
Research advancement in the processes and mechanisms of transporting methane by emerged herbaceous plants and hygrophytes
DOUBo-Kai
通讯作者:
收稿日期:2017-03-31
接受日期:2017-11-14
网络出版日期:2017-11-10
版权声明:2017植物生态学报编辑部本文是遵循CCAL协议的开放存取期刊,引用请务必标明出处。
展开
摘要
关键词:
Abstract
Keywords:
-->0
PDF (2089KB)元数据多维度评价相关文章收藏文章
本文引用格式导出EndNoteRisBibtex收藏本文-->
甲烷(CH4)是大气中的一种痕量气体, 它参与大气光化学反应(Montzka et al., 2011), 且对全球变暖有重要影响。CH4的单分子增温潜势是CO2的28倍(IPCC, 2013), 对温室效应的贡献率为14%, 仅次于CO2。工业革命后大气中的CH4浓度在不断增加, 2011年达到80.79 × 10-3 μmol·L-1, 比工业革命前增加了1.5倍(IPCC, 2013)。
大气中的CH4有70%-80%来自生物源(Mer & Roger, 2001), 其中水生与湿生环境是两个主要排放源(Mer & Roger, 2001; Bridgham et al., 2013)。CH4排放是产生、消耗(氧化)和传输3个环节综合作用的结果; 但是与产生和消耗二者相比, 目前对于传输的研究比较薄弱(Mer & Roger, 2001; 丁维新和蔡祖聪, 2003; 陈槐等, 2006; 冯小平等, 2015)。在水生和湿生环境中, CH4传输有3种途径: 扩散、气泡和植物传输, 其中常以挺水与湿生草本植物传输为主(Mer & Roger, 2001; 张晓艳等, 2012; Berg et al., 2016; Andresen et al., 2017)。在生长旺盛期, 植物传输排放量可占总排放量的90% (Schütz et al., 1989b; Whiting & Chanton, 1992; Chanton et al., 2002)。因此, 认识挺水与湿生草本植物传输CH4的过程与机制具有重要的理论和现实意义。众所周知, 挺水与湿生草本植物具有发达的通气组织, 其气体输导作用被认为是能够高效传输CH4的主要原因, 因为通气组织在主动向根系输送氧气(O2)的同时, 也被动传输CH4 (Yu et al., 1997; 成水平等, 2003; Colmer, 2003), 而且这种CH4高传输能力进一步减少了CH4的氧化损失(Schimel, 1995)。但是, 深入认识植物传输CH4的内在过程与机制需要系统的科学研究工作。近几十年来, 植物究竟如何传输CH4这一科学问题受到了越来越多的关注, 且取得了系列研究进展。鉴于此, 本文系统综述了挺水与湿生草本植物传输CH4方面的研究进展, 梳理了整体传输过程与机制, 并形成了较为完整的框架图(图1), 以期引起国内同行的重视并合力推动此领域的研究进程。
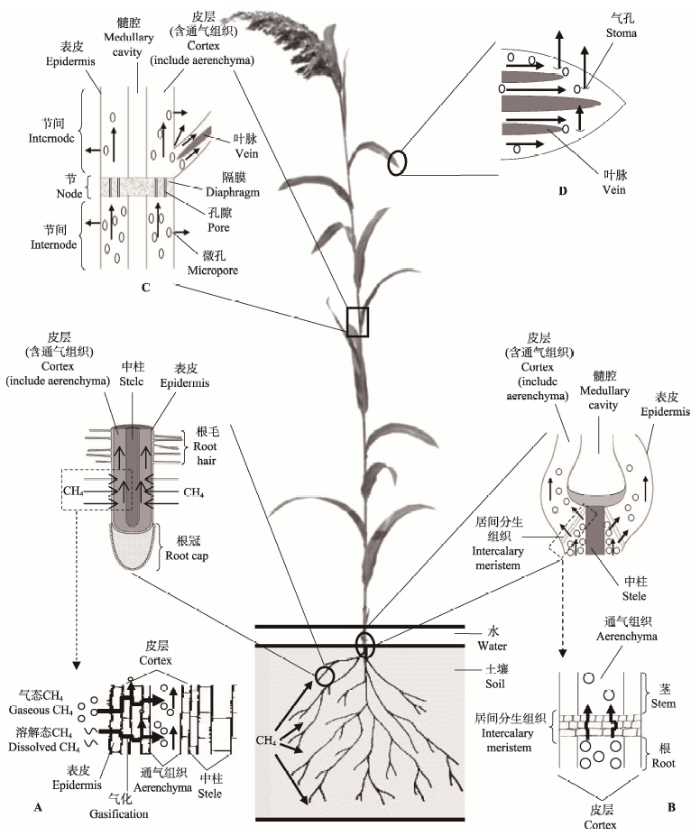
图1植物传输CH4的关键过程与机制示意图。A, 根系部位。B, 根茎连接部位。C, 茎节与节间部位。D, 叶片部位。箭头表示CH4传输方向。
-->Fig. 1Illustrations of key transporting processes and mechanisms of methane by emerged herbaceous plants and hygrophytes. A, root zone. B, root collar zone. C, node and internode of stem zone. D, leaf zone. The arrows indicate the direction of methane flows.
-->
1 土-水环境中CH4的赋存状态与运动机制
自然环境下, CH4由于自身沸点低, 主要以气态的方式存在, 其密度在标准状况下为0.717 g·L-1, 要低于近地面大气的密度, 这可能有利于其在植物通气组织内向上扩散传输并排放到大气中。同时CH4作为一种非极性分子, 溶解度低(Harden & Chanton, 1994), 标准状态下在水中的溶解度为0.033 g·L-1 (Yu et al., 1997), 因此容易气化或者脱离水相体系。认识CH4的这些基本性质是深入探讨其传输与排放机制的理论基础。湿地土-水环境中, CH4在产生率不高时一般不会或极少产生气泡, 此时溶解态的CH4主要以液相扩散的方式进行扩散迁移(杨平和仝川等, 2015)。但是, 当CH4产生速率高、溶解态CH4达到饱和并且不能及时被消耗和转运时, CH4气体分子则会不断聚集, 形成气体分子团, 并主要以此气体形式赋存在土-水环境中(张晓艳等, 2012)。气体在液相中的传输速率比在气相中慢约104倍, 加之土壤颗粒结构等物理阻隔影响, CH4气体在湿地土-水环境中的扩散速率十分缓慢。可见, CH4在土-水环境中的这种赋存状态与运动机制为挺水与湿生草本植物高效传输CH4提供了前提条件。此外, 如果CH4的产生速率远远大于其消耗与转运的速率, 部分CH4将以气泡的形式传输并排放, 这种情况主要发生在没有挺水与湿生草本植物生长的环境(Mer & Roger, 2001)。
2 植物各界面的CH4传输过程与机制
2.1 根际
土-水环境中, CH4由根际进入根系是植物传输CH4的初始过程。根系被认为是植物与土-水-气系统间进行气体交换的关键部位(Beckett et al., 2001; Hu et al., 2016)。室内控制实验结果表明, 气态的CH4可直接进入根系(Nouchi et al., 1990), 但其传输机制尚不清楚。相比之下, 溶解态的CH4进入根系并传输的过程则较为明确: 根际溶解态的CH4首先通过表皮层, 然后进入皮层并在此气化或脱离水相体系(可能是由于植物细胞主动吸收水分而未吸收CH4的原因), 气化后的CH4经细胞间隙与通气组织向植物上部传输(Wang et al., 1997; 贾仲君和蔡祖聪, 2003; 图1A)。然而, 根际界面CH4传输过程是被动扩散渗透过程还是根系主动吸收过程尚不明确。Nouchi等(1990)认为根际自土-水环境向根内形成了较大CH4浓度梯度, 从而促成了被动扩散渗透过程。然而, 也有****认为这是植物主动吸收的过程(王明星等, 1998; 曹云英等, 2003)。
根系不同部位的CH4传输性能被发现存在差异。一方面, 从整个根系系统来看, Henneberg等(2012)在对灯心草(Juncus effusus)的研究中发现, CH4主要通过侧根进入植物体。这可能是由于侧根是植物根际物质交换的主要部位(Colmer, 2003), 而且侧根的出现往往会增加植物对外界物质的吸收与输导(杨世杰等, 2000)。另一方面, 随着对根系泌氧的深入研究, 针对同一条根不同位置的CH4传输 性能也有了更清楚的认识, 根尖后几厘米处被认 为是根系传输CH4的主要位置(Visser et al., 2000; Henneberg et al., 2012)。曹云英等(2003, 2005)通过对水稻(Oryza sativa)进行剪根与封根实验, 也进一步证实了这一结论, 并指出自根尖部位到根基部, 对于CH4传输的贡献呈下降趋势。解剖实验发现, 根系通过表层细胞壁的明显木质化与栓质化等次生 变化以减少根系泌氧损失、从而应对缺氧环境(Garthwaite et al., 2008), 而这一点在根基部更为明显(Jackson & Armstrong, 1999; Visser et al., 2000), 这大大减弱了根基部的渗透性, 阻碍了CH4自根际进入根系(Beckett et al., 2001)。相对于水中溶解态的CH4, 针对气态CH4直接进入根系的主要位置研究较少, 仅在新生根长出时出现的裂缝与开口处被发现(Aulakh et al., 2000b)。此外, 根部细胞的排列方式会影响到根系孔隙度(Justin & Armstrong, 1987), 从而影响CH4传输。根系的一些生物特性也被发现与CH4传输存在相关性, 如: 傅志强等(2012)发现晚稻在分蘖盛期时根质量与CH4排放通量存在正相关关系; Henneberg等(2012)也指出灯心草的细根根数、根长与CH4传输有明显的正相关关系。
2.2 根茎连接部位
根茎连接部位是CH4由根系进入茎叶的过渡部位, 它在结构上与根、茎存在明显差异。根茎连接部位对CH4传输影响显著: 无论是早期Butterbach- Bahl等(1997)对水稻根茎结合部位进行的剪切试验, 还是后来Groot等(2005)的六氟化硫示踪试验, 均表明根茎连接部位具有较大的气体传输阻力, 是调控植物传输CH4整个过程的一个重要部位(丁维新和蔡祖聪, 2003)。这是因为根茎连接部位分布着紧密的居间分生组织, 且缺乏通气组织, 使得气态CH4主要在狭窄的细胞间隙中运动并被传输(Brix et al., 1992; Aulakh et al., 2000b; Groot et al., 2005; 图1B)。有些植物在根茎连接部位发生分蘖, 其分蘖数量与群落的CH4传输速率呈现显著正相关(Singh et al., 1998; Wassmann & Aulakh, 2000; 曹云英等, 2005), 即分蘖增加了CH4传输排放的通道数量。此外, Groot等(2005)还指出根茎过渡部位的CH4传输与分蘖部位总横截面积呈正相关关系, 而与分蘖类型无关。2.3 通气组织
通气组织是CH4在植物内长距离高效传输的主要通道和载体, 因此其结构与形态对于CH4传输十分重要。研究指出, 通气组织的数量、大小与CH4传输通量存在正相关关系(Aulakh et al., 2000b), 即数量较多或形态较大的通气组织会显著提高CH4的传输速率。通气组织的内部结构直接影响着CH4传输效率, 特别是许多植物在通气组织内部发育了隔膜; 虽然隔膜上有孔隙存在, 但隔膜显著阻碍了CH4的传输通道, 对于CH4的长距离高效传输产生了一定阻力(图1C), 特别是在对流传输过程中(Schuette et al., 1994; Sorrell et al., 1997; Colmer, 2003)。但是, 对隔膜如何影响CH4的长距离传输, 目前仍缺乏深入认识。2.4 茎叶-大气界面
茎叶向大气排放CH4是植物传输CH4的最终环节, 目前针对茎叶排放CH4位置方面的研究较多。早期, 气孔被认为是CH4传输排放的重要部位(Morrissey et al., 1993; 图1D), 这在宽叶香蒲(Typha latifolia)(Knapp & Yavitt, 1992)、Carex aquatilis (Schimel, 1995)的观测中得以证实。但与此同时, 一些****通过亮/暗对比试验(Lee et al., 1981)、CO2调控试验(Seiler et al., 1984), 发现气孔并非CH4排放的主要通道, 而微孔(位于地上部除叶片以外的细小的裂隙与孔洞)才是CH4排放的主要位置(图1C)(Nouchi & Mariko, 1993)。此后, 植物茎叶排放CH4的其他部位(除叶片外)不断地被发现, 例如: Harden和Chanton (1994)发现Pontederia cordata和Sagittaria lancifolia的叶柄是茎叶排放CH4的关键部位; Yu等(1997)指出水稻的叶鞘基部可排放CH4; 而在芦苇(Phragmites australis)与Scirpus lacustris淹水实验(van der Nat & Middelburg, 2000)、互花米草(Spartina alterniflora)的静态箱测量实验(黄佳芳等, 2011)等研究中, 茎秆基部(30 cm以下位置)被发现是茎叶排放CH4的主要部位, 且CH4很可能在此部位通过微孔得到排放。需要指出的是, 在相同研究时期, 针对同一植物, 不同研究者报道的CH4排放关键部位也存在不同, 例如, 针对Carex aquatilis在6-8月的研究中: Schimel (1995)研究指出叶片是CH4排放的主要部位, 而Kelker和Chanton (1997)通过茎秆刈割实验发现CH4的排放部位主要在茎秆下部。这一矛盾结果可能源于以下几个原因: 植物排放CH4的部位多且复杂、并且受到生物与环境因子的影响, 此外还可能是实验误差。除了活体植物外, 受损伤的、枯死的植株或部位也是CH4的传输排放通道。当存在枯萎的植株时, 其内部的静态压力比鲜活、完整植株低, 这促使植物内气体多通过鲜活完整的植株向地下输送, 而由受损或枯死植株传输排出(Sebacher et al., 1985; Armstrong & Armstrong, 1991; Yavitt & Knapp, 1998; Berg et al., 2016)。因此, 受损伤、枯死的植株或部位常会促进CH4的排放(Brix, 1989; Dingemans et al., 2011)。例如, Kelker和Chanton (1997)通过模拟损伤试验, 发现Carex aquatilis的CH4排放量在损伤后比损伤前增加了97%-111%。这可能是由于破损、枯死的植株或部位CH4排放阻力较低, 从而促进了CH4排放(Yavitt & Knapp, 1998; Petruzzella et al., 2015)。
3 植物体内CH4长距离传输机制
3.1 扩散
扩散是气体分子在连通空间内由浓度差而诱发的自高浓度向低浓度的运动, 常与分子扩散系数、浓度梯度差以及连接不同空间通道的面积呈正相关关系(成水平等, 2003), 其中浓度梯度是主要的驱动因子(段晓男等, 2005; 杨平和仝川, 2015)。气体扩散易受温度的影响(Whiting & Chanton, 1996; 段晓男等, 2005), 从而使扩散作用因不同时空下外界温度的变化而产生差异。目前在Scirpus lacustris、Carex gracilis (van der Nat et al., 1998)、水稻(Bodegom et al., 2001)等植物内均发现存在CH4的扩散机制。扩散传输对于CH4短距离传输十分重要, 特别是在传输阻力较大的界面, 但是扩散作用如何影响整个 传输过程?是否起到主导作用?这些问题仍需要深入研究。3.2 对流
对流是植物传输CH4的另一种重要机制。不同于扩散传输, 对流传输主要依靠植物内外的压强差来驱使气体运动(Grosse et al., 1991; Brix et al., 1992; 杨平和仝川, 2015)。对流在输送效率(Grosse et al., 1991; Brix et al., 1992; Chanton et al., 1992; 段晓男等, 2005)和物种适应厌氧环境(Brix et al., 1992; Tornbjerg et al., 1994; Laanbroek, 2010)方面均明显优于扩散传输作用。对流促进植物地上部分向根系输送O2的同时, 也更有利于CH4的反向传输(Sorrell & Boon, 1994)。Eleocharis sphacelata (Sorrell & Boon, 1994)、芦苇(van der Nat et al., 1998)、互花米草(段晓男等, 2005)等均被证实利用对流机制传输CH4。对流传输受到许多环境因素的影响, 如湿度、温度(Bendix et al., 1994)、光照(Whiting & Chanton, 1996)、风(Arkebauer et al., 2001)等, 其中以湿度和温度的调控作用更为普遍(Brix et al., 1992; 成水平等, 2003; 杨平和仝川, 2015)。温度主要通过植物体内外或植物不同部位间的温度梯度来诱导对流, 而湿度的诱导作用更多来源于水汽压差(Brix et al., 1992)。综上所述, 植物通过扩散与对流作用将土-水环境中产生的CH4传输到大气中, 对CH4释放通量起到了重要作用。但二者的传输性能存在差异: 扩散机制无处不在, 但传输效率较低; 对流机制对形成条件要求相对较高, 但传输效率也较高。挺水与湿生草本植物均基于自身生理结构与外界条件协调使用两种机制以完成CH4传输过程, 这是它们适应厌氧环境进化的结果。但是, 目前对于扩散与对流分别对各界面交换与整体长距离传输的内在调控作用、不同物种间的机制差异性认识不足, 有待深入研究。
4 CH4传输的生物与环境影响机制
4.1 生物影响机制
4.1.1 物种类型物种类型是植物传输CH4能力的重要影响因素(Whiting & Chanton, 1996; Han et al., 2017; Lawrence et al., 2017); 而且同一物种不同品种也存在差异。例如, 水稻不同品种间的CH4传输效率差异明显, 这得到了许多研究的证实(Lindau et al., 1995; Neue et al., 1996; 傅志强等, 2012)。物种间CH4传输效率的差异通常归因于它们不同的形态结构, 其中外部形态包括根系体积与质量(傅志强等, 2012)、分蘖特性(Schimel, 1995; 曹云英等, 2005)等; 内部结构包括通气组织的形态与发达程度(Sebacher et al., 1985; Butterbach-Bahl et al., 1997)等。值得一提的是, 群落组成结构(物种丰富度等)如何影响CH4传输尚无一致结论(Zhang et al., 2012; Mo et al., 2015; Zhao et al., 2016)。
4.1.2 生育阶段
许多****发现水稻在生长初期的CH4传输排放量较小, 但是随着生长发育不断变化, 一般在抽穗中期或开花期CH4传输排放达到峰值, 之后逐渐减小(Seiler et al., 1984; Schütz et al., 1989a; 王明星等, 1998; Aulakh et al., 2000a)。同样, 芦苇在夏季的CH4传输排放量也远高于冬季(Brix et al., 1996; 段晓男等, 2007)。这是因为, 在生育初期, 通气组织的发育程度较低, CH4传输通道不足(丁维新和蔡祖聪, 2003; 张晓艳等, 2012; Reid et al., 2015), 而在生育后期, 根系逐渐老化、特别是根表皮层渗透性的显著降低(Nouchi et al., 1994), 同样明显减弱了CH4传输量。除此之外, Kim等(1999)发现分蘖前芦苇传输CH4主要通过扩散方式, 而分蘖之后对流方式越来越占主导地位。Wang等(1997)指出在生育早期, 叶片是水稻排放CH4的主要部位; 而在生育后期, 茎节点是排放CH4的主要部位。可见, 挺水与湿生草本植物传输CH4的机制(关键部位等)受到了植物生长发育的调控。
4.1.3 生物量
生物量由于通常与通气组织数量密切相关, 因而影响CH4传输。植物地上生物量与CH4传输排放存在显著正相关关系, 这已在湿生薹草(Carex limosa)和灰株薹草(Carex rostrata) (Whiting & Chanton, 1992)、水稻(Huang et al., 1997)和Typha glauca (Lawrence et al., 2017)等许多相关研究中得到验证。然而, 相比于地上部, 对于地下生物量如何影响CH4传输排放的研究相对较少; 其中, 一些研究指出二者存在正相关关系(Mishra et al., 1997; Hu et al., 2016); 然而, 仝川等(2009)发现闽江口芦苇潮汐湿地的CH4排放通量与地下生物量并不相关, Reid等(2015)同样报道地下生物量并不能很好地预测CH4排放量。可见, 植物地下生物量如何影响CH4传输排放需要进一步研究。
4.1.4 光合作用
光合作用对植物传输CH4的影响作用尚无定论。早期有****指出, 植物的CH4传输效率并不依赖于光合作用(Seiler et al., 1984)。然而, 随后一些研究表明, 光合速率的提升往往会促进CH4的传输排放(King & Reeburgh, 2002; Berg et al., 2016), 但是光合作用的影响机制多表现在间接作用, 如提供较多的根系分泌物(Whiting & Chanton, 1992)等, 而在直接影响作用方面目前研究较少。
4.1.5 蒸腾作用
较早的研究发现, CH4在植物内基于液流的传输量很少, 这是因为CH4的传输速率并不依赖于蒸腾速率(Lee et al., 1981)。同样, Nouchi等(1990)与Wang等(1997)均指出蒸腾作用对植物传输CH4没有明显的直接影响。但值得一提的是, 有些****指出蒸腾作用可以通过降低叶片的温度(Grosse et al., 1991; Brix et al., 1992)加大植物体内外的温度差异, 最终促进由温度诱导的CH4传输, 从而起到间接作用。此外, 由于蒸腾拉力是植物吸收与运输水分的主要动力(杨世杰等, 2000), 而根系既是植物吸水主要部位, 也是CH4进入的位置, 因此深入研究蒸腾作用对CH4进入根系的方式与数量的影响具有重要意义, 需要进一步探讨。
4.2 环境影响机制
4.2.1 光照植物在光照充足条件下CH4的传输速率往往高于在弱光或无光照情况下(Armstrong & Armstrong, 1991; Brix et al., 1996; Berg et al., 2016)。Chanton等(1993)对Typha domingensis和宽叶香蒲排放CH4的日变化研究中, 以及K?ki等(2001)对芦苇排放CH4的昼夜差异研究中, 均证实光照是白天CH4传输排放速率高于夜晚的主要影响原因。光照对植物传输CH4的作用机理主要体现在以下几个方面: 首先, 在一定范围内, 光照增加会增强光合作用(Armstrong & Armstrong, 1991), 从而间接影响CH4传输。其次, 光照的刺激往往会促进气孔的开放(成水平等, 2003), 这有助于植物与大气间的气体交换, 从而影响CH4传输排放。最后, 光照会提高植物内的温度或湿度, 加大由温度和湿度诱导的气体对流, 促进CH4对流传输(Armstrong & Armstrong, 1990; 成水平等, 2003)。此外, CH4的对流与扩散传输机制对光照变化的响应存在较大的差异: 对流传输对光照较为敏感(Whiting & Chanton, 1992), 而光照对扩散作用的影响并不显著(段晓男等, 2005, 2007)。因此, 光照的改变常常会使一些植物的CH4传输机制发生昼夜变化, 在白天以更高效的对流机制为主, 在夜晚则以永恒的扩散传输为主(Whiting & Chanton, 1996; Arkebauer et al., 2001; Berg et al., 2016)。
4.2.2 温度
温度对植物内CH4的对流传输有着十分重要的调控作用。研究发现, 当外界温度较高时, 植物内部的对流速率往往也会提高(Armstrong & Armstrong, 1991; Brix et al., 1996)。这在随后的芦苇(Arkebauer et al., 2001)、灯心草(Garnet et al., 2005)等研究中得到进一步证实。温度诱导的CH4对流传输机制主要是由植物体内外的温度差引起的, 温度差诱导产生压力差, 促使气体从低温处向高温处扩散传输(刘泽雄, 2011)。因此, 外界温度的升高, 常常有利于植物对CH4的传输与排放。
4.2.3 湿度
湿度是影响植物传输CH4的又一重要环境因子。早期, Armstrong和Armstrong (1991)对芦苇的室内试验发现, 同在24 ℃中, 处于相对湿度为41%环境下植物的对流速率是相对湿度为74%环境下的3.5倍。后期大量研究同样表明, 湿度诱导下的对流作用会随着外界湿度的增加而减弱(Bendix et al., 1994; Brix et al., 1996; Yavitt & Knapp, 1998)。在我国, 刘泽雄(2011)发现闽江河口芦苇内部的CH4浓度与湿度显著正相关, 从而加大内外的CH4浓度差, 但是较高的湿度不利于对流传输。
4.2.4 风
风会影响植物的气体交换性能(Armstrong et al., 1991, 1992)。例如, 当空气流经芦苇较高处枯萎或受损伤的部位时, 在较短时间内会使局部气压降低而产生吸力, 使得茎和根茎部位的气体被吸引上来(Armstrong et al., 1992, 1996; Colmer, 2003), 从而利于CH4由植物体向大气的传输与排放。Armstrong等(1996)进一步指出, 这种效应在冬天或生长季的夜晚, 当湿度诱导的对流传输较慢或缺乏时, 会变得更为明显。虽然风对传输有显著影响, 但相对于其他环境因素, 关于风如何影响CH4传输的研究较少。
4.2.5 水位
水位显著影响植物对CH4的传输排放。在对芦苇、水稻等物种的水位调控及密闭箱收集试验中发现, 较高的水位抑制CH4的传输排放(Wang et al., 1997; 马安娜和陆健健, 2011)。这通常被认为是较高的水位淹没了茎基部的CH4排放关键区域(微孔等)(van der Nat & Middelburg, 2000; 马安娜和陆健健, 2011)。然而, Wang等(1997)发现, 将水位提升至12.5 cm后, 水稻对CH4的传输排放速率在7 h内增长了约30%; 其中一个原因可能是因为增高水位淹没了基部微孔, 而近基部微孔进一步变成了主要排放通道, 另一个原因可能是进一步加深淹水促进了CH4产生, 从而增加了CH4排放。
4.2.6 土壤性质
土壤性质通过影响植物形态结构与生理活动, 进而影响CH4传输, 但是相关研究较少。例如盐分的增加会促进喜旱莲子草(Alternanthera philoxeroides)通气组织的发展, 使根、茎与叶部位胞间隙相连(刘爱荣等, 2007), 却抑制了某些物种的气孔度与光合作用(Munns, 1993; 岳健敏等, 2015), 使盐分对植物的CH4传输性能的影响过程与机制变得更加复杂。Kludze等(1993)在研究中发现氧化还原电位处于较低态势时, 会促进通气组织的形成而减小根质量及根长, 从而对植物传输CH4产生潜在影响。土壤氮含量适度增加会增强CH4释放(Singh et al., 1998; Zhang & Song, 2016), 这一方面可能与适量氮水平会促进植物特别是根系的生长有关(黄丹萍等, 2012), 另一方面部分氮在土壤中通过微生物会转化为氨, 其对CH4氧化有着竞争性抑制作用, 从而增加了CH4进入植物的潜在量(胡荣桂, 2004)。此外, 土中铁、锰的存在会导致水稻根表形成铁、锰胶膜, 从而影响CH4进入根系(王胜春等, 1998)。
5 结语
关于挺水与湿生草本植物如何传输CH4的问题已开展了较多研究, 取得了较多的进展, 但是一些关键问题亟需深入探讨:(1)当前研究多集中在植物整体CH4传输过程上, 而对于植物各关键界面(根际界面、根茎连接部位、通气组织隔膜、茎叶-大气界面)传输CH4的过程与机制缺乏深入认识, 特别是各个界面的传输效率如何?哪个部位会对整体传输起到决定性的影响作用?这些关键问题缺乏深入的定量描述。
(2)扩散、对流对各界面CH4交换与长距离CH4传输的内在协调调控作用仍不明确, 特别是针对扩散过程机理及其生物、环境响应机制。
(3)现有研究已关注到影响CH4传输的因素, 但多限于影响因子的单独作用。而实际的自然环境下CH4的传输往往是多因素综合作用的结果, 因此各影响因子之间的耦合作用机理需要重点关注。
(4)目前针对植物传输CH4问题的研究对象多以芦苇、水稻、薹草等物种为主, 而对于其他植物物种研究则相对较少。由于不同植物的根、茎、叶形态特征存在差异, 比较不同植物物种传输CH4机制的异同对于系统认识挺水与湿生草本植物如何传输CH4十分重要。
致谢 天津市应用基础与前沿技术研究计划 (15JCYBJC49200)和天津市高等学校创新团队资助项目(TD12-5037)
The authors have declared that no competing interests exist.
作者声明没有竞争性利益冲突.
参考文献 原文顺序
文献年度倒序
文中引用次数倒序
被引期刊影响因子
[1] | . |
[2] | . Abstract Emergent aquatic macrophytes in vegetated wetlands provide routes for methane (CH(4)) transport from sites of production in oxygen-poor sediments, where CH(4) concentrations are relatively high, to the atmosphere, which typically has much lower CH(4) concentrations. Transport can occur through aerenchymatous tissue via simple diffusion. Recently, the importance of convective throughflow (i.e., mass transport of gases through plants driven by pressure gradients) in enhancing gas transport has been demonstrated in several genera (e.g., Nuphar, Nymphaea, Nelumbo, Typha, and Phragmites). This study was conducted to elucidate the governing plant-mediated gas transport mechanisms in a midlatitude prairie wetland and to determine both their diel and seasonal variations and the importance of environmental controlling factors. Pressures inside culms of the two dominant emergent aquatic macrophytes (Scirpus acutus and Phragmites australis) were measured directly throughout the growing season and on selected days in midseason. Supporting measurements included solar radiation, air temperature, relative humidity, and windspeed. Results indicated pressures inside green healthy culms of Phragmites were above atmospheric pressure by up to 1650 Pa during the day. At night culm pressures were at or slightly above atmospheric. No pressurization was detected in Scirpus. Highest pressures in Phragmites occurred during midseason when biomass and foliage area index were at their maxima (920 g/m(2) and 2.8, respectively). High internal pressures also coincided with periods of high solar radiation (>500 W/m(2)), high temperature (>20 C), and low relative humidity (<60%). Periods of high internal pressures also coincided with periods of high CH(4) efflux from the wetland as measured in concomitant studies. Convective throughflow driven by internal pressure gradients in Phragmites thus explains much of the diel variation in methane efflux previously reported from this wetland. |
[3] | . The convective throughflow of gases found recently in Phragmites australis increased with increasing fluxes of photosynthetically active radiation. Despite this, the oxygen in the venting gases remained lower than in air and the convection is thought to depend mainly on a humidity-induced diffusion of atmospheric gases across the leaf sheaths. When convection was prevented, and aeration was dependent entirely upon diffusion, no photosynthetic enhancement of rhizome and root aeration could be detected. In terms of oxygen transport, rapid rates of convection were much more effective than diffusion and substantially enhanced the entry of oxygen into the rhizome system. This resulted in large increases in the efflux of oxygen into the rhizospheres of basal laterals and apical and sub-apical regions of the adventitious roots. This effect was attributed to a greater diffusion of oxygen from rhizome to root. Substantial diurnal fluctuations in rhizome oxygen regimes and in root oxygen efflux occurred. It is estimated that because of convection and diffusion, up to 0.31 kg of oxygen d 0908081 m 0908081 may enter the rhizome system of vigorous stands of Phragmites during summer months. By comparison, diffusion alone could be expected to supply less than 0.01 kg d 0908081 . Scanning electron micrographs of root-shoot junctions showed that the resistance to gaseous diffusion from rhizome to roots must be especially small in Phragmites . |
[4] | . |
[5] | . A Venturi-induced convection of gases through the Phragmites rhizome system is reported and the effects of this, and a humidity-induced convection previously described, are quantified. The Venturi-convection is driven by a pressure differential created by wind blowing across tall dead culms which sucks air into the underground system via culms snapped off close to ground level. The results demonstrate how wind, by increasing Venturi-induced convection, and how radiant energy, by increasing humidity-induced convection, raise the oxygen concentrations in the rhizome system, thereby causing much greater fluxes of oxygen into root and rhizosphere than are achieved if rhizome aeration is by diffusion alone. In laboratory experiments convective flow rates of as low as 000·7-100·4 0103 10 0908088 m 3 s 0908081 (approx. 20-40 mm min 0908081 in the rhizome) were sufficient to raise rhizome oxygen concentrations to 90% of atmospheric. The significance of this is discussed in connexion with pollution control by constructed wetlands, and photographic evidence is provided of the rhizosphere oxidizing activities in soil and anaerobic solution. A mathematical model is derived to help to evaluate the contributions of convection and diffusion in rhizome aeration, and to assess the likely effects of root and rhizosphere oxygen demands. |
[6] | . The pathways of diffusive, humidity- and Venturi-induced convective ventilations in Phragmites australis (Cav.) Trin. ex Steud., and the mechanisms of the convections are described. Experimental evidence, indicating those factors, e.g. low relative humidity (RH), light, large leaf-sheath area, which increase humidity-induced convection, and those which increase Venturi convections, e.g. high wind speed, are reviewed. The superior aerating effects of convective as opposed to diffusive ventilation within the plant and their influence on the rhizosphere are demonstrated experimentally and by mathematical modelling. |
[7] | . |
[8] | . A major portion (60鈥90%) of the methane (CH 4 ) emitted from rice fields to the atmosphere is transported through the aerenchyma of the rice plants. However, a rapid and accurate method to study the CH 4 transport capacity (MTC) of rice plants is not available. We developed a gas sampling and analytical device based on a closed two-compartment chamber technique and analyzed the enrichment of the CH 4 mixing ratio inside the shoot compartment of cylindrical cuvettes enclosing individual rice plants under ambient conditions. The computer-controlled analytical system consists of a gas chromatograph (GC) and a pressure-controlled autosampler for eight cuvettes (seven for plants and one for CH 4 -calibration gas). The system automates closure and opening of plant cuvettes using pneumatic pressure, air sample collection and injection into the GC, and CH 4 analysis. It minimizes sources of error during air sampling by continuously mixing headspace air of each cuvette, maintaining pressure and composition of the headspace inside the cuvettes, purging the dead volumes between the sampler induction tube and GC, and running a reference CH 4 -calibration gas sample in each cycle. Tests showed that the automated system is a useful tool for accurate sampling of headspace air of cylindrical cuvettes enclosing individual rice plants and enables rapid and accurate fully automated analysis of CH 4 in the headspace air samples. A linear relationship was obtained between CH 4 transported by rice plants of two cultivars (IR72, a high-yielding dwarf, and Dular, a traditional tall cultivar) and concentration of CH 4 up to 7,500 ppm used for purging the nutrient culture solution surrounding the roots in the root compartment of the chamber. Further increase in CH 4 emission by shoots was not observed at 10,000 ppm CH 4 concentration in the root compartment of the chamber. The MTC of IR72 was measured at six development stages; it was lowest at seedling stage, increasing gradually until panicle initiation. There was no further change at flowering, but a marked decrease at maturity was noted. These results suggest that the plants have 45鈥246% greater potential to transport CH 4 than the highest CH 4 emission rates reported under field conditions, and plants would not emit CH 4 at early growth and at a reduced rate close to ripening. |
[9] | . Abstract: Aerenchyma, developed in both root and aboveground parts of rice plants, is predominantly responsible for plant-mediated transfer of methane (CH 4 ) from the soil to the atmosphere. To clarify the pathways of CH 4 transport through the rice plant and find differences that may determine the large0003variation in the patterns of methane transport capacity (MTC) of rice cultivars, we examined the appearance, the distribution pattern, and the density of aerenchyma in different parts of rice0003plants of three widely varying rice cultivars during panicle initiation, flowering, and maturity stages. The data on the amount and density of small (> 1 00030101 10 3 090808 5 00030101 10 3 0102m 2 ), medium (> 5 00030101 10 3 090808 20 00030101 10 3 0102m 2 ) and large aerenchyma lacunae (> 20 00030101 10 3 0102m 2 ) were collected using a computer assisted image-analyzing system. The brightfield optical microscopy of roots of all tested rice plants demonstrated the continuity of aerenchyma channels in the roots that function as conduits for bi-directional transport of gases. The aerenchyma channels of primary roots showed direct connection with those of culms. Intercalary meristems were found at the transition zone of rootaCculm aerenchyma connections. Well-developed aerenchyma lacunae present in the internodal region of the culm base were uniformly distributed in the peripheral cortical zone. The nodal region had relatively fewer and smaller aerenchyma lacunae that showed a non-uniform distribution pattern. As a result, few aerenchyma channels continued from the internodal region through to the nodal region. The aerenchyma in the cortex zone of the culm expanded along with the growing secondary tiller, developing continuity between the culm and the secondary tiller. The micrographs of longitudinal sections of different specimens of culmaCleaf sheath intersection showed the continuity of aerenchyma channels from the culm to the leaf. The amount of medium and large aerenchyma lacunae in the leaf sheath was respectively 2 and 33 times greater as compared to those of the tiller. The proportion of the large lacunae in the total amount of aerenchyma in leaf sheath was 75 % as compared to only 8 % in the tiller, revealing higher number and larger size of aerenchyma in the former. There were significant differences in amount and density of aerenchyma between individual cultivars at a given growth stage, as well as in the development patterns. While the amount and density of medium and small aerenchyma lacunae in the internodal region of the culm base did not show any relationship with MTC of rice cultivars, large aerenchyma lacunae exhibited highly significant correlations with MTC of different cultivars, suggesting that the wide variation in MTC of rice plants during different growth stages are related to these structural features. |
[10] | . Predictions of the quantities of methane which might be released via Phragmites roots to the atmosphere accord with examples of those previously reported from field data. |
[11] | . |
[12] | . Abstract Peatlands are interesting as a carbon storage option, but are also natural emitters of the greenhouse gas methane (CH4). Phragmites peatlands are particularly interesting due to the global abundance of this wetland plant (Phragmites australis) and the highly efficient internal gas transport mechanism, which is called humidity-induced convection (HIC). The research aims were to (1) clarify how this plant-mediated gas transport influences the CH4 fluxes, (2) which other environmental variables influence the CO2 and CH4 fluxes, and (3) whether Phragmites peatlands are a net source or sink of greenhouse gases. CO2 and CH4 fluxes were measured with the eddy covariance technique within a Phragmites-dominated fen in southwest Germany. One year of flux data (March 2013–February 2014) shows very clear diurnal and seasonal patterns for both CO2 and CH4. The diurnal pattern of CH4 fluxes was only visible when living, green reed was present. In August the diurnal cycle of CH4 was the most distinct, with 11 times higher midday fluxes (15.7 mg CH4 m6305 h6301) than night fluxes (1.41 mg CH4 m6305 h6301). This diurnal cycle has the highest correlation with global radiation, which suggests a high influence of the plants on the CH4 flux. But if the cause were the HIC, it would be expected that relative humidity would correlate stronger with CH4 flux. Therefore, we conclude that in addition to HIC, at least one additional mechanism must be involved in the creation of the convective flow within the Phragmites plants. Overall, the fen was a sink for carbon and greenhouse gases in the measured year, with a total carbon uptake of 221 g C m6305 yr6301 (26 % of the total assimilated carbon). The net uptake of greenhouse gases was 52 g CO2 eq. m6305 yr6301, which is obtained from an uptake of CO2 of 894 g CO2 eq. m6305 yr6301 and a release of CH4 of 842 g CO2 eq. m6305 yr6301. |
[13] | . A fully mechanistic model based on diffusion equations for gas transport in a flooded rice system is presented. The model has transport descriptions for various compartments in the water-saturated soil and within the plant. Plant parameters were estimated from published data and experiments independent of the validation experiment. An independent experiment is described in which the diffusion coefficient of sulfurhexafluoride (SF6) in water-saturated soil was determined. The model was validated by experiments in which transport of SF6 through soil and plant was monitored continuously by photoacoustics. The independent default settings could reasonably predict gas release dynamics in the soil-plant system. Calculated transmissivities and concentration gradients at the default settings show that transport within the soil was the most limiting step in this system, which explains why most gases are released via plant-mediated transport. The root-shoot interface represents the major resistance for gas transport within the plant. A sensitivity analysis of the model showed that gas transport in such a system is highly sensitive to the estimation of the diffusion coefficient of SF6, which helps to understand diel patterns found for greenhouse gas emissions, and to the root distribution with depth. This can be understood from the calculated transmissivities. The model is less sensitive to changes in the resistance at the root-shoot interface and in the root fraction active in gas exchange. The model thus provides an understanding of limiting steps in gas transport, but quantitative predictions of in situ gas transport rates will be difficult given the plasticity of root distribution. |
[14] | . Abstract Understanding the dynamics of methane (CH4 ) emissions is of paramount importance because CH4 has 25 times the global warming potential of carbon dioxide (CO2 ) and is currently the second most important anthropogenic greenhouse gas. Wetlands are the single largest natural CH4 source with median emissions from published studies of 164 Tg yr(-1) , which is about a third of total global emissions. We provide a perspective on important new frontiers in obtaining a better understanding of CH4 dynamics in natural systems, with a focus on wetlands. One of the most exciting recent developments in this field is the attempt to integrate the different methodologies and spatial scales of biogeochemistry, molecular microbiology, and modeling, and thus this is a major focus of this review. Our specific objectives are to provide an up-to-date synthesis of estimates of global CH4 emissions from wetlands and other freshwater aquatic ecosystems, briefly summarize major biogeophysical controls over CH4 emissions from wetlands, suggest new frontiers in CH4 biogeochemistry, examine relationships between methanogen community structure and CH4 dynamics in situ, and to review the current generation of CH4 models. We highlight throughout some of the most pressing issues concerning global change and feedbacks on CH4 emissions from natural ecosystems. Major uncertainties in estimating current and future CH4 emissions from natural ecosystems include the following: (i) A number of important controls over CH4 production, consumption, and transport have not been, or are inadequately, incorporated into existing CH4 biogeochemistry models. (ii) Significant errors in regional and global emission estimates are derived from large spatial-scale extrapolations from highly heterogeneous and often poorly mapped wetland complexes. (iii) The limited number of observations of CH4 fluxes and their associated environmental variables loosely constrains the parameterization of process-based biogeochemistry models. 2012 Blackwell Publishing Ltd. |
[15] | . |
[16] | . Internal pressurization and convective through-flow are demonstrated to be common attributes of wetland plants with cylindrical culms or linear leaves. Eight of 14 species tested produced static internal gas pressure differentials of 200-1,300 Pa relative to ambient and internal convective airflows of 0.2 to > 10 cm3min-1 culm-1, depending on species. Four species produced internal static pressure differentials of < 100 Pa. Two species did not pressurize. The driving forces are gradients in temperature and water vapor between the internal gas spaces of the plants and the ambient atmosphere (thermal transpiration and humidity-induced pressurization). A clear diel variation in pressurization and convective flow was observed; rates were highest in the afternoon and lowest at night, responding to ambient changes in light, temperature, and humidity. The resistance to airflow at the stem-rhizome junction was very high for some species, resulting in a low ability to convert internal pressurization into convective airflow through the rhizomes. Species with a high potential for internal pressurization and a low internal resistance to convective flow seem to have a competitive advantage over species that rely exclusively on diffusive gas transport, which allows them to grow in deeper waters. |
[17] | . |
[18] | . ABSTRACT Two Italian rice ( Oryza sativa var. japonica ) cultivars, Lido and Roma, were tested in the field for methane production, oxidation and emission. In two consecutive years, fields planted with the rice cultivar Lido showed methane emissions 24鈥31% lower than fields planted with the cultivar Roma. This difference was observed irrespective of fertilizer treatment. In contrast to methane emissions, differences in methane production or oxidation were not observed between fields planted with the two cultivars. Plant-mediated transport of methane from the sediment to the atmosphere was the dominating pathway of methane emission. During the entire vegetation period, the contribution of this pathway to total methane emission amounted to c. 90%, whereas the contribution of gas bubble release and of diffusion through the water column to total methane emission was of minor significance. Results obtained from transport studies of tracer gas through the aerenchyma system of rice plants demonstrated that the root鈥搒hoot transition zone is the main site of resistance to plant-mediated gas exchange between the soil and the atmosphere. The cultivar Lido, showing relatively low methane emissions in the field, had a significantly lower gas transport capacity through the aerenchyma system than the cultivar Roma. Thus, the observed differences in methane emissions in the field between the cultivars Lido and Roma can be explained by different gas transport capacities. Apparently, these differences in gas transport capacities are a consequence of differences in morphology of the aerenchyma systems, especially in the root鈥搒hoot transition zone. It is, therefore, concluded that identification and use of high-yielding rice cultivars which have a low gas transport capacity represent an economically feasible, environmentally sound and promising approach to mitigating methane emissons from rice paddy fields. |
[19] | . . 以不同水稻品种为材料,通过实验室手段测定水培稻株甲烷传输速率,研究水稻根系对甲烷传输的影响.结果表明:在水培液中加叠氮化钠对汕优63和冒子头的甲烷传输都有一定的抑制作用,但使2个品种甲烷传输速率明显减小的浓度不一致,汕优63为0.1×10-8,冒子头为0.5×10-8.说明品种间对叠氮化钠耐性存在差异.通过进一步对汕优63水稻根系剪切处理表明:剪根后甲烷传输速率随之改变,留根条数、根重与甲烷传输速率的变化均呈明显的线性正相关;仅切除根尖,甲烷传输速率大幅度下降,降低了50.8%.这些结果说明稻根的主动吸收,对稻体的甲烷传输起十分重要的作用. |
[20] | . . 以不同水稻品种为材料,通过实 验室手段测定各品种水培稻株甲烷传输速率,研究水稻品种间甲烷传输能力的差异及原因;通过控制甲烷排放路径,研究水稻植株状况对甲烷传输的影响。结果表 明:在品种演进过程中,稻株甲烷传输速率逐渐增大(南京1号偏小除外),根氧化力与甲烷传输速率趋势一致。苗期甲烷有50%是经叶片传输的;剪根后甲烷传 输速率随之改变,留根条数、根重与甲烷传输速率的变化均呈明显的线性正相关。植株茎蘖数对甲烷传输有影响,在一定的范围内,植株茎蘖越多,甲烷传输速率越 大。这说明根氧化力是造成品种间甲烷传输速率不同的主要原因。植株生长健壮,叶片多,根系发达,茎蘖多,植株甲烷传输速率大。 |
[21] | . |
[22] | . Within the Florida Everglades methane emission rates were higher in T. domingensis areas (143±19 mg CH 4 m 612 day 611 ) than in C. jamaicense areas (45±15 mg CH 4 m 612 day 611 ). These elevated rates were related to the higher above ground biomass and production in T. domingensis areas relative to C. jamaicense , which suggests that quantitative differences in plant biomass and production rather than qualitative differences between these plant species may control methane emissions. Methane emission was 2.7±1.4% of net daily ecosystem production (NEP) in a T. domingensis area and 14±5.8% and 3.4±4.2% of NEP in two C. jamaicense areas . |
[23] | . |
[24] | . . 甲烷研究已倍受科学界关注,不仅由于其对全球变暖的贡献仅次于二氧化碳,贡献率达25%,还因为单分子甲烷的增值潜势是二氧化碳的15~30倍.湿地是甲烷的重要来源,估计湿地生态系统对全球甲烷排放的贡献率约为20%,即为100~200 Tg a-1.湿地甲烷在厌氧条件下产生,在土壤氧化层以及根际中部分氧化,然后通过土壤、水体和植物体排放到大气中去.就近10 a来湿地甲烷产生、氧化、传输过程及其影响因素等的研究进展进行了综述.产甲烷菌的研究主要集中在其对环境因子的生理生态和分子生物学,尤其集中在稻田产甲烷菌的研究上,因此在深度和广度上都有待突破.产甲烷过程是一个复杂的生化过程,要对甲烷排放量估计、甲烷排放动态研究以及在甲烷排放建模等方面取得进展,必需在甲烷产生机制上进行突破.甲烷氧化菌的研究集中于菌群对环境的适应以及其自身氧化能力上,应用研究还不是很深入.甲烷氧化过程的研究已经有了一定的深度,但氧化过程具体机理的研究还有待进一步深入,这需要分子生物学以及基因组学等多学科的交叉.甲烷传输过程是研究甲烷排放动态的基础,目前相关研究较少,国内有关研究主要集中在稻田研究上,对高寒湿地的研究则几乎是空白.湿地甲烷通量的研究是目前温室气体研究的热点问题,但主要解决的问题是大气中甲烷气体浓度增加与湿地甲烷通量的关系.湿地甲烷通量是由多种因素决定的,对影响湿地甲烷通量的因素的研究相当丰富,特别是近年来对生物因素的关注.由于学科的发展,近10 a在这方面的研究较之过去更为全面和系统.图1表1参68 |
[25] | . . 水生植物能够生长在长期淹水的缺氧底泥中,其根系输氧作用是其生存的关键因素之一.水生维管束植物有一个用于气体交换和输导的通气组织,将氧气输送到地下器官供植物呼吸作用,一部分氧气还通过根系向根区释放,维持根区氧化状态,起到脱毒作用[1,2].水生植物通气量的大小直接关系到植物生长的水深和根系在底泥中的扩展程度[3,4]. |
[26] | . |
[27] | . . |
[28] | . Abstract Wetlands are significant sources of atmospheric methane. Methane produced by microbes enters roots and escapes to the atmosphere through the shoots of emergent wetland plants. Herbivorous birds graze on helophytes, but their effect on methane emission remains unknown. We hypothesized that grazing on shoots of wetland plants can modulate methane emission from wetlands. Diffusive methane emission was monitored inside and outside bird exclosures, using static flux chambers placed over whole vegetation and over single shoots. Both methods showed significantly higher methane release from grazed vegetation. Surface-based diffusive methane emission from grazed plots was up to five times higher compared to exclosures. The absence of an effect on methane-cycling microbial processes indicated that this modulating effect acts on the gas transport by the plants. Modulation of methane emission by animal-plant-microbe interactions deserves further attention considering the increasing bird populations and changes in wetland vegetation as a consequence of changing land use and climate change. |
[29] | . . 运用静态箱法,对内蒙古乌梁素海不同水位的芦苇群落(Phragmites australis )和龙须眼子菜群落(Potamogeton pectinatus)的甲烷排放通量进行2a(2003-2004)野外观测.发现龙须眼子菜群落(沉水植物)甲烷平均排放速率为(3.44±1.60)mg·(m^2·h)^-1,仅为芦苇群落(挺水植物)的平均排放速率的21.94%.高水位芦苇的排放速率要大于低水位芦苇群落.芦苇群落的甲烷排放具有明显的季节性和日变化规律,并且5cm土壤温度和光合有效辐射分别是影响季节性和日变化的主要环境因子.根据观测数据和植物的分布面积,计算得出乌梁素海2003和2004年的甲烷排放量为1024.6t和1156.7t. |
[30] | . . 综合评述了维管植物在自然湿地甲烷产生、氧化、传输和排放过程中的作用.维管植物光合作用的产物是甲烷产生的主要碳源,植物根系的周转和碳物质的分泌为产甲烷细菌提供底物;维管植物根际氧化是甲烷氧化最主要的途径,在植物的生长期占到总氧化量的80%左右.植物传输O2的能力和根际O2的需求是影响根际氧化的主要因素;维管植物通气组织的传输促进了甲烷从土壤向大气的输送,但所采用的传输机制影响着气体的输送效率.此外,自然湿地甲烷排放的各个过程均受到维管植物形态和植被类型的影响.维管植物在甲烷排放中的作用可以部分解释自然湿地甲烷在排放的时间(季节性变化、日变化)和空间尺度上的差异.维管植物对于自然湿地甲烷排放具有指示意义,可以用于大尺度自然湿地甲烷排放量的估算. |
[31] | . . |
[32] | . . 通过湖南双季稻区温室气体排放差异的水稻品种田间试验,研究了不同品种温室气体排放与根系特征的相关性。结果表明,早稻分蘖盛期CH4排放通量与根干重、伤流量均呈显著负相关(P0.05);晚稻CH4排放通量与根伤流量呈极显著负相关(P0.01);早稻N2O排放通量在分蘖盛期与根伤流量相关性极显著(P0.01),在齐穗期与根体积、干重、根伤流量均呈极显著负相关(P0.01);晚稻分蘖盛期,根系干重与体积均与N2O排放通量呈显著正相关(P0.05);齐穗期,根系体积与N2O排放通量呈显著负相关(P0.05);早稻分蘖盛期CO2排放通量与根系干重和根伤流量呈显著正相关关系(P0.05),晚稻齐穗期根系伤流量与CO2排放通量负相关性达到极显著水平(P0.01)。因此,根系特性是影响水稻温室气体排放的重要因素。 |
[33] | . The transport of methane from the rhizosphere to the atmosphere takes place in the intercellular spaces and stomata of wetland plants, and foliar gas exchange is one of the critical steps of the transport process. The objectives of our research were to investigate: (i) variation in foliar gas exchange among four common wetland plant species (i.e., Peltandra virginica L., Orontium aquaticum L., Juncus effusus L., and Taxodium distichum L.), (ii) the role of key environmental factors (i.e., light, temperature, and carbon dioxide concentration) in controlling foliar methane emission, and (iii) physiological mechanisms underlying the variation in methane emission due to species and the environment. Experiments were conducted in an instantaneous, flow-through gas-exchange system that operated on a mass balance approach and concurrently measured foliar fluxes of methane, water vapor, and carbon dioxide. The chamber system allowed for the control of light, temperature, humidity, and carbon dioxide concentration. Diel patterns of methane emission varied among species, with daylight emissions from P. virginica and O. aquaticum 2–4 times those of J. effusus and T. distichum in saturating light. Foliar methane emission from P. virginica (1.8002μmol02m 612 02s 611 ) under ambient daylight conditions was an order of magnitude higher than that of the other three species (650.2002μmol02m 612 02s 611 ). As leaf temperature was increased by 1002°C, methane emission increased by a factor of 1.5–2.2, and the temperature effect was independent of stomatal conductance. When data were pooled among the four species, varying the light and carbon dioxide concentrations in a stepwise manner produced changes in foliar methane emission that were associated with stomatal conductance ( r 2 02=020.52). To scale our observations to other wetland plant species, a stepwise multiple regression model is offered that incorporates stomatal conductance and net carbon dioxide assimilation to estimate instantaneous methane emission from foliar surfaces. The model indicates that changes in stomatal conductance affect methane emission three times more than equivalent changes in net carbon dioxide assimilation. |
[34] | . |
[35] | . Rice plants ( Oryza sativa L.) are mainly cultivated in flooded paddy fields and are thus dependent on oxygen transport through the plant to maintain aerobic root metabolism. This gas transport is effectuated through the aerenchyma of roots and shoots. However, the efficiency of gas transport through the root–shoot transition zone is disputed and there are indications that the root–shoot transition zone may represent one of the largest resistances for gas transport. Therefore, we present gas conductance measurements of the root–shoot transition of individual rice tillers measured using SF 6 . SF 6 was detected with a highly advanced laser based photoacoustic detection scheme allowing sensitive, high resolution measurements. In conjunction with these measurements, various plant morphological parameters were quantified. These measurements indeed indicate that the conductance at the root–shoot transition may be much smaller than the conductance of root and shoot aerenchyma within the rice plant. Conductance was strongly correlated to tiller transverse area. After elimination of tiller area from the conductance equation, the resulting permeance coefficient was still correlated to tiller area, but negatively and related to the process of radial tiller expansion. In addition, a decrease in the permeance coefficient was also observed for increasing distance from the plant centre. No correlation was found with tiller type or age of the mother tiller. Incorporation of estimates of the conductance of the root–shoot transition zone coupled to plant morphological parameters will allow considerable improvement of understanding and models on gas transport through plants. |
[36] | . Abstract Species of wetland plants that aerate their submerged organs by thermo-osmotic transport of gas have been identified in six genera of the angiosperm families Butomacea, Nymphaeacea and Menyanthaceae. Flow rates of 14 ml gas h 1 in the monocotyledon Hydrocleys nymphoides (Humb. & Bonpl. ex Willd.) Buchenau to 5000 ml in the dicotyledon Victoria amazonica (Poepp.) Sowerby are comparable to those rates found in Nelumbo nucifera Gaertn. from the Nelumbonacean family, but an order higher than those in Alnus glutinosa (L.) Gaertn. from the Betulacean family as reported in the literature. The aeration is driven by a pressurizaion of the gas in th e aerenchyma of young floating leaves as a consequence of the physical effect of thermo-osmosis of gases. Differences in hygrometric state of the leaves and the ambient air effect the flow rates. Increased transpirational cooling of the upper leaf surface seems to give rise to a steeper temperature gradient within the leaf, intensifying the aeration. As these five plant families are not closely related, the ability to generate a pressurized ventilation by thermo-osmosis of gas can be seen as a special adaptation of the wetland plants to the anoxic environment. |
[37] | . Abstract Constructed wetlands (CWs) have been widely used for treating wastewater. CWs also are the sources of greenhouse gas (GHG) due to high pollutant load. It has been reported that plant species diversity can enhance nitrogen (N) removal efficiency in CWs for treating wastewater. However, the influence of plant species diversity on GHG emissions from CWs in habitats with high N levels still lack research. This study established four species richness levels (1, 2, 3, 4) and 15 species compositions by using 75 simulated vertical flow CWs microcosms to investigate the effects of plant species diversity on the GHG emissions and N removal efficiency of CWs with a high N level. Results showed plant species richness reduced nitrous oxide (N 2 O) emission and N (NO 3 - -N, NH 4 + -N, and TIN) concentrations in wastewater, but had no effect on methane (CH 4 ) emission. Especially, among the 15 compositions of plant species, the four-species mixture emitted the lowest N 2 O and had under-depletion of N (D min TIN0002<00020). The presence of Oenanthe javanica had a significantly negative effect on the N 2 O emission but had no effect on N removal efficiency. The presence of Rumex japonicus significantly reduced the N (NO 3 - -N and TIN) concentrations in wastewater but had no effect on the N 2 O and CH 4 emissions. The N concentrations and GHG emissions in the community of R. japonicus 0103 Phalaris arundinacea were as low as those in the four-species mixture. Assembling plant communities with relatively high species richness (four-species mixture) or particular composition (R. japonicus 0103 P. arundinacea) could enhance the N removal efficiency and reduce the GHG emissions from CWs for treating wastewater with a high N level. |
[38] | . Factors responsible for the alteration of $CH_4$ isotopic composition during gas transport by emergent macrophytes were investigated in Pontederia cordata (Pickerlweed) and Sagittaria lancifolia (Bull tongue). Measured rates of $CH_4$ emission from petioles and leaves indicated that the locus of gas release from these plants is the petiole and not the leaf. Methane concentration profiles of gases within petioles further indicated that most $CH_4$ is emitted from the lower portion of the petiole near the waterline. Ethane and propane tracer experiments confirmed mass-dependent fractionation during gas transport through these plants. After injection with a gas mixture, both plant types emitted ethane 12% faster than propane. Twenty-five minutes or more after injection, ethane was found to be depleted in gas within petioles relative to the injected gas. The degree of fractionation observed for the $^13CH_4/^12CH_4$ and ethane/propane pairs was similar to values predicted by kinetic gas theory. |
[39] | . Aerenchymatous plants can transport methane (CH4) from the root zone to the atmosphere, bypassing the surface-oxidizing layers of the soil, yet morphological and anatomical factors that govern the transport of methane have rarely been critically tested in manipulative experiments.Here, we investigated the methane transport capacity of hydroponically grown Juncus effusus, in experiments with roots submerged in nutrient solutions sparged with methane (1.16 mmol CH4 l 1). Through a range of manipulations of the above- and below-ground plant parts, we tested the contradictory claims in the literature regarding which sites provide the greatest resistance to gas transport.Root manipulations had the greatest effect on methane transport. Removing root material reduced methane transport significantly, and especially the lateral roots and the root tips were important. Cutting of the shoots, with or without subsequent sealing, did not alter methane transport significantly.We confirm modelling predictions that the limiting factor for methane transport in the tussock forming wetland graminoid, J. effusus, is the amount of permeable root surface, estimated using the proxy measurement of root length. The aerial tissues do not provide any significant resistance to methane transport, and the methane is emitted from the lower 50 mm of the shoots. |
[40] | . Plants have been suggested to have significant effects on methane (CH4) and nitrous oxide (N2O) fluxes from littoral wetlands, but it remains unclear in subtropical lakes. We conducted in situ measure |
[41] | . . 甲烷是重要的温室气体之一,对全球变暖的贡献率是20%.大气中甲烷浓度的迅速增加与甲烷的源和汇的不平衡有关.土壤中存在甲烷氧化菌,在一定条件下可以氧化大气中的甲烷.不过土壤对甲烷的氧化能力受一些农业生产活动的影响.氮肥的施用对土壤甲烷氧化有一定的影响.长期定位施肥实验的结果表明氮肥对土壤甲烷氧化的影响主要来源于铵态氮而不是硝态氮,因为氨对甲烷氧化有着竞争性抑制作用.此外,长期施用氮肥还改变了土壤微生物的区系及其活性,从而降低甲烷的氧化速率.氮肥施用的短期效应则与土壤的耕作管理历史、土壤性质、氮肥施用量等因素有关.有机肥对土壤甲烷氧化的影响比化肥要低得多,其物理化学性质及施用方法的不同都会影响到土壤对甲烷的氧化. |
[42] | . . |
[43] | . . 于各测定月的小潮日,使用悬管装置采集气样并结合气相色谱测定,对闽江河口互花米草(Spartina alterniflora)沼泽湿地互花米草植株的甲烷传输量及其主要释放部位进行了研究,另又采用静态箱法-气相色谱法测定了互花米草沼泽湿地甲烷的排放通量,以此分析互花米草植物体甲烷传输对互花米草沼泽湿地甲烷排放通量的贡献率,最后测定了互花米草植株髓腔内的甲烷浓度。结果表明,不同生长阶段的互花米草植株甲烷传输量明显不同,快速生长阶段的互花米草植株甲烷传输量最高;互花米草植株甲烷传输对互花米草沼泽湿地甲烷排放通量的贡献率介于9%-94%之间;互花米草植物体传输甲烷的主要释放部位是距地面0-20cm处,该部位对植物甲烷传输量的贡献率为50%(平均值);髓腔内的甲烷浓度远高于大气中的甲烷浓度,且自下而上呈递减趋势。 |
[44] | . Abstract Measurements focused on seasonal contribution of rice productivity to methane emission were made in three experiments conducted in Texas flooded paddy soils during 1994 and 1995 growing seasons. A total of five rice cultivars representing two distinct groups in methane emission were involved. Over a 10-week period after permanent flooding, total seasonal methane emission was positively correlated with rice above-ground biomass ( r 2 = 0.845, n = 11). A very strong dependence of daily methane emission on above-ground vegetative biomass ( r 2 = 0.887, n = 93) and on root biomass ( r 2 = 0.816, n = 33) was also observed. Calculation from three developmental periods (vegetative, reproductive and ripening) of rice plant indicated that more than 75% of total seasonal methane was emitted during the last 5-week period in concert with reproductive and ripening stages, while rice biomass production during the same period amounted to 鈮 50% of the seasonal total. According to the correlation of cumulative methane emission with above-ground biomass increment between every two-week interval ( r 2 = 0.490, n = 93, P = 0.000), the carbon released as methane is approximately equivalent to 3% and 4.5% of photosynthetically fixed carbon in the biomass for low and high emission cultivars, respectively. A further investigation showed that these fractions are related to plant growth and development. The carbon ratio of methane emitted to net photosynthetic production during vegetative, reproductive, and ripening periods averaged 0.9%, 3.6% and 7.9%, respectively, for low emission cultivars, and 2.0%, 5.0% and 8.3%, respectively, for high emission cultivars. Moreover, the ratio was strongly dependent on plant biomass, resulting in r 2 values from 0.775 to 0.907. |
[45] | |
[46] | . |
[47] | . . 稻田CH4排放是稻田土壤中CH4产生、氧化和传输不同过程的净效应 .水稻植株强烈影响稻田CH4的产生、氧化和传输过程 ,是导致稻田CH4排放季节性变化规律的一个重要因素 .本文综述了水稻植株对稻田CH4排放过程的不同影响 .水稻植株根系分泌物和脱落物作为产甲烷前体促进稻田土壤中CH4的产生 ,在水稻生长后期 ,植株根系分泌物和脱落物被认为是稻田土壤甲烷产生的主要基质 ,是导致这一时期稻田CH4高排放通量的主要原因 ;水稻植株根系泌氧在根际环境形成一个微氧区域氧化稻田甲烷 ,整个水稻生长季稻田土壤中产生的CH4大约 36 %~ 90 %在植株根际环境中被氧化 ;约 80 %甚至更多的稻田CH4通 |
[48] | . |
[49] | . |
[50] | The purpose of this study was to estimate theresistance to methane release of the above-groundportion of Carex, a wetland sedge, and todetermine the locus of methane release from the plant. Measurements conducted on plants clipped to differentheights above the water level revealed that themethane flux from clipped plants was on the order of97% to 111% of control (unclipped) values. Thegreatest increase was observed in the initial fluxmeasurement after the plants had been clipped to aheight of 10 cm. Subsequent measurements on the 10 cmhigh stubble were similar to control values. When theends of plants which had been clipped to 10 cm weresealed, the methane flux was reduced to 65% ofcontrol values. However, sealing had no effect on theflux from plants which were clipped at 15 cm andhigher, indicating that virtually all methane wasreleased on the lower 15 cm of the plants as theyemerged from the water. The results indicate that theabove-ground portions of Carex at our studysite offered only slight resistance to the passage ofmethane, and that the main sites limiting methaneemission are below-ground, at either theporewater-root or root-shoot boundary. We hypothesizethat the transitory increase in flux associated withclipping was due to the episodic release of methaneheld within the plant lacunae. The buildup ofCH 4 partial pressure within lacunal spacesovercomes the resistance to gas transport offered byaboveground parts. |
[51] | . |
[52] | . We conducted a 14C pulse-labeling experiment under field conditions to estimate the contribution of recent photosynthates to methane (CH 4) emission in arctic wet sedge tundra dominated by Carex aquatilis and Eriophorum angustifolium. The average CH 4 emission rate from plant–soil mesocosms in this study was 0.45 g C m 612 d 611. Carbon assimilated by plants via photosynthesis during pulse-labeling turned over rapidly and appeared as emitted CH 4 within 24 h. Integration of flux measurements made over a 2-week period shows that the contribution of recent photosynthates to mid-season CH 4 emission is relatively low. Less than 1% of the 14C-labeled carbon dioxide taken up through photosynthesis was emitted as 14CH 4 during this study. |
[53] | . |
[54] | . ABSTRACT Closed-flow chambers are commonly used to measure CH 4 emissions from aquatic plants. We monitored environmental and plant physiological responses to closed chambers during measurements of CH 4 emission from Typha latifolia (cattails) to evaluate potential errors in emission estimates. In 1990, two wetlands were studied: one in NE Kansas and one in central New York. Leaves and stems of Typha enclosed in gas-impermeable, collapsible bags were exposed to rapid increases in chamber air temperature and, thus, tissue temperatures (up to 9°C above ambient) as well as rapid reductions in both CO 2 partial pressure due to photosynthesis and the leaf-to-air water vapor pressure deficit due to transpiration. These rapid (<5min) environmental changes within chambers are known to affect stomatal aperture through which CH 4 exits Typha leaves. Indeed, stomatal conductance ( g ) increased up to 42% compared to control leaves after 19min of enclosure in chambers, and chamber effects on interactions between irradiance and g were detected. The duration of enclosure also critically affected the CH 4 gradient within chambers. Estimated CH 4 emission from leaves in chambers sampled after 19min (3nmol m 612 s 611 ) was 10-fold lower than estimates based on 30-s sampling periods (30nmol m 612 s 611 ) due to reduction in the leaf-to-air concentration gradient for CH 4 . Since closed chambers (1) induce alterations in g, (2) may result in rapid environmental changes, and (3) lead to almost instantaneous reductions in the leaf-to-air concentration gradient for CH 4 diffusion, we suggest that only very short enclosure times should be used when measuring CH 4 emission from plants. |
[55] | . Abstract BACKGROUND: According to the Intergovernmental Panel on Climate Change (IPCC) 2007, natural wetlands contribute 20-39 % to the global emission of methane. The range in the estimated percentage of the contribution of these systems to the total release of this greenhouse gas is large due to differences in the nature of the emitting vegetation including the soil microbiota that interfere with the production and consumption of methane. SCOPE: Methane is a dominant end-product of anaerobic mineralization processes. When all electron acceptors except carbon dioxide are used by the microbial community, methanogenesis is the ultimate pathway to mineralize organic carbon compounds. Emergent wetland plants play an important role in the emission of methane to the atmosphere. They produce the carbon necessary for the production of methane, but also facilitate the release of methane by the possession of a system of interconnected internal gas lacunas. Aquatic macrophytes are commonly adapted to oxygen-limited conditions as they prevail in flooded or waterlogged soils. By this system, oxygen is transported to the underground parts of the plants. Part of the oxygen transported downwards is released in the root zone, where it sustains a number of beneficial oxidation processes. Through the pores from which oxygen escapes from the plant into the root zone, methane can enter the plant aerenchyma system and subsequently be emitted into the atmosphere. Part of the oxygen released into the root zone can be used to oxidize methane before it enters the atmosphere. However, the oxygen can also be used to regenerate alternative electron acceptors. The continuous supply of alternative electron acceptors will diminish the role of methanogenesis in the anaerobic mineralization processes in the root zone and therefore repress the production and emission of methane. The role of alternative element cycles in the inhibition of methanogenesis is discussed. CONCLUSIONS: The role of the nitrogen cycle in repression of methane production is probably low. In contrast to wetlands particularly created for the purification of nitrogen-rich waste waters, concentrations of inorganic nitrogen compounds are low in the root zones in the growing season due to the nitrogen-consuming behaviour of the plant. Therefore, nitrate hardly competes with other electron acceptors for reduced organic compounds, and repression of methane oxidation by the presence of higher levels of ammonium will not be the case. The role of the iron cycle is likely to be important with respect to the repression of methane production and oxidation. Iron-reducing and iron-oxidizing bacteria are ubiquitous in the rhizosphere of wetland plants. The cycling of iron will be largely dependent on the size of the oxygen release in the root zone, which is likely to be different between different wetland plant species. The role of the sulfur cycle in repression of methane production is important in marine, sulfate-rich ecosystems, but might also play a role in freshwater systems where sufficient sulfate is available. Sulfate-reducing bacteria are omnipresent in freshwater ecosystems, but do not always react immediately to the supply of fresh sulfate. Hence, their role in the repression of methanogenesis is still to be proven in freshwater marshes. |
[56] | . |
[57] | . |
[58] | . A field experiment was conducted to determine the effect local cultivars of L. () had on evolution from flooded plots. Semidwarf and tall varieties were drill-seeded into a Crowley silt loam (Typic Albaqualf) and fluxes were measured twice a week over the first and ratoon cropping seasons. Semidwarf varieties evolved significantly less over both growing seasons. Over the main cropping season, semidwarfs emitted an average 185 kg CH4 ha-1 to the atmosphere compared with 300 kg CH4 ha-1 for the tall varieties. During the ratoon sampling season, semidwarf and tall varieties evolved 540 and 830 kg CH4 ha-1, respectively. Compared with tall cultivars, semidwarfs evolved 36% less over both seasons. |
[59] | . . |
[60] | . . 植物传输甲烷是湿地甲烷排放的主要路径之一,深入了解植物传输甲烷是认识、应对与调控湿地甲烷排放的重要 环节。运用静态箱/自我设计的悬管+气相色谱对闽江河口芦苇湿地甲烷排放通量、单株植物传输甲烷速率以及植株不同部位释放甲烷能力进行了和日进程观测,结 果表明:春和夏季甲烷排放高于秋和冬季,白天高于夜间;单株植物传输甲烷速率动态变化规律不明显;芦苇植株下部是传输过程中释放甲烷的主要部位。潮水水淹 降低了甲烷排放通量和单株传输甲烷速率;遮光抑制植株传输甲烷的能力;芦苇髓腔内甲烷气体浓度自下而上递减,浓度白天低夜间高,温度和湿度均是其浓度昼夜 变化的影响因素。 |
[61] | . . 以长江口微咸水河口湿地为研究对象,通过模拟潮汐淹没和改进的静态箱法,针对芦苇在不同淹水条件下对甲烷的传输作用进行了研究。结果表明,长期淹没样点割除芦苇前后平均甲烷释放分别为1.60 mgCH4 m-2h-1和0.50 mgCH4 m-2h-1,而短期淹没样点割除芦苇前后平均甲烷释放分别为0.94 mgCH4 m-2h-1和0.55 mgCH4 m-2h-1。芦苇的存在增加了大约41.5%—69%的甲烷释放,割除芦苇导致长期淹没样点甲烷释放显著地减少。从淹水前到淹水后的一个潮周期内,长期淹没样点和短期淹没样点的甲烷释放分别在淹没期达到最小和最大释放(1.21 mgCH4 m-2h-1和1.18 mgCH4 m-2h-1),说明芦苇对甲烷的传输作用受到潮汐淹没程度的影响。实验室针对芦苇自身产甲烷的可能性进行的离体芦苇茎干和叶片的甲烷释放研究发现,离体茎干释放大约0.7μL/L和0.2μL/L甲烷,且下部茎干的甲烷释放量要明显高于上部茎干和叶片,随后均不再增加。结果进一步证实芦苇增加的甲烷排放来自对土壤气体的传输。同时,传输作用主要发生在植株的下部茎干。 |
[62] | . Methane emission by soils results from antagonistic but correlated microbial activities. Methane is produced in the anaerobic zones of submerged soils by methanogens and is oxidised into CO 2 by methanotrophs in the aerobic zones of wetland soils and in upland soils. Methanogens and methanotrophs are ubiquitous in soils where they remain viable under unfavourable conditions. Methane transfer from the soil to the atmosphere occurs mostly through the aerenchyma of aquatic plants, but also by diffusion and as bubbles escaping from wetland soils. Methane sources are mainly wetlands. However 60 to more than 9002% of CH 4 produced in the anaerobic zones of wetlands is reoxidised in their aerobic zones (rhizosphere and oxidised soil-water interface). Methane consumption occurs in most soils and exhibits a broad range of values. Highest consumption rates or potentials are observed in soils where methanogenesis is or has been effective and where CH 4 concentration is or has been much higher than in the atmosphere (ricefields, swamps, landfills, etc.). Aerobic soils consume atmospheric CH 4 but their activities are very low and the micro-organisms involved are largely unknown. Methane emissions by cultivated or natural wetlands are expressed in mg CH 4·m –2·h –1 with a median lower than 1002mg CH 4·m –2·h –1. Methanotrophy in wetlands is most often expressed with the same unit. Methane oxidation by aerobic upland soils is rarely higher than 0.102mg CH 4·m –2·h –1. Forest soils are the most active, followed by grasslands and cultivated soils. Factors that favour CH 4 emission from cultivated wetlands are mostly submersion and organic matter addition. Intermittent drainage and utilisation of the sulphate forms of N-fertilisers reduce CH 4 emission. Methane oxidation potential of upland soils is reduced by cultivation, especially by ammonium N-fertiliser application. |
[63] | . In a greenhouse study, the effect of moisture regimes (continuously flooded, continuously nonflooded, alternately flooded) on methane efflux from an alluvial soil planted to rice was studied using the closed chamber method. Methane efflux was almost 10 times more pronounced under continuously flooded conditions than under continuously nonflooded conditions. Intermittently flooded regimes (alternately flooded and nonflooded cycles of 40 or 20 days each) emitted distinctly less methane than the continuously flooded system. A significant negative correlation was found between methane emission under different water regimes and rhizosphere redox potential. Extractable Fe 2+ , readily mineralizable carbon (RMC) and root biomass presented a significant positive correlation with cumulative methane emission. The correlation of methane emission with other plant parameters and microbial biomass was not significant. Our results further suggest the possibility of reduced methane emissions through appropriate water management in a rainfed rice ecosystem. |
[64] | Summary Plant species richness may affect greenhouse gas emissions because it may change ecosystem productivity. Emergent plants are an important component of wetland ecosystems, but little is known about how emergent plant richness affects greenhouse gas emissions. We assembled experimental communities consisting of 1, 2, 4 or 8 emergent plant species in aquatic microcosms and measured community biomass and emissions of CO2 and CH4. Emergent plant richness had little effect on cumulative emissions of CO2 or CH4, most likely because it did not affect community biomass. This conclusion is supported by the fact that CO2 emission was significantly positively related to community biomass. The reason for the unresponsiveness of community biomass to species richness of emergent plants may be that all the plants are in the same functional group so that complementarity among species is weak. CH4 emissions from monocultures of different species differed greatly. Our results do not support the hypothesis that richness of emergent plants plays an important role in regulating greenhouse gas emissions from wetlands. A significant richness effect on greenhouse gas emissions might be detected in wetlands consisting of different plant functional groups such as emergent, floating and submerged plants. |
[65] | . Abstract Earth's climate is warming as a result of anthropogenic emissions of greenhouse gases, particularly carbon dioxide (CO(2)) from fossil fuel combustion. Anthropogenic emissions of non-CO(2) greenhouse gases, such as methane, nitrous oxide and ozone-depleting substances (largely from sources other than fossil fuels), also contribute significantly to warming. Some non-CO(2) greenhouse gases have much shorter lifetimes than CO(2), so reducing their emissions offers an additional opportunity to lessen future climate change. Although it is clear that sustainably reducing the warming influence of greenhouse gases will be possible only with substantial cuts in emissions of CO(2), reducing non-CO(2) greenhouse gas emissions would be a relatively quick way of contributing to this goal. |
[66] | . ABSTRACT Experiments were conducted in -dominated communities of Arctic and subarctic Alaska to determine the importance of plant stomatal behavior in modifying methane release from wetland plants. Daily methane flux was positively correlated (r = 0.95) with daily leaf conductance at three lake margin sites over an 8-day period. Furthermore, methane flux was reduced significantly following stomatal closure induced by application of an antitranspirant to the plant surface and by enclosure in an opaque chamber. These are the first data to show evidence of stomatal control of release of methane from plants. Significant methane flux continued, however, even with stomatal closure, suggesting that cuticular conductance remains an important mechanism of methane release. The importance of stomatal pathway, relative to the cuticular pathway, is expected to vary through the growing season. These results have significant implications both in the design of chamber-based measurement studies and in interpreting regional to global methane emissions from wetlands. |
[67] | . Recent progress in improving the salt tolerance of cultivated plants has been slow. Physiologists have been unable to define single genes or even specific metabolic processes that molecular biologists could target, or pinpoint the part of the plant in which such genes for salt tolerance might be expressed. While the physiological might be expressed. While the physiological processes are undoubtedly complex, faster progress on unraveling mechanisms of salt tolerance might be made if there were more effort to test hypotheses rather than to accumulate data, and to integrate cellular and whole plant responses. This article argues that salts taken up by the plant do not directly control plant growth by affecting turgor, photosynthesis or the activity of any one enzyme. Rather, the build-up of salt in old leaves hasten their death, and the loss of these leaves affects the supply of assimilates or hormones to the growing regions and thereby affects growth. |
[68] | . Emission of CH 4 from ricefields is the result of anoxic bacterial methane production. Global estimates of annual CH 4 emission from ricefields is 100 Tg. CH 4 emission data from limited sites are tentative. It is essential that uncertainty in individual sources is reduced in order to develop feasible and effective mitigation options which do not negate gains in rice production and productivity. Field studies at the International Rice Research Institute show that soil and added organic matter are the sources for initial methane production. Addition of rice straw enhances methane production. Roots and root exudates of wetland rice plants appear to be the major carbon sources at ripening stage. The production and transport of CH 4 to the atmosphere depend on properties of the rice plant. Under the same spacing and fertilization, the traditional variety Dular emitted more CH 4 per day than did the new plant type IR65597. Upon flooding for land preparation anaerobic conditions result in significant amount of methane being formed. Drying the field at midtillering significantly reduced total CH 4 emissions. Large amounts of entrapped CH 4 escape to the atmosphere when floodwater recedes upon drying at harvest. Cultural practices may account for 20% of the overall seasonal CH 4 emissions. |
[69] | . To attempt to develop physicochemical and physiological modelling for methane transport from the rhizosphere to the atmosphere through rice plants, methane flux, methane concentration in the soil water, and the biomass of rice were measured in lysimeter rice paddies (2.5 脳 4 m, depth 2.0 m) once per week throughout the entire growing season in 1992 at Tsukuba, Japan. The addition of exogenous organic matter (rice straw) or soil amendments with the presence or absence of vegetation were also examined for their influence on methane emissions. The total methane emission over the growing season varied from 3.2 g CH 4 m -2 y -1 without the addition of rice straw to 49.7 g CH 4 m -2 y -1 with rice straw and microbiological amendment. In the unvegetated plot with the addition of rice straw, there was much ebullition of gas bubbles, particularly in the summer. The annual methane emission due to the ebullition of gas bubbles,from the unvegetated plot with the addition of rice straw was estimated to be almost the same as that from the vegetated site with the addition of rice straw. In the early growth stage, the methane flux can be analyzed by the diffusion model (Flux=Methane concentration 脳 Conductance of rice body) using parameters for methane concentration in the soil water as a difference in concentration between the atmosphere and the rhizosphere, and for the biomass of rice as a conductance of rice body. On the other hand, although the diffusion model was inapplicable to a large extent from the middle to late growth stage, methane flux could be estimated by air temperature and concentration in the soil water. Thus, methane transport from the rhizosphere to the atmosphere through rice plants consisted of two phases: one was an explainable small part by diffusion in rice body; the other was a large part strongly, governed by air temperature. The existence of gas bubbles in the soil may be related to the transition between the two phases |
[70] | |
[71] | . Abstract To clarify the mechanisms of methane transport from the rhizosphere into the atmosphere through rice plants (Oryza sativa L.), the methane emission rate was measured from a shoot whose roots had been kept in a culture solution with a high methane concentration or exposed to methane gas in the gas phase by using a cylindrical chamber. No clear correlation was observed between change in the transpiration rate and that in the methane emission rate. Methane was mostly released from the culm, which is an aggregation of leaf sheaths, but not from the leaf blade. Micropores which are different from stomata were newly found at the abaxial epidermis of the leaf sheath by scanning electron microscopy. The measured methane emission rate was much higher than the calculated methane emission rate that would result from transpiration and the methane concentration in the culture solution. Rice roots could absorb methane gas in the gas phase without water uptake. These results suggest that methane dissolved in the soil water surrounding the roots diffuses into the cell-wall water of the root cells, gasifies in the root cortex, and then is mostly released through the micropores in the leaf sheaths. |
[72] | . Wetlands are the largest natural methane (CH4) source and the vegetated littoral areas are the major contributors for CH4release from sediment to the atmosphere. Although the effects of herbivores on biomass removal, growth and reproduction of emergent macrophytes have been well documented, their effect on plant-mediated CH4fluxes, especially by insects, remains unknown. We performed a mesocosm experiment in which we simulated the damage caused by herbivorous insects and manipulated the density of damaged culms ofEleocharis equisetoides(4 levels 鈥 0, 20, 50 and 100%) measuring the corresponding CH4emission, concentration and potential production in the sediment. We hypothesized that an increased percentage of culms with simulated herbivory would be associated with increased CH4fluxes from sediment toward the atmosphere. Simulated herbivory positively affected CH4emissions, but only under high herbivory pressure. The average CH4flux from mesocosms with 50% and 100% damaged culms was 3.5 higher than those with intact or low levels of damage. These results indicate that physical damage on macrophytes affects gas transport within the plants. A field survey in our studied system revealed that plant biomass consumed by herbivores is relatively low. This result highlight that insects may have a disproportional effect on CH4emissions, i.e., a very small damage (low biomass removal), when performed in many culms (50% and 100% of damaged culms treatments), may substantially increase CH4fluxes. In summary, our findings bring a new perspective to the influence of herbivory on CH4and carbon cycling, especially regarding the role insects might play. |
[73] | . Abstract Gas transfer processes are fundamental to the biogeochemical and water quality functions of wetlands, yet there is limited knowledge of the rates and pathways of soil-atmosphere exchange for gases other than oxygen and methane (CH4). In this study, we use a novel push-pull technique with sulfur hexafluoride (SF6) and helium (He) as dissolved gas tracers to quantify the kinetics of root-mediated gas transfer, which is a critical efflux pathway for gases from wetland soils. This tracer approach disentangles the effects of physical transport from simultaneous reaction in saturated, vegetated wetland soils. We measured significant seasonal variation in first-order gas exchange rate constants, with smaller spatial variations between different soil depths and vegetation zones in a New Jersey tidal marsh. Gas transfer rates for most biogeochemical trace gases are expected to be bracketed by the rate constants for SF6 and He, which ranged from 6510612 to 2 × 10611 h611 at our site. A modified Damk02hler number analysis is used to evaluate the balance between biochemical reaction and root-driven gas exchange in governing the fate of environmental trace gases in rooted, anaerobic soils. This approach confirmed the importance of plant gas transport for CH4, and showed that root-driven transport may affect nitrous oxide (N2O) balances in settings where N2O reduction rates are slow. |
[74] | . |
[75] | . ABSTRACT The influence of stem lacunar structure on the potential of diffusion and mass flow to meet estimated root O 2 demands was evaluated and compared in four submersed aquatic plant species. Internodal lacunae formed large continuous gas canals which were constricted at the nodes by thin, perforated diaphragms. Gas transport studies showed that nodes had little effect on diffusion, but significantly reduced mass flow. Measured diffusive resistances approximated those predicted by Fick's first law, ranged from 203 to 5107 × 10 8 s m 614 and increased as lacunar area decreased in Potamogeton praelongus , two Myriophyllum species and Elodea canadensis. Our analysis suggested that diffusion could satisfy estimated root O 2 demands given the development of relatively steep O 2 gradients (0.15–0.35 mol O 2 mor 611 per 0.5 m stem) between shoots and roots. Plants with high resistances (e.g. > 750 × 10 8 s m 614 ) and long lacunar pathlengths may be unable, even during active photosynthesis, to support the O 2 demands of a large root system by diffusion alone. Measured nodal resistances to mass flow approximated those predicted by Hagen-Poiseuille law and ranged from 46 to 2029 × 10 8 Pa s m 613 . Our analysis suggested that these resistances were quite low and that relatively small pressure differentials (< 150 Pa per 0.5 m stem) could drive mass flow at rates which would support root O 2 demands. Possible mechanisms whereby plant architecture may serve to maintain these pressure differentials are proposed. |
[76] | . CH4 emission rates have been measured in an Italian rice paddy between 1984 and 1986, covering three vegetation periods. For these measurements a fully automated, computerized sampling and analyzing system was developed which allowed the simultaneous determination of CH4 emission rates at 16 different field plots. CH4 emission rates showed strong diurnal and seasonal variations. Diurnal changes correlated with changes in soil temperature. During the season, CH4 emission rates showed a first maximum in May090009June before tillering and a second maximum in July during the reproductive stage of the rice plants. In 1985 and 1986 two maxima were observed during summer in addition to the first maximum in the rate of CH4 emission during spring. Application of mineral and/or organic fertilizer strongly influenced the CH4 emission rates, depending on the type, rate, and mode of fertilizer application. Thus the rates decreased by at most 40% and 60% after fertilization by deep incorporation with 200 kg N/ha urea and 200 kg N/ha ammonium sulfate, respectively. Application of 200 kg N/ha calcium cyanamide led to a reduction of the first maximum of CH4 emission but caused the second maximum to increase, the overall result being that the seasonally averaged CH4 emission rate was comparable to that observed in unfertilized fields. Application of rice straw at a rate of 12 t/ha enhanced the rate of CH4 emission by a factor of 2 compared with the control. Higher application rates of rice straw did not cause a further increase in CH4 emission. The complete records of CH4 emissions over three vegetation periods indicate an average seasonal CH4 emission rate from unfertilized fields of 0.28 g CH4/m2 d, with a range of 0.160900090.38 g CH4/m2 d. Based on this value and applying the observed temperature dependence of the CH4 emission rates, the global annual CH4 emission from rice paddies is estimated to be in the range of 50090009150 Tg, with a likely average of 100 Tg. This figure represents between 19% and 25% of the global CH4 emission, indicating that rice paddies are one of the most important individual sources of atmospheric CH4. |
[77] | . The seasonal change of the rates of production and emission of methane were determined under in-situ conditions in an Italian rice paddy in 1985 and 1986. The contribution to total emission of CH 4 of plant-mediated transport, ebullition, and diffusion through the flooding water was quantified by cutting the plants and by trapping emerging gas bubbles with funnels. Both production and emission of CH 4 increased during the season and reached a maximum in August. However, the numbers of methanogenic bacteria did not change. As the rice plants grew and the contribution of plant-mediated CH 4 emission increased, the percentage of the produced CH 4 which was reoxidized and thus, was not emitted, also increased. At its maximum, about 300 ml CH 4 were produced per m 2 per hour. However, only about 6% were emitted and this was by about 96% via plant-mediated transport. Radiotracer experiments showed that CH, was produced from H 2 /CO 2 . (30–50%) and from acetate. The pool concentration of acetate was in the range of 6–10 mM. The turnover time of acetate was 12–16 h. Part of the acetate pool appeared to be not available for production of CH 4 or CO 2 |
[78] | . ABSTRACT The movement of methane (CH4) from anaerobic sediments through the leaves, stems, and flowers of aquatic plants and into the atmosphere was found to provide a significant pathway for the emission of CH4 from the aquatic substrates of flooded wetlands. Methane concentrations well above the surrounding ambient air levels were found in the mesophyll of 16 varies of aquatic plants and are attributed to transpiration, diffusion, and pressure-induced flow of gaseous CH4 from the roots when they are embedded in CH4-saturated anaerobic sediments. Methane emissions from the emergent parts of aquatic plants were measured using floating chamber techniques and by enclosing the plants in polyethylene bags of known volume. Concentration changes were monitored in the trapped air using syringes and gas chromatographic techniques. Vertical profiles of dissolved CH4 in sediment pore water surrounding the aquatic plants' rhizomes were obtained using an interstitial sampling technique. Methane emissions from the aquatic plants studied varied from 14.8 mg CH4/d to levels too low to be detectable. Rooted and unrooted freshwater aquatic plants were studied as well as saltwater and brackish water plants. Included in the experiment is detailed set of measurements on CH4 emissions from the common cattail (Typha latifolia). This paper illustrates that aquatic plants play an important gas exchange role in the C cycle between wetlands and the atmosphere. |
[79] | . Methane release rates from rice paddies have been measured in Andalusia, Spain, during almost a complete vegetation period in 1982 using the static box system. The release rates ranged between 2 and 14 mg/m 2 /h and exhibited a strong seasonal variation with low values during the tillering stage and shortly before harvest, while maximum values were observed at the end of the flowering stage. The CH 4 release rate, averaged over the complete vegetation period, accounted for 4 mg/m 2 /h which results in a worldwide CH 4 emission from rice paddies of 35 59 10 12 g/yr if we assume that the observed CH 4 release rates are representative of global conditions. The CH 4 release rates showed diurnal variations with higher values late in the afternoon which were most likely caused by temperature variations within the upper layers of the paddy soils. Approximately 95% of the CH 4 emitted into the atmosphere by rice paddies was due to transport through the rice plants. Transport by bubbles or diffusion through the paddy water was of minor importance. Incubation experiments showed that CH 4 was neither produced nor consumed in the paddy water. The relase of CH 4 from rice paddies caused a diurnal variation of CH 4 in ambient air within the rice-growing area with maximum values of up to 2.3 ppmv during the early morning, compared to average daytime values of 1.75 ppmv. |
[80] | . Influence of urea application on growth parameters (shoot height, and weight, root volume, weight and porosity; number of tillers; grain yield) and their relationship with methane (CH 4 ) flux was investigated in Oryza sativa (var. Pant Dhan-4) under flooded soil condition. The study design consisted of (a) fertilized vegetated, (b) control vegetated, (c) fertilized bare, and (d) control bare plots. Crop growth and CH 4 flux measurements were conducted from 9 to 115 days of rice transplanting at regular intervals of 10 days. Results showed that there were significant differences due to days (dates of measurement) and fertilization in all growth parameters except shoot height. Day 脳 fertilization interaction was significant for all growth parameters. CH 4 fluxes ranged from 0.4 to 20.2, 0.1 to 11.9, 0.09 to 2.2 and 0.004 to 1.5 mg m -2 h -1 under treatments (a), (b), (c) and (d), respectively. Maximum CH 4 flux was recorded at the flowering stage. All the growth parameters, including number of tillers, showed strong positive relationship with total methane flux. Root porosity was also strongly correlated with total CH 4 emission. It was concluded that CH 4 emission was substantially influenced by crop phenology and growth, and fertilization. The study emphasizes the substrate production and conduit effects of rice plants on CH 4 flux. |
[81] | . |
[82] | |
[83] | . . |
[84] | . The gas flow pathways within L. and L. were evaluated by studying the internal pressurization and convective flow through individual leaves and the internal resistances to gas flow within the plants. Air enters the middle-aged leaves against a small pressure gradient by humidity-induced pressurization, is convected down against a small pressure gradient by humidity-induced pressurization, is convected down the lacunae of the leaves to the rhizome, and from there is vented back to the atmosphere, through old or damaged leaves or through horizontal rhizomes to other shoots. A model was developed to analyze the gas flow pattern within the plants under different conditions. The analysis showed that the throughflow pattern is dynamic: leaves can be influx leaves under a certain set of conditions and efflux leaves under different conditions. The specific internal resistance to gas flow was generally low in leaves (less than 1 Pa s mm) and rhizomes (3 Pa s mm), and somewhat higher in junctions between leaves and rhizome (5-14 Pa s mm). generally produced a greater leaf area specific convective flow rate (up to 31 碌l mincm) than (up to 11 碌l mincm). It was suggested that this greater ventilation capacity of may be significant for its ability to grow in deeper water, and may contribute to the explanation of the depth distribution of the two species. |
[85] | . |
[86] | . In two tidal freshwater marshes, methane emission,production and accumulation in the pore-water have beenstudied. The two sites differ in their dominantvegetation, i.e., reed and bulrush, and in theirheights above sea level. The reed site was elevated inrelation to the bulrush site and had higher rates ofmethane emission and production. It is argued thatthis difference in methane emission between sites wasprimarily due to a different effect of reed andbulrush plants on methane dynamics rather than methaneoxidation related to tidal elevation. Methane emissionshowed strong seasonality related primarily to plantphysiology and only secondarily to temperature. Twocontrol sites at which vegetation was removedsystematically had lower emission rates indicating anoverall stimulating effect of plants on methaneemission from tidal marshes. Flooding reduced methaneemission, probably by blocking the primary sites ofmethane release in the lower part of the plantstems. |
[87] | . Growth in stagnant, oxygen-deficient nutrient solution increased porosity in adventitious roots of two monocotyledonous (Carex acuta and Juncus effusus) and three dicotyledonous species (Caltha palustris, Ranunculus sceleratus and Rumex palustris) wetland species from 10 to 30% under aerated conditions to 20-45%. The spatial patterns of radial oxygen loss (ROL), determined with root-sleeving oxygen electrodes, indicated a strong constitutive 'barrier' to ROL in the basal root zones of the two monocotyledonous species. In contrast, roots of the dicotyledonous species showed no significant 'barrier' to ROL when grown in aerated solution, and only a partial 'barrier' when grown in stagnant conditions. This partial 'barrier' was strongest in C. palustris, so that ROL from basal zones of roots of R. sceleratus and R. palustris was substantial when compared to the monocotyledonous species. ROL from the basal zones would decrease longitudinal diffusion of oxygen to the root apex, and therefore limit the maximum penetration depth of these roots into anaerobic soil. Further studies of a larger number of dicotyledonous wetland species from a range of substrates are required to elucidate the ecophysiological consequences of developing a partial, rather than a strong, 'barrier' to ROL. |
[88] | . Methane emitted at different plant conditions through the different organs of rice plants was studied using a closed chamber technique under the laboratory, phytotron, and greenhouse conditions in order to clarify and quantify the role of different organs of rice plant as methane emission sites. Rice plants grown in flooded soils emit methane to the atmosphere via the aerenchyma of leaves, nodes and panicles. Emission through the rice plants is controlled by diffusion. No methane is emitted via the transpiration stream. Leaves are the major release sites at the early growth stage while nodes become more important later. Cracks and porous structure were found in the nodes. Panicles generally contribute little to methane emission. Increasing water depth temporarily reduces methane emission while concentration gradients in rice plants readjust to unsubmerged emission sites. Methane emissions in rice plants cease only when the plants become totally submerged. |
[89] | . . |
[90] | . . 盆栽结果表明,不同土壤类型铁、锰含量的差异是造成不同土壤类型间甲烷排放量差异甚大的重要因素之一。土壤中铁、锰通过对土壤氧化还原电位及根膜形成的影响而影响甲烷的排放。 |
[91] | . Rice plants play a pivotal role in different levels of the methane (CH 4 ) budget of rice fields. CH 4 production in rice fields largely depends on plant-borne material that can be either decaying tissue or root exudates. The quantity and quality of root exudates is affected by mechanical impedance, presence of toxic elements, nutrient deficiencies, water status of growing medium, and nitrogenase activity in the rhizosphere. CH 4 oxidation in rice fields is localized in the rhizosphere where the concentration gradients of CH 4 and oxygen overlap. CH 4 oxidation capacity is a function of the downward transport of oxygen through the aerenchyma, which, in turn, also acts as a conduit for CH 4 from the soil to the atmosphere. The decisive step in the passage of CH 4 through rice plant is the transition from root to stem. However, rice plants show an enormous variety of morphological and physiological properties, including differences in root exudation and gas transfer capacity. Comparative studies on different cultivars are deemed crucial for accomplishing a better understanding of the mechanisms of CH 4 consumption in the rhizosphere and CH 4 transport through the rice plant as well as the interaction of these processes. The results of such studies are considered tools for devising mitigation options. |
[92] | . The importance of vegetation in affecting CH4 emissions was investigated in a Carex-dominated fen located near Schefferville, Quebec, and in the Experimental Lakes Area, Ontario. Comparison of emission rates with and without the presence of aboveground vegetation indicated that over 90% of the emission was plant-associated transport. Further evidence of this association was found in a linear correlation of CH4 emission with aboveground plant biomass (R=0.93). To test the importance of aboveground plant photosynthetic production on methane production, aboveground vegetation was clipped from sites continually over the growing season. Both emissions and dissolved pore water CH4 were reduced relative to adjacent vegetated areas. A significant correlation (R=0.93) of CH4 emissions with net CO2 exchange in this fen gives evidence of the close association between new plant production and methanogenesis. |
[93] | . Methane emissions from Typha latifolia L. showed a large mid-morning transient peak associated with rising light levels. This peak was also associated with a steep decline in lacunal CH 4 concentrations near the stem base. This pattern contrasted sharply with emissions from Peltandra virginica (L.) Kunth that gradually rose to a peak in the mid-afternoon, corresponding to elevated air temperatures. Internal CH 4 concentrations within P. virginica stems did not change significantly over the diurnal period. Stomatal conductance appeared to correlate directly with light levels in both plant types and were not associated with peak CH 4 emission events in either plant. These patterns are consistent with a convective throughflow and diffusive gas ventilation systems for Typha and Peltandra , respectively. Further effects of the convective throughflow in T. latifolia were evident in the elevated CH 4 concentrations measured within brown leaves as contrasted to the near ambient levels measured within live green leaves. Experimental manipulation of elevated and reduced CO 2 levels in the atmosphere surrounding the plants and of light/dark periods suggested that stomatal aperture has little or no control of methane emissions from T. latifolia . |
[94] | . 淡水水生生态系统是全球陆域生态系统的重要组成部分,近年来,关于淡水水生生态系统温室气体排放的研究日 益增多.基于国内外目前对湖泊、河流、水库及浅水池塘等淡水生态系统开展的最新研究成果,总结分析了淡水水生生态系统温室气体排放的3个主要途径及相应观 测方法.气泡排放的观测方法有倒置漏斗法、开放式动态箱法和超声探测技术;植物传输的观测方法有密闭箱法和植株切割法;扩散途径的观测方法有静态浮箱法、 模型估算法/梯度法、微气象学法、TDLAS吸收光谱法等.从物理因素、化学因素、生物因素、水动力因素和人类活动等角度,深入探讨了淡水水生生态系统温 室气体排放通量的影响因素.最后根据当前研究中存在的不足,对今后的研究方向提出了建议,以期为我国进一步深入开展相关研究提供借鉴. |
[95] | |
[96] | . We measured the flow of methane in Typha latifolia L. (cattail)-dominated wetlands from microbial production in anoxic sediment into, through, and out of emergent T. latifolia shoots (i.e. plant transport). The purpose was to identify key environmental and plant factors that might affect rates of methane efflux from wetlands to the Earth's atmosphere. Methane accumulated in leafy T. latifolia shoots overnight, reaching concentrations up to 10000 0204l l 0908081 (vs. atmospheric concentrations <4 0204l l 0908081 ), suggesting that lower stomatal conductance at night limits methane efflux from the plant into ambient air. Daytime light and (or) lower atmospheric humidity that induce convective gas flow through the plant coincided with ( a ) an increase in the rate of methane efflux from T. latifolia leaves to ambient air (from <000·1 to >200·0 0204mol m 0908082 (leaf) s 0908081 ) and ( b ) a decrease in shoot methane concentration to <70 0204l l 0908081 . Very short fluctuation in stomatal conductance during the day did not affect the methane efflux rate unless, possibly, the rate of photosynthesis decreased. A strong relationship between the maximum daily rate of methane efflux and shoot methane concentration (measured before the onset of convective gas flow) suggests T. latifolia plants behave like a capacitor (filling with methane at night, emitting the stored methane during the day). Experimentally cutting leaves (to prevent pressurization) reduced plant capacitance for methane. |
[97] | . The separate closed chamber technique was used to study the potential of rice plants for transporting N 2 O and CH 4 produced in soil to the atmosphere. The results indicate that N 2 O produced in soil can be conducted to the atmosphere via rice plants similarly to CH 4 transport. More than 80% of both N 2 O and CH 4 was emitted through rice plants. The rest was emitted through the soil/water/atmosphere interface by ebullition and diffusion. Nitrate addition increased the total N 2 O emission rate substantially but decreased the total CH 4 emission. Nitrate addition did not change the CH 4 emission ratio through rice plants, but lowered the percentage of N 2 O emission through rice plants. The results suggest that rice plants serve not only as a conduit for most of the CH 4 leaving the soil but also for the N 2 O produced in the soil. |
[98] | . . 目前全球约有8.31亿hm2 的土壤受到盐渍化的威胁,造林绿化是盐碱地治理的重要措施之一,掌握植物盐胁迫的机理和研究趋势至关重要。笔者在分析国内外植物盐胁迫研究的基础上,从植 物形态结构变化、离子吸收及区隔化、渗透调节、抗氧化酶诱导及光合作用响应等方面总结了植物的耐盐机理,认为今后应当深入研究盐胁迫下植物不同器官和不同 组织的离子响应及光合特性的变化,将植物组织的功能和结构与环境因子的研究相结合,加强植物抗性基因及相关蛋白、植物改良剂等的研究,从而对盐碱地土地改 良和植被恢复提供更有效的实践指导。 |
[99] | . Effect of plant species richness on the methane (CH 4 ) flux was investigated in vertical flow microcosms fed with the modified Hoagland solution. The CH 4 flux increased significantly with the plant speciesrichness (Linear regression analysis, r 2 =0.203, P =0.010), with an average range from 1.59 to 4.30mgm 612 d 611 . Above-ground plant biomass followed the CH 4 flux trend with the plant species richness ( r 2 =0.108, P =0.023), but both methanogenic number and activity decreased to different extents with the plant species richness ( r 2 =0.103, P =0.023; r 2 =0.020, P =0.337), respectively. Accordingly, the present study highlights the significance of plant species diversity and its biomass production in manipulating the CH 4 flux in the constructed wetlands. |
[100] | . Freshwater marshes are the single largest source of atmospheric methane (CH4). Climate change and anthropogenic nitrogen (N) deposition can alter the production and oxidation of CH4 respectively and thereby also CH4 exchange. But there was little known about how N regulate key carbon (C) processes, like CH4 production and oxidation under freshwater marshland conditions. In this study, field-fertilization and incubation experiments were conducted to investigate the effects of four N-fertilization treatments (0, 6, 12, 24 g N m-2) using ammonium nitrate (NH4NO3) on CH4 production and oxidation and the potential mechanisms involved, in the Sanjiang Plain, Northeast China. Both the CH4 production and oxidation of N24 (24 g N m-2) treatment was significantly higher than control and other N treatments. The ammonium nitrogen (NH4+--N), nitrate nitrogen (NO3---N), dissolved organic carbon (DOC) and dissolved inorganic carbon (DIC) concentrations were higher at the N24 treatment compared with the control. The incubation experiment indicated that N-addition stimulated CH4 production more than CH4 oxidation resulting in net CH4 emission from N-fertilization treatments. |
[101] | . . 稻田生态系统中CH_4排放是由土壤中CH_4产生、氧化和向大气传输这3个过程相互作用的结果,CH_4传输主要通过液相扩散、冒气泡和植株传输3种方式。这3种途径的相对强弱取决于水稻植株通气组织的完善与否以及水稻品种、种植密度和温度的季节变化等。但大量研究表明,稻田土壤中产生的CH_4绝大部分通过植株通气组织排放到大气中。在应用稳定性碳同位素方法研究水稻植株向大气传输CH_4的过程时发现,在传输过程也会发生同位素分馏,据现有文献报道,CH_4传输的同位素分馏系数ε_(传输)有两种计算方法,获得的结果也比较接近,为-18‰~-9‰。但研究方法还存在一些缺陷,可能对结果的准确性产生影响。此外有关稻田CH_4传输在模型的建立方面还比较缺乏。 |
[102] | . To find an effective approach that improves nitrogen removal while reducing methane (CH 4 ) emission during the process of wastewater treatment in constructed wetlands (CWs), we conducted a microcosm experiment simulating CWs with two treatments. The first treatment was plant diversity (species richness and identity), and the second treatment was substrate sand particle size (fine sand and coarse sand). Results showed that, (1) the range of CH 4 emission in fine sand microcosms (618.46 to 21.0302mg CH 4 m 612 02d 611 ) was much greater than that in coarse sand (5.06 to 6.8502mg CH 4 m 612 02d 611 ); (2) in coarse sand microcosms, plant species richness increased nitrogen removal ( P 02020.05); (3) in fine sand microcosms, species identity surpassed species richness as a key driver of CH 4 emission, and the presence of Oenanthe javanica significantly decreased CH 4 emission, whereas the presence of Rumex japonicus notably increased CH 4 emission ( P 02<020.05, respectively); (4) the nitrogen removal in fine sand microcosms was higher than in coarse sand microcosms ( P 02<020.05). Results also showed that Phalaris arundinacea monoculture in fine sand microcosms had a high N removal and low CH 4 emission. Overall, we conclude that the best combination of low CH 4 emission and high N removal rates could be achieved in fine sand microcosms with special plant species as P. arundinacea . |
1
2017
... 大气中的CH4有70%-80%来自生物源(
Field measurements of internal pressurization in Phragmites australis(Poaceae) and implications for regulation of methane emissions in a midlatitude prairie wetland
3
2001
... 对流是植物传输CH4的另一种重要机制.不同于扩散传输, 对流传输主要依靠植物内外的压强差来驱使气体运动(
... 植物在光照充足条件下CH4的传输速率往往高于在弱光或无光照情况下(
... 温度对植物内CH4的对流传输有着十分重要的调控作用.研究发现, 当外界温度较高时, 植物内部的对流速率往往也会提高(
Light enhanced convective through flow increases oxygenation in rhizomes and rhizosphere of Phragmites australis (Cav.) Trin. ex Steud
1
1990
... 植物在光照充足条件下CH4的传输速率往往高于在弱光或无光照情况下(
A convective through-flow of gases in Phragmites australis (Cav.) Trin. ex Steud
1
1991
... 风会影响植物的气体交换性能(
Phragmites australis: Venturi and humidity induced pressure flows enhance rhizome aeration and rhizosphere oxidation
2
1992
... 风会影响植物的气体交换性能(
... ).例如, 当空气流经芦苇较高处枯萎或受损伤的部位时, 在较短时间内会使局部气压降低而产生吸力, 使得茎和根茎部位的气体被吸引上来(
Pathways of aeration and the mechanisms and beneficial effects of humidity- and Venturi- induced convections inPhragmites australis(Cav.) Trin. ex Steud
1
1996
... 风会影响植物的气体交换性能(
Convective gas-flows in wetland plant aeration
4
1991
... 除了活体植物外, 受损伤的、枯死的植株或部位也是CH4的传输排放通道.当存在枯萎的植株时, 其内部的静态压力比鲜活、完整植株低, 这促使植物内气体多通过鲜活完整的植株向地下输送, 而由受损或枯死植株传输排出(
... 植物在光照充足条件下CH4的传输速率往往高于在弱光或无光照情况下(
... 的作用机理主要体现在以下几个方面: 首先, 在一定范围内, 光照增加会增强光合作用(
... 温度对植物内CH4的对流传输有着十分重要的调控作用.研究发现, 当外界温度较高时, 植物内部的对流速率往往也会提高(
Methane transport capacity of rice plants. I. Influence of methane concentration and growth stage analyzed with an automated measuring system
4
2000a
... 根系不同部位的CH4传输性能被发现存在差异.一方面, 从整个根系系统来看, Henneberg等(2012)在对灯心草(Juncus effusus)的研究中发现, CH4主要通过侧根进入植物体.这可能是由于侧根是植物根际物质交换的主要部位(
... 根茎连接部位是CH4由根系进入茎叶的过渡部位, 它在结构上与根、茎存在明显差异.根茎连接部位对CH4传输影响显著: 无论是早期Butterbach- Bahl等(1997)对水稻根茎结合部位进行的剪切试验, 还是后来Groot等(2005)的六氟化硫示踪试验, 均表明根茎连接部位具有较大的气体传输阻力, 是调控植物传输CH4整个过程的一个重要部位(
... 通气组织是CH4在植物内长距离高效传输的主要通道和载体, 因此其结构与形态对于CH4传输十分重要.研究指出, 通气组织的数量、大小与CH4传输通量存在正相关关系(
... 许多****发现水稻在生长初期的CH4传输排放量较小, 但是随着生长发育不断变化, 一般在抽穗中期或开花期CH4传输排放达到峰值, 之后逐渐减小(
Pattern and amount of aerenchyma relate to variable methane transport capacity of different rice cultivars
2000b
Mathematical modelling of methane transport by Phragmites: The potential for diffusion within the roots and rhizosphere
2
2001
... 土-水环境中, CH4由根际进入根系是植物传输CH4的初始过程.根系被认为是植物与土-水-气系统间进行气体交换的关键部位(
... 根系不同部位的CH4传输性能被发现存在差异.一方面, 从整个根系系统来看, Henneberg等(2012)在对灯心草(Juncus effusus)的研究中发现, CH4主要通过侧根进入植物体.这可能是由于侧根是植物根际物质交换的主要部位(
Internal gas transport in Typha latifolia L. and Typha angustifolia L. 1. Humidity- induced pressurization and convective throughflow
2
1994
... 对流是植物传输CH4的另一种重要机制.不同于扩散传输, 对流传输主要依靠植物内外的压强差来驱使气体运动(
... 湿度是影响植物传输CH4的又一重要环境因子.早期, Armstrong和Armstrong (1991)对芦苇的室内试验发现, 同在24 ℃中, 处于相对湿度为41%环境下植物的对流速率是相对湿度为74%环境下的3.5倍.后期大量研究同样表明, 湿度诱导下的对流作用会随着外界湿度的增加而减弱(
The role of Phragmites in the CH4 and CO2 fluxes in a minerotrophic peatland in southwest Germany
5
2016
... 大气中的CH4有70%-80%来自生物源(
... 除了活体植物外, 受损伤的、枯死的植株或部位也是CH4的传输排放通道.当存在枯萎的植株时, 其内部的静态压力比鲜活、完整植株低, 这促使植物内气体多通过鲜活完整的植株向地下输送, 而由受损或枯死植株传输排出(
... 光合作用对植物传输CH4的影响作用尚无定论.早期有****指出, 植物的CH4传输效率并不依赖于光合作用(
... 植物在光照充足条件下CH4的传输速率往往高于在弱光或无光照情况下(
... ;
Diffusive gas transport through flooded rice systems
1
2001
... 扩散是气体分子在连通空间内由浓度差而诱发的自高浓度向低浓度的运动, 常与分子扩散系数、浓度梯度差以及连接不同空间通道的面积呈正相关关系(
Methane emissions from wetlands: Biogeochemical, microbial and modeling perspectives from local to global scales
1
2013
... 大气中的CH4有70%-80%来自生物源(
Gas exchange through dead culms of reed,Phragmites australis(Cav.) Trin. ex Steud
1
1989
... 除了活体植物外, 受损伤的、枯死的植株或部位也是CH4的传输排放通道.当存在枯萎的植株时, 其内部的静态压力比鲜活、完整植株低, 这促使植物内气体多通过鲜活完整的植株向地下输送, 而由受损或枯死植株传输排出(
Internal pressurization and convective gas flow in some emergent freshwater macrophytes
7
1992
... 根茎连接部位是CH4由根系进入茎叶的过渡部位, 它在结构上与根、茎存在明显差异.根茎连接部位对CH4传输影响显著: 无论是早期Butterbach- Bahl等(1997)对水稻根茎结合部位进行的剪切试验, 还是后来Groot等(2005)的六氟化硫示踪试验, 均表明根茎连接部位具有较大的气体传输阻力, 是调控植物传输CH4整个过程的一个重要部位(
... 对流是植物传输CH4的另一种重要机制.不同于扩散传输, 对流传输主要依靠植物内外的压强差来驱使气体运动(
... ;
... )和物种适应厌氧环境(
... )等, 其中以湿度和温度的调控作用更为普遍(
... ).温度主要通过植物体内外或植物不同部位间的温度梯度来诱导对流, 而湿度的诱导作用更多来源于水汽压差(
... 较早的研究发现, CH4在植物内基于液流的传输量很少, 这是因为CH4的传输速率并不依赖于蒸腾速率(
Gas fluxes achieved by in situ convective flow in Phragmites australis
4
1996
... 许多****发现水稻在生长初期的CH4传输排放量较小, 但是随着生长发育不断变化, 一般在抽穗中期或开花期CH4传输排放达到峰值, 之后逐渐减小(
... 植物在光照充足条件下CH4的传输速率往往高于在弱光或无光照情况下(
... 温度对植物内CH4的对流传输有着十分重要的调控作用.研究发现, 当外界温度较高时, 植物内部的对流速率往往也会提高(
... 湿度是影响植物传输CH4的又一重要环境因子.早期, Armstrong和Armstrong (1991)对芦苇的室内试验发现, 同在24 ℃中, 处于相对湿度为41%环境下植物的对流速率是相对湿度为74%环境下的3.5倍.后期大量研究同样表明, 湿度诱导下的对流作用会随着外界湿度的增加而减弱(
Impact of gas transport through rice cultivars on methane emission from rice paddy fields
1
1997
... 物种类型是植物传输CH4能力的重要影响因素(
水稻根系对甲烷传输速率的影响
1
2003
... 表皮层, 然后进入皮层并在此气化或脱离水相体系(可能是由于植物细胞主动吸收水分而未吸收CH4的原因), 气化后的CH4经细胞间隙与通气组织向植物上部传输(
水稻植株状况对甲烷传输速率的影响及其品种间差异
2
2005
... 根茎连接部位是CH4由根系进入茎叶的过渡部位, 它在结构上与根、茎存在明显差异.根茎连接部位对CH4传输影响显著: 无论是早期Butterbach- Bahl等(1997)对水稻根茎结合部位进行的剪切试验, 还是后来Groot等(2005)的六氟化硫示踪试验, 均表明根茎连接部位具有较大的气体传输阻力, 是调控植物传输CH4整个过程的一个重要部位(
... 物种类型是植物传输CH4能力的重要影响因素(
Diel variation in lacunal CH4 and CO2 concentration and δ13C in Phragmites australis
1
2002
... 大气中的CH4有70%-80%来自生物源(
Contrasting rates and diurnal patterns of methane emission from emergent aquatic macrophytes
1993
Methane flux from Peltandra virginica: Stable isotope tracing and chamber effects
1
1992
... 对流是植物传输CH4的另一种重要机制.不同于扩散传输, 对流传输主要依靠植物内外的压强差来驱使气体运动(
湿地甲烷的产生、氧化及排放通量研究进展
1
2006
... 大气中的CH4有70%-80%来自生物源(
挺水植物的气体交换与输导代谢
5
2003
... 大气中的CH4有70%-80%来自生物源(
... 扩散是气体分子在连通空间内由浓度差而诱发的自高浓度向低浓度的运动, 常与分子扩散系数、浓度梯度差以及连接不同空间通道的面积呈正相关关系(
... 对流是植物传输CH4的另一种重要机制.不同于扩散传输, 对流传输主要依靠植物内外的压强差来驱使气体运动(
... 植物在光照充足条件下CH4的传输速率往往高于在弱光或无光照情况下(
... ;
Long-distance transport of gases in plants: a perspective on internal aeration and radial oxygen loss from roots
4
2003
... 大气中的CH4有70%-80%来自生物源(
... 根系不同部位的CH4传输性能被发现存在差异.一方面, 从整个根系系统来看, Henneberg等(2012)在对灯心草(Juncus effusus)的研究中发现, CH4主要通过侧根进入植物体.这可能是由于侧根是植物根际物质交换的主要部位(
... 通气组织是CH4在植物内长距离高效传输的主要通道和载体, 因此其结构与形态对于CH4传输十分重要.研究指出, 通气组织的数量、大小与CH4传输通量存在正相关关系(
... 风会影响植物的气体交换性能(
植物在CH4产生、氧化和排放中的作用
3
2003
... 大气中的CH4有70%-80%来自生物源(
... 根茎连接部位是CH4由根系进入茎叶的过渡部位, 它在结构上与根、茎存在明显差异.根茎连接部位对CH4传输影响显著: 无论是早期Butterbach- Bahl等(1997)对水稻根茎结合部位进行的剪切试验, 还是后来Groot等(2005)的六氟化硫示踪试验, 均表明根茎连接部位具有较大的气体传输阻力, 是调控植物传输CH4整个过程的一个重要部位(
... 许多****发现水稻在生长初期的CH4传输排放量较小, 但是随着生长发育不断变化, 一般在抽穗中期或开花期CH4传输排放达到峰值, 之后逐渐减小(
Aquatic herbivores facilitate the emission of methane from wetlands
1
2011
... 除了活体植物外, 受损伤的、枯死的植株或部位也是CH4的传输排放通道.当存在枯萎的植株时, 其内部的静态压力比鲜活、完整植株低, 这促使植物内气体多通过鲜活完整的植株向地下输送, 而由受损或枯死植株传输排出(
乌梁素海湖泊湿地植物区甲烷排放规律
2
2007
... 许多****发现水稻在生长初期的CH4传输排放量较小, 但是随着生长发育不断变化, 一般在抽穗中期或开花期CH4传输排放达到峰值, 之后逐渐减小(
... 植物在光照充足条件下CH4的传输速率往往高于在弱光或无光照情况下(
维管植物对自然湿地甲烷排放的影响
5
2005
... 扩散是气体分子在连通空间内由浓度差而诱发的自高浓度向低浓度的运动, 常与分子扩散系数、浓度梯度差以及连接不同空间通道的面积呈正相关关系(
... ;
... 对流是植物传输CH4的另一种重要机制.不同于扩散传输, 对流传输主要依靠植物内外的压强差来驱使气体运动(
... )、互花米草(
... 植物在光照充足条件下CH4的传输速率往往高于在弱光或无光照情况下(
盐分对湿地甲烷排放影响的研究进展
1
2015
... 大气中的CH4有70%-80%来自生物源(
水稻根系生物特性与稻田温室气体排放相关性研究
2
2012
... 物种类型是植物传输CH4能力的重要影响因素(
... 传输效率的差异通常归因于它们不同的形态结构, 其中外部形态包括根系体积与质量(
Physiological control of leaf methane emission from wetland plants
1
2005
... 温度对植物内CH4的对流传输有着十分重要的调控作用.研究发现, 当外界温度较高时, 植物内部的对流速率往往也会提高(
Assessment of O2 diffusivity across the barrier to radial O2 loss in adventitious roots of Hordeum marinum
1
2008
... 根系不同部位的CH4传输性能被发现存在差异.一方面, 从整个根系系统来看, Henneberg等(2012)在对灯心草(Juncus effusus)的研究中发现, CH4主要通过侧根进入植物体.这可能是由于侧根是植物根际物质交换的主要部位(
Gas transport through the root-shoot transition zone of rice tillers
1
2005
... 根茎连接部位是CH4由根系进入茎叶的过渡部位, 它在结构上与根、茎存在明显差异.根茎连接部位对CH4传输影响显著: 无论是早期Butterbach- Bahl等(1997)对水稻根茎结合部位进行的剪切试验, 还是后来Groot等(2005)的六氟化硫示踪试验, 均表明根茎连接部位具有较大的气体传输阻力, 是调控植物传输CH4整个过程的一个重要部位(
Pressurized ventilation in wetland plants
3
1991
... 对流是植物传输CH4的另一种重要机制.不同于扩散传输, 对流传输主要依靠植物内外的压强差来驱使气体运动(
... ).对流在输送效率(
... 较早的研究发现, CH4在植物内基于液流的传输量很少, 这是因为CH4的传输速率并不依赖于蒸腾速率(
Plant species diversity reduces N2O but not CH4 emissions from constructed wetlands under high nitrogen levels
1
2017
... 物种类型是植物传输CH4能力的重要影响因素(
Locus of methane release and mass-dependent fractionation from two wetland macrophytes
1
1994
... 自然环境下, CH4由于自身沸点低, 主要以气态的方式存在, 其密度在标准状况下为0.717 g·L-1, 要低于近地面大气的密度, 这可能有利于其在植物通气组织内向上扩散传输并排放到大气中.同时CH4作为一种非极性分子, 溶解度低(
Internal methane transport through Juncus effusus: Experimental manipulation of morphological barriers to test above- and below-ground diffusion limitation
1
2012
... 根系不同部位的CH4传输性能被发现存在差异.一方面, 从整个根系系统来看, Henneberg等(2012)在对灯心草(Juncus effusus)的研究中发现, CH4主要通过侧根进入植物体.这可能是由于侧根是植物根际物质交换的主要部位(
Plant-mediated methane and nitrous oxide fluxes from a carex meadow in Poyang Lake during drawdown periods
2
2016
... 土-水环境中, CH4由根际进入根系是植物传输CH4的初始过程.根系被认为是植物与土-水-气系统间进行气体交换的关键部位(
... 生物量由于通常与通气组织数量密切相关, 因而影响CH4传输.植物地上生物量与CH4传输排放存在显著正相关关系, 这已在湿生薹草(Carex limosa)和灰株薹草(Carex rostrata) (
氮肥对旱地土壤甲烷氧化能力的影响
1
2004
... 土壤性质通过影响植物形态结构与生理活动, 进而影响CH4传输, 但是相关研究较少.例如盐分的增加会促进喜旱莲子草(Alternanthera philoxeroides)通气组织的发展, 使根、茎与叶部位胞间隙相连(
高氮磷胁迫下菖蒲(Acorus calamus Linn.)通气组织和根系释氧的响应
1
2012
... 土壤性质通过影响植物形态结构与生理活动, 进而影响CH4传输, 但是相关研究较少.例如盐分的增加会促进喜旱莲子草(Alternanthera philoxeroides)通气组织的发展, 使根、茎与叶部位胞间隙相连(
沼泽湿地互花米草植物体传输与排放甲烷特征
1
2011
... 茎叶向大气排放CH4是植物传输CH4的最终环节, 目前针对茎叶排放CH4位置方面的研究较多.早期, 气孔被认为是CH4传输排放的重要部位(
Methane emission from Texas rice paddy soils. 2. Seasonal contribution of rice biomass production to CH4 emission
1
1997
... 生物量由于通常与通气组织数量密切相关, 因而影响CH4传输.植物地上生物量与CH4传输排放存在显著正相关关系, 这已在湿生薹草(Carex limosa)和灰株薹草(Carex rostrata) (
3
2013
... 甲烷(CH4)是大气中的一种痕量气体, 它参与大气光化学反应(
... , 比工业革命前增加了1.5倍(
... 根系不同部位的CH4传输性能被发现存在差异.一方面, 从整个根系系统来看, Henneberg等(2012)在对灯心草(Juncus effusus)的研究中发现, CH4主要通过侧根进入植物体.这可能是由于侧根是植物根际物质交换的主要部位(
Formation of aerenchyma and the processes of plant ventilation in relation to soil flooding and submergence
1
1999
... 表皮层, 然后进入皮层并在此气化或脱离水相体系(可能是由于植物细胞主动吸收水分而未吸收CH4的原因), 气化后的CH4经细胞间隙与通气组织向植物上部传输(
水稻植株对稻田甲烷排放的影响
1
2003
... 根系不同部位的CH4传输性能被发现存在差异.一方面, 从整个根系系统来看, Henneberg等(2012)在对灯心草(Juncus effusus)的研究中发现, CH4主要通过侧根进入植物体.这可能是由于侧根是植物根际物质交换的主要部位(
The anatomical characteristics of roots and plant response to soil flooding
1987
Diel variation in methane emissions from stands of Phragmites australis(Cav.) Trin. ex Steud. and Typha latifolia L. in a boreal lake
2001
The effect of clipping on methane emissions from
1997
Seasonal variation in methane emission from a temperate Phragmites-dominated marsh: Effect of growth stage and plant-mediated transport
1
1999
... 光合作用对植物传输CH4的影响作用尚无定论.早期有****指出, 植物的CH4传输效率并不依赖于光合作用(
A pulse-labeling experiment to determine the contribution of recent plant photosynthates to net methane emission in arctic wet sedge tundra
2002
Aerenchyma formation and methane and oxygen exchange in rice
1
1993
... 茎叶向大气排放CH4是植物传输CH4的最终环节, 目前针对茎叶排放CH4位置方面的研究较多.早期, 气孔被认为是CH4传输排放的重要部位(
Evaluation of a closed-chamber method for estimating methane emissions from aquatic plants
1
1992
... 对流是植物传输CH4的另一种重要机制.不同于扩散传输, 对流传输主要依靠植物内外的压强差来驱使气体运动(
Methane emission from natural wetlands: Interplay between emergent macrophytes and soil microbial processes. A mini-review
2
2010
... 物种类型是植物传输CH4能力的重要影响因素(
... 生物量由于通常与通气组织数量密切相关, 因而影响CH4传输.植物地上生物量与CH4传输排放存在显著正相关关系, 这已在湿生薹草(Carex limosa)和灰株薹草(Carex rostrata) (
Wetland invasion by Typha × glauca increases soil methane emissions
2
2017
... 茎叶向大气排放CH4是植物传输CH4的最终环节, 目前针对茎叶排放CH4位置方面的研究较多.早期, 气孔被认为是CH4传输排放的重要部位(
... 较早的研究发现, CH4在植物内基于液流的传输量很少, 这是因为CH4的传输速率并不依赖于蒸腾速率(
Gas transport through rice
1
1981
... 物种类型是植物传输CH4能力的重要影响因素(
Effect of rice variety on methane emission from Louisiana rice
1
1995
... 土壤性质通过影响植物形态结构与生理活动, 进而影响CH4传输, 但是相关研究较少.例如盐分的增加会促进喜旱莲子草(Alternanthera philoxeroides)通气组织的发展, 使根、茎与叶部位胞间隙相连(
NaCl处理对空心莲子草营养器官解剖结构的影响
2007
闽江河口芦苇湿地植物传输甲烷研究
3
2010
... 温度对植物内CH4的对流传输有着十分重要的调控作用.研究发现, 当外界温度较高时, 植物内部的对流速率往往也会提高(
... 水位显著影响植物对CH4的传输排放.在对芦苇、水稻等物种的水位调控及密闭箱收集试验中发现, 较高的水位抑制CH4的传输排放(
... ;
芦苇在微咸水河口湿地甲烷排放中的作用
5
2011
... 大气中的CH4有70%-80%来自生物源(
... ), 其中水生与湿生环境是两个主要排放源(
... 排放是产生、消耗(氧化)和传输3个环节综合作用的结果; 但是与产生和消耗二者相比, 目前对于传输的研究比较薄弱(
... 传输有3种途径: 扩散、气泡和植物传输, 其中常以挺水与湿生草本植物传输为主(
... 湿地土-水环境中, CH4在产生率不高时一般不会或极少产生气泡, 此时溶解态的CH4主要以液相扩散的方式进行扩散迁移(
Production, oxidation, emission and consumption of methane by soils: A Review
1
2001
... 生物量由于通常与通气组织数量密切相关, 因而影响CH4传输.植物地上生物量与CH4传输排放存在显著正相关关系, 这已在湿生薹草(Carex limosa)和灰株薹草(Carex rostrata) (
Effect of continuous and alternate water regimes, on methane efflux from rice under greenhouse conditions
1
1997
... 物种类型是植物传输CH4能力的重要影响因素(
Does richness of emergent plants affect CO2 and CH4 emissions in experimental wetlands?
1
2015
... 甲烷(CH4)是大气中的一种痕量气体, 它参与大气光化学反应(
Non-CO2 greenhouse gases and climate change
1
2011
... 茎叶向大气排放CH4是植物传输CH4的最终环节, 目前针对茎叶排放CH4位置方面的研究较多.早期, 气孔被认为是CH4传输排放的重要部位(
Significance of stomatal control on methane release fromCarex-dominated wetlands
1
1993
... 土壤性质通过影响植物形态结构与生理活动, 进而影响CH4传输, 但是相关研究较少.例如盐分的增加会促进喜旱莲子草(Alternanthera philoxeroides)通气组织的发展, 使根、茎与叶部位胞间隙相连(
Physiological processes limiting plant growth in saline soils: Some dogmas and hypotheses
1
1993
... 物种类型是植物传输CH4能力的重要影响因素(
Factors affecting methane emission from rice fields
1
1996
... 许多****发现水稻在生长初期的CH4传输排放量较小, 但是随着生长发育不断变化, 一般在抽穗中期或开花期CH4传输排放达到峰值, 之后逐渐减小(
Seasonal variation in methane flux from rice paddies associated with methane concentration in soil water, rice biomass and temperature, and its modelling
1994
2
1993
... 土-水环境中, CH4由根际进入根系是植物传输CH4的初始过程.根系被认为是植物与土-水-气系统间进行气体交换的关键部位(
... 茎叶向大气排放CH4是植物传输CH4的最终环节, 目前针对茎叶排放CH4位置方面的研究较多.早期, 气孔被认为是CH4传输排放的重要部位(
Mechanism of methane transport from the rhizosphere to the atmosphere through rice plants
1
1990
... 除了活体植物外, 受损伤的、枯死的植株或部位也是CH4的传输排放通道.当存在枯萎的植株时, 其内部的静态压力比鲜活、完整植株低, 这促使植物内气体多通过鲜活完整的植株向地下输送, 而由受损或枯死植株传输排出(
Herbivore damage increases methane emission from emergent aquatic macrophytes
1
2015
... 许多****发现水稻在生长初期的CH4传输排放量较小, 但是随着生长发育不断变化, 一般在抽穗中期或开花期CH4传输排放达到峰值, 之后逐渐减小(
Dissolved gas dynamics in wetland soils: Root-mediated gas transfer kinetics determined via push-pull tracer tests
3
2015
... 大气中的CH4有70%-80%来自生物源(
... 茎叶向大气排放CH4是植物传输CH4的最终环节, 目前针对茎叶排放CH4位置方面的研究较多.早期, 气孔被认为是CH4传输排放的重要部位(
... 物种类型是植物传输CH4能力的重要影响因素(
Plant transport and methane production as controls on methane flux from arctic wet meadow tundra
1
1995
... 通气组织是CH4在植物内长距离高效传输的主要通道和载体, 因此其结构与形态对于CH4传输十分重要.研究指出, 通气组织的数量、大小与CH4传输通量存在正相关关系(
Influence of stem lacunar structure on gas transport: Relation to the oxygen transport potential of submersed vascular plants
1994
A 3-year continuous record on the influence of daytime, season, and fertilizer treatment on methane emission rates from an Italian rice paddy
2
1989a
... 大气中的CH4有70%-80%来自生物源(
... 许多****发现水稻在生长初期的CH4传输排放量较小, 但是随着生长发育不断变化, 一般在抽穗中期或开花期CH4传输排放达到峰值, 之后逐渐减小(
Processes involved in formation and emission of methane in rice paddies
2
1989b
... 除了活体植物外, 受损伤的、枯死的植株或部位也是CH4的传输排放通道.当存在枯萎的植株时, 其内部的静态压力比鲜活、完整植株低, 这促使植物内气体多通过鲜活完整的植株向地下输送, 而由受损或枯死植株传输排出(
... 物种类型是植物传输CH4能力的重要影响因素(
Methane emissions to the atmosphere through aquatic plants
3
1985
... 茎叶向大气排放CH4是植物传输CH4的最终环节, 目前针对茎叶排放CH4位置方面的研究较多.早期, 气孔被认为是CH4传输排放的重要部位(
... 许多****发现水稻在生长初期的CH4传输排放量较小, 但是随着生长发育不断变化, 一般在抽穗中期或开花期CH4传输排放达到峰值, 之后逐渐减小(
... 光合作用对植物传输CH4的影响作用尚无定论.早期有****指出, 植物的CH4传输效率并不依赖于光合作用(
Methane emission from rice paddies
2
1984
... 根茎连接部位是CH4由根系进入茎叶的过渡部位, 它在结构上与根、茎存在明显差异.根茎连接部位对CH4传输影响显著: 无论是早期Butterbach- Bahl等(1997)对水稻根茎结合部位进行的剪切试验, 还是后来Groot等(2005)的六氟化硫示踪试验, 均表明根茎连接部位具有较大的气体传输阻力, 是调控植物传输CH4整个过程的一个重要部位(
... 土壤性质通过影响植物形态结构与生理活动, 进而影响CH4传输, 但是相关研究较少.例如盐分的增加会促进喜旱莲子草(Alternanthera philoxeroides)通气组织的发展, 使根、茎与叶部位胞间隙相连(
Methane flux in relation to growth and phenology of a high yielding rice variety as affected by fertilization
2
1998
... 对流是植物传输CH4的另一种重要机制.不同于扩散传输, 对流传输主要依靠植物内外的压强差来驱使气体运动(
... (
Convective gas flow in Eleocharis sphacelata R. Br.: Methane transport and release from wetlands
1
1994
... 通气组织是CH4在植物内长距离高效传输的主要通道和载体, 因此其结构与形态对于CH4传输十分重要.研究指出, 通气组织的数量、大小与CH4传输通量存在正相关关系(
1997
闽江河口芦苇潮汐湿地甲烷通量及主要影响因子
1
2009
... 对流是植物传输CH4的另一种重要机制.不同于扩散传输, 对流传输主要依靠植物内外的压强差来驱使气体运动(
Internal gas transport in Typha latifolia L. and Typha angustifolia L. 2. Convective throughflow pathways and ecological significance
2
1994
... 扩散是气体分子在连通空间内由浓度差而诱发的自高浓度向低浓度的运动, 常与分子扩散系数、浓度梯度差以及连接不同空间通道的面积呈正相关关系(
... 对流是植物传输CH4的另一种重要机制.不同于扩散传输, 对流传输主要依靠植物内外的压强差来驱使气体运动(
Diel methane emission patterns from Scirpus lacustris and Phragmites australis
2
1998
... 茎叶向大气排放CH4是植物传输CH4的最终环节, 目前针对茎叶排放CH4位置方面的研究较多.早期, 气孔被认为是CH4传输排放的重要部位(
... 水位显著影响植物对CH4的传输排放.在对芦苇、水稻等物种的水位调控及密闭箱收集试验中发现, 较高的水位抑制CH4的传输排放(
Methane emission from tidal freshwater marshes
2
2000
... 根系不同部位的CH4传输性能被发现存在差异.一方面, 从整个根系系统来看, Henneberg等(2012)在对灯心草(Juncus effusus)的研究中发现, CH4主要通过侧根进入植物体.这可能是由于侧根是植物根际物质交换的主要部位(
... ;
Changes in growth, porosity, and radial oxygen loss from adventitious roots of selected mono- and dicotyledonous wetland species with contrasting types of aerenchyma
2
2000
... 表皮层, 然后进入皮层并在此气化或脱离水相体系(可能是由于植物细胞主动吸收水分而未吸收CH4的原因), 气化后的CH4经细胞间隙与通气组织向植物上部传输(
... 水位显著影响植物对CH4的传输排放.在对芦苇、水稻等物种的水位调控及密闭箱收集试验中发现, 较高的水位抑制CH4的传输排放(
Role of rice in mediating methane emission
2
1997
... 表皮层, 然后进入皮层并在此气化或脱离水相体系(可能是由于植物细胞主动吸收水分而未吸收CH4的原因), 气化后的CH4经细胞间隙与通气组织向植物上部传输(
... 许多****发现水稻在生长初期的CH4传输排放量较小, 但是随着生长发育不断变化, 一般在抽穗中期或开花期CH4传输排放达到峰值, 之后逐渐减小(
稻田甲烷排放及产生、转化、输送机理
1
1998
... 土壤性质通过影响植物形态结构与生理活动, 进而影响CH4传输, 但是相关研究较少.例如盐分的增加会促进喜旱莲子草(Alternanthera philoxeroides)通气组织的发展, 使根、茎与叶部位胞间隙相连(
不同母质发育的水稻土中铁、锰对甲烷排放的影响
1
1998
... 根茎连接部位是CH4由根系进入茎叶的过渡部位, 它在结构上与根、茎存在明显差异.根茎连接部位对CH4传输影响显著: 无论是早期Butterbach- Bahl等(1997)对水稻根茎结合部位进行的剪切试验, 还是后来Groot等(2005)的六氟化硫示踪试验, 均表明根茎连接部位具有较大的气体传输阻力, 是调控植物传输CH4整个过程的一个重要部位(
The role of rice plants in regulating mechanisms of methane emissions
4
2000
... 大气中的CH4有70%-80%来自生物源(
... 生物量由于通常与通气组织数量密切相关, 因而影响CH4传输.植物地上生物量与CH4传输排放存在显著正相关关系, 这已在湿生薹草(Carex limosa)和灰株薹草(Carex rostrata) (
... 光合作用对植物传输CH4的影响作用尚无定论.早期有****指出, 植物的CH4传输效率并不依赖于光合作用(
... 植物在光照充足条件下CH4的传输速率往往高于在弱光或无光照情况下(
Plant-dependent CH4 emission in a subarctic Canadian fen
4
1992
... 扩散是气体分子在连通空间内由浓度差而诱发的自高浓度向低浓度的运动, 常与分子扩散系数、浓度梯度差以及连接不同空间通道的面积呈正相关关系(
... 对流是植物传输CH4的另一种重要机制.不同于扩散传输, 对流传输主要依靠植物内外的压强差来驱使气体运动(
... 物种类型是植物传输CH4能力的重要影响因素(
... 植物在光照充足条件下CH4的传输速率往往高于在弱光或无光照情况下(
Control of the diurnal pattern of methane emission from emergent aquatic macrophytes by gas transport mechanisms
4
1996
... 湿地土-水环境中, CH4在产生率不高时一般不会或极少产生气泡, 此时溶解态的CH4主要以液相扩散的方式进行扩散迁移(
... 扩散是气体分子在连通空间内由浓度差而诱发的自高浓度向低浓度的运动, 常与分子扩散系数、浓度梯度差以及连接不同空间通道的面积呈正相关关系(
... 对流是植物传输CH4的另一种重要机制.不同于扩散传输, 对流传输主要依靠植物内外的压强差来驱使气体运动(
... ;
淡水挺水生态系统温室气体排放的主要途径及影响因素研究进展. 生态学报,
2015
5
2000
... 根系不同部位的CH4传输性能被发现存在差异.一方面, 从整个根系系统来看, Henneberg等(2012)在对灯心草(Juncus effusus)的研究中发现, CH4主要通过侧根进入植物体.这可能是由于侧根是植物根际物质交换的主要部位(
... 除了活体植物外, 受损伤的、枯死的植株或部位也是CH4的传输排放通道.当存在枯萎的植株时, 其内部的静态压力比鲜活、完整植株低, 这促使植物内气体多通过鲜活完整的植株向地下输送, 而由受损或枯死植株传输排出(
... 排放(
... 较早的研究发现, CH4在植物内基于液流的传输量很少, 这是因为CH4的传输速率并不依赖于蒸腾速率(
... 湿度是影响植物传输CH4的又一重要环境因子.早期, Armstrong和Armstrong (1991)对芦苇的室内试验发现, 同在24 ℃中, 处于相对湿度为41%环境下植物的对流速率是相对湿度为74%环境下的3.5倍.后期大量研究同样表明, 湿度诱导下的对流作用会随着外界湿度的增加而减弱(
Aspects of methane flow from sediment through emergent cattail (Typha latifolia) plants
2
1998
... 大气中的CH4有70%-80%来自生物源(
... 自然环境下, CH4由于自身沸点低, 主要以气态的方式存在, 其密度在标准状况下为0.717 g·L-1, 要低于近地面大气的密度, 这可能有利于其在植物通气组织内向上扩散传输并排放到大气中.同时CH4作为一种非极性分子, 溶解度低(
Nitrous oxide and methane transport through rice plants
1
1997
... 土壤性质通过影响植物形态结构与生理活动, 进而影响CH4传输, 但是相关研究较少.例如盐分的增加会促进喜旱莲子草(Alternanthera philoxeroides)通气组织的发展, 使根、茎与叶部位胞间隙相连(
植物盐耐机理研究进展
1
2015
... 物种类型是植物传输CH4能力的重要影响因素(
Plant species richness enhanced the methane emission in experimental microcosms
1
2012
... 土壤性质通过影响植物形态结构与生理活动, 进而影响CH4传输, 但是相关研究较少.例如盐分的增加会促进喜旱莲子草(Alternanthera philoxeroides)通气组织的发展, 使根、茎与叶部位胞间隙相连(
Effects of nitrogen on methane production and oxidation and dissolved organic carbon in a freshwater marsh
3
2016
... 大气中的CH4有70%-80%来自生物源(
... 湿地土-水环境中, CH4在产生率不高时一般不会或极少产生气泡, 此时溶解态的CH4主要以液相扩散的方式进行扩散迁移(
... 许多****发现水稻在生长初期的CH4传输排放量较小, 但是随着生长发育不断变化, 一般在抽穗中期或开花期CH4传输排放达到峰值, 之后逐渐减小(
稻田甲烷传输的研究进展
1
2012
... 物种类型是植物传输CH4能力的重要影响因素(
Effects of plant diversity and sand particle size on methane emission and nitrogen removal in microcosms of constructed wetlands
2016
