


Cloning and Functional Analysis of Salt Stress Response Gene GhPEAMT1 in Upland Cotton
WANG Na


通讯作者:
责任编辑: 李莉
收稿日期:2020-06-13接受日期:2020-08-27网络出版日期:2021-01-16
基金资助: |
Received:2020-06-13Accepted:2020-08-27Online:2021-01-16
作者简介 About authors
王娜,E-mail:

摘要
关键词:
Abstract
Keywords:
PDF (6735KB)元数据多维度评价相关文章导出EndNote|Ris|Bibtex收藏本文
本文引用格式
王娜, 赵资博, 高琼, 何守朴, 马晨辉, 彭振, 杜雄明. 陆地棉盐胁迫应答基因GhPEAMT1的克隆及功能分析[J]. 中国农业科学, 2021, 54(2): 248-260 doi:10.3864/j.issn.0578-1752.2021.02.002
WANG Na, ZHAO ZiBo, GAO Qiong, HE ShouPu, MA ChenHui, PENG Zhen, DU XiongMing.
开放科学(资源服务)标识码(OSID):
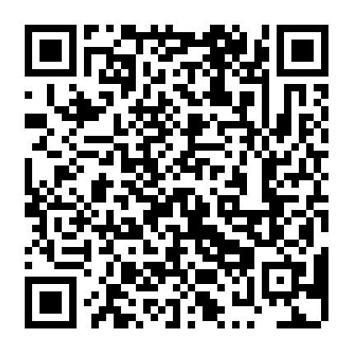
0 引言
【研究意义】棉花作为世界上最重要的天然纤维作物和最重要的经济作物,既是纺织工业的主要原料,也是国防、医药、化工等工业部门的原料[1,2]。土壤盐渍化是全球面临的重大问题,由于人类的活动,农作物的生长受到了土壤盐渍条件的限制,严重降低了农作物的产量,非生物胁迫每年造成数亿美元的损失,严重地威胁着农业的可持续性发展[3,4,5]。棉花是中度耐盐作物,它的盐容忍阈值为7.7 ds·S-1[6,7]。虽然棉花是耐盐作物,但是盐碱地高浓度的盐分依然制约着棉花的产量[8]。因此,解析植物盐胁迫的响应机制可有助于提高作物的耐盐性[7]。【前人研究进展】棉花渗透调节盐胁迫条件下,外界渗透势降低容易造成根系细胞脱水,失去膨压,进而叶片萎蔫影响棉花的生理功能。因此,棉花的耐盐机理之一,是在盐胁迫条件下能对渗透胁迫进行调节[3]。棉花渗透调节有2种方式:一种方式是吸收大量的无机盐离子积累到液泡中,提高浓度,降低水势,使其能在较高的渗透条件下吸水,保持膨压,正常生长,但这种耐胁迫能力有限,毕竟Na+在胞质积累过度会造成离子毒害;另一种方式主要依靠棉花体内合成一些小分子有机物质如脯氨酸、甜菜碱、甘油、甘露醇、海藻糖等来提高细胞的渗透压,降低水势。防止细胞脱水和保持细胞各种酶在高盐环境下的功能[9]。目前,已知的有机渗透调节物质中,甜菜碱是最重要的一种。甜菜碱是一种水溶性生物碱,是甘氨酸甜菜碱(glycinebetaine,GB)的简称,广泛存在于植物中,它的积累可以增强植物的抗性[10,11]。此外甜菜碱合成后几乎不再被进一步代谢,属于永久性或半永久性渗透调节剂,因此,甜菜碱被认为是最有希望的渗透保护剂,在植物抗盐、耐旱研究中已越来越受到重视。陈少良等[12]研究发现,生长于中国西北盐碱干旱地区的胡杨,在100 mmol·L-1 NaCl处理下叶中甜菜碱浓度增加243倍,根中增加9倍。在高等植物中,合成甘氨酸甜菜碱(GB)的底物是胆碱(choline,Cho),磷酸胆碱(phosphocholine,P-Cho)在磷酸胆碱磷酸酶的水解作用下可生成胆碱(Cho)和磷脂酸(phos- phatidic acid,PA)[13]。磷酸胆碱(P-Cho)是由磷酸乙醇胺N-甲基转移酶(phosphoethanolamine N-methyltransferse,PEAMT)通过连续3次甲基化生成,PEAMT的连续3次的催化被认为是磷酸胆碱(P-Cho)合成中的限速步骤[14]。研究发现在高等植物中胆碱(Cho)的合成速度受到严格控制,而磷酸乙醇胺N-甲基转移酶在甜菜碱的底物胆碱的合成中起到重要作用[15,16],因此,PEAMT在植物的耐盐胁迫调控中具有重要的作用。【本研究切入点】近年来,研究表明磷酸乙醇胺N-甲基转移酶(PEAMT)促进GlyBet的合成,有助于提高植物抗盐的能力。然而目前棉花中PEAMT基因的研究较少。基于PENG等[17]发表的转录组和蛋白组数据,发现GhPEAMT1无论在mRNA水平还是蛋白质水平均表达量较高且在耐盐性不同材料差异显著。因此,研究GhPEAMT1的功能,进一步解析它在棉花中的耐盐机制。【拟解决的关键问题】本研究通过克隆磷酸乙醇胺N-甲基转移酶基因(GhPEAMT1A和GhPEAMT1D)cDNA全长,并对核酸序列和蛋白质序列进行生物信息学分析,以及亚细胞定位载体、基因超表达载体和基因沉默载体的构建,进而对其生物学功能进行验证,确定GhPEAMT1的功能,为解析GhPEAMT1在棉花盐胁迫应答中的调控机制提供科学依据,同时也可为棉花耐盐品种的选育提供基因资源。1 材料与方法
1.1 试验材料
试验材料为陆地棉品种早熟长绒7号(耐盐)和南丹巴地大花(感盐)。这些材料的种子均由中国农业科学院棉花研究所国家棉花种质资源中期库提供,并经过多代自交,且性状稳定。拟南芥哥伦比亚野生型(Col-0)和本氏烟草由中国农业科学院棉花研究所种质资源课题组提供。1.2 总RNA的提取和cDNA的制备
选取陆地棉耐盐品种早熟长绒7号和感盐品种南丹巴地大花。通过霍格兰营养液培养,在苗期三叶一心期用200 mmol·L-1 NaCl溶液处理,分别在处理前(0 h)和处理后0.5、4、12、24和72 h取棉花的叶片和根,3个生长一致的棉花植株混合取样,-80℃保存备用。采用天根生化科技(北京)有限公司的多酚多糖植物总RNA提取试剂盒(NO.DP441),提取经过处理后的根和叶中的RNA。用TaKaRa公司的PrimeScriptTM RT Reagent Kit with gDNA Eraser(Perfect real time)试剂盒(NO.RR047A),将提取的RNA反转录为cDNA,用于后续试验。1.3 GhPEAMT1的克隆
根据GhPEAMT1A(Gh_A03G080)和GhPEAMT1D(Gh_D02G1225)的基因序列,利用Primer Premier 5软件设计特异引物(表1)。利用TOYOBO的KOD-Plus-Neo高保真酶进行PCR扩增,得到基因片段。纯化后,连接到Blunt-zero载体上,并转化Trans1-T1大肠杆菌感受态中。筛选阳性单克隆,送深圳华大基因科技有限公司测序,获得正确的GhPEAMT1A和GhPEAMT1D片段。吸取测序正确的菌液20 μL加入含有20 mL LB(Kan+)液体培养基中,37℃过夜培养,用北京全式金生物技术有限公司的质粒小提试剂盒提取质粒。Table 1
表1
表1研究中使用的引物
Table 1
引物名称Primer name | 正向引物Forward primer (5′-3′) | 反向引物Reverse primer (5′-3′) |
---|---|---|
GhActin | ATCCTCCGTCTTGACCTTG | TGTCCGTCAGGCAACTCAT |
GhPEAMT1A | GCTCTAGAATGGCGGCTAACGGATTT | CCGGGATCCTTAATTCTTCTTCTTCTTGGC |
GhPEAMT1D | GCTCTAGAATGGCGGCTAACGGATTT | CGGGATCCTTAGTTCTTCTTCTTGGC |
qPEAMT1A | GAGCACAGGAGGAATTGCAG | TTACAGAGAGGTCGTTGCCC |
qPEAMT1D | GAGCACAGGAGGAATTGCAG | GGTCGATGCCCACAACATGA |
CPEAMT1A | ACGCGTCGACGCGGCTAACGGATTTGTA | GTCGACTTAATTCTTCTTCTTCTTGGC |
CPEAMT1D | ACGCGTCGACGCGGCTAACGGATTTGTAGG | GTCGACTTAGTTCTTCTTCTTGGCAAGG |
VPEAMT1A | GCTCTAGAATGGCGGCTAACGGATTTGT | CGGGATCCATAATGCCCATTGATGGTTT |
VPEAMT1D | GCTCTAGATCTTGCTTTCATCAATCTGG | CGGGATCCAAAGACACGCTCATAACG |
新窗口打开|下载CSV
1.4 生物信息学分析
用Protparam(https://web.expasy.org/protparam/)分析GhPEAMT1氨基酸的理化性质;用CDD(https:// www.ncbi.nlm.nih.gov/Structure/cdd/wrpsb.cgi)在线软件预测蛋白质的保守结构域;用Signal(http://www.cbs. dtu.dk/services/SignalP/)进行跨膜结构的分析;用CELLO V.2.5(http://cello.life.nctu.edu.tw/)在线软件对蛋白质进行亚细胞定位预测;用DNAMAN软件进行基因序列比对;运用MEGA7软件对陆地棉基因GhPEAMT1及其他物种同源基因构建进化树。1.5 GhPEAMT1的表达模式分析
为了研究GhPEAMT1不同组织中的表达模式,使用Primer Premier 5设计特异引物(表1)。使用LightCycler480Ⅱ(Roche,德国)对1.2方法获得的NaCl胁迫的不同时期cDNA样本进行qRT-PCR试验。按照北京全式金生物技术有限公司的荧光定量试剂盒(TranStart Top Green qPCR SuperMix,AQ131)说明书确定qRT-PCR的反应体系和反应程序。内参基因为GhActin(AY305733),每个反应设置3个重复。采用2-ΔΔCt计算基因的相对表达量。1.6 GhPEAMT1亚细胞定位载体的构建
分别以CPEAMT1A-F、CPEAMT1A-R和CPEAMT1D-F、CPEAMT1D-R为引物(表1)和1.3中提取的质粒为模板进行PCR扩增。用限制性内切酶SalⅠ37℃酶切,按照NEB的小牛肠碱性磷酸酶(CIP,#M0290S)的步骤对酶切产物进行碱性磷酸化,回收,16℃过夜连接到表达载体P438-GFP中。将构建好的表达载体通过冻融法转入农杆菌GV3101中,得到亚细胞定位载体P438-GFP-GhPEAMT1A和P438-GFP- GhPEAMT1D。1.7 GhPEAMT1过表达载体的构建及拟南芥的遗传转化
扩增GhPEAMT1A和GhPEAMT1D的CDS片段,用限制性内切酶XbaⅠ和BamHⅠ对目的片段和表达载体PBI121进行双酶切,然后连接,重组得到过表达载体PBI121-GhPEAMT1A和PBI121-GhPEAMT1D。使用冻融法将其转入农杆菌GV3101,筛选阳性单克隆菌。通过花序浸染法浸染拟南芥,收获的种子即是T0代。把消毒后的种子,平铺在含有卡那霉素抗性的MS培养基上,在培养箱中培养约15 d后,获得拟南芥阳性苗。把阳性苗种植到营养土中,待拟南芥长到7—8片叶片,取叶片进行PCR检测,确定目的基因是否转入拟南芥中,此时为T1代拟南芥。继续种植,最后得到纯合的T3代。1.8 转基因拟南芥种子盐胁迫处理
将拟南芥种子在0.1%的HgCl2溶液中振荡浸泡4 min,灭菌水清洗6次后,分别把转基因拟南芥种子和野生型拟南芥种子点种在含不同浓度NaCl(0、50、100、150和200 mmol·L-1)的MS培养基中,4℃,春化48 h后,放入23℃的光照培养箱中,16 h光照/8 h黑暗萌发,生长10 d后观察统计拟南芥种子的萌发率,然后选取生长一致的野生型拟南芥和转基因拟南芥转移到不同浓度NaCl的MS培养基中,垂直置于恒温培养箱中培养,一周后统计根长,试验重复3次。1.9 病毒诱导GhPEAMT1沉默载体的构建
基因沉默载体的构建选用的载体是TRV2,选取特异的目的片段,片段大小为300 bp,酶切位点为XbaⅠ和BamHⅠ。用Primer Premier5软件设计引物为VPEAM1TA-F、VPEAMT1A-R和VPEAMT1D-F、VPEAMT1D-R(表1)。以1.4提取的质粒为模板进行PCR扩增,用限制性内切酶XbaⅠ和BamHⅠ对目的片段进行酶切,然后连接到TRV2沉默载体上,测序正确导入农杆菌LBA4404中,挑取单克隆,验证条带大小,得到基因沉默载体TRV2::GhPEAMT1A和TRV2::GhPEAMT1D。1.10 GhPEAMT1沉默棉花植株的盐胁迫处理
把TRV2::GhPEAMT1A、TRV2::GhPEAMT1D、TRV2:00、CLA1和辅助菌液在含有卡那霉素和利福平的LB液体培养基中置于28℃的摇床里过夜培养(约16 h),菌液离心收集菌体,用重悬液(MES、MgCl2、乙酰丁香酮)重悬,测OD值为1.0—1.2,把辅助载体重悬液和各个载体的重悬液按1﹕1混合,然后在25℃黑暗环境中静置2 h。选取在24℃,16 h光照/8 h黑暗环境下生长7 d的棉花幼苗完全展开的子叶进行注射,注射之后黑暗处理24 h,置于23℃的温室继续培养。等出现白化苗后,对试验组和对照组单株取样,进行沉默效率的检测,三叶期对试验组和对照组分别进行盐处理,盐浓度为40 g·L-1,每株浇灌100 mL盐水。试验重复3次。2 结果
2.1 GhPEAMT1在转录组和蛋白质组中的表现
通过比较陆地棉耐盐品种早熟长绒7号和感盐品种南丹巴地大花苗期叶片盐胁迫诱导的转录组和蛋白组数据[17],图1-A表明,Gh_A03G0803(GhPEAMT1A)和Gh_D02G1225(GhPEAMT1D)2个基因在NaCl胁迫后4和24 h具有显著的差异表达,尤其是在24 h时表达差异最大,其中,GhPEAMT1A在早熟长绒7号中的表达差异倍数为5.22,GhPEAMT1D在早熟长绒7号中的表达差异倍数为6.71。图1-B表明,2个基因在蛋白质水平受盐胁迫4和24 h时有显著的差异表达,其中GhPEAMT1D蛋白表达差异最大,耐盐陆地棉早熟长绒7号中的表达差异倍数为4.70,感盐陆地棉南丹巴地大花中的表达倍数为3.90。表明GhPEAMT1A和GhPEAMT1D可能是耐盐相关基因。图1
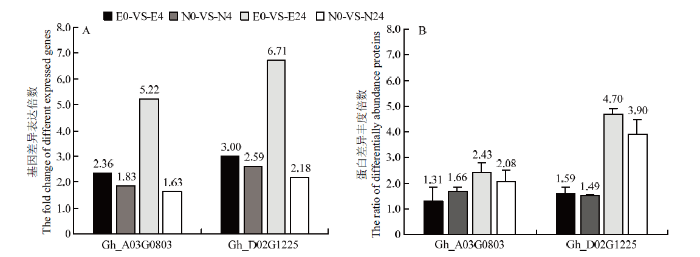
图12个耐盐性差异品种受盐胁迫后磷酸乙醇胺N-甲基转移酶基因GhPEAMT1A和GhPEAMT1D转录组和蛋白质组的差异表达
A:差异表达基因;B:差异丰度蛋白。E:早熟长绒7号;N:南丹巴地大花;0:CK;4:200 mmol·L-1 NaCl处理4 h;24:200 mmol·L-1 NaCl处理24 h。转录组数据无生物学重复,蛋白组数据有3个生物学重复
Fig. 1The differential expression ratio of the transcriptome and proteome of the two phosphoethanolamine N-methyltransferases genes GhPEAMT1A and GhPEAMT1D at 4 h and 24 h after salt stress
A: Differentially expressed genes; B: Differential abundance protein. E: Earlistape 7; N: Nandanbadidahua; 4: 200 mmol·L-1 NaCl treatment for 4 h; 24: 200 mmol·L-1 NaCl treatment for 24 h. There were no biological replicates in transcriptome data, but three in proteome data
2.2 GhPEAMT1的克隆与序列分析
用200 mmol·L-1 NaCl溶液处理后12 h的早熟长绒7号和南丹巴地大花的cDNA为模板,通过基因克隆、测序得到正确的GhPEAMT1A和GhPEAMT1D的CDS序列,经分析,这两个基因的CDS区核酸序列分别在2个品种中均表现无差异。GhPEAMT1A的CDS全长为1 488 bp(图2-A),编码495个氨基酸,蛋白质分子式为C2528H3924N670O749S19,相对分子质量为56.296 kD,理论等电点为6.27,具有亲水性,不具有跨膜结构。GhPEAMT1D的CDS全长为1 485 bp(图2-B),编码494个氨基酸,蛋白质的分子式为C2520H3906N662O746S19,相对分子质量为56.021 kD,理论等电点为6.02,具有亲水性,不具有跨膜结构。通过保守结构域分析,GhPEAMT1都具有2个高度保守甲基转移酶结构域(PF08241和PF13489)(图2-C)。图2
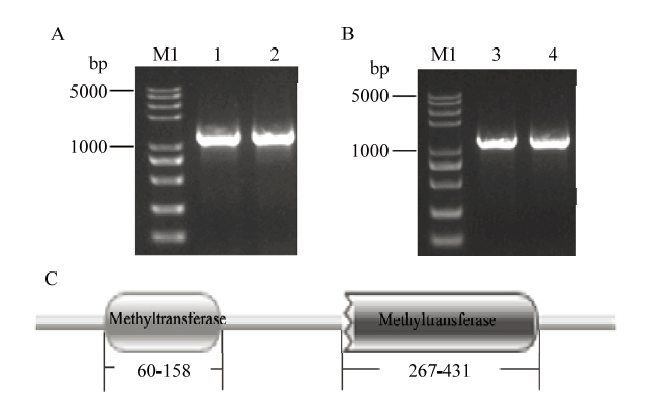
图2GhPEAMT1克隆及保守结构域分析
A:GhPEAMT1A克隆电泳图;B:GhPEAMT1D克隆电泳图;C:GhPEAMT1蛋白保守结构域分析;M1:Trans2K?Plus II Marker;1和3:早熟长绒7号;2和4:南丹巴地大花
Fig. 2GhPEAMT1 gene cloning and analysis of conserved domain
A: GhPEAMT1A clone electrophoresis; B: GhPEAMT1D clone electrophoresis; C: Analysis of conserved domain of GhPEAMT1 protein; M1: Trans2K? Plus II Marker; 1&3: Earlistable 7; 2&4: Nandanbadidahua
将陆地棉GhPEAMT1A的氨基酸序列与亚洲棉(Gossypium arboreum,XM_017755899)、海岛棉(Gossypium barbadense,XM_016811336、XM_ 016835662)、雷蒙德氏棉(Gossypium raimondii,XM_012623073)、拟南芥(Arabidopsis thaliana,NP_188427.2、NP_973993.1)、水稻(Oryza sativa,XM_015785333、XP_015622327)、玉米(Zea mays,NP_001307897.2)、可可(Theobroma cacao,XM_ 007036740)和杨树(Populus trichocarpa,XM_ 024582451)进行序列比对,发现GhPEAMT1的氨基酸序列与以上物种存在高度保守区域(图3-A),进化树分析表明GhPEAMT1与海岛棉同源基因的亲缘关系最近,同源性较高,而棉种与其他物种相比,与可可同源性最高(图3-B)。
图3
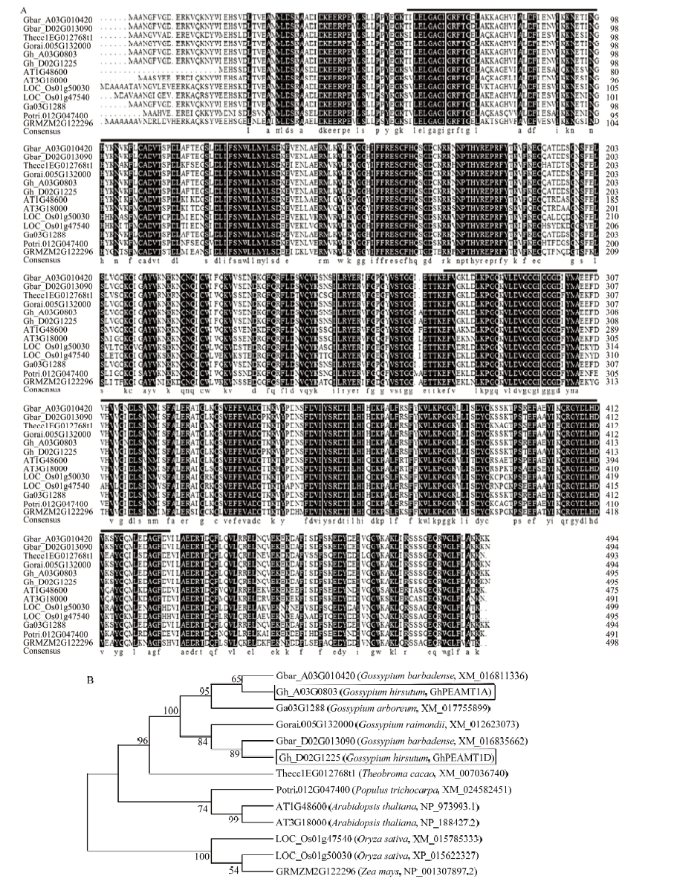
图3GhPEAMT1氨基酸序列比对和进化树分析
A:GhPEAMT1氨基酸的多序列比对。Gbar_A03G010420:海岛棉,XM_016811336;Gbar_D02G013090:海岛棉,XM_016835662;Thecc1EG012768t1:可可,XM_007036740;Gorai.005G132000:雷蒙德氏棉,XM_012623073;Gh_A03G0803:陆地棉,GhPEAMT1A;Gh_D02G1225:陆地棉,GhPEAMT1D;AT1G48600:拟南芥,NP_973993.1;AT3G18000:拟南芥,NP_188427.2;LOC_Os01g47540:水稻,XM_015785333;LOC_Os01g50030:水稻,XP_015622327;Ga03G1288:亚洲棉,XM_017755899;Potri.012G047400:杨树,XM_024582451;GRMZM2G122296:玉米,NP_001307897.2;黑色线段为保守结构域的位置;B:GhPEAMT1的系统进化分析;黑色方框表示目的基因
Fig. 3Amino acid sequence alignment and phylogenetic tree analysis for gene GhPEAMT1
A: Multiple sequence alignment of GhPEAMT1. Gbar_A03G010420: Gossypium barbadense, XM_016811336; Gbar_D02G013090: Gossypium barbadense, XM_016835662; Thecc1EG012768t1: Theobroma cacao, XM_007036740; Gorai.005G132000: Gossypium raimondii, XM_012623073; Gh_A03G0803: Gossypium hirsutum, GhPEAMT1A; Gh_D02G1225: Gossypium hirsutum, GhPEAMT1D; AT1G48600: Arabidopsis thaliana, NP_973993.1; AT3G18000: Arabidopsis thaliana, NP_188427.2; LOC_Os01g47540: Oryza sativa, XM_015785333; LOC_Os01g50030: Oryza sativa, XP_015622327; Ga03G1288: Gossypium arboreum, XM_017755899; Potri.012G047400: Populus trichocarpa, XM_024582451; GRMZM2G122296: Zea mays, NP_001307897.2; The black line indicates the position of conserved domain; B: Phylogenetic analysis of GhPEAMT1; The black box indicates the target gene
2.3 GhPEAMT1的表达特征分析
通过对经NaCl溶液不同处理时间棉花中GhPEAMT1表达的分析(图4),结果表明,不同的棉花品种在不同组织和时期GhPEAMT1A和GhPEAMT1D的表达量呈不同程度上调,但是表达特征有所不同。在12和24 h时,耐盐棉花品种早熟长绒7号中的GhPEAMT1A和GhPEAMT1D表达量显著高于南丹巴地大花,且24 h表达量达到最高;相对于叶片中的表达趋势,在根中有所区别。2个基因在早熟长绒7号根中受NaCl胁迫12 h表达量达到最大值,整体表现为先升后降。感盐品种南丹巴地大花的表达量在4、12和24 h显著低于早熟长绒7号。因此,推测2个基因在耐盐品种中响应盐胁迫过程中起重要作用。图4
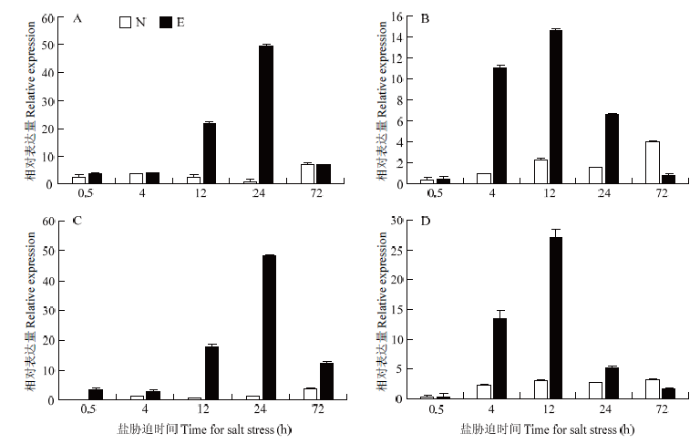
图4GhPEAMT1在棉花组织中的表达特征分析
A:GhPEAMT1A在早熟长绒7号和南丹巴地大花叶片中的表达;B:GhPEAMT1A在早熟长绒7号和南丹巴地大花根中的表达;C:GhPEAMT1D在早熟长绒7号和南丹巴地大花叶片中的表达;D:GhPEAMT1D在早熟长绒7号和南丹巴地大花根中的表达。E:早熟长绒7号;N:南丹巴地大花
Fig. 4Analysis of the expression characteristics of GhPEAMT1 gene in cotton tissues
A: The expression of GhPEAMT1A gene in the leaves of Earlistape 7 and Nandanbadidahua; B: Expression of GhPEAMT1A gene in roots of Earlistape 7 and Nandanbadidahua; C: Expression of GhPEAMT1D gene in leaves of Earlistape 7 and Nandanbadidahua; D: GhPEAMT1D gene in early expression in roots of Earlistape 7 and Nandanbadidahua. E: Earlistape 7; N: Nandanbadidahua
2.4 陆地棉GhPEAMT1的亚细胞定位分析
运用CELLOV.2.5软件预测GhPEAMT1位于细胞质中,通过激光共聚焦扫描显微镜观察,发现空载体在细胞膜、细胞质和细胞核中均能检测到绿色荧光蛋白,而目的基因融合蛋白在细胞质中检测到绿色荧光蛋白(图5),说明GhPEAMT1A和GhPEAMT1D位于细胞质中。图5
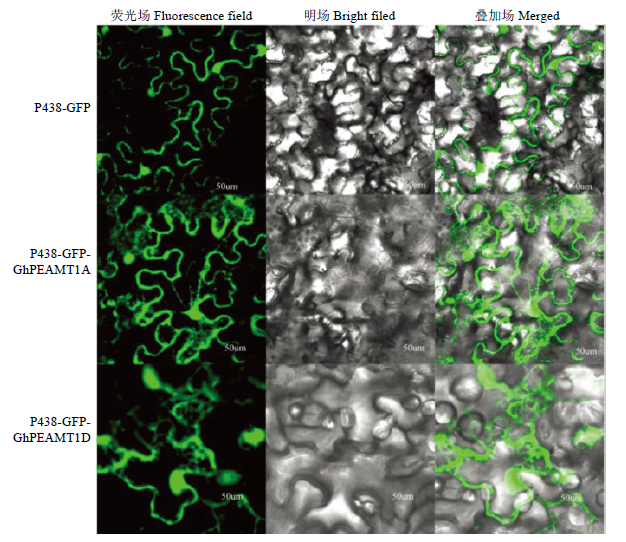
图5GhPEAMT1A和GhPEAMT1D亚细胞定位
Fig. 5GhPEAMT1A and GhPEAMT1D gene subcellular localization
2.5 转基因拟南芥的获得与鉴定
通过花序浸染法得到转基因拟南芥种子,用MS培养基(Kan+)筛选得到阳性植株,使用基因特异引物对筛选出的阳性植株进行PCR鉴定,去除假阳性植株,为了得到纯合的单拷贝阳性植株,继续筛选阳性植株与非阳性植株比值为3﹕1的株系,直至得到纯合株系。获得9株转GhPEAMT1A阳性植株(图6-A),获得5株转GhPEAMT1D阳性植株(图6-B),通过RT-PCR分析,GhPEAMT1A和GhPEAMT1D在转基因植株中均能正常表达,在野生型拟南芥中未检测到转录信号(图6-C)。图6
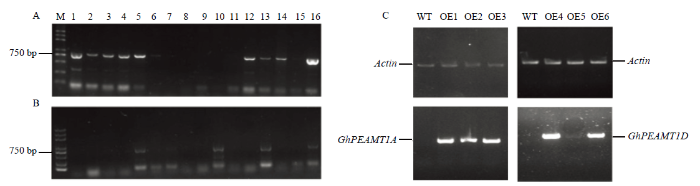
图6转基因拟南芥的获得
A:转基因拟南芥植株GhPEAMT1A的PCR检测,M:Marker;1—16:转基因植株;B:转基因拟南芥植株GhPEAMT1D的PCR检测;C:转基因拟南芥植株GhPEAMT1的表达鉴定;WT:野生型拟南芥;OE1—OE6:转基因拟南芥植株
Fig. 6Obtaining transgenic Arabidopsis
A: PCR detection transgenic Arabidopsis plants GhPEAMT1A, M: Marker; 1-16: Transgenic plants; B: PCR detection transgenic Arabidopsis plants GhPEAMT1D, C: Expression and identification of GhPEAMT1 in transgenic Arabidopsis plants; WT: Wild type Arabidopsis; OE1-OE6: Transgenic Arabidopsis plants
2.6 转基因拟南芥的耐盐性分析
为了进一步研究GhPEAMT1的耐盐性,通过对WT、转GhPEAMT1A拟南芥和转GhPEAMT1D拟南芥在不同浓度NaCl(0、50、100、150和200 mmol·L-1)处理下进行萌发率和根长的数据统计(图7)。0 mmol·L-1 NaCl浓度时野生型与转基因拟南芥的萌发率和主根长无显著差异;50 mmol·L-1 NaCl浓度时野生型拟南芥萌发率无显著差异,主根长有显著差异,但能正常生长;100 mmol·L-1 NaCl浓度时野生型拟南芥萌发率显著低于转基因拟南芥,野生型拟南芥的主根显著长于野生型拟南芥;150 mmol·L-1 NaCl浓度时野生型拟南芥的萌发率显著低于转基因拟南芥的萌发率,野生型拟南芥叶片发黄逐渐死去,转基因拟南芥叶片发黄且主根长度较短。图7
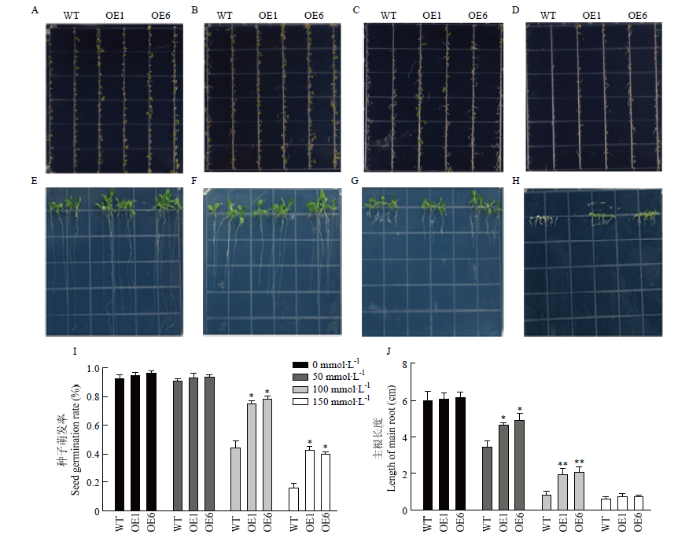
图7盐胁迫对拟南芥幼苗生长的影响
A—D:0、50、100和150 mmol·L-1 NaCl处理下拟南芥萌发率情况;E—H:0、50、100和150 mmol·L-1 NaCl处理下拟南芥根伸长情况;I:不同NaCl浓度下拟南芥的萌发率;J:不同NaCl浓度下拟南芥的主根长度。*:差异显著(P<0.05),**:差异极显著(P<0.01)。下同
Fig. 7Effect of Salt Stress on seedling growth in Arabidopsis
A-D: Germination rate of Arabidopsis under NaCl treatment of 0, 50, 100 and 150 mmol·L-1; E-H: Root elongation of Arabidopsis thaliana treated with 0, 50, 100 and 150 mmol·L-1 NaCl; I: Germination rate (%) of Arabidopsis under different NaCl concentrations; J: Main root length of Arabidopsis under different NaCl concentration. *: Significant difference (P<0.05), **: Extremely significant difference (P<0.01). The same as below
2.7 GhPEAMT1沉默后的功能分析
对陆地棉早熟长绒7号中的GhPEAMT1A和GhPEAMT1D进行沉默。棉花中与叶绿素合成相关基因CLA1发生沉默,棉花的叶绿素合成受到干扰,后期新出现的真叶表现为白化[18]。侵染棉花植株后观察表型,当出现白化表明基因沉默成功(图8-A)。然后单株取棉花的叶片,通过实时荧光定量(qRT-PCR)验证对照(CK)、TRV2:00、GhPEAMT1A和GhPEAMT1D沉默后棉花植株的表达量,CK棉花植株和TRV2:00棉花植株表达量无显著变化,目的基因沉默后棉花植株的表达量显著降低,结果表明,GhPEAMT1成功沉默(图8-B)。用40 g·L-1 NaCl溶液对基因沉默棉花植株、TRV2:00棉花植株和CK棉花植株进行盐胁迫处理。观察盐处理后的表型发现盐胁迫48 h后,棉花GhPEAMT1沉默的植株比对照植株萎蔫严重(图8-C和图8-D)。图8
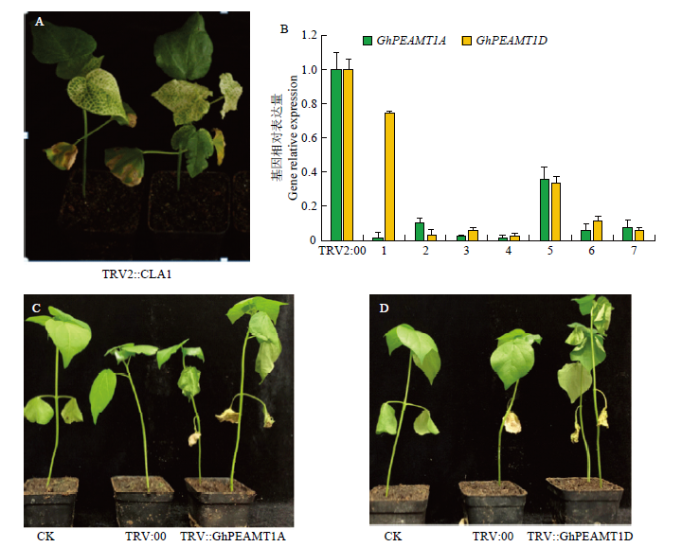
图8GhPEAMT1沉默效率和盐处理后的表型
A:沉默标记基因TRV2:CLA植株;B:病毒诱导基因沉默后,GhPEAMT1A/D沉默效率分析,1—7:注射TRV2::GhPEAMT1A、TRV2::GhPEAMT1D菌株的株系;C—D:盐胁迫48 h后,CK、TRV2:00、TRV2::GhPEAMT1A、TRV2::GhPEAMT1D棉花幼株表型
Fig. 8GhPEAMT1 gene silencing efficiency and phenotype after salt treatment
A: Silence marker gene TRV2: CLA plants; B: Analysis of GhPEAMT1 gene silencing efficiency after virus-induced gene silencing, abscissas 1-7: Strains injected with TRV2::GHPEAMT1A, TRV2::GhPEAMT1D strains; C-D: 48 h after salt stress, CK, TRV2:00, TRV2::GHPEAMT1A, TRV2::GHPEAMT1D phenotype of cotton young plants
盐胁迫后,注射TRV2::GhPEAMT1A棉花植株与注射空载棉花植株相比过氧化氢酶(CAT)活性降低、谷胱甘肽过氧化物酶(GPX)活性降低(图9-A和图9-B)。沉默TRV2::GhPEAMT1D棉花植株TRV2:00相比CAT、GPX活性都略微升高,但没有显著差异(图9-A和图9-B),由此推测沉默GhPEAMT1A后,CAT、GPX的活性降低,直接影响活性氧清除系统的不同成员如CAT、GPX、POD的活性(随着胁迫时间延长,最终结果都是降低),继而表现出对盐敏感。而沉默GhPEAMT1D后对活性氧清除系统没有影响,推测沉默GhPEAMT1D的植株受盐胁迫后可能影响其他代谢途径。
图9
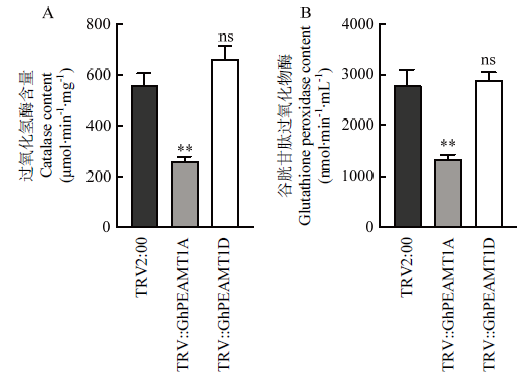
图9 GhPEAMT1沉默盐处理后酶的活性测定
A:盐胁迫48 h后,TRV2:00、TRV2::GHPEAMT1A棉花幼苗CAT的活性;B:盐胁迫48 h后,TRV2:00、TRV2::GHPEAMT1D棉花幼苗GPX的活性
Fig. 9Determination of enzyme activity after GhPEAMT1 gene silencing treatment
A: The active of CAT in TRV2:00, TRV2::GHPEAMT1A cotton seedlings after 48 h of salt stress; B: The active of GPX in TRV2:00, TRV2::GHPEAMT1A cotton seedlings after 48 h of salt stress
3 讨论
当植物受到盐胁迫时,可发生多种胁迫应答反应,例如,可以积累一些可溶性物质来提高细胞渗透压来减轻盐胁迫对植物造成的伤害[19]。植物PEAMT基因通过提高Cho的含量促进渗调物质甘氨酸甜菜碱(GB)的生成,从而增强了植物对盐胁迫或干旱胁迫的耐受能力[20]。研究表明,在转基因植物中GB含量低在很大程度上是由于Cho合成能力不足[21]。其中磷酸乙醇胺N-甲基转移酶(PEAMT)是磷酸胆碱合成的关键酶[22],因此,研究植物中的PEAMT的基因在应对盐胁迫中所起的作用显得非常重要。近年来,随着分子生物学的发展,通过基因工程技术培育和筛选耐盐性的作物得到了大力的发展。截至目前,研究人员已克隆、鉴定出一批与耐盐相关的基因,并研究了抗逆境功能,通过遗传转化还获得了一些转基因株系。比如,用NaCl处理菠菜叶片后,PEAMT的酶活都高于未处理的[23];PEEL等[11]克隆了玉米中的PEAMT基因在盐碱胁迫下的玉米叶片,PEAMT酶的活性增加了400%;LI等[24]在辽宁蓬碱中克隆了PEAMT基因,在盐胁迫后,基因的表达量升高;对大麦的根中进行了PEAMT基因转录调控的分析,在24 h和3 d上调表达[25];在无隐芒子中克隆出CsPEAMT,干旱胁迫诱导8 d时,在叶和根中表达量分别是未干旱对照的43.35倍和13.25倍,表明CsPEAMT可能是无芒隐子草抗旱性相关的基因[26]。过量表达PEAMT基因的转基因植株通过增加胆碱和GB的合成来提高自身对盐胁迫的耐受能力[14,27],表明PEAMT基因参与植物对非生物逆境的响应。MOU等[27]研究表明,拟南芥peamt突变体t365的Cho生物合成量减少约64%,从而导致突变体植株对盐渍高度敏感。目前,PEAMT基因在棉花中研究比较少。本研究在转录组学和蛋白质组学数据分析的基础上筛选到的该耐盐相关的候选基因。前人的研究表明不同植物PEAMT基因的mRNA转录水平受到盐胁迫诱导上调表达[28,29]。WU等[30]通过对玉米ZmPEAMT1进行实时定量PCR分析,表明受到盐胁迫的诱导和高温抑制;MOU等[27]对盐角草ShPEAMT启动子区分析含有脱落酸(ABA)诱导元件、热激应答元件和低温诱导元件等顺式调控元件,表明ShPEAMT在转录水平上可能受赤霉素、低温、光、ABA与热激的调控,这和盐草角适应其恶劣多变的环境相关。本研究对盐耐性不同的棉花品种进行表达特征分析表明在受到盐胁迫后根和叶在12和24 h表达量最高,且在耐盐棉花品种中的表达量高于感盐品种,因此,推测陆地棉GhPEAMT1在植物耐盐中有着重要作用。继而对目的基因GhPEAMT1的功能进行验证,通过转基因过表达拟南芥与野生型相比,在100 mmol·L-1 NaCl胁迫下萌发率和主根长显著较高;通过VIGS技术沉默GhPEAMT1A后陆地棉的抗氧化能力相比对照植株下降,由此推测GhPEAMT1A在棉花在盐胁迫响应中能提高抗氧化能力,继而表现对盐胁迫的耐受性。
土壤盐渍化已经严重影响到世界范围内不少作物的产量等性状。而当前利用基因工程手段来提高作物的耐盐性是解决这一世界难题的有效策略之一。也有大量研究表明向作物转入与耐盐相关的基因可以在一定程度提高作物的耐盐性。本研究通过转基因拟南芥,提高了耐盐性;通过沉默该基因导致棉花沉默株系对盐敏感,都证明该基因GhPEAMT1A是耐盐相关基因,这为进一步棉花基因工程育种,培育棉花抗逆新品系提供理论和试验依据。
4 结论
从陆地棉中克隆了2个同源基因GhPEAMT1A和GhPEAMT1D,其CDS长度分别为1 488和1 485 bp,编码495和494个氨基酸,推导的多肽均包含2个高度保守甲基转移酶结构域。GhPEAMT1不具有跨膜结构,在进化中功能高度保守。陆地棉GhPEAMT1A和GhPEAMT1D的表达在盐胁迫后被诱导,推测GhPEAMT1在响应盐胁迫过程中起正向调控作用。参考文献 原文顺序
文献年度倒序
文中引用次数倒序
被引期刊影响因子
URL [本文引用: 1]
[本文引用: 1]
[本文引用: 1]
[本文引用: 1]
[本文引用: 2]
URL [本文引用: 1]
URLPMID:16309626 [本文引用: 1]
[本文引用: 1]
[本文引用: 2]
DOI:10.1016/j.tplants.2014.02.001URL [本文引用: 1]

Crop performance is severely affected by high salt concentrations in soils. To engineer more salt-tolerant plants it is crucial to unravel the key components of the plant salt-tolerance network. Here we review our understanding of the core salt-tolerance mechanisms in plants. Recent studies have shown that stress sensing and signaling components can play important roles in regulating the plant salinity stress response. We also review key Na+ transport and detoxification pathways and the impact of epigenetic chromatin modifications on salinity tolerance. In addition, we discuss the progress that has been made towards engineering salt tolerance in crops, including marker-assisted selection and gene stacking techniques. We also identify key open questions that remain to be addressed in the future.
[本文引用: 1]
[本文引用: 1]
[本文引用: 1]
URLPMID:20004921 [本文引用: 2]
[本文引用: 1]
[本文引用: 1]
[本文引用: 1]
DOI:10.1074/jbc.M109.022657URLPMID:19762471 [本文引用: 2]

In plants the triple methylation of phosphoethanolamine to phosphocholine catalyzed by phosphoethanolamine N-methyltransferase (PEAMT) is considered a rate-limiting step in the de novo synthesis of phosphatidylcholine. Besides being a major membrane phospholipid, phosphatidylcholine can be hydrolyzed into choline and phosphatidic acid. Phosphatidic acid is widely recognized as a second messenger in stress signaling, and choline can be oxidized within the chloroplast to yield the putative osmoprotectant glycine betaine. Here we describe the cloning and biochemical characterization of a second wheat PEAMT isoform that has a four times higher specific activity than the previously described WPEAMT/TaPEAMT1 enzyme and is less sensitive to product inhibition by S-adenosyl homocysteine, but more sensitive to inhibition by phosphocholine. Both enzymes follow a sequential random Bi Bi mechanism and show mixed-type product inhibition patterns with partial inhibition for TaPEAMT1 and a strong non-competitive component for TaPEAMT2. An induction of TaPEAMT protein expression and activity is observed after cold exposure, ahead of an increase in gene expression. Our results demonstrate direct repression of in vitro enzymatic activities by phosphatidic acid for both enzymes, with TaPEAMT1 being more sensitive than TaPEAMT2 in the physiological concentration range. Other lipid ligands identified in protein-lipid overlays are phosphoinositide mono- as well as some di-phosphates and cardiolipin. These results provide new insights into the complex regulatory circuits of phospholipid biosynthesis in plants and underline the importance of head group biosynthesis in adaptive stress responses.
URLPMID:12228655 [本文引用: 1]
DOI:10.1104/pp.71.3.692URLPMID:16662890 [本文引用: 1]

Like other chenopods, sugarbeets (Beta vulgaris L. cv Great Western D-2) accumulate glycine betaine when salinized; this may be an adaptive response to stress. The pathway of betaine synthesis in leaves of salinized (150-200 millimolar NaCl) sugarbeet plants was investigated by supplying [(14)C]formate, phosphoryl[(14)C]monomethylethanolamine ([(14)C][unk] MME) or phosphoryl[(14)C]choline ([(14)C][unk] choline) to leaf discs and following (14)C incorporation into prospective intermediates. The (14)C kinetic data were used to develop a computer model of the betaine pathway.When [(14)C]formate was fed, [unk] MME, phosphoryldimethylethanolamine ([unk] DME) and [unk] choline were the most prominent methylated products at short labeling times, after which (14)C appeared in free choline and in betaine. Phosphatidylcholine labeled more slowly than [unk] choline, choline, and betaine, and behaved as a minor end product. Very little (14)C entered the free methylethanolamines. When [(14)C][unk] MME was supplied, a small amount was hydrolyzed to the free base but the major fate was conversion to [unk] DME, [unk] choline, free choline, and betaine; label also accumulated slowly in phosphatidylcholine. Label from supplied [(14)C][unk] choline entered choline and betaine rapidly, while phosphatidylcholine labeled only slowly and to a small extent.These results are consistent with the pathway [unk] MME -->[unk] DME --> [unk] choline --> choline --> --> betaine, with a minor side branch leading from [unk] choline into phosphatidylcholine. This contrasts markedly (a) with the pathway of stress-induced choline and betaine synthesis in barley, in which phosphatidylcholine apparently acts as an intermediate (Hitz, Rhodes, Hanson 1981, Plant Physiol 68: 814-822); (b) with choline biogenesis in mammalian liver and microorganisms. Computer modeling of the experimental data pointed strongly to regulation at the [unk] choline --> choline step, and also indicated that the rate of [unk] choline synthesis is subject to feedback inhibition by [unk] choline.
URLPMID:29925319 [本文引用: 2]
[本文引用: 1]
DOI:10.3390/ijms14046757URLPMID:23531537 [本文引用: 1]

The review is focused on plant proteome response to salinity with respect to physiological aspects of plant salt stress response. The attention is paid to both osmotic and ionic effects of salinity stress on plants with respect to several protein functional groups. Therefore, the role of individual proteins involved in signalling, changes in gene expression, protein biosynthesis and degradation and the resulting changes in protein relative abundance in proteins involved in energy metabolism, redox metabolism, stress- and defence-related proteins, osmolyte metabolism, phytohormone, lipid and secondary metabolism, mechanical stress-related proteins as well as protein posttranslational modifications are discussed. Differences between salt-sensitive (glycophytes) and salt-tolerant (halophytes) plants are analysed with respect to differential salinity tolerance. In conclusion, contribution of proteomic studies to understanding plant salinity tolerance is summarised and discussed.
[本文引用: 1]
URLPMID:9881168 [本文引用: 1]
[本文引用: 1]
[本文引用: 1]
DOI:10.1104/pp.103.4.1269URLPMID:12232019 [本文引用: 1]

Choline metabolism was examined in spinach (Spinacia oleracea L.) plants growing under nonsaline and saline conditions. In spinach, choline is required for phosphatidylcholine synthesis and as a precursor for the compatible osmolyte glycine betaine (betaine). When control (nonsalinized) leaf discs were incubated for up to 2 h with [1,2-14C]ethanolamine, label appeared in the N-methylated derivatives of phosphoethanolamine including phosphomono-, phosphodi-, and phosphotri- (i.e. phosphocholine) methyl-ethanolamine, as well as in choline and betaine, whereas no radioactivity could be detected in the mono- and dimethylated derivatives of the free base ethanolamine. Leaf discs from salinized plants showed the same pattern of labeling, although the proportion of label that accumulated in betaine was almost 3-fold higher in the salinized leaf discs. Enzymes involved in choline metabolism were assayed in crude leaf extracts of plants. The activites of ethanolamine kinase and of the three S-adenosylmethionine:phospho-base N-methyltransferase enzymes responsible for N-methylating phosphoethanolamine to phosphocholine were all higher in extracts of plants salinized step-wise to 100, 200, or 300 mM NaCI compared with controls. In contrast, choline kinase, phosphocholine phosphatase, and cytidine 5[prime]-triphosphate: phosphocholine cytidylyltransferase activities showed little variation with salt stress. Thus, the increased diversion of choline to betaine in salt-stressed spinach appears to be mediated by the increased activity of several key enzymes involved in choline biosynthesis.
[本文引用: 1]
DOI:10.1186/2008-2231-22-26URLPMID:24495506 [本文引用: 1]

BACKGROUND: Chronic obstructive pulmonary disease (COPD) is a worldwide epidemic disease and a major cause of death and disability. The present study aimed to elucidate pharmacological effects of adipose derived stromal cells (ASCs) on pathological and biochemical factors in a guinea pig model of COPD. Guinea pigs were randomized into 5 groups including: Control, COPD, COPD + intratracheal delivery of PBS as a vehicle (COPD-PBS), COPD + intratracheal delivery of ASCs (COPD-ITASC) and COPD + intravenous injection of ASCs (COPD-IVASC). COPD was induced by exposing animals to cigarette smoke for 3 months. Cell therapy was performed immediately after the end of animal exposure to cigarette smoke and 14 days after that, white blood cells, oxidative stress indices and pathological changes of the lung were measured. RESULTS: Compared with control group, emphysema was clearly observed in the COPD and COPD-PBS groups (p < 0.001). Lung histopathologic changes of COPD-ITASC and COPD-IVASC groups showed non-significant improvement compared to COPD-PBS group. The COPD-ITASC group showed a significant increase in total WBC compared to COPD-PBS group but there was not a significant increase in this regard in COPD-IVASC group. The differential WBC showed no significant change in number of different types of leukocytes. The serum level of malondialdehyde (MDA) significantly decreased but thiol groups of broncho-alveolar lavage fluid (BALF) increased in both cell treated groups (p < 0.05 for all cases). Weight of animals decreased during smoke exposure and improved after PBS or cell therapy. However, no significant change was observed between the groups receiving PBS and the ones receiving ASCs. CONCLUSION: Cell therapy with ASCs can help in reducing oxidative damage during smoking which may collectively hold promise in attenuation of the severity of COPD although the lung structural changes couldn't be ameliorated with these pharmacological therapeutic methods.
URL [本文引用: 1]
[本文引用: 1]
DOI:10.1105/tpc.001701URLPMID:12215503 [本文引用: 3]

S-Adenosyl-L-methionine:phosphoethanolamine N-methyltransferase (PEAMT; EC 2.1.1.103) catalyzes the key step in choline (Cho) biosynthesis, the N-methylation of phosphoethanolamine. Cho is a vital precursor of the membrane phospholipid phosphatidylcholine, which accounts for 40 to 60% of lipids in nonplastid plant membranes. Certain plants use Cho to produce the osmoprotectant glycine betaine, which confers resistance to salinity, drought, and other stresses. An Arabidopsis mutant, t365, in which the PEAMT gene is silenced, was identified using a new sense/antisense RNA expression system. t365 mutant plants displayed multiple morphological phenotypes, including pale-green leaves, early senescence, and temperature-sensitive male sterility. Moreover, t365 mutant plants produced much less Cho and were hypersensitive to salinity. These results demonstrate that Cho biosynthesis not only plays an important role in plant growth and development but also contributes to tolerance to environmental stresses. The temperature-sensitive male sterility caused by PEAMT silencing may have a potential application in agriculture for engineering temperature-sensitive male sterility in important crop plants.
[本文引用: 1]
DOI:10.1007/s11103-007-9155-xURLPMID:17406792 [本文引用: 1]

From a library of 3,000 expression sequence tags (ESTs), derived from the epidermis of a diploid wheat (Triticum monococcum) inoculated with Blumeria graminis f. sp. tritici (Bgt), we cloned 23 cDNAs representing 12 genes that are involved in the pathways of biosynthesis and supply of methyl units. We studied the transcription of these genes to investigate how the methyl units are generated and regulated in response to Bgt infection and abiotic stresses in wheat. Expression of 5, 10-methylene-tetrahydrofolate reductase, methionine synthase, S-adenosylmethionine synthetase, and S-adenosylhomocystein hydrolase transcripts were highly induced at an early stage of infection. This induction was specific to the epidermis and linked to host cell wall apposition (CWA) formation, suggesting that the pathways for generation of methyl units are transcriptionally activated for the host defense response. Levels of S-adenosylmethionine decarboxylase, caffeic acid 3-O-methyltransferase, 1-aminocyclopropane-1-carboxylate oxidase mRNA, but not phosphoethanolamine N-methyltransferase and nicotianamine synthase mRNA, were up-regulated after infection and showed similar expression patterns to genes involved in the pathways of generation of methyl units, revealing possible routes of methyl transfer towards polyamine, lignin and ethylene biosynthesis rather than glycine betaine and nicotianamine in response to Bgt attack. After imposing various abiotic stresses, genes involved in the pathways of generation and supply of methyl units were also up-regulated differentially, suggesting that the generation of sufficient methyl units at an early stage might be crucial to the mitigation of multiple stresses.
DOI:10.1007/s12033-007-0009-1URLPMID:17914189 [本文引用: 1]

N-methylation of phosphoethanolamine, the committing step in choline (Cho) biosynthesis in plants, is catalyzed by S-adenosyl-L-methionine: phosphoethanolamine N-methyltransferase (PEAMT, EC 2.1.1.103). Herein we report the cloning and characterization of the novel maize phosphoethanolamine N-methyltransferase gene (ZmPEAMT1) using a combination of bioinformatics and a PCR-based allele mining strategy. The cDNA sequence of ZmPEAMT1 gene is 1,806 bp in length and translates a 495 amino acids peptide. The upstream promoter sequence of ZmPEAMT1 were obtained by TAIL-PCR, and contained four kinds of putative cis-acting regulatory elements, including stress-responsive elements, phytohormone-responsive elements, pollen developmental special activation elements, and light-induced signal transduction elements, as well as several other structural features in common with the promoter of rice and Arabidopsis homologies. RT-PCR analysis showed that expression of ZmPEAMT1 was induced by salt stress and suppressed by high temperature. Over-expression of ZmPEAMT1 enhanced the salt tolerance, root length, and silique number in transgenic Arabidopsis. These data indicated that ZmPEAMT1 maybe involved in maize root development and stress resistance, and maybe having a potential application in maize genetic engineering.