
Effects of biochar addition on dynamics of soil respiration and temperature sensitivity in a Phyllostachys edulis forest
GEXiao-Gai
通讯作者:
收稿日期:2017-04-10
接受日期:2017-08-29
网络出版日期:2017-11-10
版权声明:2017植物生态学报编辑部本文是遵循CCAL协议的开放存取期刊,引用请务必标明出处。
基金资助:
展开
摘要
关键词:
Abstract
Methods We conducted a two-year biochar addition experiment in a subtropical P. edulis forest from 2014.05 to 2016.04. The study site is located in the Miaoshanwu Nature Reserve in Fuyang district of Hangzhou, Zhejiang Province, in southern China. The biochar addition treatments included: control (CK, no biochar addition), low rate of biochar addition (LB, 5 t·hm-2), medium rate of biochar addition (MB, 10 t·hm-2), and high rate of biochar addition (HB, 20 t·hm-2). Soil respiration was measured by using a LI-8100 soil CO2 efflux system.
Important findings Soil respiration was significantly reduced by biochar addition, and exhibited an apparent seasonal pattern, with the maximum occurring in June or July (except LB in one of the replicated stand) and the minimum in January or February. There were significant differences in soil respiration between the CK and the treatments. Annual mean soil respiration rate in the CK, LB, MB and HB were 3.32, 2.66, 3.04 and 3.24 μmol·m-2·s-1, respectively. Compared with CK, soil respiration rate was 2.33%-54.72% lower in the LB, 1.28%-44.21% lower in the MB, and 0.09%-39.22% lower in the HB. The soil moisture content was increased by 0.97%-75.58% in LB, 0.87%-48.18% in MB, and 0.68%-74.73% in HB, respectively, compared with CK. Soil respiration exhibited a significant exponential relationship with soil temperature and a significant linear relationship with combination of soil temperature and moisture at the depth of 5 cm; no significant relationship was found between soil respiration and soil moisture alone. The temperature sensitivity (Q10) value was reduced in LB and HB. Annual accumulative soil carbon emission in the LB, MB and HB was reduced by 7.98%-35.09%, 1.48%-20.63%, and -4.71%-7.68%, respectively. Biochar addition significantly reduced soil carbon emission and soil temperature sensitivity, highlighting its role in mitigating climate change.
Keywords:
-->0
PDF (1343KB)元数据多维度评价相关文章收藏文章
本文引用格式导出EndNoteRisBibtex收藏本文-->
大气中温室气体CO2浓度升高导致的全球变暖及一系列其他生态问题已引起世界各国的高度关注, 成为科学研究领域的热点问题(Marland & Marland, 2003; 徐涌, 2012; Mitchell et al., 2015)。森林作为陆地生态系统的主体, 在减缓大气CO2浓度升高调节全球碳平衡方面有着重要作用; 在森林生态系统中, 生物质炭是森林火干扰的主要产物, 影响土壤物理、化学和生物过程, 在多数森林生态系统中很普遍, 但生物质炭的生物功能在森林生态系统中却被忽略(DeLuca et al., 2006)。有研究证实, 生物质炭具有高度稳定性和较强的吸附性能, 因而可长期存在于环境中, 在稳定土壤有机碳库、增加土壤碳库容量、减缓土壤碳释放方面有重要意义(Lal et al., 2007), 在应对全球气候变化及环境系统中发挥着重要作用(Sohi et al., 2010)。目前, 生物质炭添加因能增加植物生产力和作物产量, 在农业系统中广泛应用, 而在林业上的应用关注较少(Mitchell et al., 2015)。然而, 森林土壤中添加生物质炭亦能增加森林生产力(Thomas & Gale, 2015), 更重要的是, 生物质炭有潜在的碳吸存能力并能减缓土壤CO2排放。因此, 生物质炭在林业上的应用研究很有必要, 添加生物质炭对森林土壤碳矿化、吸存及土壤物理化学性质的影响将日益受到关注。
研究表明生物质炭添加明显影响原位土壤有机碳矿化(Herath et al., 2015)。土壤呼吸速率是研究土壤碳矿化的重要指标, 是陆地生态系统碳循环的重要过程, 全球年平均土壤呼吸通量为91 Pg·a-1 (1965-2012年为87-95 Pg·a-1)(Hashimoto et al., 2015), 因此, 土壤呼吸的强弱在很大程度上决定了全球气候变化与碳循环间的反馈关系(葛晓改等, 2016)。生物质炭对原位土壤有机碳排放的影响存在不确定性(He et al., 2016): 一方面, 生物质炭添加促进原位土壤碳矿化(正激发效应), 这可能诱发土壤理化性质的改变或生物质炭自身碳矿化(Wardle et al., 2008)或为微生物提供养分而增加微生物活性; 另一方面, 生物质炭添加抑制原位土壤碳矿化(负激发效应), 生物质炭表面吸附原位土壤易溶物或促进土壤团聚体及原位土壤有机碳的稳定性(Herath et al., 2015)。研究表明生物质炭的正激发效应随着时间会降低, 甚至转化成负激发效应(Zimmerman et al., 2011)。目前, 生物质炭添加实验大多基于室内培养或短期的野外试验研究, 培养试验过程通常是在最优化状态下进行, 如常温状态和田间持水量在60%-70%的情况不能代表野外干湿状态下真实的土壤呼吸(Yuste et al., 2007); 生物质炭添加增加土壤水分含量且明显影响土壤呼吸的温度敏感性(Q10)(He et al., 2016)。因此, 有必要开展野外生物质炭添加对土壤呼吸激发效应的大小、持久性和温度敏感性的相关研究。
竹林是我国重要的森林资源类型, 中国现有竹林面积约601万hm2, 且具有生长速度快、隔年连续采伐和可长期利用等特点, 每年有大量的新笋成竹向周围扩展, 因而竹林固定CO2的潜力巨大, 对吸存大气中的CO2具有重要作用。然而, 中国每年竹材砍伐量为800-900万t, 相当于1 000余万m3木材, 但在竹材被砍伐加工利用的同时, 38%-84%的生物质废弃物在燃烧过程中被释放(Hughes et al., 1999)。如果地上竹材加工和砍伐的副产品炭化成生物质炭并应用到土壤, 这些废弃物中50%以上的碳将以高度稳定的形式被吸存在土壤中(Lehmann et al., 2006), 对全球碳循环和缓解全球气候变化具有重要意义(Forbes et al., 2006)。本研究以浙江省广泛分布的毛竹(Phyllostachys edulis)林为研究对象, 通过生物质炭添加试验研究不同毛竹林分2年的土壤呼吸月动态及其温度、水分含量响应, 以探讨和验证生物质炭添加对森林土壤呼吸激发效应的方向、大小、持久性及其温度敏感性, 为竹林土壤碳排放的激发效应研究的系统性、完整性和深入性奠定科学基础, 对生物质炭输入的变化规律、稳定机理及其与土壤相互作用的深入研究有重要意义。
1 材料和方法
1.1 试验地区概况
研究区域位于浙江省杭州市富阳区国家林业局钱江源森林生态系统定位研究站庙山坞林区, 地理位置为119.93°-120.03° E, 30.05°-30.10° N。庙山坞自然保护林区面积约536.7 hm2, 核心区面积348.8 hm2, 海拔11-536 m。保护区内土壤类型有红壤、黄红壤、幼红壤, 红壤面积约占保护区面积的61%, 土壤pH值平均为5.72。研究区具有典型的亚热带季风气候特征, 年平均气温为16.9 ℃, 最热月平均气温25.4 ℃, 最冷月平均气温4.5 ℃ (周本智, 2006)。夏春秋冬, 雨量依次减少, 平均年降水量为1 513 mm。年日照时间达1 995 h, 全年无霜期达237天。研究区地带性植被为常绿阔叶林, 由于过去用材和薪炭的需求, 以及农业活动的发展, 地带性植被已被破坏, 现在主要植被类型包括毛竹林、杉木(Cunninghamia lanceolata)林、马尾松(Pinus massoniana)林、天然次生林、灌木林等, 其中人工毛竹林占总面积的30%。
1.2 样地布置与土壤呼吸测定
2014年4月, 随机选择立地条件相似的毛竹林4块, 每块林分随机各设置3个20 m × 30 m的样地, 每个样地中随机设置4个1 m ×1 m的小样方, 用于生物质炭添加处理, 每处理3次重复。为防止相互干扰, 小样方间至少相隔10 m。根据国内外生物质炭添加试验研究的强度和竹林经营实况(Tammeorg et al., 2014), 生物质炭添加试验分4个处理, 分别为对照(CK)、低生物质炭(LB, 5 t·hm-2)、中生物质炭(MB, 10 t·hm-2)和高生物质炭处理(HB, 20 t·hm-2)。样地基本概况见表1。Table 1
表1
表1研究林分特征(平均值±标准偏差)
Table 1General characteristics of the forest stands studied ((mean ± SD)
林分 Stand | 海拔 Elevation (m) | 树高 Tree height (m) | 胸径 DBH (cm) | 坡度 Slope (°) | 坡向 Aspect | 凋落物层厚度 LLD (cm) | 土壤有机碳 SOC (g·kg-1) | 总氮 TN (g·kg-1) | 总磷 TP (g·kg-1) |
---|---|---|---|---|---|---|---|---|---|
1 | 141 | 12.8 | 10.2 | 10 | S | 2.04 | 33.10 ± 9.06 | 2.86 ± 0.79 | 0.56 ± 0.93 |
2 | 115 | 13.0 | 10.3 | 15 | S | 2.22 | 30.1 ± 8.04 | 2.59 ± 0.48 | 0.37 ± 1.03 |
3 | 86 | 13.3 | 10.7 | 20 | S | 2.47 | 28.8 ± 8.21 | 2.38 ± 0.29 | 0.43 ± 1.09 |
4 | 157 | 12.2 | 10.1 | 20 | S | 2.60 | 34.1 ± 8.04 | 2.59 ± 0.29 | 0.72 ± 1.09 |
新窗口打开
生物质炭添加采用平铺法。在上述试验区样地外选择环境条件和土壤状况与样地相似的毛竹林, 挖取0-15 cm深的土壤约100 kg (土壤容重为1.04 g·kg-1), 剔除石砾等杂质, 风干过筛后混匀约60 kg, 分成4份, 每份约15 kg。4份土壤与生物质炭分别按0、0.32%、0.64%和1.28%混合备用(分别对应上述对照、低生物质炭、中生物质炭和高生物质炭处理), 以防生物质炭流失。在选定的样方中分别添加上述对应的混合土(约1 kg·m-2)。同时测定风干土有机碳含量, 每林分3次重复。供试生物质炭是在450 ℃条件下炭化所得, 炭粒直径在1-2 mm间(分别过1 mm和2 mm筛)。
2014年4月, 分别在设定的毛竹林样地各处理小样方内随机布设3个聚氯乙烯(PVC)土壤环(内径20 cm, 高10 cm, 埋入土壤7-8 cm)。土壤呼吸用LI-8100便携式土壤碳通量测定系统(LI-COR, Nebraska, USA)进行测定, 用其自带的土壤温度、水分探头测5 cm深度的土壤温度和湿度(土壤体积含水量)。从2014年5月至2016年4月, 于每月中旬选择晴朗天气测定。
1.3 数据处理
土壤呼吸速率(Rs, μmol·m-2·s-1)与土壤温度的关系拟合采用如下模型:${{R}_{s}}={{a}_{1}}{{\text{e}}^{{{a}_{2}}T}}$
式中: T为5 cm深度土壤温度(℃), a1是温度为0 ℃的土壤呼吸速率, a2为土壤呼吸的温度反应系数。
土壤呼吸的温度敏感性(Q10)采用如下模型:
${{Q}_{10}}={{\text{e}}^{10{{a}_{2}}}}$
式中: a2为温度反应系数。
土壤呼吸与土壤湿度的关系采用如下模型(Saiz et al., 2006):
${{R}_{s}}={{b}_{1}}+{{b}_{2}}\times W+{{b}_{3}}\times {{W}^{2}}$
式中: W为土壤湿度(土壤体积含水量%), b1、b2、b3为方程拟合参数。
土壤呼吸与土壤温度和湿度的关系, 采用如下模型(Saiz et al., 2006):
${{R}_{s}}=({{c}_{1}}{{\text{e}}^{{{c}_{2}}T}})({{c}_{3}}W+{{c}_{4}}{{W}^{2}})$
式中: T为5 cm深度土壤温度(℃), W是5 cm深度土壤湿度(%), c1、c2、c3、c4为方程拟合参数。
土壤呼吸累积量(Rc, kg·m-2·a-1)计算根据如下等式:
${{R}_{c}}=\sum\limits_{i-1}^{12}{{{R}_{\text{si}}}\times {{t}_{i}}\times {{10}^{-9}}\times 44}$
式中: Rsi为各个月呼吸速率平均值(μmol·m-2·s-1), ti为各个月时间(s), 44为CO2摩尔质量(g·mol-1)。
利用Excel 2007和SPSS 16.0软件进行统计分析和作图, 生物质炭添加处理下土壤呼吸速率和土壤呼吸年累积量之间的差异采用单因子方差分析和最小显著差异法(LSD)分析, 显著性水平设定为α = 0.05; 土壤呼吸速率与土壤温度、土壤湿度关系采用回归分析。
2 结果
2.1 生物质炭添加对土壤呼吸季节动态的影响
生物质炭添加和对照中土壤呼吸均呈明显的季节变化和单峰模式, 土壤呼吸速率均在6-7月最高(只有林分1的LB处理是8月份最高), 1月或2月最低(图1)。生物质炭添加显著降低了年平均土壤呼吸速率(林分3的HB处理除外)(p < 0.01), 毛竹林CK、LB、MB和HB处理的年平均土壤呼吸速率分别为(3.32 ± 0.16)、(2.66 ± 0.45)、(3.04 ± 0.18)和(3.24 ± 0.19) μmol·m-2·s-1, 即随着添加量的增加土壤呼吸降低量变小。林分1到林分3中年平均土壤呼吸速率均是CK最高, HB、MB次之, LB最低; 林分3的年平均土壤呼吸速率则是HB最高, CK、MB次之, LB最低。与对照相比, LB、MB、HB处理下土壤呼吸速率分别降低2.33%-54.72%、1.28%-44.21%和0.09%-39.22%。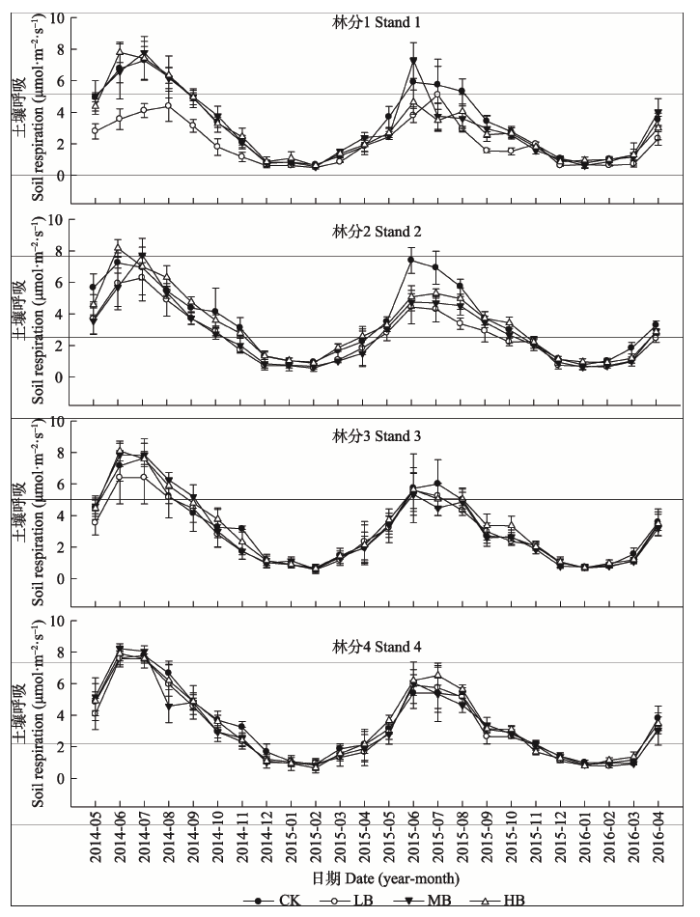
图1生物质炭添加下毛竹林土壤呼吸月动态(平均值±标准偏差)。CK, 对照; LB, 低生物质炭施加(5 t·hm-2); HB, 高生物质炭施加(20 t·hm-2); MB, 中生物质炭施加(10 t·hm-2)。
-->Fig. 1Monthly dynamics of soil respiration in Phyllostachys edulis forest stands with different biochar addition treatments (mean ± SD). CK, control; LB, low rate of biochar addition (5 t·hm-2); HB, high rate of biochar addition (20 t·hm-2); MB, medium rate of biochar addition (10 t·hm-2).
-->
生物质炭添加对生长季(4-10月)和非生长季(11月到次年3月)土壤呼吸影响显著(p < 0.01)(图2; 表2), 生物质炭添加处理与季节的交互影响仅林分1显著(p < 0.01)(表2)。林分1到林分3中LB处理均明显降低生长季土壤呼吸(p < 0.01)。与对照相比, 林分1中生长季的LB、MB、HB分别降低37.02%、3.06%和9.69%, 林分2中分别降低25.70%、19.11%和5.52%, 林分3中LB处理降低7.25%, MB和HB处理分别增加0.79%和5.73%; 林分4中LB、MB分别降低6.19%和4.09%, HB处理增加3.59%。非生长季中所有林分的LB处理、林分2和3中MB处理、林分4中HB处理较对照显著降低(p < 0.01)(图2), 1-4林分中LB较对照分别降低27.69%、32.42%、17.95%和18.68%, 林分2和3中MB处理较对照分别降低28.77%和17.78%, 林分4中HB处理比对照降低16.22%。
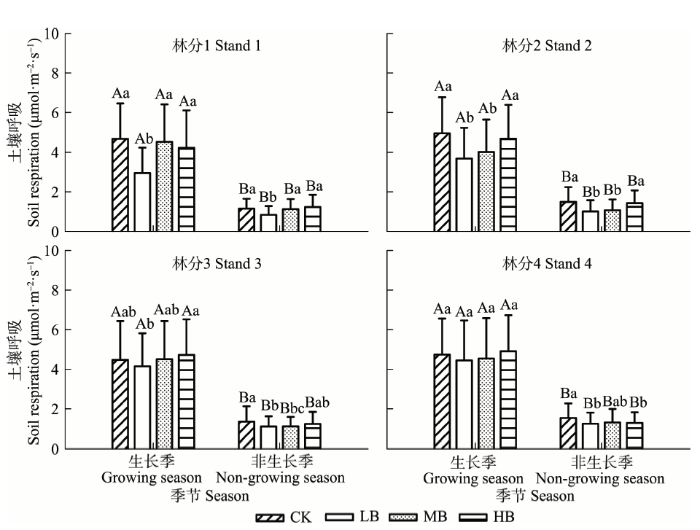
图2生物质炭添加下毛竹林生长季和非生长季土壤呼吸特征(平均值±标准偏差)。不同小写字母表示相同季节不同生物质炭处理间差异显著; 不同大写字母表示同一生物质炭处理不同季节差异显著。CK, 对照; LB, 低生物质炭施加(5 t·hm-2); HB, 高生物质炭施加(20 t·hm-2); MB, 中生物质炭施加(10 t·hm-2)。
-->Fig. 2The characteristics of soil respiration in growing and non-growing seasons in Phyllostachys edulis forest stands with different biochar treatments (mean ± SD). Different lowercase letters indicate significant differences among biochar treatments within seasons, and different capital letters indicate significant differences among seasons within biochar treatments. CK, control; LB, low rate of biochar addition (5 t·hm-2); HB, high rate of biochar addition (20 t·hm-2); MB, medium rate of biochar addition (10 t·hm-2).
-->
Table 2
表2
表2生物质炭添加和季节对毛竹林土壤呼吸影响的双因子方差分析
Table 2Two-way ANOVA for the effects of biochar addition treatments and seasons on soil respiration in Phyllostachys edulis forest stands
林分 Stands | 季节 Season | 生物质炭添加 Biochar addition | 季节×生物质炭添加 Season × biochar addition | |||
---|---|---|---|---|---|---|
F | p | F | p | F | p | |
林分1 Stand 1 | 673.41 | 0.00 | 16.66 | 0.00 | 7.64 | 0.00 |
林分2 Stand 2 | 731.47 | 0.00 | 13.36 | 0.00 | 2.30 | 0.08 |
林分3 Stand 3 | 695.20 | 0.00 | 1.54 | 0.204 | 0.77 | 0.51 |
林分4 Stand 4 | 655.78 | 0.00 | 1.14 | 0.334 | 0.64 | 0.60 |
新窗口打开
2.2 生物质炭添加对毛竹林土壤温度、水分的影响
生物质炭添加对土壤水分影响显著, 尤其是HB处理(图3)。在试验的24个月中, LB处理中, 林分1中仅有3个月水分含量增加(0.97%-17.39%), 林分2中14个月水分含量增加(1.65%-75.58%), 林分3中12个月水分含量增加(0.87%-18.58%), 林分4中有15个月水分含量增加(0.62%-41.49%); MB处理中, 林分1到林分4中分别有5个月(3.65%-16.43%)、18个月(1.59%-48.18%)、13个月(0.87%-18.58%)、9个月(1.11%-36.34%)水分含量增加; HB处理中, 林分1到林分4中分别有13个月(0.89%-21.18%)、21个月(2.33%-50.40%)、17个月(0.86%-74.73%)和17个月(0.68%-39.24%)水分含量增加。生物质炭添加对毛竹林土壤温度影响不明显(图4); 最高土壤温度均是在2015年7月, 最低土壤温度是在2015年2月。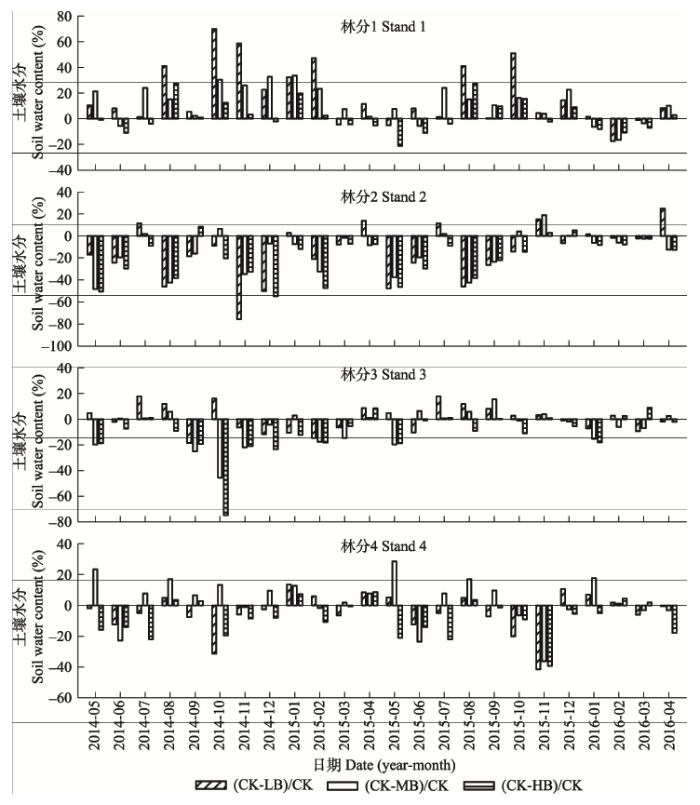
图3生物质炭添加对毛竹林土壤水分含量月动态的影响。(CK-LB)/CK、(CK-MB)/CK、(CK-HB)/CK分别表示低、中、高生物质炭添加处理下土壤水分含量较对照降低(负的表示增加)的百分比。缩写同
-->Fig. 3The effects of biochar addition on monthly soil moisture in Phyllostachys edulis forest stands. The Y-axis means the percent of soil moisture difference by biochar addition to the control. (CK-LB)/CK, (CK-MB)/CK, (CK-HB)/CK mean the decreased (minus value indicates a increase) percent of soil moisture on LB, MB and HB treatment, compared with control treatment. The abbreviations are the same as in
-->
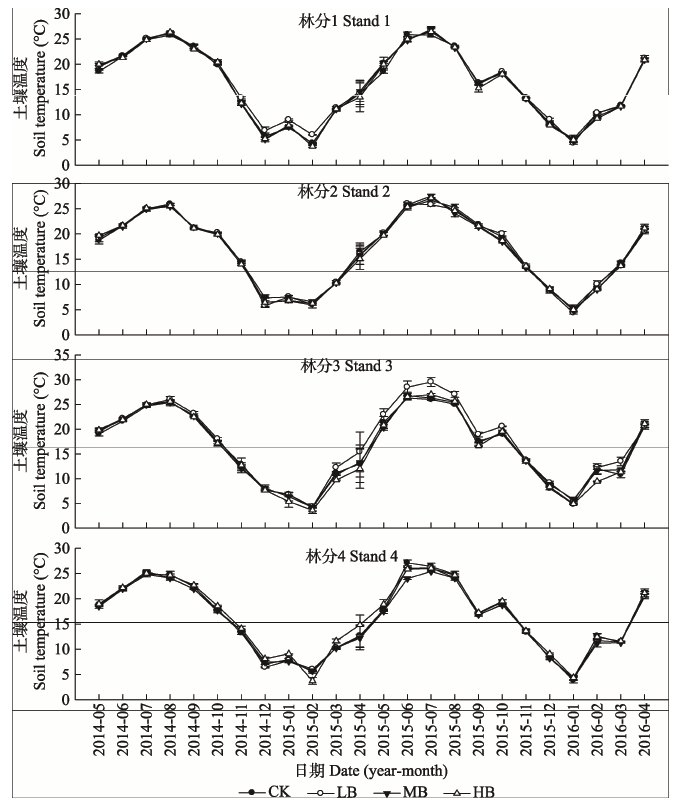
图4生物质炭添加下毛竹林土壤温度月动态特征。CK, 对照; LB, 低生物质炭施加(5 t·hm-2); HB, 高生物质炭施加(20 t·hm-2); MB, 中生物质炭施加(10 t·hm-2)。
-->Fig. 4The characteristics of monthly soil temperaturein Phyllostachys edulis forest stands with different biochar addition treatments. CK, control; LB, low rate of biochar addition (5 t·hm-2); HB, high rate of biochar addition (20 t·hm-2); MB, medium rate of biochar addition (10 t·hm-2).
-->
2.3 生物质炭添加下土壤呼吸与土壤温度和水分的关系
生物质炭添加下各林分土壤呼吸均与土壤温度呈显著正相关关系(图5)。基于土壤温度的非线性模型拟合发现, 与对照相比, 林分1到林分2中生物质炭添加处理后土壤温度对土壤呼吸的解释量(R2)均降低, 林分3和林分4 (除MB处理)中温度对土壤呼吸变量的解释量(R2)则增加。生物质炭添加处理改变土壤呼吸的温度敏感性, 与对照相比, 林分1中生物质炭添加处理Q10均降低, 林分2仅HB处理Q10降低, 林分3中仅LB处理Q10降低, 林分4中生物质炭添加处理Q10均增加。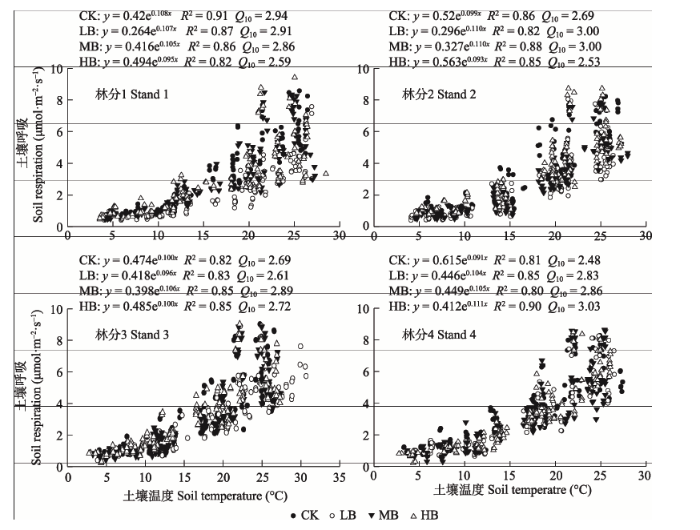
图5生物质炭添加下毛竹林土壤呼吸速率和土壤温度的关系。CK, 对照; LB, 低生物质炭施加(5 t·hm-2); HB, 高生物质炭施加(20 t·hm-2); MB, 中生物质炭施加(10 t·hm-2)。Q10, 土壤呼吸温度敏感性系数。
-->Fig. 5Relationships between soil respiration and soil temperature in Phyllostachys edulis forest stands with different biochar addition treatments. CK, control; LB, low rate of biochar addition (5 t·hm-2); HB, high rate of biochar addition (20 t·hm-2); MB, medium rate of biochar addition (10 t·hm-2). Q10, soil temperature sensitivity.
-->
生物质炭添加处理后, 利用线性模型拟合发现土壤呼吸与土壤水分含量没有显著相关关系, 但土壤呼吸与温度、水分交互呈显著的非线性相关关系(p < 0.01, 表3)。与对照相比, 林分1和林分2 (除LB处理)中生物质炭添加处理的R2值均降低, 林分3 (除LB处理)和林分4 (除MB处理)的R2值均增加, CK处理的土壤温度、水分共同解释土壤呼吸变量的72.3%-80.2%, LB处理解释变量的68.6%-79.5%, MB处理解释变量的64.9%-75.6%, HB处理解释变量的64.9%-85.8%。
Table 3
表3
表3生物质炭添加下毛竹林土壤呼吸速率(y)与土壤温度(x)、水分(W)关系的拟合方程
Table 3Regression models of soil respiration (y) with soil temperature (x) and moisture (W) in Phyllostachys edulis forest stands with different biochar addition treatments
林分 Stand | CK | LB | ||||
---|---|---|---|---|---|---|
拟合方程 Fitted equation | R2 | p | 拟合方程 Fitted equation | R2 | p | |
1 | y = 0.025e0.095x(2.609W - 0.066W2) | 0.80 | ** | y = 0.067e0.097x(0.523W - 0.012W2) | 0.79 | ** |
2 | y = 0.029e0.088x(2.372W - 0.056W2) | 0.76 | ** | y = 0.112e0.093x(0.437W - 0.010W2) | 0.69 | ** |
3 | y = 0.018e0.085x(3.968W - 0.096W2) | 0.72 | ** | y = 0.098e0.072x(0.819W - 0.020W2) | 0.72 | ** |
4 | y = 0.030e0.077x(2.828W - 0.060W2) | 0.73 | ** | y = 0.021e0.093x(0.387W- 0.068W2) | 0.80 | ** |
林分 Stand | MB | HB | ||||
拟合方程 Fitted equation | R2 | p | 拟合方程 Fitted equation | R2 | p | |
1 | y = 0.080e0.086x(0.653W - 0.012W2) | 0.65 | ** | y = 0.091e0.083x(0.510W - 0.008W2) | 0.65 | ** |
2 | y = 0.024e0.091x(1.776W - 0.035W2) | 0.76 | ** | y = 0.096e0.078x(0.769W - 0.017W2) | 0.71 | ** |
3 | y = 0.009e0.086x(6.901W - 0.157W2) | 0.73 | ** | y = 0.032e0.079x(2.237W - 0.049W2) | 0.74 | ** |
4 | y = 0.062e0.090x(1.059W - 0.025W2) | 0.69 | ** | y = 0.015e0.095x(3.825W - 0.087W2) | 0.86 | ** |
新窗口打开
年平均土壤呼吸的温度敏感性与土壤温度、土壤含水量的相关关系不显著(p > 0.05, 图6), 表明土壤水分含量增加对温度敏感性Q10的变化影响较小。
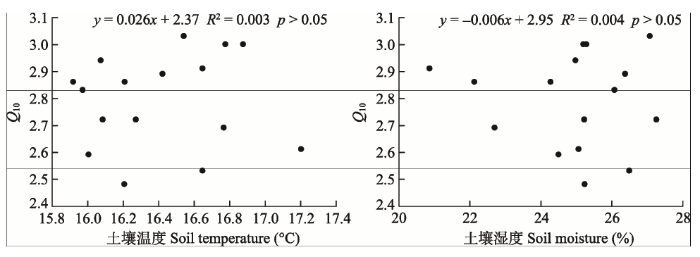
图6生物质炭添加下毛竹林土壤呼吸温度敏感性系数(Q10)与土壤温度、土壤水分的关系。
-->Fig. 6Relationships of soil temperature sensitivity (Q10) with soil temperature and moisture in Phyllostachys edulis forest stands with biochar addition.
-->
2.4 生物质炭添加对土壤呼吸年累积碳排放的影响
生物质炭添加处理降低土壤呼吸年总量(林分3中HB处理除外)(图7)。各林分土壤呼吸年总量均是LB处理最低, CK处理最高(林分3中HB处理除外)。林分1中CK中土壤呼吸年总量是4.47 kg·m-2·a-1, LB是2.88 kg·m-2·a-1, MB是4.33 kg·m-2·a-1, HB是4.14 kg·m-2·a-1, 与对照相比, LB、MB和HB分别降低35.09%、3.07%和7.68%; 林分2中, LB (3.58 kg·m-2·a-1) < MB (3.87 kg·m-2·a-1) < HB (4.63 kg·m-2·a-1) < CK (4.89 kg·m-2·a-1), LB、MB和HB处理的土壤呼吸年总量分别降低27.00%、20.63%和5.53%; 林分3中LB (4.01 kg·m-2·a-1) < MB (4.31 kg·m-2·a-1) < CK (4.42 kg·m-2·a-1) < HB (4.56 kg·m-2·a-1), 且与对照相比, LB和MB处理分别降低8.34%、1.48%, HB处理则增加4.71%; 林分4中LB (4.34 kg·m-2·a-1) < MB (4.46 kg·m-2·a-1) < HB (4.74 kg·m-2·a-1) < CK (4.75 kg·m-2·a-1), 与对照相比, LB、MB处理的土壤呼吸年总量分别降低7.98%、5.77%, HB则增加0.86%。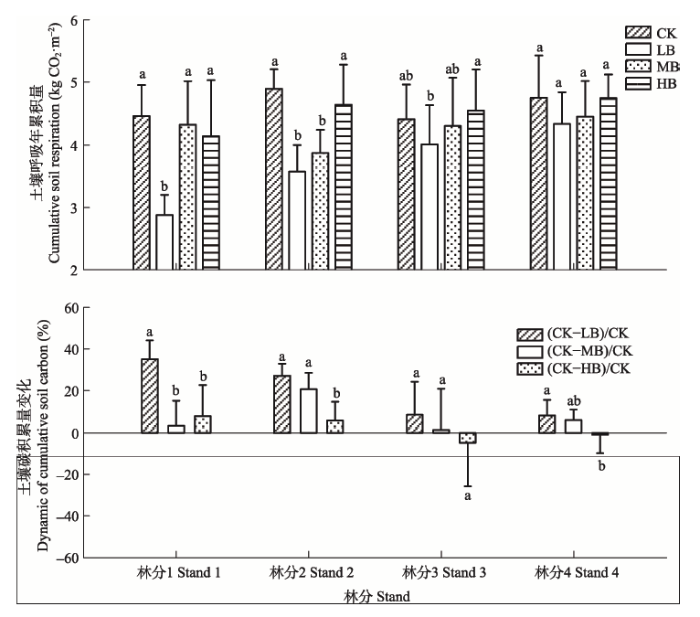
图7生物质炭添加对毛竹林土壤碳年累积排放的影响 (平均值±标准偏差)。不同小写字母表示相同林分不同处理间差异显著。CK, 对照; LB, 低生物质炭施加(5 t·hm-2); MB, 中生物质炭施加(10 t·hm-2); HB, 高生物质炭施加(20 t·hm-2); (CK-LB)/CK、(CK-MB)/CK、(CK-HB)/CK分别表示低、中、高生物质炭添加处理中土壤碳年累积排放量较对照降低的比重。
-->Fig. 7The effects of biochar addition on annual cumulative soil respiration in Phyllostachys edulis forest stands (mean ± SD). Different lowercase letters indicate significant differences among biochar treatments within forest stands. CK, control; LB, low rate of biochar addition (5 t·hm-2); MB, medium rate of biochar addition (10 t·hm-2); HB, high rate of biochar addition (20 t·hm-2). (CK-LB)/CK, (CK-MB)/CK, (CK-HB)/CK mean the decrease percent of annual cumulative soil respiration on LB, MB and HB treatment, compared with control treatment.
-->
3 讨论
3.1 生物质炭添加对毛竹林土壤呼吸激发效应和持效性的影响
土壤激发效应是指各种有机质添加等处理引起土壤有机质周转强烈的短期改变(Kuzyakov et al., 2000), 土壤中添加生物质炭后激发原位土壤碳排放增加(正激发效应)或激发原位土壤碳排放降低(负激发效应)。生物质炭添加对土壤的激发效应因土壤类型和养分的不同对土壤呼吸的影响存在着诸多争议, 主要包括促进(Luo et al., 2011)、抑制(Jones et al., 2011; Zimmerman et al., 2011)和无影响(Liu et al., 2016)。生物质炭添加后土壤碳矿化较对照明显增加, 这是因为生物质炭快速利用生物质炭自身的易溶碳组分, 没有激发原位土壤有机质的碳排放(Cross & Sohi, 2011); 降低原位土壤碳矿化可能是因为增强了原位土壤易溶碳库的稳定性。本研究发现生物质炭添加明显降低毛竹林土壤呼吸速率, 与对照相比, LB处理降低2.33%-54.72%, MB处理降低1.28%- 44.2%, HB处理则是降低了0.09%-39.22% (图1), 均呈现负激发效应, 这与Novak等(2010)和Spokas等(2010)的研究结果一致。生物质炭添加降低毛竹林土壤呼吸的原因可能是生物质炭表面孔隙结构吸收了原位土壤有机质、促进原位土壤团聚体的稳定性, 同时土壤微生物因适应环境而发生变化(Herath et al., 2015); 低生物质炭添加因环境改变降低土壤碳矿化明显, 高生物质炭添加处理下微生物快速利用生物质炭自身易溶养分而促进碳矿化, 同时弥补因适应环境而降低的碳矿化, 致使添加剂量与土壤呼吸降低程度呈非线性关系(图7), 也可能因为非生物因素(如降水)或生物质炭添加增加水分而使土壤中碳酸盐含量增加(Bruun et al., 2014)。总之, 一方面适宜的温度和水分能促进微生物生长和碳排放, 另一方面土壤中添加生物质炭的激发方向和程度随着土壤和生物炭类型而发生变化(Major et al., 2010)。生物质炭添加对土壤呼吸速率的季节变化影响不同(图2; 表2)。本研究中生物质炭添加下土壤呼吸速率均呈单峰模式, 土壤呼吸速率均在6-7月最高(除林分1中LB处理是8月份最高外), 1月或2月最低; 生物质炭添加对生长季土壤呼吸速率的影响较非生长季明显, 主要是因为生长季水分适宜(雨季), 土壤温度相对适合微生物活性、根系生长和植物的发育阶段(吴君君等, 2014); 生长季温度相对较高时, 根呼吸和微生物呼吸相对旺盛, 生物质炭添加增加微生物活性、微生物丰度和根系生物量(Mitchell et al., 2015); 在非生长季当温度较低时, 土壤呼吸速率主要受生化反应限制, 生物质炭添加对根系呼吸和微生物活性影响相对较小, 因此, 生物质炭添加处理下非生长季碳排放对年碳排放的贡献相对较小。
生物质炭添加对毛竹林土壤呼吸速率的后续影响与添加剂量相关。研究表明生物质炭添加到土壤后, 自身碳矿化随着时间逐渐降低(Ameloot et al., 2013); 生物质炭添加的起始阶段, 2%-20%的生物质炭自身碳在2-60天矿化, 随后矿化率降低。如Major等(2010)研究表明生物质炭添加对土壤呼吸第一年的影响较第二年大, 生物质炭自身易溶碳矿化75%集中在第一年且随着时间的延长逐渐降低。本研究中低生物质炭添加处理降低土壤呼吸的程度随着时间的延长略微降低, 中、高生物质炭添加处理降低土壤呼吸程度随时间的延长略微增加, 这可能是因为土壤微生物与生物质炭自身易溶养分相互作用或适应环境程度与生物质炭添加剂量有关。因此, 生物质炭与土壤互作对土壤碳矿化持久性的影响因素需要进一步深入研究。
3.2 生物质炭添加对土壤温度、水分含量及温度敏感性的影响
土壤温度、土壤水分是影响土壤CO2通量的主导因子, 它们通过对凋落物分解过程、微生物活性、根系生长及光合作用调节而影响土壤呼吸速率(Lellei-Kovács et al., 2016)。本研究中, 生物质炭添加增加土壤水分含量(图3), 可能是因为生物质炭添加通过增加田间持水量孔隙(30 μm)和永久萎蔫点间孔隙(0.2 μm)的比重而增加土壤有效水分(Hardie et al., 2014)。对照和生物质炭处理下土壤呼吸与温度呈显著的指数函数关系(图5), 因为土壤温度通过影响微生物呼吸、根系生长和养分矿化等影响土壤呼吸; 土壤呼吸与水分含量均无显著相关关系, 可能是因为毛竹林土壤含水量相对适宜, 土壤含水量不是土壤呼吸的限制因子, 高浓度生物质炭添加虽增加土壤水分含量但对土壤呼吸的解释量较小(He et al., 2016)。土壤呼吸速率与温度、水分加和呈显著相关关系(表3), 说明土壤水分通过土壤温度间接影响土壤呼吸速率; 生物质炭添加明显增加了土壤水分含量, 尤其是增加土壤有效水分容量的0.3%-1.3%时, 生物质炭添加增加的土壤水分含量引起传导性和电解质损失而形成一个相对高温的环境, 间接影响土壤呼吸速率(Ulyett et al., 2014)。温度和水分等环境因子通过土壤生物和底物间接影响土壤呼吸的温度敏感性(杨庆朋等, 2011), 通常土壤含水量增加会提高土壤呼吸的温度敏感性, 在一定范围内, 随着土壤含水量的升高, 土壤呼吸对温度的响应会更为剧烈(Almagro et al., 2009)。本研究中生物质炭添加增加土壤水分含量和改变土壤呼吸底物等引起土壤呼吸的温度敏感性变化(图5), 这可能是因为: 1)环境因子变化, 生物质炭添加后土壤水分含量增加直接影响温度敏感性; 2)土壤呼吸底物变化, 生物质炭自身养分含量或生物质炭表面物质抑制原位土壤有机碳和根系分解, 或生物质炭添加引起温度变化(He et al., 2016); 3)土壤根系(生物)变化, 生物质炭添加使土壤根系增加, 而根呼吸是土壤呼吸的主要组成部分且具有较高的温度敏感性(Saiz et al., 2006)。He等(2016)研究表明生物质炭自身孔隙吸收CO2, 生物质炭添加降低土壤温度变动, 在一定程度上解释土壤温度敏感性变化。Suseela等(2012)研究表明春、秋季Q10与平均年土壤水分和平均年土壤温度呈正相关关系; 夏季Q10与土壤温度和水分不相关。本研究中, Q10与土壤平均年温度和水分不相关(图6), 可能是因为土壤水分含量变化没有超出毛竹林温度敏感性响应的阈值(刘殿军等, 2016); 当土壤水分含量低于0.11 m3·m-3或大于田间持水量时, Q10降低, 土壤水分胁迫影响土壤温度敏感性(Jassal et al., 2008)。总之, 土壤含水量相对适宜的时候温度敏感性最高, 而土壤温度敏感性在含水量较低或较高时都会发生变化, 变化方向和幅度与土壤类型紧密相关(杨庆朋等, 2011)。
3.3 生物质炭添加对土壤碳排放的影响
生物质炭对土壤CO2排放(土壤呼吸)的影响因素包括微生物的生物降解和环境的非有机碳部分的非生物因素(Jones et al., 2011); 生物质炭添加能改变土壤物理化学性质和微生物繁殖的环境, 并影响土壤微生物活性和群落结构(Liu et al., 2016)。本研究中生物质炭添加剂量对土壤碳排放的影响显著(图7), 与Chen等(2017)和Bamminger等(2014)的研究吻合, 即生物质炭添加后累积CO2排放与对照相比降低了2%-56%, 且随着添加量的增加而下降, 因为生物质炭添加对土壤碳排放的生物因子影响明显, 尤其生物质炭添加明显增加根际区域面积, 生物质炭自身养分或增加的水分吸引植物根系; 同时可能因其降低根系新陈代谢活动而降低根系呼吸(Prendergast-Miller et al., 2014)。因此, 大规模野外试验之前有必要粗略地评估生物质炭添加效果, 尤其是土壤养分状况、生物质炭类型和森林经营措施(Kammann et al., 2011)。
生物质炭添加对土壤碳排放的影响与非生物因子关系密切, 生物质炭添加的负激发效应与土壤碳酸盐含量紧密相关(Verheijen et al., 2014)。用14C标记的生物质炭研究表明在培养的土壤中碳酸盐含量与底物总CO2排放紧密相关(Bruun et al., 2014)。试验条件也是生物质炭添加影响土壤呼吸的一个重要因素: 在野外, 生物质炭添加增加土壤团聚体的稳定性(Hardie et al., 2014), 有机物质被大团聚体保护, 或被土壤矿化颗粒束缚, 不易被土壤微生物直接利用(Liang et al., 2010)。本研究中野外原位土壤添加生物质炭后土壤通过有机质吸收过程和物理保护提高土壤碳库吸存, 降低土壤碳排放(图7)。有研究表明土壤早期培养阶段的碳释放明显来自碳酸盐; 尽管碳酸盐能解释CO2的非生物释放, 其他的非生物过程如生物质炭表面的非生物氧化也很重要(Bruun et al., 2014)。
土壤呼吸除受到非生物因子影响外, 还受到土壤微生物、植被类型等生物因子的影响。生物质炭添加改变微生物群落, 因为其通过对底物有效养分和pH值的改变而改变微生物群落组成(Fierer et al., 2009)。本研究中, 生物质炭添加明显改变了微生物的群落结构, 中、高生物质炭处理增加了细菌和总微生物群落生物量(未发表)。生物质炭与根系相互作用, 增加根系生物量和根长密度, 植物根系对生物质炭添加因水分含量不同而响应不同(Verheijen et al., 2014)。
The authors have declared that no competing interests exist.
作者声明没有竞争性利益冲突.
参考文献 原文顺序
文献年度倒序
文中引用次数倒序
被引期刊影响因子
[1] | . |
[2] | . SummaryThe stability of biochar in soils is the cornerstone of the burgeoning worldwide interest in the potential of the pyrolysis/biochar platform for carbon (C) sequestration. While biochar is more recalcitrant in soil than the original organic feedstock, an increasing number of studies report greater C-mineralization in soils amended with biochar than in unamended soils. Soil organisms are believed to play a central role in this process. In this review, the variety of interactions that occur between soil micro-, meso- and macroorganisms and biochar stability are assessed. In addition, different factors reported to influence biochar stability, such as biochar physico-chemical characteristics, soil type, soil organic carbon (SOC) content and agricultural management practices are evaluated. A meta-analysis of data in the literature revealed that biochar-C mineralization rates decreased with increasing pyrolysis temperature, biochar-C content and time. Enhanced release of CO2 after biochar addition to soil may result from (i) priming of native SOC pools, (ii) biodegradation of biochar components from direct or indirect stimulation of soil organisms by biochar or (iii) abiotic release of biochar-C (from carbonates or chemi-sorbed CO2). Observed biphasic mineralization rates suggest rapid mineralization of labile biochar compounds by microorganisms, with stable aromatic components decomposed at a slower rate. Comparatively little information is available on the impact of soil fauna on biochar stability in soil, although they may decrease biochar particle size and enhance its dispersion in the soil. Elucidating the impacts of soil fauna directly and indirectly on biochar stability is a top research priority. |
[3] | . SummaryThe success and feasibility of CO2-sequestration through incorporation of biochar into soils depends strongly on the long-term biochar stability and on the improvements of physical and microbial soil properties. In this study, the stability of two maize-derived biochars (from pyrolysis and hydrothermal carbonization) and of a compost-biochar mixture and their effects on microbial biomass and enzyme activity were determined in two soils during a 57-day incubation. Soil samples amended with biochar increased soil organic carbon (SOC) content by 20 or 40%. Samples amended with hydrothermal biochar showed the largest respiration rates and the largest increase in microbial and enzymatic activity compared with the untreated controls. Carbon and 13C mass balances showed that between 13 and 16% of the added hydrochar was mineralized within 8 weeks. In the arable soil, hydrochar additions greatly stimulated the degradation of SOC, thus inducing positive priming effects. The mineralization of pyrogenic biochar (pyrochar and a pyrochar-compost mixture) was significantly less (1.4 3%) and comparable to the SOC mineralization in the control soils. |
[4] | . SummaryThe stability of biochar in soil is of importance if it is to be used for carbon sequestration and long-term improvement of soil properties. It is well known that a significant fraction of biochar is highly stable in soil, but carbon dioxide (CO2) is also released immediately after application. This study investigated the nature of the early release of CO2 and the degree to which stabilizing mechanisms protect biochar from microbial attack. Incubations of 14C-labelled biochar produced at different temperatures were performed in soils with different clay contents and in sterilized and non-sterilized soils. It emerged that carbonate may be concentrated or form during or after biochar production, resulting in significant carbonate contents. If CO2 released from carbonates in short-term experiments is misinterpreted as mineralization of biochar, the impact of this process may be significantly over-estimated. In addition to the CO2 released from carbonates, there appears to be a labile fraction of biochar that is oxidized quickly during the first days of incubation, probably by both abiotic and biotic processes. Later in the incubation, biotic mineralization appears to be the primary cause of CO2 evolution. Finally, we found that both production temperature and clay content affect biochar mineralization. As protective mechanisms hypothesized to prevent degradation of organic matter in soil usually implicate clay, we conclude that biochar is likely to be protected from mineralization during the early stages of incubation by its own recalcitrant chemical and physical nature as well as by physical protective mechanisms. |
[5] | . |
[6] | . Recognition of biochar as a potential tool for long-term carbon sequestration with additional agronomic benefits is growing. However, the functionality of biochar in soil and the response of soils to biochar inputs are poorly understood. It has been suggested, for example, that biochar additions to soils could prime for the loss of native organic carbon, undermining its sequestration potential. This work examines the priming potential of biochar in the context of its own labile fraction and procedures for their assessment. A systematic set of biochar samples produced from C4 plant biomass under a range of pyrolysis process conditions were incubated in a C3 soil at three discrete levels of organic matter status (a result of contrasting long-term land management on a single site). The biochar samples were characterised for labile carbon content ex-situ and then added to each soil. Priming potential was determined by a comparison of CO 2 flux rates and its isotopic analysis for attribution of source. The results conclusively showed that while carbon mineralisation was often higher in biochar amended soil, this was due to rapid utilisation of a small labile component of biochar and that biochar did not prime for the loss of native organic soil organic matter. Furthermore, in some cases negative priming occurred, with lower carbon mineralisation in biochar amended soil, probably as a result of the stabilisation of labile soil carbon. |
[7] | . |
[8] | . Although belowground ecosystems have been studied extensively and soil biota play integral roles in biogeochemical processes, surprisingly we have a limited understanding of global patterns in belowground biomass and community structure. To address this critical gap, we conducted a meta-analysis of published data (> 1300 datapoints) to compare belowground plant, microbial and faunal biomass across seven of the major biomes on Earth. We also assembled data to assess biome-level patterns in belowground microbial community composition. Our analysis suggests that variation in microbial biomass is predictable across biomes, with microbial biomass carbon representing 0.6-1.1% of soil organic carbon (r(2) = 0.91) and 1-20% of total plant biomass carbon (r(2) = 0.42). Approximately 50% of total animal biomass can be found belowground and soil faunal biomass represents < 4% of microbial biomass across all biomes. The structure of belowground microbial communities is also predictable: bacterial community composition and fungal : bacterial gene ratios can be predicted reasonably well from soil pH and soil C : N ratios respectively. Together these results identify robust patterns in the structure of belowground microbial and faunal communities at broad scales which may be explained by universal mechanisms that regulate belowground biota across biomes. |
[9] | . Abstract Black carbon (BC) is ubiquitous in terrestrial environments and its unique physical and chemical properties suggest that it may play an important role in the global carbon budget (GCB). A critical issue is whether the global production of BC results in significant amounts of carbon (C) being removed from the short-term bio-atmospheric carbon cycle and transferred to the long-term geological carbon cycle. Several dozen field and laboratory based studies of BC formation during the burning of biomass have been documented. Findings are difficult to interpret because they have been expressed in an inconsistent manner, and because different physical and chemical methods have been used to derive them. High error terms documented in many of these studies also highlight the problems associated with the quantification of the amount of biomass C consumed in fire, the amount of residue produced and the constituents of that residue. To be able to estimate the potential for BC as a carbon sink, issues regarding its definition, the methods used in its identification and measurement, and the way it is expressed in relation to other components of the carbon cycle need to be addressed. This paper presents BC data in a standard way; BC production as a percentage of the amount of C consumed by fire (BC/CC), which can be readily integrated into a larger carbon budget. Results from previous studies and new data from Australian ecosystems were recalculated in this way. As part of this process, several BC estimates derived solely from physical methods were discarded, based on their inability to accurately identify and quantify the BC component of the post-fire residue. Instead, more focus was placed on BC estimates obtained by chemical methods. This recalculated data lowered the estimate for BC formation in forest fires from 4% to 5% to <3% BC/CC. For savannah and grassland fires a value of <3% is consistent with reported data, but considerable variation among estimates remains. An updated flow-chart linking the sources, fluxes and pools of BC formed in the terrestrial environment with the aquatic and marine environments, and estimates of mean residence times for BC are also presented. |
[10] | . . 生物质炭因其特殊结构分解缓慢而长期固存在土壤中,在稳定有机碳库、增加碳库容量、保持土壤肥力、改变土壤质地方面具有重要作用。同时,随着生物质炭输入土壤团聚体结构、水分渗透性、养分吸附和微生物活性也发生改变,引起原位土壤有机碳周转改变的激发效应。文章综述了生物质炭输入对土壤环境、碳排放、生物质炭自身碳矿化的影响,对生物质炭-土壤互作产生的激发效应持久性、大小、方向和机制进行总结,即生物质炭输入后的正激发效应可能表现为生物质炭中可溶组分与微生物共代谢而促进生物质炭自身碳矿化;生物质炭添加引起的负激发效应可能表现为生物质炭诱发原位土壤有机碳更加稳定或生物质炭中易挥发有机物抑制原位土壤微生物活性而降低土壤碳排放。并根据目前的研究现状,就生物质炭输入量、与土壤微生物群落和植物的相互作用、生物质炭添加的风险预测和评估及开展长期研究的必要性等问题进行展望,以期为生态系统长期碳吸存研究奠定基础,为应对气候变化提供选择和参考。 |
[11] | Aims This study aims to (i) determine the effects of incorporating 4702Mg02ha 611 acacia green waste biochar on soil physical properties and water relations, and (ii) to explore the different mechanisms by which biochar influences soil porosity. Methods The pore size distribution of the biochar was determined by scanning electron microscope and mercury porosimetry. Soil physical properties and water relations were determined by in situ tension infiltrometers, desorption and evaporative flux on intact cores, pressure chamber analysis at 611,50002kPa, and wet aggregate sieving. Results Thirty months after incorporation, biochar application had no significant effect on soil moisture content, drainable porosity between –1.0 and 611002kPa, field capacity, plant available water capacity, the van Genuchten soil water retention parameters, aggregate stability, nor the permanent wilting point. However, the biochar-amended soil had significantly higher near-saturated hydraulic conductivity, soil water content at 610.102kPa, and significantly lower bulk density than the unamended control. Differences were attributed to the formation of large macropores (>1,20002μm) resulting from greater earthworm burrowing in the biochar-amended soil. Conclusion We found no evidence to suggest application of biochar influenced soil porosity by either direct pore contribution, creation of accommodation pores, or improved aggregate stability. |
[12] | . The flux of carbon dioxide from the soil to the atmosphere (soil respiration) is one of the major fluxes in the global carbon cycle. At present, the accumulated field observation data cover a wide range of geographical locations and climate conditions. However, there are still large uncertainties in the magnitude and spatiotemporal variation of global soil respiration. Using a global soil respiration dataset, we developed a climate-driven model of soil respiration by modifying and updating Raich's model, and the global spatiotemporal distribution of soil respiration was examined using this model. The model was applied at a spatial resolution of 0.5 and a monthly time step. Soil respiration was divided into the heterotrophic and autotrophic components of respiration using an empirical model. The estimated mean annual global soil respiration was 91 Pg C yr-1 (between 1965 and 2012; Monte Carlo 95% confidence interval: 87-95 Pg C yr-1) and increased at the rate of 0.09 Pg C yr-2. The contribution of soil respiration from boreal regions to the total increase in global soil respiration was on the same order of magnitude as that of tropical and temperate regions, despite a lower absolute magnitude of soil respiration in boreal regions. The estimated annual global heterotrophic respiration and global autotrophic respiration were 51 and 40 Pg C yr-1, respectively. The global soil respiration responded to the increase in air temperature at the rate of 3.3 Pg C yr-1 C-1, and Q10 = 1.4. Our study scaled up observed soil respiration values from field measurements to estimate global soil respiration and provide a data-oriented estimate of global soil respiration. Our results, including the modeled spatiotemporal distribution of global soil respiration, are based on a semi-empirical model parameterized with over one thousand data points. We expect that these spatiotemporal estimates will provide a benchmark for future studies and also help to constrain process-oriented models. |
[13] | . Long-term effects of biochar application on soil carbon mineralization are important to evaluate the potential of biochar in carbon sequestration. Over a one-year period of time, we monitored soil respiration from a wheat-maize rotation cropping system after five years (once a year) of consecutive biochar application. In doing so four treatments with three replications each in a random design were examined: (1) a control plot without biochar and straw addition (CT), (2) 4.5 Mg biochar ha 611 02year 611 (B4.5), (3) 9.0 Mg biochar ha 611 02year 611 (B9.0) and (4) straw return (SR, 6515 Mg wheat02+02maize aboveground biomass ha 611 02year 611 ). An equivalent amount of inorganic fertilizers with biochar was broadcasted on the soil surface and then plowed into 651602cm soil depth prior to seeding. Both temporal dynamics and cumulative amounts of soil respiration were not significantly changed under the two biochar applications than under CT, but significantly increased under straw return than under CT and two biochar treatments. The annual respiration was 29.42, 29.96, 30.08, and 39.00 Mg CO 2 ha 611 02year 611 in CT, B4.5, B9.0 and SR treatments, respectively. Meanwhile, soil respiration positively correlated with soil temperature but negatively with soil moisture. Sensitivity of soil respiration to temperature (Q 10 ) was significantly decreased due to biochar addition. These results indicated the potential of applying biochar to enhance soil carbon sequestration. |
[14] | . Abstract There is a need for further studies to compare the decomposition of biochar to that of the original feedstock and determine how these amendments affect the cycling of native organic matter (NOM) of different soils to improve our understanding of the resulting net C sequestration potential. A 510-days incubation experiment was conducted (i) to investigate the evolution of CO2 from soils amended with either fresh corn stover (CS) or with biochars produced from fresh CS at either 350 (CS-350) or 55002°C (CS-550), and (ii) to evaluate the priming effect of these amendments on NOM decomposition. Two soil types were studied: an Alfisol and an Andisol, with organic C contents of 4% and 10%, respectively. Except for the controls (with no C addition), all treatments received 7.1802t C02ha611. We measured C efflux in short-term intervals and its isotopic signature to distinguish between C evolved from C4 amendments and C3-dominated NOM. Emission rates were then integrated for the whole time period to cover total emissions. Total CO2-C evolved from the original C in fresh CS, CS-350 and CS-550 was greater in the Andisol (78%, 13% and 14%) than in the Alfisol (66%, 8% and 7%). For both soils, (i) no significant differences ( P02 > 02 0.05) were observed in the rate of CO2 evolution between controls and biochar treatments; and (ii) total accumulated CO2 evolved from the uncharred amendment was significantly higher ( P02 02 0.05) net priming effect was observed. A C balance indicated that the C lost from both biochar production and decomposition ‘broke even’ with that lost from fresh residue decomposition after 2</sub> emissions from original feedstock while protecting native soil organic matter. |
[15] | . |
[16] | . We analyzed 17 months (August 2005 to December 2006) of continuous measurements of soil CO 2 efflux or soil respiration ( R S ) in an 18-year-old west-coast temperate Douglas-fir stand that experienced somewhat greater than normal summertime water deficit. For soil water content at the 4 cm depth ( θ ) > 0.11 m 3 m 613 (corresponding to a soil water matric potential of 612 MPa), R S was positively correlated to soil temperature at the 2 cm depth ( T S ). Below this value of θ , however, R S was largely decoupled from T S , and evapotranspiration, ecosystem respiration and gross primary productivity (GPP) began to decrease, dropping to about half of their maximum values when θ reached 0.07 m 3 m 613 . Soil water deficit substantially reduced R S sensitivity to temperature resulting in a Q 10 significantly < 2. The absolute temperature sensitivity of R S (i.e. d R S /d T S ) increased with θ up to 0.15 m 3 m 613 , above which it slowly declined. The value of d R S /d T S was nearly 0 for θ < 0.08 m 3 m 613 , thereby confirming that R S was largely unaffected by temperature under soil water stress conditions. Despite the possible effects of seasonality of photosynthesis, root activity and litterfall on R S , the observed decrease in its temperature sensitivity at low θ was consistent with the reduction in substrate availability due to a decrease in (a) microbial mobility, and diffusion of substrates and extracellular enzymes, and (b) the fraction of substrate that can react at high T S , which is associated with low θ . We found that an exponential (van't Hoff type) model with Q 10 and R 10 dependent on only θ explained 92% of the variance in half-hourly values of R S , including the period with soil water stress conditions. We hypothesize that relating Q 10 and R 10 to θ not only accounted for the effects of T S on R S and its temperature sensitivity but also accounted for the seasonality of biotic (photosynthesis, root activity, and litterfall) and abiotic (soil moisture and temperature) controls and their interactions. |
[17] | . The application of biochar to soil has been shown to cause an apparent increase in soil respiration. In this study we investigated the mechanistic basis of this response. We hypothesized that increased CO 2 efflux could occur by: (1) Biochar-induced changes in soil physical properties (bulk density, porosity, moisture content); (2) The biological breakdown of organic carbon (C) released from the biochar; (3) The abiotic release of inorganic C contained in the biochar; (4) A biochar-induced stimulation of decomposition of native soil organic matter (SOM) which could occur both biotically or abiotically; (5) The intrinsic biological activity of the biochar results in the liberation of CO 2 . Our results show that most of the extra CO 2 produced after biochar addition to soil came from the equal breakdown of organic C and the release of inorganic C contained in the biochar. Using long-term 14 C-labelled SOM, we show that biochar repressed native SOM breakdown, counteracting the release of CO 2 from the biochar. A range of mechanisms to describe this negative priming response is presented. Although biochar-induced significant changes in the physical characteristics of the soil, overall this made no contribution to changes in soil respiration. Similarly, the evidence from our study suggests that changes in soluble polyphenols do not help explain the respiration response. In summary, biochar induced a net release of CO 2 from the soil; however, this C loss was very small relative to the amount of C stored within the biochar itself (ca. 0.1%). This short-term C release should therefore not compromise its ability to contribute to long-term C sequestration in soil environments. |
[18] | |
[19] | . Priming effects are strong short-term changes in the turnover of soil organic matter caused by comparatively moderate treatments of the soil. In the course of priming effects large amounts of C, N and other nutrients can be released or immobilized in soil in a very short time. These effects have been measured in many field and laboratory experiments; however, only a few of the studies were aimed at an extended investigation of the mechanisms of such phenomena. The aim of this overview is to reveal possible causes and processes leading to priming actions using the references on agricultural ecosystems and model experiments. Multiple mechanisms and sources of released C and N are presented and summarized in Tables for positive and negative real and apparent priming effects induced after the addition of different organic and mineral substances to the soil. Soil microbial biomass plays the key role in the processes leading to the real priming effects. The most important mechanisms for the real priming effects are the acceleration or retardation of soil organic matter turnover due to increased activity or amount of microbial biomass. Isotopic exchange, pool substitution, and different uncontrolled losses of mineralized N from the soil are responsible for the apparent N priming effects. Other multiple mechanisms (predation, competition for nutrients between roots and microorganisms, preferred uptake, inhibition, etc.) in response to addition of different substances are also discussed. These mechanisms can be distinguished from each other by the simultaneous monitoring of C and N release dynamics; its comparison with the course of microbial activity; and by the labelling of different pools with 14C or 13C and 15N. Quantitative methods for describing priming effects and their dynamics using 14C and 15N isotopes, as well as for non-isotopic studies are proposed. |
[20] | . |
[21] | . The application of bio-char (charcoal or biomass-derived black carbon (C)) to soil is proposed as a novel approach to establish a significant, long-term, sink for atmospheric carbon dioxide in terrestrial ecosystems. Apart from positive effects in both reducing emissions and increasing the sequestration of greenhouse gases, the production of bio-char and its application to soil will deliver immediate benefits through improved soil fertility and increased crop production. Conversion of biomass C to bio-char C leads to sequestration of about 50% of the initial C compared to the low amounts retained after burning (3%) and biological decomposition (< 10–20% after 5–10 years), therefore yielding more stable soil C than burning or direct land application of biomass. This efficiency of C conversion of biomass to bio-char is highly dependent on the type of feedstock, but is not significantly affected by the pyrolysis temperature (within 350–500 66 C common for pyrolysis). Existing slash-and-burn systems cause significant degradation of soil and release of greenhouse gases and opportunies may exist to enhance this system by conversion to slash-and-char systems. Our global analysis revealed that up to 12% of the total anthropogenic C emissions by land use change (0.21 Pg C) can be off-set annually in soil, if slash-and-burn is replaced by slash-and-char. Agricultural and forestry wastes such as forest residues, mill residues, field crop residues, or urban wastes add a conservatively estimated 0.16 Pg C yr 611 . Biofuel production using modern biomass can produce a bio-char by-product through pyrolysis which results in 30.6 kg C sequestration for each GJ of energy produced. Using published projections of the use of renewable fuels in the year 2100, bio-char sequestration could amount to 5.5–9.5 Pg C yr 611 if this demand for energy was met through pyrolysis, which would exceed current emissions from fossil fuels (5.4 Pg C yr 611 ). Bio-char soil management systems can deliver tradable C emissions reduction, and C sequestered is easily accountable, and verifiable. |
[22] | . Soil respiration (SR) is a major component of the global carbon cycle and plays a fundamental role in ecosystem feedback to climate change. Empirical modelling is an essential tool for predicting ecosystem responses to environmental change, and also provides important data for calibrating and corroborating process-based models. In this study, we evaluated the performance of three empirical temperature R response functions (exponential, Lloyd aylor and Gaussian) at seven shrublands located within three climatic regions (Atlantic, Mediterranean and Continental) across Europe. We investigated the performance of SR models by including the interaction between soil moisture and soil temperature. We found that the best fit for the temperature functions depended on the site-specific climatic conditions. Including soil moisture, we identified thresholds in the three different response functions that improved the model fit in all cases. The direct soil moisture effect on SR, however, was weak at the annual time scale. We conclude that the exponential soil temperature function may only be a good predictor for SR in a narrow temperature range, and that extrapolating predictions for future climate based on this function should be treated with caution as modelled outputs may underestimate SR. The addition of soil moisture thresholds improved the model fit at all sites, but had a far greater ecological significance in the wet Atlantic shrubland where a fundamental change in the soil COefflux would likely have an impact on the whole carbon budget. |
[23] | . Black carbon (BC) is an important fraction of many soils worldwide and plays an important role in global C biogeochemistry. However, few studies have examined how it influences the mineralization of added organic matter (AOM) and its incorporation into soil physical fractions and whether BC decomposition is increased by AOM. BC-rich Anthrosols and BC-poor adjacent soils from the Central Amazon (Brazil) were incubated for 53202days either with or without addition of 13 C-isotopically different plant residue. Total C mineralization from the BC-rich Anthrosols with AOM was 25.5% ( P 02<020.05) lower than with mineralization from the BC-poor adjacent soils. The AOM contributed to a significantly ( P 02<020.05) higher proportion to the total C mineralized in the BC-rich Anthrosols (91–92%) than the BC-poor adjacent soils (69–80%). The AOM was incorporated more rapidly in BC-rich than BC-poor soils from the separated free light fraction through the intra-aggregate light fraction into the stable organo-mineral fraction and up to 340% more AOM was found in the organo-mineral fraction. This more rapid stabilization was observed despite a significantly ( P 02<020.05) lower metabolic quotient for BC-rich Anthrosols. The microbial biomass (MB) was up to 125% greater ( P 02<020.05) in BC-rich Anthrosols than BC-poor adjacent soils. To account for increased MB adsorption onto BC during fumigation extraction, a correction factor was developed via addition of a 13 C-enriched microbial culture. The recovery was found to be 21–41% lower ( P 02<020.05) for BC-rich than BC-poor soils due to re-adsorption of MB onto BC. Mineralization of native soil C was enhanced to a significantly greater degree in BC-poor adjacent soils compared to BC-rich Anthrosols as a result of AOM. No positive priming by way of cometabolism due to AOM could be found for aged BC in the soils. |
[24] | . . 在极端干旱区(敦煌)以泡泡刺群落为研究对象,测定了生长季内增雨对泡泡刺群落灌丛和裸地土壤呼吸温度敏感性()的影响。结果表明:增雨明显增加了裸地的,但未能显著改变灌丛的 ;与对照相比,增雨16 mm使裸地显著增加28%,达1.83±0.30 ;在整个生长季,裸地和灌丛的季节波动与土壤含水量的变化存在极显著相关关系,且裸地的对土壤水分的敏感性(1.94)高于灌丛(1.57)。 |
[25] | . Biochar addition to soil has been widely accepted as an option to enhance soil carbon sequestration by introducing recalcitrant organic matter. However, it remains unclear whether biochar will negate the net carbon accumulation by increasing carbon loss through CO 2 efflux from soil (soil respiration). The objectives of this study were to address: 1) whether biochar addition increases soil respiration; and whether biochar application rate and biochar type (feedstock and pyrolyzing system) affect soil respiration. Two series of field experiments were carried out at 8 sites representing the main crop production areas in China. In experiment 1, a single type of wheat straw biochar was amended at rates of 0, 20 and 4002t02ha 61021 in four rice paddies and three dry croplands. In experiment 2, four types of biochar (varying in feedstock and pyrolyzing system) were amended at rates of 0 and 2002t02ha 61021 in a rice paddy under rice-wheat rotation. Results showed that biochar addition had no effect on CO 2 efflux from soils consistently across sites, although it increased topsoil organic carbon stock by 38% on average. Meanwhile, CO 2 efflux from soils amended with 4002t of biochar did not significantly higher than soils amended with 2002t of biochar. While the biochars used in Experiment 2 had different carbon pools and physico-chemical properties, they had no effect on soil CO 2 efflux. The soil CO 2 efflux following biochar addition could be hardly explained by the changes in soil physic-chemical properties and in soil microbial biomass. Thus, we argue that biochar will not negate the net carbon accumulation by increasing carbon loss through CO 2 efflux in agricultural soils. |
[26] | . After 87 days, biochar350 addition caused priming effects equivalent to 250 and 319μg CO 2 –Cg 611 soil, in the low and high pH soil, respectively. The largest priming effects occurred at the start of the incubations. The size of the priming effect was decreased at higher biochar pyrolysis temperatures, which may be a way of controlling priming effects following biochar incorporation to soil, if desired. The priming effect was probably induced by the water soluble components of the biochar. At 87 days of incubation, 0.14% and 0.18% of biochar700 and 0.61% and 0.84% of biochar350 were mineralized in the low and high pH soil, respectively. Ryegrass addition gave an increased biochar350 mineralisation of 33% and 40%, and increased biochar700 at 137% and 70%, in the low and high pH soils, respectively. Certainly, on the basis of our results, if biochar is used to sequester carbon a priming effect may occur, increasing CO 2 –C evolved from soil and decreasing soil organic C. However, this will be more than compensated for by the increased soil C caused by biochar incorporation. A similar conclusion holds for accelerated mineralisation of biochar due to incorporation of fresh labile substrates. We consider that our results are the first to unequivocally demonstrate the initiation, progress and termination of a true positive priming effect by biochar on native soil organic C. |
[27] | . |
[28] | . The United Nations Framework Convention on Climate Change (UNFCCC) requires that all parties to the convention periodically report their emissions of greenhouse gases and the Intergovernmental Panel on Climate Change (IPCC) has published guidelines on how to estimate these emissions. Estimating carbon dioxide (CO 2 ) emissions is complicated by the fact that consumption of fossil-fuels and harvesting of forests do not necessarily mean that the contained C has been released to the atmosphere as CO 2 . Some fractions of fossil-fuels and harvested wood are incorporated into products that have lifetimes ranging from months to centuries. The IPCC methodology addresses durable products by assuming that some prescribed fraction goes to permanent storage while the remainder is oxidized instantly. The question posed here is whether the annual increase in stocks of durable products, i.e. the difference between the rates of production and oxidation, can be reasonably estimated as a simple fraction of their current rate of production. Although the annual stock change can be described as a simple fraction of annual production when production is growing exponentially and oxidation is a first order decay process, a description of annual stock changes needs to consider how both production and oxidation are evolving with time, regardless of the functional forms of these changes with time. |
[29] | . Biochar amendment in soil has been proposed as a carbon sequestration strategy which may also enhance soil physical and chemical properties such as nutrient and water holding capacity as well as soil fertility and plant productivity. However, biochar may also stimulate microbial activity which may lead to increased soil CO 2 respiration and accelerated soil organic matter (OM) degradation which could partially negate these intended benefits. To investigate short-term soil microbial responses to biochar addition, we conducted a 24 week laboratory incubation study. Biochar produced from the pyrolysis of sugar maple wood at 500 C was amended at concentrations of 5, 10 and 20t/ha in a phosphorus-limited forest soil which is under investigation as a site for biochar amendment. The cumulative soil CO 2 respired was higher for biochar-amended samples relative to controls. At 10 and 20t/ha biochar application rates, the concentration of phospholipid fatty acids (PLFAs) specific to Gram-positive and Gram-negative bacteria as well as actinomycetes were lower than controls for the first 16 weeks, then increased between weeks 16 24, suggesting a gradual microbial adaptation to altered soil conditions. Increases in the ratio of bacteria/fungi and lower ratios of Gram-negative/Gram-positive bacteria suggest a microbial community shift in favour of Gram-positive bacteria. In addition, decreasing ratios of cy17:0/16:1 7 PLFAs, a proxy used to examine bacterial substrate limitation, suggest that bacteria adapted to the new conditions in biochar-amended soil over time. Concentrations of water-extractable organic matter (WEOM) increased in all samples after 24 weeks and were higher than controls for two of the biochar application rates. Solution-state 1 H NMR analysis of WEOM revealed an increase in microbial-derived short-chain carboxylic acids, lower concentrations of labile carbohydrate and peptide components of soil OM and potential accumulation of more recalcitrant polymethylene carbon during the incubation. Our results collectively suggest that biochar amendment increases the activity of specific microorganisms in soil, leading to increased CO 2 fluxes and degradation of labile soil OM constituents. |
[30] | . Biochar additions to soil can increase soil organic carbon (SOC) concentrations; however, minimal information is available on relationships with soil nitrogen (N) cycle. We hypothesized that biochar additions to sandy soils should be resistant to microbial mineralization in short-term studies but may prime organic carbon (OC) mineralization of fresh residue that promotes N immobilization. A laboratory pot incubation study was conducted with a Norfolk loamy sand (Fine-loamy, kaolinitic, thermic, Typic Kandiudult) mixed with pecan-shell biochar at rates of 0, 5, 10 and 20 g kg 61 1 and with 0 and 10 g kg 61 1 dried, ground switchgrass ( Panicum virgatum L. ). On days 25 and 67 of the incubation, all pots were leached with 1.2 to 1.3 pore volumes of deionized H 2O and the leachate NO 3–N, NH 4–N, and dissolved organic (DOC) concentrations were measured. Also cumulative soil CO 2 fluxes after days 25 and 67 were determined. Biochar alone and mixed with soil and switchgrass after 67 days of incubation were characterized using Fourier transformed infrared spectroscopy (FT-IR). Mixing biochar with switchgrass after 67 days caused a significant increase in SOC content while soil total nitrogen (TN) and leachate DOC concentrations showed mixed results. Biochar mineralization by itself was found to be minimal, but by days 25 and 67, soil with biochar and switchgrass exhibited higher cumulative CO 2 fluxes implying stimulation of switchgrass mineralization. Significant NO 3–N immobilization occurred after 25 days in treatments with biochar + switchgrass; however, by day 67 the NO 3–N concentrations rebounded slightly. The FT-IR analysis revealed that switchgrass in the presence of biochar underwent some structural modifications. Biochar applications in the short-term can cause N immobilization resulting in temporary plant available NO 3–N concentration reductions. |
[31] | . SummaryRoots are the first point of contact between biochar particles and growing plants, yet detailed studies of biochar oot interactions are few. Biochar may affect root growth, and therefore plant performance, through two mechanisms: (i) as a direct nutrient source and (ii) through impacts on nutrient availability. To test the hypothesis that biochar oot interactions occur and are determined by biochar nutrient supply and impacts on soil nutrients, spring barley (Hordeum vulgare L.) was grown with and without biochar addition in rhizobox mesocosms. Biochar from unaltered and artificially weathered Miscanthus or willow (Salix sp) biochar types was used and was manipulated to alter its structure and nutrient content. After 28 ays of plant growth, biochar nutrient content, soil nutrient content and the amount of biochar were measured in the bulk soil, the rhizosphere and the rhizosheath. Plants in biochar-amended soils had larger rhizosphere zones than the control treatment. The rhizosphere contained more biochar particles than the bulk soil, an indication that roots preferred soil containing biochar particles. Biochar particles retained soil nitrogen (N) in the form of nitrate, and also supplied phosphorus (P) to the soil and plant. Miscanthus biochar had a larger extractable P content than the Salix biochar, with different effects on plant growth and root responses. Although artificial physical weathering had no effect on overall plant growth, weathering effects on N retention and P content were dependent on biochar type. Our results indicate that roots are attracted towards biochar, resulting in its partitioning between bulk and rhizosphere soil. Biochar thus controls plant root nutrient acquisition directly as a nutrient source and indirectly by altering soil nutrient content. |
[32] | . |
[33] | . |
[34] | . |
[35] | . Abstract Microbial decomposition of soil organic matter produces a major flux of CO2 from terrestrial ecosystems and can act as a feedback to climate change. Although climate-carbon models suggest that warming will accelerate the release of CO2 from soils, the magnitude of this feedback is uncertain, mostly due to uncertainty in the temperature sensitivity of soil organic matter decomposition. We examined how warming and altered precipitation affected the rate and temperature sensitivity of heterotrophic respiration ( R h) at the Boston-Area Climate Experiment, in Massachusetts, USA. We measured R h inside deep collars that excluded plant roots and litter inputs. In this mesic ecosystem, R h responded strongly to precipitation. Drought reduced R h, both annually and during the growing season. Warming increased R h only in early spring. During the summer, when R h was highest, we found evidence of threshold, hysteretic responses to soil moisture: R h decreased sharply when volumetric soil moisture dropped below ~15% or exceeded ~26%, but R h increased more gradually when soil moisture rose from the lower threshold. The effect of climate treatments on the temperature sensitivity of R h depended on the season. Apparent Q 10 decreased with high warming (~3.5 C) in spring and fall. Presumably due to limiting soil moisture, warming and precipitation treatments did not affect apparent Q 10 in summer. Drought decreased apparent Q 10 in fall compared to ambient and wet precipitation treatments. To our knowledge, this is the first field study to examine the response of R h and its temperature sensitivity to the combined effects of warming and altered precipitation. Our results highlight the complex responses of R h to soil moisture, and to our knowledge identify for the first time the seasonal variation in the temperature sensitivity of microbial respiration in the field. We emphasize the importance of adequately simulating responses such as these when modeling trajectories of soil carbon stocks under climate change scenarios. |
[36] | . Poor water retention capacity (WRC) and nutrient deficiency commonly limit crop yields in sandy soils. The use of biochar as a soil amendment has been previously reported to improve these limiting factors in subtropical and temperate soils. We studied the effects of biochar on soil properties and yield formation of spring wheat ( Triticum aestivum L.) when applied together with inorganic fertiliser or meat bone meal (MBM) to an Endogleyic Umbrisol with a loamy sand texture in boreal conditions. In a two-year field experiment, biochar was applied at 0, 5, 10, 20 and 3002t02ha 611 combined with three fertiliser treatments (unfertilised control, MBM and inorganic fertiliser) providing equal amounts of nitrogen (N), phosphorus (P) and potassium (K). Soil WRC and fertility as well as wheat yield, yield components and quality were analysed. Soil moisture content, leaf area index and leaf chlorophyll values (SPAD) were monitored during the experiment. Biochar increased the plant-available water content of the topsoil in the first year and reduced the bulk density in the second year after application. It also increased the contents of easily soluble K and soil organic C (SOC) in the 2002cm of topsoil, but had no effects on other soil nutrients, pH or moisture content. Biochar amendment decreased the soil NO 3 61 -N content below control values in the first year but increased it significantly in the second year. The addition of biochar did not significantly affect the nitrogen uptake, grain yield or quality of wheat, possibly because of its low nutrient availability and the high organic matter content of the soil. |
[37] | . iochar , or charcoal intended for use as a soil amendment, has received great attention in recent years as a means of enhancing carbon sequestration and soil properties in agricultural systems. Here we address the potential for biochar use in the context of forest restoration, reviewing relevant experimental studies on biochar use in forest ecosystems, the properties of chars generated from wood waste material, and available data on tree growth responses to biochar. To our knowledge the earliest mention of char use as a soil amendment is actually specifically in the context of forest restoration (in the 1820s in Scotland). Wood waste biochars have an unusual set of properties that suggest their applicability in a forest restoration context: namely, high recalcitrance promoting long-lasting effects, retention of cations, anions, and water, in the soil, sorptive properties that can reduce bioavailability of a wide range of toxic materials, and relative ease of production from locally available feedstocks. A meta-analysis of recent studies on biochar responses of woody plants indicates a potential for large tree growth responses to biochar additions, with a mean 41 % increase in biomass. Responses are especially pronounced at early growth stages, and appear to be higher in boreal and tropical than in temperate systems, and in angiosperms than conifers; however, there is high variability, and field studies are few. The properties of biochars also vary greatly depending on feedstock and pyrolysis conditions; while this complicates their use, it provides a means to design biochars for specific restoration situations and objectives. We conclude that there is great promise for biochar to play an important role in a wide variety of forest restoration efforts, specifically as a replacement product for other forms of organic matter and liming agents. |
[38] | . SummarySome intensive agricultural practices result in soil degradation through loss of soil organic matter. Organic farming may mitigate this problem, if managed properly, but may result in a yield penalty compared with conventional systems. Biochar addition to soil could influence both agricultural systems, but previous studies are not definitive about its impact on soil processes. Sandy soils are more susceptible to the effects of reduced soil organic matter on soil hydrology and nutrient dynamics. Nitrogen (N) is important for crop growth and soil water content can influence its transformation and cycling. This study explored the effect of biochar amendment on soil water retention and nitrification processes in soils under organic and conventional management. Carbon dioxide evolution was used as an indicator of related microbial activity. A water release curve study and a 60-day incubation experiment were set up to consider the effect of biochar application on organically and conventionally managed sandy loam soils. The results showed that addition of biochar increased water retention for both soils and this is attributed to its porous structure. On incubation of an organically managed soil, with green-waste compost, initial ammonium level was small, reflecting microbial demand for N. The large cation exchange capacity of the organically managed soil retained ammonium, reducing availability for nitrification. Carbon dioxide evolution increased with continuing small contents of ammonium and nitrate when biochar was added to the organically managed soil. Biochar enhanced nitrification without increased respiration during incubation of a conventionally managed soil with added mineral N; a possible explanation for this enhancement is the increase in pH resulting from the biochar addition. |
[39] | . @misc{4230990, author = {Verheijen, Frank and Graber, Ellen and Ameloot, Nele and Bastos, Ana Catharina and Sohi, Saran and Knicker, Heike}, issn = {1351-0754}, keyword = {RESISTANCE,MECHANISMS,CLIMATE-CHANGE,SEEDLINGS,REMEDIATION}, language = {eng}, number = {1}, pages = {22--27}, series = {EUROPEAN JOURNAL OF SOIL SCIENCE}, title = {Biochars in soils: new insights and emerging research needs}, url = {http://dx.doi.org/10.1111/ejss.12127}, volume = {65}, year = {2014}, } |
[40] | . Fire is a global driver of carbon storage and converts a substantial proportion of plant biomass to black carbon (for example, charcoal), which remains in the soil for thousands of years. Black carbon is therefore often proposed as an important long-term sink of soil carbon. We ran a 10-year experiment in each of three boreal forest stands to show that fire-derived charcoal promotes loss of forest humus and that this is associated with enhancement of microbial activity by charcoal. This result shows that charcoal-induced losses of belowground carbon in forests can partially offset the benefits of charcoal as a long-term carbon sink. |
[41] | . . 区分森林土壤呼吸组分是了解生态系统碳循环的重要环节。该文以福建省三明市格氏栲自然保护区米槠(Castanopsis carlesii)人工林和邻近的杉木(Cunninghamia lanceolata)人工林为研究对象, 于2012年8月至2013年7月, 采用LI-8100开路式土壤碳通量系统, 通过挖壕沟方法, 测定了土壤呼吸及异养呼吸的速率, 同时测定了5 cm深处的土壤温度和0–12 cm深处的土壤含水量。利用指数模型和双因素模型, 分析土壤呼吸及其组分与土壤温度和土壤含水量的关系, 同时计算了土壤呼吸各组分在土壤呼吸中所占的比例, 并分析了不同森林类型对土壤呼吸及其组分的影响。结果表明: 米槠人工林和杉木人工林土壤呼吸及其组分的季节变化显著, 均呈单峰型曲线, 与5 cm深处的土壤温度呈极显著正相关关系。土壤温度可以分别解释米槠人工林土壤呼吸、自养呼吸和异养呼吸变化的70.3%、73.4%和58.2%, 可以解释杉木人工林土壤呼吸、自养呼吸和异养呼吸变化的77.9%、65.7%和79.2%。土壤呼吸及其组分与土壤含水量没有相关关系。米槠和杉木人工林自养呼吸的年通量分别为4.00和2.18 t C·hm^–2·a^–1, 占土壤呼吸年通量的32.5%和24.1%; 异养呼吸年通量分别为8.32和6.88 t C·hm^–2·a^–1, 分别占土壤呼吸年通量的67.5%和75.9%, 米槠人工林土壤呼吸及其组分的年通量都大于杉木人工林。 |
[42] | . . 大气中温室气体,特别是CO2浓度升高导致全球变暖及其一系列生态问题已经引起了世界各国的高度关注,成为科学研究领域的热点问题。森林作为陆地生态系统的主体,在减缓大气中CO2浓度上升,调节全球碳平衡方而有着重要作用。毛竹Phyllostachys pubescens是我国南方重要的森林资源,种植而积大,经济用途广泛,成为当地农民经济收入的重要来源。毛竹具有生长速度快、能够隔年连续采伐和长期利用等特点。同时,由于毛竹特殊的无性繁殖方式,每年有大量新笋长大成竹向周围扩展,因而毛竹林固定CO2的潜力巨大,对平衡大气中CO2浓度具有重要的作用。本文采用野外调研和定位试验相结合的方法,研究了毛竹林、松林、阔叶林、针阔混交林森林群落外貌特征、优势种重要值、物种多样性;分析了立竹度对毛竹种群结构与生物量、碳密度的影;研究了毛竹快速生长过程中的碳积累规律。主要研究结果如下 1.对不同森林群落外貌特征、优势种重要值、物种多样性研究表明:阔叶林、针阔混交林具有较高的均匀度指数,而松林和毛竹林则会因为物种分布的随机性较大而导致群落总休均匀度指数仇下降,群落的稳定性较差。另外,乔木层中马尾松和苦槠种群占有很大的比例,其重要值分别达到了55.75%和28.06%,灌木层中檵木和大青在其分层中表现出较大的的优势。 2.毛竹林的单位生物量随着立竹度的增加,呈现波动上升的趋势;单株生物量和平均胸径、平均高度则呈现相反的趋势;毛竹林的单位面积生物量为66.82t·hm-2,碳密度为33.89t-hm-2,不同部位中竹杆的生物量比例最高,达到总生物量的56.39%;研究发现中高立竹度(3825株.hm-2)单位面积生物量达75.02t·hm-2,单株平均生物量为19.45kg,碳密度38.07t·hm-2,是毛竹碳汇林经营中较为理想的类型。 3.毛竹出土后近三个月中生长迅速,平均伸长量和干物质积累量分别为17cm-d-1和96g·d-1,毛竹茎秆上、中、下三部分碳密度均持续上升至接近50%,碳素主要固定于毛竹细胞壁纤维素与半纤维素组分中。研究期内毛竹茎秆不同部位氮、磷、钾、钙、镁元素浓度变化与碳浓度变化相反,均呈下降趋势。表明毛竹出土后近三个月的快速生长主要得益于体内碳素的高效同化作用。 |
[43] | . . 土壤呼吸是陆地生态系统碳循环的重要环节之一,其对温度升高的敏感程度在 很大程度上决定着全球气候变化与碳循环之间的反馈关系.为了深刻理解地下生态过程对气候变化的响应和适应,综述了土壤呼吸温度敏感性 (Q)的影响因子及其内在机制,并分析了当前研究存在的不确定性.土壤生物、底物质量和底物供应显著调控着土壤呼吸的 Q值,但研究结论仍然有很大差异.温度和水分等环境因子则通过对土壤生物和底物的影响而作用于土壤呼吸的温度敏感性,一般情况下, 随着温度的升高,土壤呼吸的Q值下降;水分过高或过低时Q值降低.从土壤温度测定深度、时空尺度、土壤呼吸 不同组分温度敏感性差异、激发效应以及采用方法的不同等几方面分析了温度敏感性研究存在的不确定性.并在此基础上,提出了未来重点研究方向:(1)土壤呼 吸不同组分温度敏感性差异的机理;(2)底物质量和底物供应对温度敏感性的交互影响;(3)生物因子对土壤呼吸温度敏感性的影响. |
[44] | . This experiment was designed to study three determinant factors in decomposition patterns of soil organic matter (SOM): temperature, water and carbon (C) inputs. The study combined field measurements with soil lab incubations and ends with a modelling framework based on the results obtained. Soil respiration was periodically measured at an oak savanna woodland and a ponderosa pine plantation. Intact soils cores were collected at both ecosystems, including soils with most labile C burnt off, soils with some labile C gone and soils with fresh inputs of labile C. Two treatments, dry-field condition and field capacity, were applied to an incubation that lasted 111 days. Short-term temperature changes were applied to the soils periodically to quantify temperature responses. This was done to prevent confounding results associated with different pools of C that would result by exposing treatments chronically to different temperature regimes. This paper discusses the role of the above-defined environmental factors on the variability of soil C dynamics. At the seasonal scale, temperature and water were, respectively, the main limiting factors controlling soil CO 2 efflux for the ponderosa pine and the oak savanna ecosystems. Spatial and seasonal variations in plant activity (root respiration and exudates production) exerted a strong influence over the seasonal and spatial variation of soil metabolic activity. Mean residence times of bulk SOM were significantly lower at the Nitrogen (N)-rich deciduous savanna than at the N-limited evergreen dominated pine ecosystem. At shorter time scales (daily), SOM decomposition was controlled primarily by temperature during wet periods and by the combined effect of water and temperature during dry periods. Secondary control was provided by the presence/absence of plant derived C inputs (exudation). Further analyses of SOM decomposition suggest that factors such as changes in the decomposer community, stress-induced changes in the metabolic activity of decomposers or SOM stabilization patterns remain unresolved, but should also be considered in future SOM decomposition studies. Observations and confounding factors associated with SOM decomposition patterns and its temperature sensitivity are summarized in the modeling framework. |
[45] | . . 竹林地下系统由竹林鞭系、竹林根系以及竹蔸组成,根系又由竹根和鞭根及其各级支根组成。散生竹林地下部分是一个相互连接的网络结构,形成一个庞大、复杂、有机的系统。无论是对竹林生产力形成,还是对生态功能发挥,它都具有不可替代的作用。 由于竹林地下系统深藏土壤,观测和测定十分困难,对它的研究也相对薄弱,研究手段和技术是制约和限制地下部分研究发展的重要因素,尤其是对地下部分动态的研究,目前的研究手段和方法都存在一定的缺陷。 小观察窗(Minirhizotron)技术的诞生和发展大大改善了这种状况,其最大的优点是,能够对土壤中处于自然状... |
[46] | . Pyrogenic carbon (biochar) amendment is increasingly discussed as a method to increase soil fertility while sequestering atmospheric carbon (C). However, both increased and decreased C mineralization has been observed following biochar additions to soils. In an effort to better understand the interaction of pyrogenic C and soil organic matter (OM), a range of Florida soils were incubated with a range of laboratory-produced biochars and CO 2 evolution was measured over more than one year. More C was released from biochar-amended than from non-amended soils and cumulative mineralized C generally increased with decreasing biomass combustion temperature and from hardwood to grass biochars, similar to the pattern of biochar lability previously determined from separate incubations of biochar alone. The interactive effects of biochar addition to soil on CO 2 evolution (priming) were evaluated by comparing the additive CO 2 release expected from separate incubations of soil and biochar with that actually measured from corresponding biochar and soil mixtures. Priming direction (positive or negative for C mineralization stimulation or suppression, respectively) and magnitude varied with soil and biochar type, ranging from 6152 to 89% at the end of 1 year. In general, C mineralization was greater than expected (positive priming) for soils combined with biochars produced at low temperatures (250 and 40002°C) and from grasses, particularly during the early incubation stage (first 9002d) and in soils of lower organic C content. It contrast, C mineralization was generally less than expected (negative priming) for soils combined with biochars produced at high temperatures (525 and 65002°C) and from hard woods, particularly during the later incubation stage (250–50002d). Measurements of the stable isotopic signature of respired CO 2 indicated that, for grass biochars at least, it was predominantly pyrogenic C mineralization that was stimulated during early incubation and soil C mineralization that was suppressed during later incubation stages. It is hypothesized that the presence of soil OM stimulated the co-mineralization of the more labile components of biochar over the short term. The data strongly suggests, however, that over the long term, biochar–soil interaction will enhance soil C storage via the processes of OM sorption to biochar and physical protection. |
1
2009
... 温度和水分等环境因子通过土壤生物和底物间接影响土壤呼吸的温度敏感性(
Interactions between biochar stability and soil organisms: Review and research needs
1
2013
... 生物质炭添加对毛竹林土壤呼吸速率的后续影响与添加剂量相关.研究表明生物质炭添加到土壤后, 自身碳矿化随着时间逐渐降低(
An incubation study on the stability and biological effects of pyrogenic and hydrothermal biochar in two soils
2014
Carbon dioxide emissions from biochar in soils: Role of clay, microorganisms and carbonates
2
2014
... 土壤激发效应是指各种有机质添加等处理引起土壤有机质周转强烈的短期改变(
... 生物质炭添加对土壤碳排放的影响与非生物因子关系密切, 生物质炭添加的负激发效应与土壤碳酸盐含量紧密相关(
Response of microbial community structure and function to short-term biochar amendment in an intensively managed bamboo (Phyllostachys praecox) plantation soil: Effect of particle size and addition rate
2017
The priming potential of biochar products in relation to labile carbon contents and soil organic matter status
1
2011
... 土壤激发效应是指各种有机质添加等处理引起土壤有机质周转强烈的短期改变(
Wildfire-produced charcoal directly influences nitrogen cycling in Ponderosa pine forests
1
2006
... 大气中温室气体CO2浓度升高导致的全球变暖及一系列其他生态问题已引起世界各国的高度关注, 成为科学研究领域的热点问题(
Global patterns in belowground communities
1
2009
... 土壤呼吸除受到非生物因子影响外, 还受到土壤微生物、植被类型等生物因子的影响.生物质炭添加改变微生物群落, 因为其通过对底物有效养分和pH值的改变而改变微生物群落组成(
Formation, transformation and transport of black carbon (charcoal) in terrestrial and aquatic ecosystems
1
2006
... 竹林是我国重要的森林资源类型, 中国现有竹林面积约601万hm2, 且具有生长速度快、隔年连续采伐和可长期利用等特点, 每年有大量的新笋成竹向周围扩展, 因而竹林固定CO2的潜力巨大, 对吸存大气中的CO2具有重要作用.然而, 中国每年竹材砍伐量为800-900万t, 相当于1 000余万m3木材, 但在竹材被砍伐加工利用的同时, 38%-84%的生物质废弃物在燃烧过程中被释放(
生物质炭输入对土壤碳排放的激发效应研究进展
1
2016
... 研究表明生物质炭添加明显影响原位土壤有机碳矿化(
Does biochar influence soil physical properties and soil water availability?
2
2014
... 土壤温度、土壤水分是影响土壤CO2通量的主导因子, 它们通过对凋落物分解过程、微生物活性、根系生长及光合作用调节而影响土壤呼吸速率(
... 生物质炭添加对土壤碳排放的影响与非生物因子关系密切, 生物质炭添加的负激发效应与土壤碳酸盐含量紧密相关(
Global spatiotemporal distribution of soil respiration modeled using a global database
1
2015
... 研究表明生物质炭添加明显影响原位土壤有机碳矿化(
Sensitivity of soil respiration to soil temperature decreased under deep biochar amended soils in temperate croplands
4
2016
... 研究表明生物质炭添加明显影响原位土壤有机碳矿化(
... )(
... 土壤温度、土壤水分是影响土壤CO2通量的主导因子, 它们通过对凋落物分解过程、微生物活性、根系生长及光合作用调节而影响土壤呼吸速率(
... 温度和水分等环境因子通过土壤生物和底物间接影响土壤呼吸的温度敏感性(
Experimental evidence for sequestering C with biochar by avoidance of CO2 emissions from original feedstock and protection of native soil organic matter
3
2015
... 研究表明生物质炭添加明显影响原位土壤有机碳矿化(
... )或为微生物提供养分而增加微生物活性; 另一方面, 生物质炭添加抑制原位土壤碳矿化(负激发效应), 生物质炭表面吸附原位土壤易溶物或促进土壤团聚体及原位土壤有机碳的稳定性(
... 土壤激发效应是指各种有机质添加等处理引起土壤有机质周转强烈的短期改变(
Biomass, carbon, and nutrient dynamics of secondary forests in a humid tropical region of méxico
1
1999
... 竹林是我国重要的森林资源类型, 中国现有竹林面积约601万hm2, 且具有生长速度快、隔年连续采伐和可长期利用等特点, 每年有大量的新笋成竹向周围扩展, 因而竹林固定CO2的潜力巨大, 对吸存大气中的CO2具有重要作用.然而, 中国每年竹材砍伐量为800-900万t, 相当于1 000余万m3木材, 但在竹材被砍伐加工利用的同时, 38%-84%的生物质废弃物在燃烧过程中被释放(
Effect of soil water stress on soil respiration and its temperature sensitivity in an 18-year-old temperate Douglas-fir stand
1
2008
... 温度和水分等环境因子通过土壤生物和底物间接影响土壤呼吸的温度敏感性(
Short-term biochar-induced increase in soil CO2 release is both biotically and abiotically mediated
2
2011
... 土壤激发效应是指各种有机质添加等处理引起土壤有机质周转强烈的短期改变(
... 生物质炭对土壤CO2排放(土壤呼吸)的影响因素包括微生物的生物降解和环境的非有机碳部分的非生物因素(
Influence of biochar on drought tolerance of
1
2011
... 研究中生物质炭添加剂量对土壤碳排放的影响显著(
Review of mechanisms and quantification of priming effects
1
2000
... 土壤激发效应是指各种有机质添加等处理引起土壤有机质周转强烈的短期改变(
Soil carbon sequestration to mitigate climate change and advance food security
1
2007
... 大气中温室气体CO2浓度升高导致的全球变暖及一系列其他生态问题已引起世界各国的高度关注, 成为科学研究领域的热点问题(
Bio-char sequestration terrestrial ecosystems—A review
1
2006
... 竹林是我国重要的森林资源类型, 中国现有竹林面积约601万hm2, 且具有生长速度快、隔年连续采伐和可长期利用等特点, 每年有大量的新笋成竹向周围扩展, 因而竹林固定CO2的潜力巨大, 对吸存大气中的CO2具有重要作用.然而, 中国每年竹材砍伐量为800-900万t, 相当于1 000余万m3木材, 但在竹材被砍伐加工利用的同时, 38%-84%的生物质废弃物在燃烧过程中被释放(
Temperature dependence of soil respiration modulated by thresholds in soil water availability across European shrubland ecosystems
1
2016
... 土壤温度、土壤水分是影响土壤CO2通量的主导因子, 它们通过对凋落物分解过程、微生物活性、根系生长及光合作用调节而影响土壤呼吸速率(
Black carbon affects the cycling of non-black carbon in soil
1
2010
... 生物质炭添加对土壤碳排放的影响与非生物因子关系密切, 生物质炭添加的负激发效应与土壤碳酸盐含量紧密相关(
极端干旱区增雨对泡泡刺(Nitraria sphaerocarpa)群落土壤呼吸温度敏感性的影响
3
2016
... 土壤激发效应是指各种有机质添加等处理引起土壤有机质周转强烈的短期改变(
... 温度和水分等环境因子通过土壤生物和底物间接影响土壤呼吸的温度敏感性(
... 生物质炭对土壤CO2排放(土壤呼吸)的影响因素包括微生物的生物降解和环境的非有机碳部分的非生物因素(
Biochar has no effect on soil respiration across Chinese agricultural soils
2016
Short term soil priming effects and the mineralization of biochar following its incorporation to soils of different pH
1
2011
... 土壤激发效应是指各种有机质添加等处理引起土壤有机质周转强烈的短期改变(
Maize yield and nutrition during 4 years after biochar application to a Colombian savanna oxisol
1
2010
... 土壤激发效应是指各种有机质添加等处理引起土壤有机质周转强烈的短期改变(
The treatment of long-lived, carbon-containing products in inventories of carbon dioxide emissions to the atmosphere
1
2003
... 大气中温室气体CO2浓度升高导致的全球变暖及一系列其他生态问题已引起世界各国的高度关注, 成为科学研究领域的热点问题(
Shifts in microbial community and water-extractable organic matter composition with biochar amendment in a temperate forest soil
2
2015
... 大气中温室气体CO2浓度升高导致的全球变暖及一系列其他生态问题已引起世界各国的高度关注, 成为科学研究领域的热点问题(
... 生物质炭添加对土壤呼吸速率的季节变化影响不同(
Short-term CO2 mineralization after additions of biochar and switchgrass to a typic kandiudult
2010
Biochar- root interactions are mediated by biochar nutrient content and impacts on soil nutrient availability
1
2014
... 研究中生物质炭添加剂量对土壤碳排放的影响显著(
Stand age-related effects on soil respiration in a first rotation Sitka spruce chronosequence in central Ireland
3
2006
... 土壤呼吸与土壤湿度的关系采用如下模型(
... 土壤呼吸与土壤温度和湿度的关系, 采用如下模型(
... 温度和水分等环境因子通过土壤生物和底物间接影响土壤呼吸的温度敏感性(
A review of biochar and its use and function in soil. In: Donald LS ed. Advances in Agronomy
1
2010
... 大气中温室气体CO2浓度升高导致的全球变暖及一系列其他生态问题已引起世界各国的高度关注, 成为科学研究领域的热点问题(
Ethylene: Potential key for biochar amendment impacts
2010
Effects of soil moisture on the temperature sensitivity of heterotrophic respiration vary seasonally in an old-field climate change experiment
2012
Short-term effects of biochar on soil properties and wheat yield formation with meat bone meal and inorganic fertiliser on a boreal loamy sand
1
2014
... 2014年4月, 随机选择立地条件相似的毛竹林4块, 每块林分随机各设置3个20 m × 30 m的样地, 每个样地中随机设置4个1 m ×1 m的小样方, 用于生物质炭添加处理, 每处理3次重复.为防止相互干扰, 小样方间至少相隔10 m.根据国内外生物质炭添加试验研究的强度和竹林经营实况(
Biochar and forest restoration: A review and meta-analysis of tree growth responses
1
2015
... 大气中温室气体CO2浓度升高导致的全球变暖及一系列其他生态问题已引起世界各国的高度关注, 成为科学研究领域的热点问题(
Impact of biochar addition on water retention, nitrification and carbon dioxide evolution from two sandy loam soils
1
2014
... 土壤温度、土壤水分是影响土壤CO2通量的主导因子, 它们通过对凋落物分解过程、微生物活性、根系生长及光合作用调节而影响土壤呼吸速率(
Biochars in soils: New insights and emerging research needs
2
2014
... 生物质炭添加对土壤碳排放的影响与非生物因子关系密切, 生物质炭添加的负激发效应与土壤碳酸盐含量紧密相关(
... 土壤呼吸除受到非生物因子影响外, 还受到土壤微生物、植被类型等生物因子的影响.生物质炭添加改变微生物群落, 因为其通过对底物有效养分和pH值的改变而改变微生物群落组成(
Fire-derived charcoal causes loss of forest humus
1
2008
... 研究表明生物质炭添加明显影响原位土壤有机碳矿化(
米槠和杉木人工林土壤呼吸及其组分分析
1
2014
... 生物质炭添加对土壤呼吸速率的季节变化影响不同(
毛竹林群落结构与碳积累规律研究
1
2012
... 大气中温室气体CO2浓度升高导致的全球变暖及一系列其他生态问题已引起世界各国的高度关注, 成为科学研究领域的热点问题(
土壤呼吸温度敏感性的影响因素和不确定性
2
2011
... 温度和水分等环境因子通过土壤生物和底物间接影响土壤呼吸的温度敏感性(
... ).总之, 土壤含水量相对适宜的时候温度敏感性最高, 而土壤温度敏感性在含水量较低或较高时都会发生变化, 变化方向和幅度与土壤类型紧密相关(
Microbial soil respiration and its dependency on carbon inputs, soil temperature and moisture
1
2007
... 研究表明生物质炭添加明显影响原位土壤有机碳矿化(
基于小观察窗技术的竹林地下系统动态研究
1
2006
... 研究区域位于浙江省杭州市富阳区国家林业局钱江源森林生态系统定位研究站庙山坞林区, 地理位置为119.93°-120.03° E, 30.05°-30.10° N.庙山坞自然保护林区面积约536.7 hm2, 核心区面积348.8 hm2, 海拔11-536 m.保护区内土壤类型有红壤、黄红壤、幼红壤, 红壤面积约占保护区面积的61%, 土壤pH值平均为5.72.研究区具有典型的亚热带季风气候特征, 年平均气温为16.9 ℃, 最热月平均气温25.4 ℃, 最冷月平均气温4.5 ℃ (
Positive and negative carbon mineralization priming effects among a variety of biochar-amended soils
2
2011
... 研究表明生物质炭添加明显影响原位土壤有机碳矿化(
... 土壤激发效应是指各种有机质添加等处理引起土壤有机质周转强烈的短期改变(
