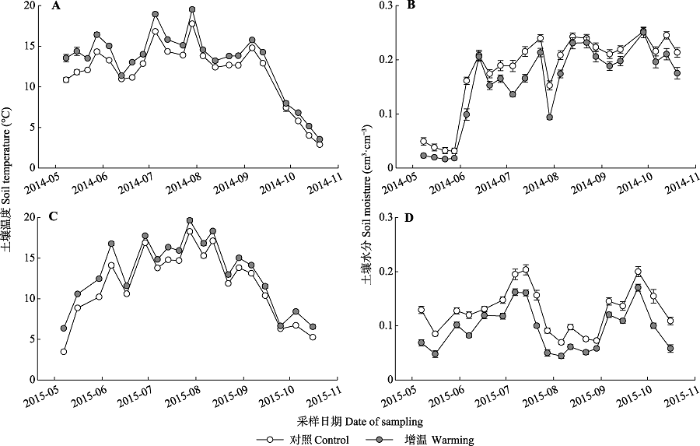
附件II2014-2015年生长季对照与增温处理下土壤的温度和含水量
A, 2014年土壤温度。B, 2015年土壤温度。C, 2014年土壤含水量。D, 2015年土壤含水量。
Appendix IISoil temperature and moisture in control and warming treatments during the growing seasons
A, Soil temperature in 2014. B, Soil temperature in 2015. C, Soil moisture in 2014. D, Soil moisture in 2015.
附件III
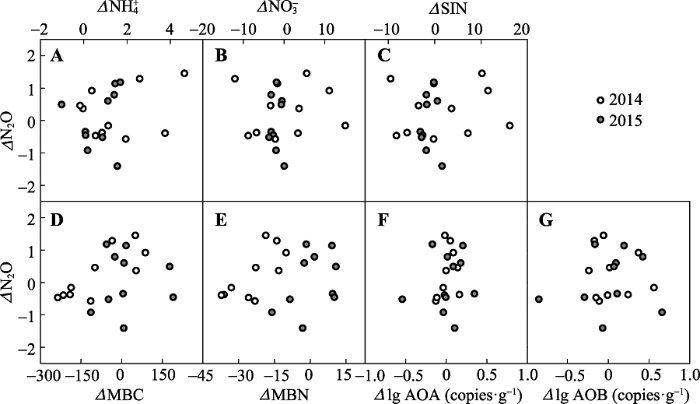
附件III增温导致的土壤N2O通量的变化(增温-对照)与土壤因素及功能基因的变化(增温-对照)、微生物属性的变化(增温-对照)之间的关系
A, NH4+ -N。B, NO3--N。C, 微生物量碳。D, 微生物量氮。E, 土壤无机氮含量。F, 氨氧化古菌。G, 氨氧化细菌。
Appendix IIIRelationships of changes in soil N2O flux with changes in edaphic variables, microbial properties, AOA and AOB
A, NH4+-N. B, NO3--N. C, Microbial biomass carbon (MBC). D, Microbial biomass nitrogen (MBN). E, Soil inorganic carbon (SIN). F, Ammonia-oxidizing archaea (AOA). G, Ammonia-oxidizing bacteria (AOB).
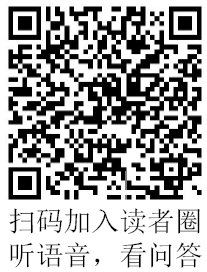
参考文献 原文顺序
文献年度倒序
文中引用次数倒序
被引期刊影响因子
DOI:10.1111/j.1365-2486.2008.01617.xURL [本文引用: 1]

Abstract We examined the influence of temperature and management practices on the nitrogen (N) cycling of turfgrass, the largest irrigated crop in the United States. We measured nitrous oxide (N 2 O) fluxes, and plant and soil N content and isotopic composition with a manipulative experiment of temperature and fertilizer application. Infrared lamps were used to increase surface temperature by 3.5 1.3 C on average and control and heated plots were split into high and low fertilizer treatments. The N 2 O fluxes increased following fertilizer application and were also directly related to soil moisture. There was a positive effect of warming on N 2 O fluxes. Soils in the heated plots were enriched in nitrogen isotope ratio ( 15 N) relative to control plots, consistent with greater gaseous losses of N. For all treatments, C 4 plant C/N ratio was negatively correlated with plant 15 N, suggesting that low leaf N was associated with the use of isotopically depleted N sources such as mineralized organic matter. A significant and unexpected result was a large, rapid increase in the proportion of C 4 plants in the heated plots relative to control plots, as measured by the carbon isotope ratio (13 C) of total harvested aboveground biomass. The C 4 plant biomass was dominated by crabgrass, a common weed in C 3 fescue lawns. Our results suggest that an increase in temperature caused by climate change as well as the urban heat island effect may result in increases in N 2 O emissions from fertilized urban lawns. In addition, warming may exacerbate weed invasions, which may require more intensive management, e.g. herbicide application, to manage species composition.
DOI:10.1016/0038-0717(85)90144-0URL [本文引用: 1]

A new “direct extraction” method for measuring soil microbial biomass nitrogen (biomass N) is described. The new method (fumigation-extraction) is based on CHC1 3 fumigation, followed by immediate extraction with 0.5 M K 2SO 4 and measurement of total N released by CHC1 3 in the soil extracts. The amounts of NH 4-N and total N extracted by K 2SO 4 immediately after fumigation increased with fumigation time up to 5 days. Total N released by CHC1 3 after 1 day fumigation (1 day CHC1 3-N) and after 5 days fumigation (5 day CHC1 3-N) were positively correlated with the flush of mineral N (F N) in 37 soils that had been fumigated, the fumigant removed and the soils incubated for 10 days (fumigation-incubation). The regression equations were 1 day CHC1 3-N = (0.79 ± 0.022) F N and 5 day CHC1 3-N = (1.01 ± 0.027) F N, both regressions accounting for 92% of the variance in the data. In field soils previously treated with 15N-labelled fertilizer, the amounts of labelled N, measured after fumigation-extraction, were very similar to the amounts of labelled N mineralized during fumigation-incubation; both were about 4 times as heavily labelled as the soil N as a whole. These results suggest that fumigation-extraction and fumigation-incubation both measure the same fraction of the soil organic N (probably the cytoplasmic component of the soil microbial biomass) and that measurement of the total N released by CHC1 3 fumigation for 24 h provides a rapid method for measuring biomass N.
DOI:10.1111/j.1365-2486.2012.02685.xURL [本文引用: 1]

Nitrogen regulates the Earth's climate system by constraining the terrestrial sink for atmospheric CO2. Proteolytic enzymes are a principal driver of the within-system cycle of soil nitrogen, yet there is little to no understanding of their response to climate change. Here, we use a single methodology to investigate potential proteolytic enzyme activity in soils from 16 global change experiments. We show that regardless of geographical location or experimental manipulation (i.e., temperature, precipitation, or both), all sites plotted along a single line relating the response ratio of potential proteolytic activity to soil moisture deficit, the difference between precipitation and evapotranspiration. In particular, warming and reductions in precipitation stimulated potential proteolytic activity in mesic sites – temperate and boreal forests, arctic tundra – whereas these manipulations suppressed potential activity in dry grasslands. This study provides a foundation for a simple representation of the impacts of climate change on a central component of the nitrogen cycle.
DOI:10.1098/rstb.2013.0122URL [本文引用: 1]
DOI:10.1111/j.1365-2486.2012.02692.xURL [本文引用: 1]

Emissions of the trace gas nitrous oxide (N2O) play an important role for the greenhouse effect and stratospheric ozone depletion, but the impacts of climate change on N2O fluxes and the underlying microbial drivers remain unclear. The aim of this study was to determine the effects of sustained climate change on field N2O fluxes and associated microbial enzymatic activities, microbial population abundance and community diversity in an extensively managed, upland grassland. We recorded N2O fluxes, nitrification and denitrification, microbial population size involved in these processes and community structure of nitrite reducers (nirK) in a grassland exposed for 4 years to elevated atmospheric CO2 (+200 ppm), elevated temperature (+3.5 C) and reduction of summer precipitations (20%) as part of a long-term, multifactor climate change experiment. Our results showed that both warming and simultaneous application of warming, summer drought and elevated CO2 had a positive effect on N2O fluxes, nitrification, N2O release by denitrification and the population size of N2O reducers and NH4 oxidizers. In situN2O fluxes showed a stronger correlation with microbial population size under warmed conditions compared with the control site. Specific lineages of nirK denitrifier communities responded significantly to temperature. In addition, nirK community composition showed significant changes in response to drought. Path analysis explained more than 85% of in situN2O fluxes variance by soil temperature, denitrification activity and specific denitrifying lineages. Overall, our study underlines that climate-induced changes in grassland N2O emissions reflect climate-induced changes in microbial community structure, which in turn modify microbial processes.
[本文引用: 3]
DOI:10.2307/1940405URL [本文引用: 1]

In undisturbed arctic tussock and wet meadow tundras we increased air temperature with a plastic greenhouse, increased nutrient availability by NPK fertilization, and decreased light intensity with shade cloth to determine the factors limiting growth of tundra plants. After 2 yr of these manipulations we measured growth of each major vascular species and one moss species. Each species showed a different pattern of growth response to alteration of light, air temperature, and nutrient regimes, indicating that no single factor limits growth of all species in these communities. Growth of canopy species (Betula nana, Ledum palustre, Carex bigelowii, and Eriophorum vaginatum) was reduced by experimental shading more than was growth of understory species (e.g., Vaccinium vitisidaea and Rubus chamaemorus). Species typical of nutrient-rich sites (Betula nana, Rubus chamaemorus, and Polygonum bistorta) generally responded more to nutrient addition than did species typical of nutrient-poor sites (e.g., Empetrum nigrum), although there were species characteristic of fertile sites (Salix pulchra) and infertile sites (Ledum palustre) which did not show this pattern of nutrient response. Species that grow in warm hollows between tussocks showed less growth in response to increased air temperature than did canopy species. We suggest that lack of a single common factor limiting growth of all species in tussock and wet meadow tundras implies that (1) each species is individualistically distributed, as described by the continuum model of community organization, (2) as a result of competition and/or distinct evolutionary histories, the growth of each species is limited by a different combination of environmental factors, and (3) production by individual species varies greatly from year to year, but production by the whole vegetation is more stable, because years that are favorable for growth of some species cause a compensatory decrease in growth of other species.
DOI:10.1111/gcb.2007.13.issue-1URL [本文引用: 1]
DOI:10.1111/gcb.12277URLPMID:23744573 [本文引用: 1]

With a pace of about twice the observed rate of global warming, the temperature on the Qinghai-Tibetan Plateau (Earth's hird pole) has increased by 0.2 C per decade over the past 50 years, which results in significant permafrost thawing and glacier retreat. Our review suggested that warming enhanced net primary production and soil respiration, decreased methane (CH4) emissions from wetlands and increased CH4 consumption of meadows, but might increase CH4 emissions from lakes. Warming-induced permafrost thawing and glaciers melting would also result in substantial emission of old carbon dioxide (CO2) and CH4. Nitrous oxide (N2O) emission was not stimulated by warming itself, but might be slightly enhanced by wetting. However, there are many uncertainties in such biogeochemical cycles under climate change. Human activities (e.g. grazing, land cover changes) further modified the biogeochemical cycles and amplified such uncertainties on the plateau. If the projected warming and wetting continues, the future biogeochemical cycles will be more complicated. So facing research in this field is an ongoing challenge of integrating field observations with process-based ecosystem models to predict the impacts of future climate change and human activities at various temporal and spatial scales. To reduce the uncertainties and to improve the precision of the predictions of the impacts of climate change and human activities on biogeochemical cycles, efforts should focus on conducting more field observation studies, integrating data within improved models, and developing new knowledge about coupling among carbon, nitrogen, and phosphorus biogeochemical cycles as well as about the role of microbes in these cycles.
DOI:10.1016/j.apsoil.2013.03.006URL [本文引用: 1]

As the first and rate-limiting step of nitrification, ammonia oxidation can be realized either by ammonia-oxidizing bacteria (AOB) or archaea (AOA). However, the key factors driving the abundance, community structure and activity of ammonia oxidizers are still unclear, and the relative importance of AOA and AOB in ammonia oxidation is unresolved. In the present study, we examined the effects of long-term (6 years) nitrogen (N) addition and simulated precipitation increment on the abundance and community composition of AOA and AOB based on a field trial in a typical temperate steppe of northern China. We used combined approaches of quantitative PCR, terminal-restriction fragment length polymorphism (T-RFLP) and clone library analyses of amoA genes. The study objective was to determine (1) AOA and AOB diversity and activity in response to N addition and increased precipitation and (2) the relative contributions of AOA and AOB to soil ammonia oxidation in the typical temperate steppe. The results showed that the potential nitrification rate (PNR) increased with N addition, but decreased with increased precipitation. Both N addition and increased precipitation significantly increased AOB but not AOA abundance, and a significant correlation was only observed between PNR and AOB amoA gene copies. The T-RFLP analysis showed that both N and precipitation were key factors in shaping the composition of AOB, while AOA were only marginally influenced. Phylogenetic analysis indicated that all AOA clones fell within the soil and sediment lineage while all AOB clones fell within the Nitrosospira. The study suggested that AOA and AOB had distinct physiological characteristics and ecological niches. AOB were shown to be more sensitive to N and precipitation than AOA, and the ammonia oxidation process was therefore supposed to be mainly driven by AOB in this temperate steppe. (C) 2013 Elsevier B.V. All rights reserved.
DOI:10.1016/j.soilbio.2016.12.013URL [本文引用: 1]

N mineralization and nitrification showed higher sensitivities in response to short-term temperature changes if the soils had been warmed. In part, the influence of short-term temperature changes could however be neutralized by the effects of N fertilization. Long-term N fertilization alone affected only gross N mineralization. However, all gross N transformation rates were significantly altered by the interactive effects of N fertilization and soil warming. We conclude that in order to reliably predict effects of global change on sub-arctic soil N transformation processes we need to consider multiple interactions among global change factors and to take into account the capacity of soil microbial populations to adapt to global change conditions.
DOI:10.1111/gcb.13257URLPMID:26913840 [本文引用: 1]

Abstract The permafrost organic carbon (OC) stock is of global significance because of its large pool size, and the potential positive feedback to climate warming. However, due to the lack of systematic field observations and appropriate upscaling methodologies, substantial uncertainties exist in the permafrost OC budget, which limits our understanding of the fate of frozen carbon in a warming world. In particular, the lack of comprehensive estimates of OC stocks across alpine permafrost means that current knowledge on this issue remains incomplete. Here we evaluated the pool size and spatial variations of permafrost OC stock to 3 metres depth on the Tibetan Plateau by combining systematic measurements from a substantial number of pedons (i.e., 342 three-metre-deep cores and 177 50-cm-deep pits) with a machine learning technique (i.e., support vector machine, SVM). We also quantified uncertainties in permafrost carbon budget by conducting a Monte Carlo simulation. Our results revealed that the combination of systematic measurements with the SVM model allowed spatially explicit estimates to be made. The OC density (OC amount per unit area, OCD) exhibited a decreasing trend from the southeastern to the northwestern plateau, with the exception that OCD in the swamp meadow was substantially higher than that in surrounding regions. Our results also demonstrated that Tibetan permafrost stored a large amount of OC in the top 3 metres, with the median OC pool size being 15.31 Pg C (interquartile range: 13.03-17.77 Pg C). 44% of OC occurred in deep layers (i.e., 100-300 cm), close to the proportion observed across the northern circumpolar permafrost region. The large carbon pool size, together with significant permafrost thawing suggests a risk of carbon emissions and positive climate feedback across the Tibetan alpine permafrost region. This article is protected by copyright. All rights reserved. This article is protected by copyright. All rights reserved.
DOI:10.1016/j.agee.2006.12.024URL [本文引用: 1]

Soil/atmosphere exchange fluxes of nitrous oxide were monitored for a 3-year period at 10 grassland sites in eight European countries (Denmark, France, Hungary, Ireland, Italy, The Netherlands, Switzerland and United Kingdom), spanning a wide range of climatic, environmental and soil conditions. Most study sites investigated the influence of one or several management practices on N 2 O exchange, such as nitrogen fertilization and grazing intensity. Fluxes were measured using non-steady state chambers at most sites, and alternative measurement techniques such as eddy covariance and fast-box using tunable diode laser spectroscopy were implemented at some sites. The overall uncertainty in annual flux estimates derived from chamber measurements may be as high as 50% due to the temporal and spatial variability in fluxes, which warrants the future use of continuous measurements, if possible at the field scale. Annual emission rates were higher from intensive than from extensive grasslands, by a factor 4 if grazed (1.77 versus 0.4802kg02N 2 O-N02ha 611 02year 611 ) and by a factor 3 if ungrazed (0.95 versus 0.3202kg02N 2 O-N02ha 611 02year 611 ). Annual emission factors for fertilized systems were highly variable, ranging from 0.01% to 3.56%, but the mean emission factor across all sites (0.75%) was substantially lower than the IPCC default value of 1.25%. Emission factors for individual fertilization events increased with soil temperature and were generally higher for water-filled pore space values in the range 60–90%, though precipitation onto dry soils was also shown to lead to high losses of N 2 O-N from applied fertilizer. An empirical, multiple regression model to predict N 2 O emission factors on the basis of soil temperature, moisture and rainfall is developed, explaining half of the variability in observed emission factors.
DOI:10.1016/j.scitotenv.2017.03.280URLPMID:28390314 [本文引用: 1]

The primary aims of this study were to (i) quantify the variations in nitrous oxide (N 2 O) emissions and soil organic carbon (SOC) sequestration rates under winter wheat-summer maize cropping systems in Guanzhong Plain and (ii) evaluate the impact of organic amendments on greenhouse gas mitigation over a long-term period. We measured N 2 O fluxes during the maize season in 2015 under four fertilizer regimes in a long-term fertilization experiment. Soil was treated with only synthetic fertilizers in the maize season and with synthetic fertilizers, synthetic fertilizers plus crop residues and synthetic fertilizers plus low and high levels of dairy manure in the winter wheat season from 1990. The SOC content (0–2002cm) was collected annually at the same site between 1990 and 2015. Synthesis of our measurements and previous observations (between 2000 and 2009) within the investigated agricultural landscape revealed that cumulative N 2 O emissions increased with the SOC content following natural logarithm models during both the maize and winter wheat seasons (r 2 02>020.77, p02020.74, p02<020.001), indicating that all the fertilizer treatments in this study sequestered SOC. By applying these regression models, we estimated that the two manure-amended treatments accumulated a negative global warming potential (ranging from 61021.9 to 610212.902t02CO 2 -equivalent02ha 61021 ) over the past 2502years. However, this benefit would most likely be offset by high N 2 O emissions at saturated SOC levels before 2020. Our estimates suggest that organic amendments may not be efficient for greenhouse gas mitigation in Guanzhong Plain over a long-term period. We recommend efforts to inhibit N 2 O production via denitrification as being critical to resolving the conflict between SOC sequestration and N 2 O emissions.
URL [本文引用: 1]

由微生物推动的反硝化作用是地球氮素循环的重要分支,尽管已被发现广泛存在于细菌、真菌和古生菌中,其功能基因的研究仍仅限于很少几个物种。现代分子生物学的发展为研究环境微生物提供了行之有效的方法,以反硝化功能基因作为分子标记的分子生态学研究迅猛发展。综述近年来国内外微生物反硝化功能基因研究及以其为标记的分子生态学研究进展。
URL [本文引用: 1]

由微生物推动的反硝化作用是地球氮素循环的重要分支,尽管已被发现广泛存在于细菌、真菌和古生菌中,其功能基因的研究仍仅限于很少几个物种。现代分子生物学的发展为研究环境微生物提供了行之有效的方法,以反硝化功能基因作为分子标记的分子生态学研究迅猛发展。综述近年来国内外微生物反硝化功能基因研究及以其为标记的分子生态学研究进展。
DOI:10.1111/1462-2920.13795URLPMID:28493368 [本文引用: 1]

Abstract Globally, drylands represent the largest terrestrial biome and are projected to expand by 23% by the end of this century. Drylands are characterized by extremely low levels of water and nutrients and exhibit highly heterogeneous distribution in plants and biocrusts which make microbial processes shaping the dryland functioning rather unique compared with other terrestrial ecosystems. Nitrous oxide (N 2 O) is a powerful greenhouse gas with ozone depletion potential. Despite of the pivotal influences of microbial communities on the production and consumption of N 2 O, we have limited knowledge of the biological pathways and mechanisms underpinning N 2 O emissions from drylands, which are estimated to account for 30% of total gaseous nitrogen emissions on Earth. In this article, we describe the key microbial players and biological pathways regulating dryland N 2 O emissions, and discuss how these processes will respond to emerging global changes such as climate warming, extreme weather events, and nitrogen deposition. We also provide a conceptual framework to precisely manipulate the dryland microbiome to mitigate N 2 O emissions in situ using emerging technologies with great specificity and efficacy. These cross-disciplinary efforts will enable the development of novel and environmental-friendly microbiome-based solutions to future mitigation strategies of climate change. This article is protected by copyright. All rights reserved. 2017 Society for Applied Microbiology and John Wiley & Sons Ltd.
DOI:10.1016/j.soilbio.2010.02.011URL [本文引用: 1]

A great deal of uncertainty is associated with estimates of global nitrous oxide (N 2 O) emissions because emissions from arid and polar climates were not included in the estimates due to a lack of available data. In particular, very few studies have assessed the response of N 2 O flux to grazing under future warming conditions. This experiment was conducted to determine the effects of warming and grazing on N 2 O flux at different time scales for three years under a controlled warming-grazing system. A free-air temperature enhancement system (FATE) using infrared heaters and grazing significantly increased soil temperatures for both of growing (average 1.8°C in 2008) and no-growing seasons (average 3.0°C for 3-years) within 20-cm depth, but only warming reduced soil moisture at 10-cm soil depth during the growing season during the drought year of 2008. Generally, the effects of warming and grazing on N 2 O flux varied with sampling date, season, and year. No interactive effect between warming and grazing was found. Warming did not affect annual N 2 O flux when grazing was moderate during the growing season because the tradeoff of the effect of warming on N 2 O flux was observed between the growing season and no-growing season. No-warming with grazing (NWG) and warming with grazing (WG) significantly increased the average annual N 2 O flux (57.8 and 31.0%) compared with no-warming with no-grazing (NWNG) and warming with no-grazing (WNG), respectively, indicating that warming reduced the response of N 2 O flux to grazing in the region. Winter accounted for 36–57% of annual N 2 O flux for NWNG and NWG, whereas only for 5–8% of annual N 2 O flux for WNG and WG. Soil temperature could explain 5–35% of annual N 2 O flux variation.
DOI:10.5846/stxb201607261526URL [本文引用: 1]

全球变暖的大背景下,土壤作为陆地生态系统中最大碳汇的载体,其微小变化都会引起大气CO2浓度显著的改变.土壤有机碳对气候变化的响应和适应对于预测未来气候变化具有十分重要的作用.然而,目前增温对土壤有机碳的影响及其影响机制仍存诸多未解决的问题.综述了目前土壤有机碳矿化的研究方式及增温对土壤有机碳矿化影响的国内外研究进展.结果发现增温往往会促进土壤有机碳排放,主要源于土壤微生物代谢活性或群落组成的改变.同时该排放强度因生态系统类型、增温方式和幅度以及增温季节和持续时间的不同而存在巨大差异,且长期增温反而使土壤微生物产生适应及驯化现象,从而降低或缓解陆地生态系统对全球变暖的正反馈效应.但这些结果大都基于温带实验,而原位增温实验对高生产力、多样性丰富的热带亚热带地区的影响是否与温带一致仍待进一步考证.室内模拟实验虽可深入研究温度对土壤有机碳矿化的影响机制,却无法真实反映野外自然环境.同时,野外增温方式及室内研究方式的多样均降低不同研究之间的可比性,进而难以预估由实验方法本身差异引起的结果变异.
DOI:10.5846/stxb201607261526URL [本文引用: 1]

全球变暖的大背景下,土壤作为陆地生态系统中最大碳汇的载体,其微小变化都会引起大气CO2浓度显著的改变.土壤有机碳对气候变化的响应和适应对于预测未来气候变化具有十分重要的作用.然而,目前增温对土壤有机碳的影响及其影响机制仍存诸多未解决的问题.综述了目前土壤有机碳矿化的研究方式及增温对土壤有机碳矿化影响的国内外研究进展.结果发现增温往往会促进土壤有机碳排放,主要源于土壤微生物代谢活性或群落组成的改变.同时该排放强度因生态系统类型、增温方式和幅度以及增温季节和持续时间的不同而存在巨大差异,且长期增温反而使土壤微生物产生适应及驯化现象,从而降低或缓解陆地生态系统对全球变暖的正反馈效应.但这些结果大都基于温带实验,而原位增温实验对高生产力、多样性丰富的热带亚热带地区的影响是否与温带一致仍待进一步考证.室内模拟实验虽可深入研究温度对土壤有机碳矿化的影响机制,却无法真实反映野外自然环境.同时,野外增温方式及室内研究方式的多样均降低不同研究之间的可比性,进而难以预估由实验方法本身差异引起的结果变异.
[本文引用: 3]
DOI:10.1029/94GB01660URLPMID:4518811 [本文引用: 1]

We investigated changes in soil-atmosphere flux of CH4, N2O, and NO resulting from the succession of pasture to forest in the Atlantic lowlands of Costa Rica. We studied a dozen sites intensively for over one year in order to measure rates and to understand controlling mechanisms for gas exchange. CH4 flux was controlled primarily by soil moisture content. Soil consumption of atmospheric CH4 was greatest when soils were relatively dry. Forest soils consumed CH4 while pasture soils which had poor drainage generally produced CH4. The seasonal pattern of N2O emissions from forest soils was related exponentially to soil water-filled pore space. Annual average N2O emissions correlated with soil exchangeable NO3 concentrations. Soil-atmosphere NO flux was greatest when soils were relatively dry. We found the largest NO emissions from abandoned pasture sites. Combining these data with those from another study in the Atlantic lowlands of Costa Rica that focused on deforestation, we present a 50-year chronosequence of trace gas emissions that extends from natural conditions, through disturbance and natural recovery. The soil-atmosphere fluxes of CH4 and N2O and of NO may be restored to predisturbance rates during secondary succession. The changes in trace gas emissions following deforestation, through pasture use and secondary succession, may be explained conceptually through reference to two major controlling factors, nitrogen availability and soil-atmosphere diffusive exchange of gases as it is influenced by soil moisture content and soil compaction.
DOI:10.1016/j.agee.2015.12.013URL [本文引用: 1]

Nitrous oxide (N2O) emissions from agricultural soils play an important role in the global greenhouse gas budget. However, the response of N2O emissions from nitrogen fertilized agricultural soils to climate warming is not yet well understood. A field experiment with simulated warming (T) using infrared heaters and its control (C) combined with a nitrogen (N1) fertilization treatment (315kgNha611y611) and no nitrogen treatment (N0) was conducted over five years at an agricultural research station in the North China Plain in a winter wheat–soybean double cropping system. N2O fluxes were measured using static chambers about once every week during July 2009–June 2014. In the N1 treatment, warming decreased the soil moisture and N2O emissions in spring, autumn and winter and the annual cumulative emissions. Across all years, N2O fluxes were positively correlated with soil temperature and soil moisture. The effect of lower soil moisture on N2O fluxes exceeded that of higher temperature, leading to less N2O being released by the drier soils under warming. Nitrogen fertilizer increased N2O emissions without warming, but did not routinely increase N2O emissions under warming treatment. In the N0 treatment, warming neither decreased soil water content nor N2O emissions. Temperature and nitrogen input had significant direct and antagonistic effects on cumulative N2O flux in the N1 treatment. The decrease in N2O emissions from N1Twas due to the significant decrease of soil water content, soil total nitrogen and organic matter, which consequently accelerated N cycle dynamics and advanced wheat growth.
DOI:10.1029/2007JG000610URL [本文引用: 1]

[1] 02The stable carbon isotope composition ( δ 13C) of tree rings in a climate-sensitive region can provide a retrospective view of changes in environment and climate. Here, we report on the development of the first annually resolved δ 13C tree ring chronology obtained from natural forests on the northeastern Tibetan Plateau. Climate data show a warming trend and more frequent droughts occurring in the research region since the 1970s. The isotope record of Qinghai spruce ( Picea crassifolia ) spans the period 1890–2002 with a general decreasing trend over the last century followed by an abrupt increase in δ 13C over the last decade. The stable carbon discrimination against heavier atmospheric carbon (Δ13C) is negatively correlated to May temperature and positively correlated with June–July precipitation. The regional Palmer drought severity index (PDSI) correlated significantly with Δ13C series after 1960, whereas this relationship was not stable over the period 1933–1960. However, much stronger correlations were observed between the high-frequency anomalies in annual Δ13C and PDSI in June and July during the period 1933–2002. The temporal stability analysis revealed trends in the response to drought stress affecting tree's Δ13C linked to climatic warming. Intrinsic water-use efficiency increased by 7.7% in Qinghai spruce in response to increased severity of regional drought during the 1990s compared to the average of the previous decade. Our preliminary results suggest that carbon isotope in certain tree taxa growing on Tibetan Plateau may be an effective proxy for reconstructing regional drought.
DOI:10.13344/j.microbiol.china.140581URL [本文引用: 1]

硝化作用先将氨氮氧化为亚硝酸盐氮并进一步氧化为硝酸盐氮,这一过程是氮进行全球生物化学循环的重要环节。随着氨氧化古菌(Ammonia-oxidizing archaea,AOA)基因组序列中氨单加氧酶编码基因(amo A)的发现以及AOA在实验室条件下的成功培养(包括分离纯化和富集培养),基于分子生物学的研究表明AOA在各种环境广泛存在,且多数生境中它的数量远远超过氨氧化细菌(Ammonia-oxidizing bacteria,AOB)。AOA相对于AOB在氮循环中的贡献也引起了多方面的论证和争论。本文就氨氧化古菌的生态分布、系统进化、生境存在丰度及参与硝化作用等进行综述,指出不同生境AOA的活性及其对氮循环的重要性仍需做进一步的研究。
DOI:10.13344/j.microbiol.china.140581URL [本文引用: 1]

硝化作用先将氨氮氧化为亚硝酸盐氮并进一步氧化为硝酸盐氮,这一过程是氮进行全球生物化学循环的重要环节。随着氨氧化古菌(Ammonia-oxidizing archaea,AOA)基因组序列中氨单加氧酶编码基因(amo A)的发现以及AOA在实验室条件下的成功培养(包括分离纯化和富集培养),基于分子生物学的研究表明AOA在各种环境广泛存在,且多数生境中它的数量远远超过氨氧化细菌(Ammonia-oxidizing bacteria,AOB)。AOA相对于AOB在氮循环中的贡献也引起了多方面的论证和争论。本文就氨氧化古菌的生态分布、系统进化、生境存在丰度及参与硝化作用等进行综述,指出不同生境AOA的活性及其对氮循环的重要性仍需做进一步的研究。
DOI:10.1073/pnas.1516684112URLPMID:26647180 [本文引用: 1]

Abstract Soil bacteria and fungi play key roles in the functioning of terrestrial ecosystems, yet our understanding of their responses to climate change lags significantly behind that of other organisms. This gap in our understanding is particularly true for drylands, which occupy 09080441% of Earth0007s surface, because no global, systematic assessments of the joint diversity of soil bacteria and fungi have been conducted in these environments to date. Here we present results from a study conducted across 80 dryland sites from all continents, except Antarctica, to assess how changes in aridity affect the composition, abundance, and diversity of soil bacteria and fungi. The diversity and abundance of soil bacteria and fungi was reduced as aridity increased. These results were largely driven by the negative impacts of aridity on soil organic carbon content, which positively affected the abundance and diversity of both bacteria and fungi. Aridity promoted shifts in the composition of soil bacteria, with increases in the relative abundance of Chloroflexi and 02±-Proteobacteria and decreases in Acidobacteria and Verrucomicrobia. Contrary to what has been reported by previous continental and global-scale studies, soil pH was not a major driver of bacterial diversity, and fungal communities were dominated by Ascomycota. Our results fill a critical gap in our understanding of soil microbial communities in terrestrial ecosystems. They suggest that changes in aridity, such as those predicted by climate-change models, may reduce microbial abundance and diversity, a response that will likely impact the provision of key ecosystem services by global drylands.
DOI:10.1016/j.soilbio.2017.01.021URL [本文引用: 1]

Nitrous oxide (N 2 O) and methane (CH 4 ) emissions were measured along three natural geothermal soil temperature (T s ) gradients in freely drained upland soils in a volcanic area in Iceland. Two of the T s gradients (underneath a grassland (GN) and a forest site (FN), respectively) were recently formed (in May 2008) and thus subjected to relatively short-term warming. The third T s gradient, underneath another grassland site (GO), had been subjected to long-term soil warming (over at least 45 years). The N 2 O and CH 4 emissions were measured using the static chamber method. In addition, subsurface soil gas concentrations (5–20cm) were studied. N 2 O emissions from GN were slightly higher than those from GO in the temperature elevation range up to+5 ° C, while CH 4 uptake rates were similar. Under moderate soil warming (<+5 ° C) there were no significant increases in gas flux rates within any of the sites, but when soil warming exceeded+20 ° C, both N 2 O and CH 4 emissions increased significantly at all sites. While net uptake of CH 4 (up to610.15mg CH 4 m 612 h 611 ) and occasional N 2 O uptake (up to6112μg N 2 O m 612 h 611 ) were measured in the unwarmed plots at all sites, net emissions were only measured from the warmest plots (up to 2600μg N 2 O m 612 h 611 and up to 1.3mg CH 4 m 612 h 611 ). The subsurface soil N 2 O concentrations increased with soil warming, indicating enhanced N-turnover. Subsurface soil CH 4 concentrations initially decreased under moderate soil warming (up to+5 ° C), but above that threshold they also increased significantly. A portion of the N 2 O and CH 4 emitted from the warmest plots may, however, be geothermally derived, this should be further confirmed with isotope studies. In conclusion, our research suggests that moderate increases in soil temperature (up to+5 ° C) may not significantly increase N 2 O and CH 4 emissions at these upland soils, both in the short and longer term. However, warming trends exceeding+5 ° C as predicted for 2100 in pessimistic scenarios may cause increased trace gas emissions and thus significant positive feedbacks to climate change.
[本文引用: 2]
DOI:10.1111/gcb.13789URLPMID:28614594 [本文引用: 1]

Abstract Unprecedented levels of nitrogen (N) have entered terrestrial ecosystems over the past century, which substantially influences the carbon (C) exchange between the atmosphere and biosphere. Temperature and moisture are generally regarded as the0002major controllers over the N effects on ecosystem C uptake and release. N-phosphorous (P) stoichiometry regulates the growth and metabolisms of plants and soil organisms, thereby affecting many ecosystem C processes. However, it remains unclear how the N-induced shift in the plant N:P ratio affects ecosystem production and C fluxes and its relative importance. We conducted a field manipulative experiment with eight N addition levels in a Tibetan alpine steppe and assessed the influences of N on aboveground net primary production (ANPP), gross ecosystem productivity (GEP), ecosystem respiration (ER), and net ecosystem exchange (NEE); we used linear mixed-effects models to further determine the relative contributions of various factors to the N-induced changes in these parameters. Our results showed that the ANPP, GEP, ER, and NEE all exhibited nonlinear responses to increasing N additions. Further analysis demonstrated that the plant N:P ratio played a dominate role in shaping these C exchange processes. There was a positive relationship between the N-induced changes in ANPP (0200ANPP) and the plant N:P ratio (0200N:P), whereas the 0200GEP, 0200ER, and 0200NEE exhibited quadratic correlations with the 0200N:P. In contrast, soil temperature and moisture were only secondary predictors for the changes in ecosystem production and C fluxes along the N addition gradient. These findings highlight the importance of plant N:P ratio in regulating ecosystem C exchange, which is crucial for improving our understanding of C cycles under the scenarios of global N enrichment. 0008 2017 John Wiley & Sons Ltd.
DOI:10.1126/science.1176985URL [本文引用: 1]
DOI:10.1098/rstb.1982.0019URL [本文引用: 2]
[本文引用: 3]
DOI:10.1007/s11284-012-0950-8URL [本文引用: 1]

The eastern Tibetan Plateau has become increasingly warmer and drier since the 1990s. Such warming and drying has a great impact on ecosystem processes on the eastern Tibetan Plateau . To determine their combined effects on CO 2 and N 2 O emission rates, we conducted a field manipulative experiment in an alpine meadow of the eastern Tibetan Plateau during the growing season of 2009. The experiment showed that warming manipulation increased soil temperature by 102°C, and drying manipulation decreased soil water content by 6.802%. We found that by counteracting the effect of low temperature in the area, experimental warming significantly increased soil microbial biomass, the number of bacteria, fungi, actinomycetes, ammonifying bacteria, nitrobacteria and denitrifying bacteria, and facilitated the emission rates of CO 2 and N 2 O by 33.4 and 31.502%, respectively. However, decreased precipitation further aggravated soil water stress and inhibited the numbers of these organisms, and reduced the emission rates of CO 2 and N 2 O by 47.4 and 37.902%, respectively. So decreased soil water content tended to offset the positive effect of warming. Compared to the positive effects of warming, decreased soil water content was shown in our study to have even greater impact on the eastern Tibetan Plateau during the growing season. Therefore, inhibition of CO 2 and N 2 O emission rates (32.3 and 29.302%, respectively) by warming and drying will intensify if the combined effects of these climatic trends persist in the region.
DOI:10.1890/12-2119.1URL [本文引用: 2]

Abstract Soils, plants, and microbial communities respond to global change perturbations through coupled, nonlinear interactions. Dynamic ecological responses complicate projecting how global change disturbances will influence ecosystem processes, such as carbon (C) storage. We developed an ecosystem-scale model (Stoichiometrically Coupled, Acclimating Microbe lant oil model, SCAMPS) that simulates the dynamic feedbacks between aboveground and belowground communities that affect their shared soil environment. The belowground component of the model includes three classes of soil organic matter (SOM), three microbially synthesized extracellular enzyme classes specific to these SOM pools, and a microbial biomass pool with a variable C-to-N ratio (C:N). The plant biomass, which contributes to the SOM pools, flexibly allocates growth toward wood, root, and leaf biomass, based on nitrogen (N) uptake and shoot-to-root ratio. Unlike traditional ecosystem models, the microbial community can acclimate to changing soil resources by shifting its C:N between a lower C:N, faster turnover (bacteria-like) community, and a higher C:N, slower turnover (fungal-like) community. This stoichiometric flexibility allows for the microbial C and N use efficiency to vary, feeding back into system decomposition and productivity dynamics. These feedbacks regulate changes in extracellular enzyme synthesis, soil pool turnover rates, plant growth, and ecosystem C storage. We used SCAMPS to test the interactive effects of winter, summer, and year-round soil warming, in combination with microbial acclimation ability, on decomposition dynamics and plant growth in a tundra system.Over 50-year simulations, both the seasonality of warming and the ability of the microbial community to acclimate had strong effects on ecosystem C dynamics. Across all scenarios, warming increased plant biomass (and therefore litter inputs to the SOM), while the ability of the microbial community to acclimate increased soil C loss. Winter warming drove the largest ecosystem C losses when the microbial community could acclimate, and the largest ecosystem C gains when it could not acclimate. Similar to empirical studies of tundra warming, modeled summer warming had relatively negligible effects on soil C loss, regardless of acclimation ability. In contrast, winter and year-round warming drove marked soil C loss when decomposers could acclimate, despite also increasing plant biomass. These results suggest that incorporating dynamically interacting microbial and plant communities into ecosystem models might increase the ability to link ongoing global change field observations with macro-scale projections of ecosystem biogeochemical cycling in systems under change.
DOI:10.1016/j.soilbio.2012.01.016URL [本文引用: 2]

Production and consumption of greenhouse gases such as CO 2 , CH 4 and N 2 O are key factors driving climate change. While CO 2 sinks are commonly reported and the mechanisms relatively well understood, N 2 O sinks have often been overlooked and the driving factors for these sinks are poorly understood. We examined CO 2 , CH 4 and N 2 O flux in three High Arctic polar deserts under both light (measured in transparent chambers) and dark (measured in opaque chambers) conditions. We further examined if differences in soil moisture, evapotranspiration, Photosynthetically Active Radiation (PAR), and/or plant communities were driving gas fluxes measured in transparent and opaque chambers at each of our sites. Nitrous oxide sinks were found at all of our sites suggesting that N 2 O uptake can occur under extreme polar desert conditions, with relatively low soil moisture, soil temperature and limited soil N. Fluxes of CO 2 and N 2 O switched from sources under dark conditions to sinks under light conditions, while CH 4 fluxes at our sites were not affected by light conditions. Neither evapotranspiration nor PAR were significantly correlated with CO 2 or N 2 O flux, however, soil moisture was significantly correlated with both gas fluxes. The relationship between soil moisture and N 2 O flux was different under light and dark conditions, suggesting that there are other factors, in addition to moisture, driving N 2 O sinks. We found significant differences in N 2 O and CO 2 flux between plant communities under both light and dark conditions and observed individual communities that shifted between sources and sinks depending on light conditions. Failure of many studies to include plant-mediated N 2 O flux, as well as, N 2 O soil sinks may account for the currently unbalanced global N 2 O budget.
DOI:10.1111/j.1574-6941.2010.00853.xURLPMID:20298502 [本文引用: 2]

Complete cycling of mineral nitrogen (N) in soil requires the interplay of microorganisms performing nitrification and denitrification, whose activity is increasingly affected by extreme rainfall or heat brought about by climate change. In a pristine forest soil, a gradual increase in soil temperature from 5 to 25 00°C in a range of water contents stimulated N turnover rates, and N gas emissions were determined by the soil water-filled pore space (WFPS). NO and N2O emissions dominated at 30% WFPS and 55% WFPS, respectively, and the step-wise temperature increase resulted in a threefold increase in the NO3090808 concentrations and a decrease in the NH4+ concentration. At 70% WFPS, NH4+ accumulated while NO3090808 pools declined, indicating gaseous N loss. AmoA- and nirK-gene-based analysis revealed increasing abundance of bacterial ammonia oxidizers (AOB) with increasing soil temperature and a decrease in the abundance of archaeal ammonia oxidizers (AOA) in wet soil at 25 00°C, suggesting the sensitivity of the latter to anaerobic conditions. Denitrifier (nirK) community structure was most affected by the water content and nirK gene abundance rapidly increased in response to wet conditions until the substrate (NO3090808) became limiting. Shifts in the community structure were most pronounced for nirK and most rapid for AOA, indicating dynamic populations, whereas distinct adaptation of the AOB communities required 5 weeks, suggesting higher stability.
DOI:10.1016/S1570-0232(03)00363-5URL [本文引用: 1]

The magnitude, temporal, and spatial patterns of soil-atmospheric greenhouse gas (hereafter referred to as GHG) exchanges in forests near the Tropic of Cancer are still highly uncertain. To contribute towards an improvement of actual estimates, soil-atmospheric CO2, CH4, and N2O fluxes were measured in three successional subtropical forests at the Dinghushan Nature Reserve (hereafter referred to as DNR) in southern China. Soils in DNR forests behaved as N2O sources and CH4 sinks. Annual mean CO2, N2O, and CH4 fluxes (mean +/- SD) were 7.7 +/- 4.6 Mg CO2-C ha(-1) yr(-1), 3.2 +/- 1.2 kg N2O-N ha(-1) yr(-1), and 3.4 +/- 0.9 kg CH4-C ha(-1) yr(-1), respectively. The climate was warm and wet from April through September 2003 (the hot-humid season) and became cool and dry from October 2003 through March 2004 (the cool-dry season). The seasonality of soil CO2 emission coincided with the seasonal climate pattern, with high CO2 emission rates in the hot-humid season and low rates in the cool-dry season. In contrast, seasonal patterns of CH4 and N2O fluxes were not clear, although higher CH4 uptake rates were often observed in the cool-dry season and higher N2O emission rates were often observed in the hot-humid season. GHG fluxes measured at these three sites showed a clear increasing trend with the progressive succession. If this trend is representative at the regional scale, CO2 and N2O emissions and CH4 uptake in southern China may increase in the future in light of the projected change in forest age structure. Removal of surface litter reduced soil CO2 effluxes by 17-44% in the three forests but had no significant effect on CH4 absorption and N2O emission rates. This suggests that microbial CH4 uptake and N2O production was mainly related to the mineral soil rather than in the surface litter layer.
DOI:10.1007/s00442-014-2894-xURLPMID:24532178 [本文引用: 1]

Global change phenomena, such as forest disturbance and land-use change, significantly affect elemental balances as well as the structure and function of terrestrial ecosystems. However, the importance of shifts in soil nutrient stoichiometry for the regulation of belowground biota and soil food webs have not been intensively studied for tropical ecosystems. In the present account, we examine the effects of land-use change and soil depth on soil and microbial stoichiometry along a land-use sequence (natural forest, pastures of different ages, secondary succession) in the tropical mountain rainforest region of southern Ecuador. Furthermore, we analyzed (PLFA-method) whether shifts in the microbial community structure were related to alterations in soil and microbial stoichiometry. Soil and microbial stoichiometry were affected by both land-use change and soil depth. After forest disturbance, significant decreases of soil C:N:P ratios at the pastures were followed by increases during secondary succession. Microbial C:N ratios varied slightly in response to land-use change, whereas no fixed microbial C:P and N:P ratios were observed. Shifts in microbial community composition were associated with soil and microbial stoichiometry. Strong positive relationships between PLFA-markers 18:2n6,9c (saprotrophic fungi) and 20:4 (animals) and negative associations between 20:4 and microbial N:P point to land-use change affecting the structure of soil food webs. Significant deviations from global soil and microbial C:N:P ratios indicated a major force of land-use change to alter stoichiometric relationships and to structure biological systems. Our results support the idea that soil biotic communities are stoichiometrically flexible in order to adapt to alterations in resource stoichiometry.
DOI:10.1016/0038-0717(87)90052-6URL [本文引用: 1]

The effects of fumigation on organic C extractable by 0.5 M K 2SO 4 were examined in a contrasting range of soils. E C (the difference between organic C extracted by 0.5 M K 2SO 4 from fumigated and non-fumigated soil) was about 70% of F C (the flush of CO 2-C caused by fumigation during a 10 day incubation), meaned for ten soils. There was a close relationship between microbial biomass C, measured by fumigation-incubation (from the relationship Biomass C = F C/0.45) and E C given by the equation: Biomass C = (2.64 0.060) E C that accounted for 99.2% of the variance in the data. This relationship held over a wide range of soil pH (3.9 8.0). ATP and microbial biomass N concentrations were measured in four of the soils. The (ATP) (E C) ratios were very similar in the four soils, suggesting that both ATP and the organic C rendered decomposable by CHCl 3 came from the soil microbial biomass. The C:N ratio of the biomass in a strongly acid (pH 4.2) soil was greater (9.4) than in the three less-acid soils (mean C:N ratio 5.1). We propose that the organic C rendered extractable to 0.5 m K 2SO 4 after a 24 h CHCl 3-fumigation ( E C) comes from the cells of the microbial biomass and can be used to estimate soil microbial biomass C in both neutral and acid soils.
DOI:10.1111/gcb.13563URLPMID:27862698 [本文引用: 1]

Abstract Rapidly rising temperatures in the Arctic might cause a greater release of greenhouse gases (GHGs) to the atmosphere. To study the effect of warming on GHG dynamics, we deployed open-top chambers in a subarctic tundra site in Northeast European Russia. We determined carbon dioxide (CO2), methane (CH4), and nitrous oxide (N2O) fluxes as well as the concentration of those gases, inorganic nitrogen (N) and dissolved organic carbon (DOC) along the soil profile. Studied tundra surfaces ranged from mineral to organic soils and from vegetated to unvegetated areas. As a result of air warming, the seasonal GHG budget of the vegetated tundra surfaces shifted from a GHG sink of -300 to -198 g CO2 q m-2 to a source of 105 to 144 g CO2 q m-2. At bare peat surfaces we observed increased release of all three GHGs. While the positive warming response was dominated by CO2, we provide here the first in situ evidence of increasing N2O emissions from tundra soils with warming. Warming promoted N2O release not only from bare peat, previously identified as a strong N2O source, but also from the abundant, vegetated peat surfaces that do not emit N2O under present climate. At these surfaces elevated temperatures had an adverse effect on plant growth, resulting in lower plant N uptake and, consequently, better N availability for soil microbes. Although the warming was limited to the soil surface and did not alter thaw depth, it increased concentrations of DOC, CO2 and CH4 in the soil down to the permafrost table. This can be attributed to downward DOC leaching, fuelling microbial activity at depth. Taken together, our results emphasize the tight linkages between plant and soil processes, and different soil layers, which need to be taken into account when predicting the climate change feedback of the Arctic.
DOI:10.1007/s11368-016-1530-2URL [本文引用: 2]

Abstract PurposeAlpine ecosystems on the Qinghai-Tibetan Plateau are sensitive to global climatic changes. However, the effects of temperature change resulting from global warming or seasonal variation on soil N availability in those ecosystems are largely unknown. Materials and methodsWe therefore conducted a 15N tracing study to investigate the effects of various temperatures (5–35 °C) on soil gross N transformation rates in an alpine meadow (AM) soil on the Qinghai-Tibetan Plateau. A natural secondary coniferous forest (CF) soil from the subtropical region was chosen as a reference to compare the temperature sensitivity of soil gross N transformation rates between alpine meadow and coniferous forest. Results and discussionOur results showed that increasing temperature increased gross N mineralization and NH4+ immobilization rates and overall enhanced N availability for plants in both soils. However, both rates in the CF soil were less sensitive to a temperature change from 5 to 15 °C compared to the AM soil. In both soils, different N retention mechanisms could have been operating with respect to changing temperatures in the different climatic regions. In the CF soil, the absence of NO361 production at all incubation temperatures suggests that in the subtropical soil which is characterized by high rainfall, an increase in N availability due to increasing temperature could be completely retained in soils. In contrast, the AM soil may be vulnerable to N losses with respect to temperature changes, in particular at 35 °C, in which higher nitrification rates were coupled with lower NH4+ and NO361 immobilization rates. Conclusions Our results suggest that increased soil temperature arising from global warming and seasonal variations will most likely enhance soil N availability for plants and probably increase the risk of N losses in the alpine meadow on the Qinghai-Tibetan Plateau.
DOI:10.11654/jaes.2015.11.019URL [本文引用: 2]

通过对35例2009—2015年初发表的增温控制试验的96组数据进行Meta分析,研究了土壤微生物对增温的响应模式,结果表明:增温提高了土壤微生物碳(1.4%)、土壤微生物氮(1.7%)和土壤真菌量(3.7%),降低了土壤细菌量(-1.9%);土壤微生物碳的增温响应与试验地年平均降水量和增温幅度显著相关;土壤微生物氮的变化受试验地年均温、年均降水量及增温幅度的影响。
DOI:10.11654/jaes.2015.11.019URL [本文引用: 2]

通过对35例2009—2015年初发表的增温控制试验的96组数据进行Meta分析,研究了土壤微生物对增温的响应模式,结果表明:增温提高了土壤微生物碳(1.4%)、土壤微生物氮(1.7%)和土壤真菌量(3.7%),降低了土壤细菌量(-1.9%);土壤微生物碳的增温响应与试验地年平均降水量和增温幅度显著相关;土壤微生物氮的变化受试验地年均温、年均降水量及增温幅度的影响。
DOI:10.1111/ele.12167URLPMID:23953244 [本文引用: 1]

Understanding the effects of warming on greenhouse gas feedbacks to climate change represents a major global challenge. Most research has focused on direct effects of warming, without considering how concurrent changes in plant communities may alter such effects. Here, we combined vegetation manipulations with warming to investigate their interactive effects on greenhouse gas emissions from peatland. We found that although warming consistently increased respiration, the effect on net ecosystem CO2 exchange depended on vegetation composition. The greatest increase in CO2 sink strength after warming was when shrubs were present, and the greatest decrease when graminoids were present. CH4 was more strongly controlled by vegetation composition than by warming, with largest emissions from graminoid communities. Our results show that plant community composition is a significant modulator of greenhouse gas emissions and their response to warming, and suggest that vegetation change could alter peatland carbon sink strength under future climate change.
[本文引用: 1]
[本文引用: 1]
DOI:10.3724/SP.J.1258.2014.00076URL [本文引用: 1]

目前,有关增温条件下荒漠生物土壤结皮(BSCs)-土壤系统与大气之间主要温室气体(CO2、CH4和N2O)通量变化的研究十分匮乏,以致很难准确地评估荒漠生态系统温室气体通量对气候变暖的响应与反馈的方向和程度。该文选择腾格里沙漠东南缘天然植被区由藻类、藓类以及二者混生的3种类型的BSCs覆盖土壤为研究对象,以开顶式生长室(OTC)为增温方式模拟全球变暖,采用静态箱-气相色谱法探究了2012年7月至2013年6月增温和不增温处理下CO2、CH4和N2O通量的变化特征。结果表明:增温和结皮类型对CO2、CH4和N2O通量没有显著影响。采样日期、结皮类型与采样日期,以及增温与结皮类型和采样日期的互作显著影响CO2和CH4通量,增温和采样日期互作显著影响CH4通量。BSCs-土壤系统的CO2、CH4和N2O年通量及其增温潜能在增温和不增温处理下的差异均不显著。CO2通量与5 cm深度的土壤温度呈显著的指数正相关关系,与10 cm深度的土壤湿度呈线性正相关关系;藓类、混生结皮的CH4通量与5 cm深度的土壤温度和10 cm深度的土壤湿度均呈显著的线性负相关关系;3种结皮类型的N2O通量与5 cm深度的土壤温度均无相关关系,藓类结皮的N2O通量与10 cm深度的土壤湿度呈显著的线性负相关关系。藓类结皮的CO2和CH4在增温和不增温两种处理下的通量差异与5 cm深度的土壤温度差异呈显著的负线性相关,藻类结皮N2O的通量差异与温度差异呈近似正相关关系(p=0.051)。以上结果说明:在全球变暖的背景下,荒漠BSCs-土壤系统主要温室气体通量不会有明显的变化,意味着荒漠生态系统温室气体的排放可能对气候变暖没有明显的反馈。
DOI:10.3724/SP.J.1258.2014.00076URL [本文引用: 1]

目前,有关增温条件下荒漠生物土壤结皮(BSCs)-土壤系统与大气之间主要温室气体(CO2、CH4和N2O)通量变化的研究十分匮乏,以致很难准确地评估荒漠生态系统温室气体通量对气候变暖的响应与反馈的方向和程度。该文选择腾格里沙漠东南缘天然植被区由藻类、藓类以及二者混生的3种类型的BSCs覆盖土壤为研究对象,以开顶式生长室(OTC)为增温方式模拟全球变暖,采用静态箱-气相色谱法探究了2012年7月至2013年6月增温和不增温处理下CO2、CH4和N2O通量的变化特征。结果表明:增温和结皮类型对CO2、CH4和N2O通量没有显著影响。采样日期、结皮类型与采样日期,以及增温与结皮类型和采样日期的互作显著影响CO2和CH4通量,增温和采样日期互作显著影响CH4通量。BSCs-土壤系统的CO2、CH4和N2O年通量及其增温潜能在增温和不增温处理下的差异均不显著。CO2通量与5 cm深度的土壤温度呈显著的指数正相关关系,与10 cm深度的土壤湿度呈线性正相关关系;藓类、混生结皮的CH4通量与5 cm深度的土壤温度和10 cm深度的土壤湿度均呈显著的线性负相关关系;3种结皮类型的N2O通量与5 cm深度的土壤温度均无相关关系,藓类结皮的N2O通量与10 cm深度的土壤湿度呈显著的线性负相关关系。藓类结皮的CO2和CH4在增温和不增温两种处理下的通量差异与5 cm深度的土壤温度差异呈显著的负线性相关,藻类结皮N2O的通量差异与温度差异呈近似正相关关系(p=0.051)。以上结果说明:在全球变暖的背景下,荒漠BSCs-土壤系统主要温室气体通量不会有明显的变化,意味着荒漠生态系统温室气体的排放可能对气候变暖没有明显的反馈。
DOI:10.1007/s11356-017-8799-6URLPMID:28324256 [本文引用: 1]

Abstract Although the effect of simulated climate change on nitrous oxide (N 2 O) emissions and on associated microbial communities has been reported, it is not well understood if these effects are short-lived or long-lasting. Here, we conducted a field study to determine the interactive effects of simulated warmer and drier conditions on nitrifier and denitrifier communities and N 2 O emissions in an acidic soil and the longevity of the effects. A warmer (+2.3 C) and drier climate (-7.4% soil moisture content) was created with greenhouses. The variation of microbial population abundance and community structure of ammonia-oxidizing archaea (AOA), bacteria (AOB), and denitrifiers (nirK/S, nosZ) were determined using real-time PCR and high-throughput sequencing. The results showed that the simulated warmer and drier conditions under the greenhouse following urea application significantly increased N 2 O emissions. There was also a moderate legacy effect on the N 2 O emissions when the greenhouses were removed in the urea treatment, although this effect only lasted a short period of time (about 60 days). The simulated climate change conditions changed the composition of AOA with the species affiliated to marine group 1.1a-associated lineage increasing significantly. The abundance of all the functional denitrifier genes decreased significantly under the simulated climate change conditions and the legacy effect, after the removal of greenhouses, significantly increased the abundance of AOB, AOA (mainly the species affiliated to marine group 1.1a-associated lineage), and nirK and nosZ genes in the urea-treated soil. In general, the effect of the simulated climate change was short-lived, with the denitrifier communities being able to return to ambient levels after a period of adaptation to ambient conditions. Therefore, the legacy effect of simulated short-time climate change conditions on the ammonia oxidizer and denitrifier communities and N 2 O emissions were temporary and once the conditions were removed, the microbial communities were able to adapt to the ambient conditions.
DOI:10.1111/gcb.2013.20.issue-1URL [本文引用: 1]
DOI:10.1016/j.apsoil.2014.11.012URL

Alpine ecosystems at high altitudes and latitudes are notably sensitive to climatic warming and the Tibetan Plateau is a widely distributed alpine ecosystem. The magnitude of climatic warming on the Tibetan Plateau is expected to be considerably greater than the global average. However, a synthesis of the experimental warming soil carbon and nitrogen data is still lacking and whether forest soils are more sensitive to warming than grassland soils remains unclear. In this study, we used a meta-analysis approach to synthesise 196 observations from 25 published studies on the Tibetan Plateau. Warming significantly increased microbial biomass carbon (MBC) by 14.3% (95% CI: 2.9–24.6%), microbial biomass nitrogen (MBN) by 20.1% (95% CI: 2.0–45.1%), net nitrogen mineralization by 49.2% (95% CI: 38.1–62.3%) and net nitrification by 56.0% (95% CI: 51.4–66.1%), but did not significantly affect soil carbon (95% CI: 6113.9 to 2.7%) or nitrogen (95% CI: 6112.4 to 2.6%). The mean annual air temperature was negatively correlated with the warming effects on MBC and MBN. Grasslands exhibited significant MBC and MBN responses to warming. Specifically, soil microbial biomass was more responsive to warming in colder environments. Moreover, forest soils are not always more sensitive to warming than grassland soils as previous studies have suggested. These findings indicate that clarifying the effect of warming on alpine soils need consider ecosystem types and their local climate.
Effects of temperature and fertilization on nitrogen cycling and community composition of an urban lawn
1
2008
... id="C38">本研究结果显示, 土壤含水量对高寒草原土壤N2O通量起着明显的调控作用.增温导致了土壤含水量的显著下降(
Chloroform fumigation and the release of soil nitrogen: A rapid direct extraction method to measure microbial biomass nitrogen in soil
1
1985
... id="C16">土壤样品采用1 mol·L-1 KCl溶液浸提, 之后采用连续流动分析仪(Autoanalyzer 3 SEAL, Bran and Luebbe, Norderstedt, Germany)测定浸提液中的NH4+-N和NO3--N含量, 二者相加得到土壤总无机氮(SIN)含量.土壤微生物碳(MBC)和微生物氮(MBN)采用氯仿熏蒸法(
The effect of experimental warming and precipitation change on proteolytic enzyme activity: Positive feedbacks to nitrogen availability are not universal
1
2012
... id="C37">本研究结果表明, 增温并没有显著改变青藏高原高寒草原土壤N2O通量.一般来讲, 作为硝化和反硝化等生物地球化学过程的产物, 土壤N2O通量会受到土壤温度变化的影响.以往的研究显示, 土壤温度的上升会促进土壤N矿化, 提高土壤无机氮的含量, 为硝化和反硝化过程提供更充足的底物(
Nitrous oxide emissions from soils: How well do we understand the processes and their controls?
1
2013
... id="C5">近10年来, 国内外****相继开展了土壤N2O对增温响应方面的研究.多数研究报道增温会促进不同生态系统中土壤N2O的排放(
Four years of experimental climate change modifies the microbial drivers of N2O fluxes in an upland grassland ecosystem
1
2012
... id="C37">本研究结果表明, 增温并没有显著改变青藏高原高寒草原土壤N2O通量.一般来讲, 作为硝化和反硝化等生物地球化学过程的产物, 土壤N2O通量会受到土壤温度变化的影响.以往的研究显示, 土壤温度的上升会促进土壤N矿化, 提高土壤无机氮的含量, 为硝化和反硝化过程提供更充足的底物(
Principles of Terrestrial Ecosystem Ecology.
3
2011
... id="C5">近10年来, 国内外****相继开展了土壤N2O对增温响应方面的研究.多数研究报道增温会促进不同生态系统中土壤N2O的排放(
... id="C37">本研究结果表明, 增温并没有显著改变青藏高原高寒草原土壤N2O通量.一般来讲, 作为硝化和反硝化等生物地球化学过程的产物, 土壤N2O通量会受到土壤温度变化的影响.以往的研究显示, 土壤温度的上升会促进土壤N矿化, 提高土壤无机氮的含量, 为硝化和反硝化过程提供更充足的底物(
... id="C39">本研究结果表明, 高寒草原土壤N2O通量呈现明显的年际变化.在两年观测期间, 2014年生长季降水量较为丰沛(534.7 mm), 而2015年生长季降水量明显偏低(371.7 mm), 导致土壤含水量明显低于2014年(
Individualistic growth response of tundra plant species to environmental manipulations in the field
1
1985
... id="C8">我们于2013年6月在研究区建立了高寒草原增温控制实验平台(
Soils, a sink for N2O? A review
1
2007
... id="C5">近10年来, 国内外****相继开展了土壤N2O对增温响应方面的研究.多数研究报道增温会促进不同生态系统中土壤N2O的排放(
The impacts of climate change and human activities on biogeochemical cycles on the Qinghai-Tibetan Plateau
1
2013
... id="C6">高寒草原是青藏高原面积最大的草地生态系统, 其分布面积达6.4 × 105 km2 (
Responses of ammonia-oxidizing bacteria and archaea to nitrogen fertilization and precipitation increment in a typical temperate steppe in Inner Mongolia
1
2013
... id="C5">近10年来, 国内外****相继开展了土壤N2O对增温响应方面的研究.多数研究报道增温会促进不同生态系统中土壤N2O的排放(
Soil warming and fertilization altered rates of nitrogen transformation processes and selected for adapted ammonia-oxidizing archaea in sub-arctic grassland soil
1
2017
... id="C38">本研究结果显示, 土壤含水量对高寒草原土壤N2O通量起着明显的调控作用.增温导致了土壤含水量的显著下降(
The permafrost carbon inventory on the Tibetan Plateau: A new evaluation using deep sediment cores
1
2016
... id="C6">高寒草原是青藏高原面积最大的草地生态系统, 其分布面积达6.4 × 105 km2 (
Effects of climate and management intensity on nitrous oxide emissions in grassland systems across Europe
1
2007
... id="C4">氧化亚氮(N2O)是大气中一种重要的温室气体, 其温室效应是CO2的298倍(
Trade-off between soil organic carbon sequestration and nitrous oxide emissions from winter wheat-?summer maize rotations: Implications of a 25-year fertilization experiment in Northwestern China
1
2017
... id="C37">本研究结果表明, 增温并没有显著改变青藏高原高寒草原土壤N2O通量.一般来讲, 作为硝化和反硝化等生物地球化学过程的产物, 土壤N2O通量会受到土壤温度变化的影响.以往的研究显示, 土壤温度的上升会促进土壤N矿化, 提高土壤无机氮的含量, 为硝化和反硝化过程提供更充足的底物(
反硝化菌功能基因及其分子生态学研究进展
1
2011
... id="C5">近10年来, 国内外****相继开展了土壤N2O对增温响应方面的研究.多数研究报道增温会促进不同生态系统中土壤N2O的排放(
反硝化菌功能基因及其分子生态学研究进展
1
2011
... id="C5">近10年来, 国内外****相继开展了土壤N2O对增温响应方面的研究.多数研究报道增温会促进不同生态系统中土壤N2O的排放(
Microbial nitrous oxide emissions in dryland ecosystems: Mechanisms, microbiome and mitigation
1
2017
... id="C38">本研究结果显示, 土壤含水量对高寒草原土壤N2O通量起着明显的调控作用.增温导致了土壤含水量的显著下降(
Effects of warming and grazing on N2O fluxes in an alpine meadow ecosystem on the Tibetan Plateau
1
2010
... id="C5">近10年来, 国内外****相继开展了土壤N2O对增温响应方面的研究.多数研究报道增温会促进不同生态系统中土壤N2O的排放(
增温对土壤有机碳矿化的影响研究综述
1
2017
... id="C37">本研究结果表明, 增温并没有显著改变青藏高原高寒草原土壤N2O通量.一般来讲, 作为硝化和反硝化等生物地球化学过程的产物, 土壤N2O通量会受到土壤温度变化的影响.以往的研究显示, 土壤温度的上升会促进土壤N矿化, 提高土壤无机氮的含量, 为硝化和反硝化过程提供更充足的底物(
增温对土壤有机碳矿化的影响研究综述
1
2017
... id="C37">本研究结果表明, 增温并没有显著改变青藏高原高寒草原土壤N2O通量.一般来讲, 作为硝化和反硝化等生物地球化学过程的产物, 土壤N2O通量会受到土壤温度变化的影响.以往的研究显示, 土壤温度的上升会促进土壤N矿化, 提高土壤无机氮的含量, 为硝化和反硝化过程提供更充足的底物(
3
2013
... id="C4">氧化亚氮(N2O)是大气中一种重要的温室气体, 其温室效应是CO2的298倍(
... O排放总量的60% (
... id="C10">我们于2013年6月在对照和增温处理中分别布设了一个40 cm × 40 cm的不锈钢底座, 底座打入土壤约6 cm.在2014和2015年的生长季(5-10月)利用静态箱-气相色谱法(
Soil-atmosphere exchange in nitrous oxide, nitric oxide, and methane under secondary succession of pasture to forest in the Atlantic lowlands of Costa Rica
1
1994
... id="C5">近10年来, 国内外****相继开展了土壤N2O对增温响应方面的研究.多数研究报道增温会促进不同生态系统中土壤N2O的排放(
Experimental warming-driven soil drying reduced N2O emissions from fertilized crop rotations of winter wheat-soybean/fallow, 2009-2014
1
2016
... id="C6">高寒草原是青藏高原面积最大的草地生态系统, 其分布面积达6.4 × 105 km2 (
Response and dendroclimatic implications of δ 13C in tree rings to increasing drought on the northeastern Tibetan Plateau.
1
2008
... id="C5">近10年来, 国内外****相继开展了土壤N2O对增温响应方面的研究.多数研究报道增温会促进不同生态系统中土壤N2O的排放(
氨氧化古菌及其对氮循环贡献的研究进展
1
2015
... id="C38">本研究结果显示, 土壤含水量对高寒草原土壤N2O通量起着明显的调控作用.增温导致了土壤含水量的显著下降(
氨氧化古菌及其对氮循环贡献的研究进展
1
2015
... id="C38">本研究结果显示, 土壤含水量对高寒草原土壤N2O通量起着明显的调控作用.增温导致了土壤含水量的显著下降(
Increasing aridity reduces soil microbial diversity and abundance in global drylands
1
2015
... id="C37">本研究结果表明, 增温并没有显著改变青藏高原高寒草原土壤N2O通量.一般来讲, 作为硝化和反硝化等生物地球化学过程的产物, 土壤N2O通量会受到土壤温度变化的影响.以往的研究显示, 土壤温度的上升会促进土壤N矿化, 提高土壤无机氮的含量, 为硝化和反硝化过程提供更充足的底物(
The emissions of nitrous oxide and methane from natural soil temperature gradients in a volcanic area in southwest Iceland
1
2017
... id="C5">近10年来, 国内外****相继开展了土壤N2O对增温响应方面的研究.多数研究报道增温会促进不同生态系统中土壤N2O的排放(
2
2008
... id="C7">本研究在青海省刚察县三角城种羊场(地理位置37.30° N, 100.25° E, 海拔3β290 m)进行.该地区属高原大陆性气候, 1980-2012年年平均气温为0.08 ℃, 年降水量为387 mm (
... (
Linkages of plant stoichiometry to ecosystem production and carbon fluxes with increasing nitrogen inputs in an alpine steppe
1
2017
... id="C4">氧化亚氮(N2O)是大气中一种重要的温室气体, 其温室效应是CO2的298倍(
Nitrous oxide (N2O): The dominant ozone-depleting substance emitted in the 21st Century
1
2009
... id="C5">近10年来, 国内外****相继开展了土壤N2O对增温响应方面的研究.多数研究报道增温会促进不同生态系统中土壤N2O的排放(
Nitrification in forested ecosystems
2
1982
... id="C5">近10年来, 国内外****相继开展了土壤N2O对增温响应方面的研究.多数研究报道增温会促进不同生态系统中土壤N2O的排放(
... id="C19">土壤样品AOA和AOB的amoA基因丰度通过定量PCR反应检测.反应在StepOne Plus PCR System (Applied Biosystems, Carlsbad, USA)中进行.反应使用的引物分别为AOA: amoAF(5’-STAATGGTCTGGCTTAGACG-3’)/amoAR(5’-GCGGCCATCCATCTGTATGT-3’); AOB: amoA1F(5’-GGGGTTTCTACTGGTGGT-3’)/amoA2R(5’-CCCCTCKGSAAAGCCTTCTTC-3’)(
The ammonia monooxygenase structural gene amoA as a functional marker: Molecular fine-scale analysis of natural ammonia-?oxidizing populations.
3
1997
... id="C5">近10年来, 国内外****相继开展了土壤N2O对增温响应方面的研究.多数研究报道增温会促进不同生态系统中土壤N2O的排放(
... O排放(
... id="C37">本研究结果表明, 增温并没有显著改变青藏高原高寒草原土壤N2O通量.一般来讲, 作为硝化和反硝化等生物地球化学过程的产物, 土壤N2O通量会受到土壤温度变化的影响.以往的研究显示, 土壤温度的上升会促进土壤N矿化, 提高土壤无机氮的含量, 为硝化和反硝化过程提供更充足的底物(
The combined effects of warming and drying suppress CO2 and N2O emission rates in an alpine meadow of the eastern Tibetan Plateau
1
2012
... id="C38">本研究结果显示, 土壤含水量对高寒草原土壤N2O通量起着明显的调控作用.增温导致了土壤含水量的显著下降(
Responses of a tundra system to warming using SCAMPS: A stoichiometrically coupled, acclimating microbe-plant-soil model
2
2014
... id="C37">本研究结果表明, 增温并没有显著改变青藏高原高寒草原土壤N2O通量.一般来讲, 作为硝化和反硝化等生物地球化学过程的产物, 土壤N2O通量会受到土壤温度变化的影响.以往的研究显示, 土壤温度的上升会促进土壤N矿化, 提高土壤无机氮的含量, 为硝化和反硝化过程提供更充足的底物(
... id="C38">本研究结果显示, 土壤含水量对高寒草原土壤N2O通量起着明显的调控作用.增温导致了土壤含水量的显著下降(
N2O flux from plant-soil systems in polar deserts switch between sources and sinks under different light conditions
2
2012
... id="C38">本研究结果显示, 土壤含水量对高寒草原土壤N2O通量起着明显的调控作用.增温导致了土壤含水量的显著下降(
... O产生量也没有显著差异(
Nitrifiers and denitrifiers respond rapidly to changed moisture and increasing temperature in a pristine forest soil
2
2010
... id="C10">我们于2013年6月在对照和增温处理中分别布设了一个40 cm × 40 cm的不锈钢底座, 底座打入土壤约6 cm.在2014和2015年的生长季(5-10月)利用静态箱-气相色谱法(
... id="C11">土壤N2O排放通量用以下公式(
Soil-atmospheric exchange of CO2, CH4, and N2O in three subtropical forest ecosystems in southern China
1
2006
... id="C16">土壤样品采用1 mol·L-1 KCl溶液浸提, 之后采用连续流动分析仪(Autoanalyzer 3 SEAL, Bran and Luebbe, Norderstedt, Germany)测定浸提液中的NH4+-N和NO3--N含量, 二者相加得到土壤总无机氮(SIN)含量.土壤微生物碳(MBC)和微生物氮(MBN)采用氯仿熏蒸法(
Land-use and soil depth affect resource and microbial stoichiometry in a tropical mountain rainforest region of southern Ecuador
1
2014
... id="C16">土壤样品采用1 mol·L-1 KCl溶液浸提, 之后采用连续流动分析仪(Autoanalyzer 3 SEAL, Bran and Luebbe, Norderstedt, Germany)测定浸提液中的NH4+-N和NO3--N含量, 二者相加得到土壤总无机氮(SIN)含量.土壤微生物碳(MBC)和微生物氮(MBN)采用氯仿熏蒸法(
An extraction method for measuring soil microbial biomass C
1
1987
... id="C5">近10年来, 国内外****相继开展了土壤N2O对增温响应方面的研究.多数研究报道增温会促进不同生态系统中土壤N2O的排放(
Warming of subarctic tundra increases emissions of all three important greenhouse gases—Carbon dioxide, methane, and nitrous oxide
1
2016
... id="C37">本研究结果表明, 增温并没有显著改变青藏高原高寒草原土壤N2O通量.一般来讲, 作为硝化和反硝化等生物地球化学过程的产物, 土壤N2O通量会受到土壤温度变化的影响.以往的研究显示, 土壤温度的上升会促进土壤N矿化, 提高土壤无机氮的含量, 为硝化和反硝化过程提供更充足的底物(
Temperature sensitivity of gross N transformation rates in an alpine meadow on the Qinghai-Tibetan Plateau
2
2016
... id="C38">本研究结果显示, 土壤含水量对高寒草原土壤N2O通量起着明显的调控作用.增温导致了土壤含水量的显著下降(
... id="C39">本研究结果表明, 高寒草原土壤N2O通量呈现明显的年际变化.在两年观测期间, 2014年生长季降水量较为丰沛(534.7 mm), 而2015年生长季降水量明显偏低(371.7 mm), 导致土壤含水量明显低于2014年(
土壤微生物对增温响应的Meta分析
2
2015
... id="C5">近10年来, 国内外****相继开展了土壤N2O对增温响应方面的研究.多数研究报道增温会促进不同生态系统中土壤N2O的排放(
... id="C37">本研究结果表明, 增温并没有显著改变青藏高原高寒草原土壤N2O通量.一般来讲, 作为硝化和反硝化等生物地球化学过程的产物, 土壤N2O通量会受到土壤温度变化的影响.以往的研究显示, 土壤温度的上升会促进土壤N矿化, 提高土壤无机氮的含量, 为硝化和反硝化过程提供更充足的底物(
土壤微生物对增温响应的Meta分析
2
2015
... id="C5">近10年来, 国内外****相继开展了土壤N2O对增温响应方面的研究.多数研究报道增温会促进不同生态系统中土壤N2O的排放(
... id="C37">本研究结果表明, 增温并没有显著改变青藏高原高寒草原土壤N2O通量.一般来讲, 作为硝化和反硝化等生物地球化学过程的产物, 土壤N2O通量会受到土壤温度变化的影响.以往的研究显示, 土壤温度的上升会促进土壤N矿化, 提高土壤无机氮的含量, 为硝化和反硝化过程提供更充足的底物(
Warming effects on greenhouse gas fluxes in peatlands are modulated by vegetation composition
1
2013
... id="C16">土壤样品采用1 mol·L-1 KCl溶液浸提, 之后采用连续流动分析仪(Autoanalyzer 3 SEAL, Bran and Luebbe, Norderstedt, Germany)测定浸提液中的NH4+-N和NO3--N含量, 二者相加得到土壤总无机氮(SIN)含量.土壤微生物碳(MBC)和微生物氮(MBN)采用氯仿熏蒸法(
1
2006
... id="C5">近10年来, 国内外****相继开展了土壤N2O对增温响应方面的研究.多数研究报道增温会促进不同生态系统中土壤N2O的排放(
1
2006
... id="C5">近10年来, 国内外****相继开展了土壤N2O对增温响应方面的研究.多数研究报道增温会促进不同生态系统中土壤N2O的排放(
模拟增温对荒漠生物土壤结皮-土壤系统CO2、CH4和N2O通量的影响
1
2014
... id="C5">近10年来, 国内外****相继开展了土壤N2O对增温响应方面的研究.多数研究报道增温会促进不同生态系统中土壤N2O的排放(
模拟增温对荒漠生物土壤结皮-土壤系统CO2、CH4和N2O通量的影响
1
2014
... id="C5">近10年来, 国内外****相继开展了土壤N2O对增温响应方面的研究.多数研究报道增温会促进不同生态系统中土壤N2O的排放(
Legacy effects of simulated short-term climate change on ammonia oxidisers, denitrifiers, and nitrous oxide emissions in an acid soil
1
2017
... id="C5">近10年来, 国内外****相继开展了土壤N2O对增温响应方面的研究.多数研究报道增温会促进不同生态系统中土壤N2O的排放(
Responses of CO2, N2O and CH4 fluxes between atmosphere and forest soil to changes in multiple environmental conditions
1
2014
... id="C6">高寒草原是青藏高原面积最大的草地生态系统, 其分布面积达6.4 × 105 km2 (
A meta-analysis of the effects of experimental warming on soil carbon and nitrogen dynamics on the Tibetan Plateau
2015