

Analysis on Limiting Factors of Efficient Utilization of Winter Wheat and Summer Maize Farmland Resources
LIU XiaoYu

通讯作者:
责任编辑: 杨鑫浩
收稿日期:2020-05-14接受日期:2020-08-17网络出版日期:2020-10-01
基金资助: |
Received:2020-05-14Accepted:2020-08-17Online:2020-10-01
作者简介 About authors
刘肖瑜,E-mail:

摘要
关键词:
Abstract
Keywords:
PDF (5770KB)元数据多维度评价相关文章导出EndNote|Ris|Bibtex收藏本文
本文引用格式
刘肖瑜, 张豆豆, 焦进宇, 陈国庆, 李勇. 冬小麦-夏玉米周年农田资源高效利用限制因素分析[J]. 中国农业科学, 2020, 53(19): 3900-3914 doi:10.3864/j.issn.0578-1752.2020.19.005
LIU XiaoYu, ZHANG DouDou, JIAO JinYu, CHEN GuoQing, LI Yong.
0 引言
【研究意义】小麦、玉米是我国最主要的2种粮食作物,在我国农业生产中位居重要地位。近年来,全球大部分粮食主产区出现了单产停滞现象,在中国,56%的小麦和52%的玉米均出现了这一现象[1]。同时由于粮食安全问题的迫切要求,持续提升粮食总产仍是农业领域的热点问题[2]。在中国、印度等为代表的发展中国家,依靠大量资源的投入代价成本的增产模式不可持续,如何在节约资源的基础上,提高农田资源利用效率、增加作物单位面积产量是确保粮食安全的唯一途径[3,4]。细化不同生态区域限制增产增效的因素,有针对性地提高区域作物产量,是解决这一问题的有效方法。【前人研究进展】作物生产是一个复杂的系统,是影响作物生长发育的各种因子,如温度、光照、降水、品种及各种耕作措施等综合作用的结果。作物高产高效的实现必须全面考虑作物气候条件、品种、肥料、灌溉、种植密度等诸多因素。为明确不同生态因子对产量的影响,前人提出了作物生产潜力和产量差的概念,国内外在量化驱动因素(如气候变化、品种更替以及栽培管理措施等)对作物生产潜力的贡献方面做了大量研究工作,通过比较实际产量与不同潜力产量水平的差异,明确不同等级产量差[5,6,7,8,9,10,11,12]。研究表明,管理因素(如播种期、播种密度、品种选择、灌水量、施肥量等)对产量的影响远高于气候因素和土壤因素[12,13],且作物产量变化不是被单一因素主导,而是多种因素共同作用下的结果[13,14,15]。APSIM模型被广泛应用于气候变化评估[16,17,18,19,20]和农田管理[21,22,23,24,25,26],对作物种植制度、轮作生理生态等方面具有较好的模拟能力[27]。【本研究切入点】为实现粮食增产和环境绿色发展,本文从资源匹配角度出发,明确不同生态区限制作物高产高效的主要限制因子,针对性地提高区域作物产量。【拟解决的关键问题】本研究以冬小麦、夏玉米为研究对象,在山东省设置不同生态区,并利用该地区已验证的APSIM模型,基于气象站点地面气象资料,研究不同区域气候条件、栽培管理条件下的可获得产量,明确冬小麦、夏玉米季节内资源利用特征,并提出定量化评价指标,分析影响各生态区域资源利用及产量提升的主要限制因素,以期为不同生产区域作物高产高效生产提供指导。1 材料与方法
1.1 研究区域概况
本文以山东省(34°23′—38°24′N,114°48′—122°42′E)为研究区域,山东省位于黄淮海平原东部,根据地理位置及气候特点将其划分为4个生态区域:鲁东(ES)、鲁中(CS)、鲁西北(NS)和鲁西南地区(SS)(图1)。分别选取鲁东地区龙口站点、鲁中地区博山站点、鲁西北地区德州站点以及鲁西南地区兖州站点进行研究,4个生态区冬小麦季、夏玉米季2008—2017年气候条件如表1所示。图1
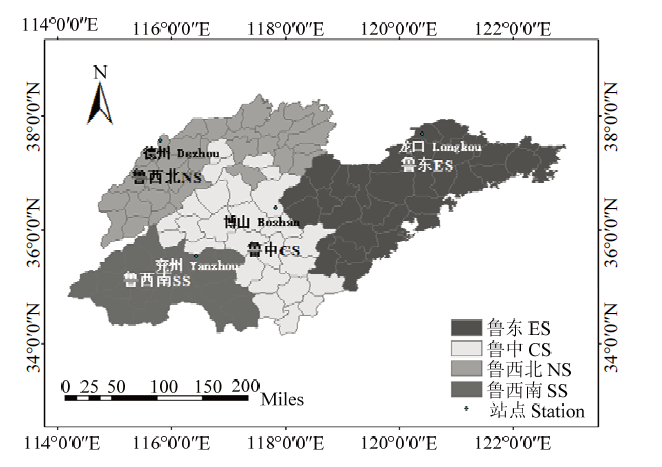
图1研究区域
Fig. 1Study area
Table 1
表1
表14个生态区冬小麦季、夏玉米季气候条件
Table 1
生长季 Growth season | 参数 Parameter | 鲁东 ES | 鲁中 CS | 鲁西北 NS | 鲁西南 SS |
---|---|---|---|---|---|
冬小麦季 Winter wheat season | 太阳辐射Solar radiation (MJ·m-2) | 3362.75 | 3141.43 | 3387.37 | 3136.13 |
平均温度Average temperature (℃) | 7.92 | 8.91 | 7.77 | 8.73 | |
降水量Precipitation (mm) | 182.32 | 193.05 | 127.56 | 190.86 | |
夏玉米季 Summer maize season | 太阳辐射Solar radiation (MJ·m-2) | 2389.75 | 2083.91 | 2340.37 | 2113.68 |
平均温度Average temperature (℃) | 24.47 | 25.12 | 24.70 | 24.90 | |
降水量Precipitation (mm) | 462.01 | 463.10 | 453.65 | 491.45 |
新窗口打开|下载CSV
1.2 数据来源
1.2.1 气象数据 研究区域内气象站点2008—2017年的逐日气象资料来自中国气象数据网(http://data. cma.cn/),主要包括日最高气温(℃)、最低气温(℃)、平均气温(℃)、日照时数(h)和降水量(mm)等。根据Angstrom方程阐述的太阳总辐射与日照时数关系,结合逐日日照时数实测资料,模拟计算逐日太阳辐射(MJ·m-2)数据。1.2.2 土壤数据 土壤数据整理自中国土壤数据库(http://www.soil.csdb.cn/),包括各深度土层的容重、土壤饱和含水量、田间持水量、萎蔫系数、土壤有机碳、pH等。
1.2.3 作物栽培管理数据 参考前人对山东省冬小麦夏玉米的研究,作物品种选择山东省种植范围最广且连续种植年份最多的冬小麦品种济麦22和夏玉米品种郑单958作为基准品种,田间管理随实际生产设置。
1.2.4 模型验证数据 试验于山东省泰安市泰山区大汶口镇(35°58′N,117°3′E)进行。设置裂区试验,主区为施氮量处理,分别为N0(小麦季不施氮+玉米季不施氮)、N1(小麦季施氮80 kg N·hm-2+玉米季施氮150 kg N·hm-2)、N2(小麦季施氮160 kg N·hm-2 +玉米季施氮300 kg N·hm-2)、N3(小麦季施氮240 kg N·hm-2+玉米季施氮450 kg N·hm-2)和N4(小麦季施氮320 kg N·hm-2+玉米季施氮600 kg N·hm-2);副区为种植作物和不种植2种处理,两因素组合为10个处理。冬小麦品种为济麦22,播种密度为180株/m2;玉米品种为郑单958,播种密度为7.6株/m2。氮肥、磷肥、钾肥分别选用尿素(含氮46%)、过磷酸钙(含磷14%)、硫酸钾(含钾50%),磷肥和钾肥作为基肥,分别为147 kg·hm-2和450 kg·hm-2,氮肥于播种期施入40%,大喇叭口期施入60%。其他田间管理措施统一。
1.3 计算方法
1.3.1 光温生产潜力的计算 本文冬小麦、夏玉米光温生产潜力采用逐级订正法[28],通过在光合生产潜力的基础上进行温度订正计算得出。计算公式如下:Y(Q)= εφΩ(1-α)(1-β)(1-ρ)(1-γ)(1-ω)(1-η)-1(1-ζ)-1q-1sΣQ
式中,Y(Q)为光合生产潜力,kg·hm-2;ε为光合作用量子效率;ψ为光合有效辐射占总辐射比例;Ω为作物光合固定二氧化碳能力;α为作物群体反射率;β为作物群体对太阳辐射的漏射率;ρ为非光合器官截留辐射比例;γ为光饱和限制率;ω为呼吸消耗占光合产量的比例;η为成熟产品的含水率;ζ为植物无机灰分含量的比例;q为单位干物质含热量,MJ·kg-1;s为作物经济系数;ΣQ为作物生长季内太阳总辐射量,MJ·m-2。根据文献查阅相近地区各参数取值如表2。
Table 2
表2
表2光合生产潜力计算中各参数取值
Table 2
生长季 Growth season | ε | ψ | Ω | α | β | ρ | γ | ω | η | ζ | q | s |
---|---|---|---|---|---|---|---|---|---|---|---|---|
冬小麦季 Winter wheat season | 0.224 | 0.49 | 0.85 | 0.10 | 0.07 | 0.10 | 0.05 | 0.33 | 0.14 | 0.08 | 17.58 | 0.40 |
夏玉米季 Summer maize season | 0.224 | 0.49 | 1.00 | 0.08 | 0.06 | 0.10 | 0.01 | 0.30 | 0.15 | 0.08 | 17.20 | 0.40 |
新窗口打开|下载CSV
光温生产潜力在光合生产潜力的基础上用温度订正得出:
Y(Q,T)=Y(Q)f(T)
式中,Y(Q,T)为光温生产潜力,kg·hm-2;?(T)为温度订正函数。
1.3.2 冬小麦-夏玉米轮作资源高效利用系数计算 农业资源是光、温、水、土、肥、气及其他生产要素与生命物质组成的一个耦合系统。农业资源利用率直接影响我国粮食生产。本文综合考虑光温水肥因素计算冬小麦-夏玉米轮作资源利用系数。
$R{{I}_{wheat}}=GP{{I}_{wheat}}\times Vt{{I}_{wheat}}\times Cu{{I}_{wheat}}\times W{{I}_{wheat}}\times F{{I}_{wheat}}$
$R{{I}_{maize}}=GP{{I}_{maize}}\times Vt{{I}_{maize}}\times Cu{{I}_{maize}}\times W{{I}_{maize}}\times F{{I}_{maize}}$
$GPI=\frac{A{{T}_{a}}}{A{{T}_{s}}}\times \frac{{{Y}_{s}}}{{{P}_{t}}}$
$\frac{A{{T}_{a}}}{A{{T}_{s}}}=\left\{ \begin{matrix} \frac{A{{T}_{a}}}{A{{T}_{s}}}\begin{matrix}{} & {} \\ \end{matrix}A{{T}_{a}}<A{{T}_{s}} \\ 1\begin{matrix} {} & {} \\ \end{matrix}\begin{matrix} {} & {} \\ \end{matrix}A{{T}_{a}}\ge A{{T}_{s}} \\ \end{matrix} \right.$
$VtI=\frac{{{Y}_{c}}}{{{Y}_{o}}}$
$CuI=\frac{{{Y}_{dc}}}{{{Y}_{do}}}$
式中,RIwheat、RImaize分别为冬小麦季、夏玉米季资源利用系数;GPIwheat、GPImaize分别为冬小麦季、夏玉米季光温资源匹配系数;VtIwheat、VtImaize 分别为冬小麦、夏玉米品种光温敏感性系数;CuIwheat、CuImaize 分别为冬小麦季、夏玉米季栽培模式匹配系数;WIwheat、WImaize分别为冬小麦季、夏玉米季水资源利用系数;FIwheat、FImaize分别为冬小麦季、夏玉米季养分资源利用系数;ATa为实际生育期内积温,℃·d;ATs 为适宜生育期内积温,℃·d ;Ys 为当前光温水平可获得产量;Pt 为光温生产潜力,kg·hm-2;Yc 为当前品种参数条件下可获得产量,kg·hm-2;Yo 为冬小麦最佳春化敏感性指数和光周期敏感性指数组合可获得的产量/夏玉米最佳出苗到拔节的积温和开花到成熟的积温组合可获得产量,kg·hm-2;Ydc 为实际种植密度条件下产量,kg·hm-2;Ydo为最优种植密度条件下可获得产量,kg·hm-2。
根据作物不同时期耗水量不同[29],计算冬小麦、夏玉米水资源利用系数。
$W{{I}_{wheat}}=\frac{\sum\limits_{a=1}^{s}{\frac{{{P}_{a}}+{{W}_{a}}}{E{{T}_{a}}}}}{s}$
$W{{I}_{maize}}=\frac{\sum\limits_{b=1}^{t}{\frac{{{P}_{b}}+{{W}_{b}}}{E{{T}_{b}}}}}{t}$
$\frac{P+W}{ET}=\left\{ \begin{matrix} \frac{P+W}{ET}\begin{matrix} {} & {} \\ \end{matrix}P+W<ET \\ \begin{array}{*{35}{l}} \begin{array}{*{35}{l}} {} \\ \end{array} \\ \end{array}1\begin{matrix} {} & \begin{matrix} {} & {} \\ \end{matrix} \\ \end{matrix}P+W\ge ET \\ \end{matrix} \right.$
式中,Pa、Pb分别表示冬小麦、夏玉米生育阶段降水量,mm;Wa、Wb分别表示冬小麦、夏玉米生育阶段灌水量,mm;ETa、ETb分别表示冬小麦、夏玉米生育阶段耗水量,mm;a表示冬小麦播种—拔节、拔节—开花、开花—成熟阶段,b表示夏玉米播种—拔节、拔节—吐丝、吐丝—成熟阶段;s、t分别表示冬小麦、夏玉米生育阶段个数。
优化氮肥管理可以提高氮肥利用效率,而最优氮肥管理的确定要基于作物整个生长季内氮肥需求和供应之间的同步性。
$F{{I}_{wheat}}=\frac{{{N}_{0}}+{{N}_{fer}}}{{{N}_{C1}}}$
$F{{I}_{maize}}=\frac{{{N}_{0}}+{{N}_{fer}}}{{{N}_{C2}}}$
式中,N0为0—100 cm土壤氮含量,mg·kg-1;Nfer为全生育期氮肥施入量,kg·hm-2;Nc1、Nc2分别为冬小麦季、夏玉米季作物需氮量,kg·hm-2。
1.4 模型验证方法
本研究采用APSIM7.10版本进行模拟。在APSIM模型调参和验证时,利用模拟值与实测值之间的均方根误差(RMSE)、归一化均方根误差(NRMSE)、决定系数(R2)和一致性指标(D指标)进行评价。RMSE和NRMSE可反映模拟值与实测值之间的相对误差和绝对误差,值越小表明模拟效果越好;R2 和D指标反映模拟值与实测值之间的一致性,值越接近1表明模拟效果越好。具体计算公式如下:$RMSE\text{=}\sqrt{\frac{1}{\text{n}}\sum\limits_{i=1}^{n}{{{\left( {{Y}_{i}}-{{X}_{i}} \right)}^{2}}}}$
$NRMSE\text{=}\frac{\sqrt{\frac{1}{\text{n}}\sum\limits_{i=1}^{n}{{{\left( {{Y}_{i}}-{{X}_{i}} \right)}^{2}}}}}{\overline{X}}\times 100%$
$D=\frac{\sum\limits_{i=1}^{n}{{{\left( {{Y}_{i}}-{{X}_{i}} \right)}^{2}}}}{\sum\limits_{i=1}^{n}{{{\left( \left| {{Y}_{i}}-\overline{X} \right|+\left| {{X}_{i}}-\overline{X} \right| \right)}^{2}}}}$
式中,Xi为实测值,Yi为模拟值,?X为实测数据平均值,n为数据样本数。
1.5 数据分析
用Microsoft Excel 2010 对数据进行整理分析,用ArcGIS、Matlab绘制图表。2 结果
2.1 APSIM模型验证
采用试错法,对APSIM模型进行充分调试和验证。通过参数调试与验证后,对冬小麦、夏玉米生长季及周年生物量和产量的模拟值和实测值进行比较。由图2可以看出,生物量数据点均匀地分布在1﹕1线两侧,冬小麦、夏玉米及周年生物量斜率分别为1.002、0.9173和1.070,决定系数(R2)分别为0.9805、0.8478和0.9364,归一化均方根误差(NRMSE)在评价生物量时变动范围在5.02%—8.58%,一致性指标(D指标)分别为0.9949、0.9587和0.9807。由图3可看出,产量数据点亦均匀地分布在1﹕1线两侧,冬小麦、夏玉米及周年产量斜率分别为1.026、0.8803和1.156,R2分别为0.9591、0.7266和0.8908,NRMSE在评价产量时变动范围在7.26%—11.73%,D指标分别为0.9876、0.9190和0.9575。总体看来,数据点偏离程度较小,生物量、产量斜率均接近于1,R2均达0.70以上,NRMSE变动范围均小于20%,D指标均在0.90以上,模拟值和实测值吻合度较好,模型验证结果良好,APSIM模型在该地区有较好的适用性。图2
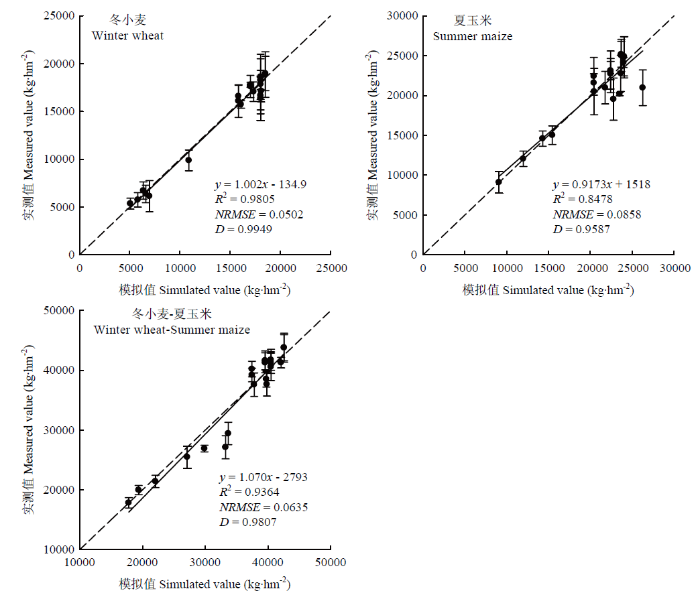
图2冬小麦夏玉米及周年生物量模拟值与实测值的比较
Fig. 2Comparison between simulated and measured value of biomass for winter wheat, summer maize and annual
图3
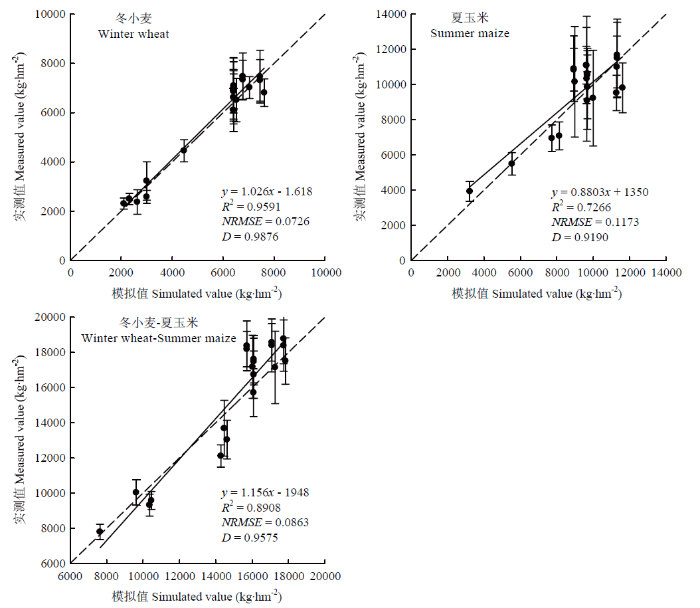
图3冬小麦夏玉米及周年产量模拟值与实测值的比较
Fig. 3Comparison between simulated and measured value of yield for winter wheat, summer maize and annual
2.2 冬小麦、夏玉米生长季光温资源匹配分析
基于山东省鲁东、鲁中、鲁西北和鲁西南4个生态区2008—2017年气象资料,利用APSIM模型模拟不同播期处理下冬小麦、夏玉米产量,通过产量指标确定各生态区冬小麦-夏玉米轮作最佳播种期及收获期。对各生态区域冬小麦、夏玉米生长季适宜生育期内有效积温、可获得产量以及光温生产潜力数据进行分析(表3),结果表明鲁东、鲁中、鲁西北和鲁西南地区冬小麦光温资源匹配系数分别为0.8812、0.7097、0.8569、0.7401;4个地区夏玉米光温资源匹配系数分别为0.5481、0.5589、0.5491、0.5701,各地区夏玉米等光温资源匹配系数差异不大。整体来看,夏玉米季光温资源匹配系数远低于冬小麦季。Table 3
表3
表3各生态区冬小麦、夏玉米适宜生育期内GDD、产量、光温生产潜力及匹配系数
Table 3
生态区 Ecological region | 适宜生育期内有效积温 GDD (℃·d) | 适宜生育期获得产量 Available yield (kg·hm-2) | 光温生产潜力 Light-temperature potential productivity (kg·hm-2) | 匹配系数 Matching coefficient | |
---|---|---|---|---|---|
冬小麦季 Wheat season | 鲁东ES | 2289.22 | 9845.51 | 11172.61 | 0.8812 |
鲁中CS | 2377.91 | 8369.21 | 11792.80 | 0.7097 | |
鲁西北NS | 2191.03 | 9692.29 | 11310.78 | 0.8569 | |
鲁西南SS | 2239.41 | 8419.19 | 11375.36 | 0.7401 | |
夏玉米季 Maize season | 鲁东ES | 2697.94 | 11531.90 | 21039.04 | 0.5481 |
鲁中CS | 2740.21 | 10616.70 | 18997.41 | 0.5589 | |
鲁西北NS | 2802.96 | 11441.68 | 20836.07 | 0.5491 | |
鲁西南SS | 2724.02 | 10848.45 | 19031.06 | 0.5701 |
新窗口打开|下载CSV
2.3 冬小麦、夏玉米生长季品种匹配分析
利用APSIM模型,设置冬小麦品种参数中春化敏感性指数(Vern_sens)、光周期敏感性指数(Photop_sens)2个品种参数水平,通过给定品种参数的范围,随机组合生成169组品种参数,其他品种参数保持不变(表4),确定在给定环境条件下最佳产量表现的最佳品种(图4)。结果表明,鲁东地区龙口站点冬小麦春化敏感性指数(Vern_sens)、光周期敏感性指数(Photop_sens)均为3.5时,产量最高,高于基准品种可获得产量452.87 kg·hm-2。鲁中地区博山站点冬小麦春化敏感性指数为4.3、光周期敏感性指数为3.9,鲁西北地区德州站点两参数分别为3.1、3.9,鲁西南地区兖州站点两参数分别为4.3、3.9时产量最高,分别高于当地基准品种可获得产量1 139.47、652.24和1 580.35 kg·hm-2。鲁东、鲁中、鲁西北和鲁西南地区冬小麦品种匹配系数分别为0.8552、0.8449、0.8473、0.8162,4个生态区差异不大。Table 4
表4
表4作物品种参数设置
Table 4
品种参数 Variety parameter | 冬小麦Winter wheat | 夏玉米Summer maize | |||
---|---|---|---|---|---|
春化敏感性参数 Vern_sens | 光周期敏感性参数 Photop_sens | 出苗-拔节积温 tt_emerg_to_endjuv (℃·d) | 开花-成熟积温 tt_flower_to_maturity (℃·d) | ||
处理1 Level1 | 0.3 (VS1) | 0.3 (PS1) | 200 (EE1) | 600 (FM1) | |
处理2 Level2 | 0.7 (VS2) | 0.7 (PS2) | 215 (EE2) | 650 (FM2) | |
处理3 Level3 | 1.1 (VS3) | 1.1 (PS3) | 225 (EE3) | 700 (FM3) | |
处理4 Level4 | 1.5 (VS4) | 1.5 (PS4) | 245 (EE4) | 750 (FM4) | |
处理5 Level5 | 1.9 (VS5) | 1.9 (PS5) | 250 (EE5) | 800 (FM5) | |
处理6 Level6 | 2.3 (VS6) | 2.3 (PS6) | 255 (EE6) | 850 (FM6) | |
处理7 Level7 | 2.7 (VS7) | 2.7 (PS7) | 265 (EE7) | 900 (FM7) | |
处理8 Level8 | 3.1 (VS8) | 3.1 (PS8) | 275 (EE8) | 950 (FM8) | |
处理9 Level9 | 3.5 (VS9) | 3.5 (PS9) | 285 (EE9) | 1000 (FM9) | |
处理10 Level10 | 3.9 (VS10) | 3.9 (PS10) | 300 (EE10) | 1050 (FM10) | |
处理11 Level11 | 4.3 (VS11) | 4.3 (PS11) | 325 (EE11) | 1100 (FM11) | |
处理12 Level12 | 4.7 (VS12) | 4.7 (PS12) | 350 (EE12) | 1150 (FM12) | |
处理13 Level13 | 5.0 (VS13) | 5.0 (PS13) | 375 (EE13) | 1200 (FM13) | |
处理14 Level14 | — | — | 400 (EE14) | 1250 (FM14) |
新窗口打开|下载CSV
图4
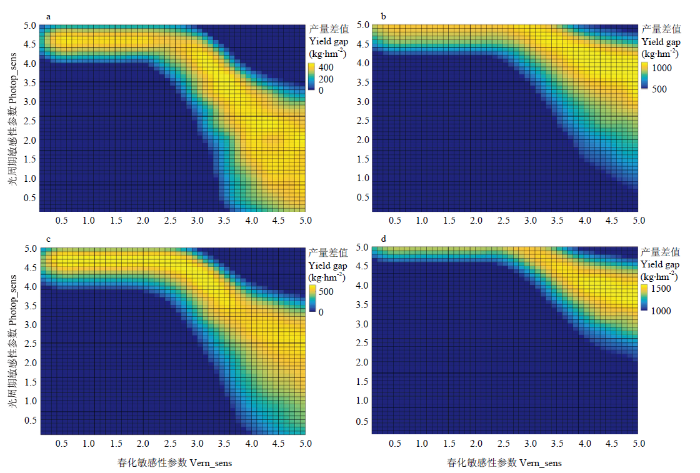
图4冬小麦不同品种参数组合获得产量与基准品种可获得产量差值
产量差值=不同春化敏感性指数和光周期敏感性指数组合获得产量-基准品种获得产量。(a)、(b)、(c)、(d)分别代表鲁东、鲁中、鲁西北、鲁西南
Fig. 4The gap between the yield of winter wheat from different varieties and the yield of standard varieties
Yield gap = yield available by different combinations of Vern_sens and Photop_sens - yield available by standard varieties. (a), (b), (c), (d) represent ES, CS, NS, SS, respectively
利用APSIM模型,通过设置夏玉米品种参数中出苗到拔节期的积温(tt_emerg_to_endjuv)、开花到成熟的积温(tt_flower_to_maturity)2个品种参数水平,随机组合生成196组品种参数(表4),其他品种参数保持不变(图5)。结果显示,鲁东地区龙口站点夏玉米出苗到拔节期的积温为200、开花到成熟的积温为1 150时,产量显著高于基准品种可获得产量。鲁中地区博山站点夏玉米出苗到拔节期的积温为200、开花到成熟的积温为1 200时产量最高,鲁西北地区德州站点夏玉米出苗到拔节期的积温为200、开花到成熟的积温为1 250时产量最高。鲁西南地区兖州站点两参数分别为255、1 100时产量最高。鲁东、鲁中、鲁西北和鲁西南地区夏玉米品种匹配系数分别为0.9165、0. 9154、0.8013、0.9637(表5)。
图5
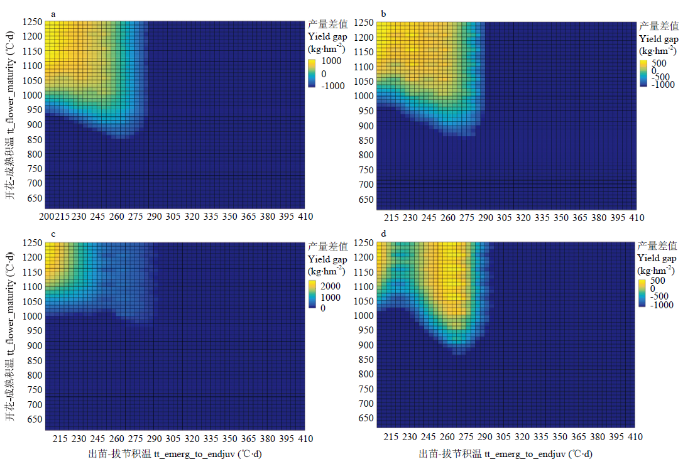
图5夏玉米不同品种参数组合获得产量与基准品种可获得产量差值
产量差值=不同出苗到拔节期积温和开花到成熟积温组合获得产量-基准品种获得产量。(a)、(b)、(c)、(d)分别代表鲁东、鲁中、鲁西北、鲁西南
Fig. 5The gap between the yield of summer maize from different varieties and the yield of standard varieties
Yield gap = yield available by different combinations of tt_emerg_to_endjuv and tt_flower_to_maturity - yield available by standard varieties. (a), (b), (c), (d) represent ES, CS, NS, SS, respectively
Table 5
表5
表5各生态区冬小麦、夏玉米最佳品种参数组合、获得产量及匹配系数
Table 5
作物Crop | 参数Parameter | 鲁东ES | 鲁中CS | 鲁西北NS | 鲁西南SS |
---|---|---|---|---|---|
冬小麦 Winter wheat | 春化敏感性系数 Vern_sens | 3.5 | 4.3 | 3.1 | 4.3 |
光周期敏感性系数 Photop_sens | 3.5 | 3.9 | 3.9 | 3.9 | |
产量 Yield (kg·hm-2) | 10298.38 | 9508.68 | 10344.53 | 9999.54 | |
匹配系数 Matching coefficient | 0.8552 | 0.8449 | 0.8473 | 0.8162 | |
夏玉米 Summer maize | 出苗至拔节期积温tt_emerg_to_endjuv (℃·d) | 200 | 200 | 200 | 255 |
开花至成熟积温 tt_flower_to_maturity (℃·d) | 1150 | 1200 | 1250 | 1100 | |
产量 Yield (kg·hm-2) | 12582.62 | 11598.12 | 14279.07 | 11256.58 | |
匹配系数 Matching coefficient | 0.9165 | 0.9154 | 0.8013 | 0.9637 |
新窗口打开|下载CSV
2.4 冬小麦、夏玉米生长季栽培模式匹配分析
根据山东省各生态区冬小麦、夏玉米产量随播种密度的变化趋势分析,随着播种密度的增加,鲁东、鲁中、鲁西北和鲁西南地区冬小麦、夏玉米产量均增加。当冬小麦和夏玉米的播种密度分别高于550株/m2和8株/m2时,产量增量趋于平稳。因此,冬小麦和夏玉米的最佳播种密度分别为550株/m2和8株/m2。最佳播种密度条件下,鲁东、鲁中、鲁西北和鲁西南地区冬小麦季可获得产量分别为10 049.86 kg·hm-2、8 471.86 kg·hm-2、9 825.92 kg·hm-2、8 527.92 kg·hm-2,夏玉米季分别为11 531.94 kg·hm-2、10 616.74 kg·hm-2、11 441.68 kg·hm-2、10 848.51 kg·hm-2(表6)。根据山东省冬小麦大田一般种植密度约为540株/m2、夏玉米一般生产密度为6—7株/m2,模拟不同生态区冬小麦、夏玉米平均可获得产量并计算得出鲁东、鲁中、鲁西北及鲁西南地区冬小麦季栽培模式匹配系数分别为0.9903、0.9879、0.9904、0.9873,夏玉米季栽培模式匹配系数分别为0.9021、0.9092、0.8820、0.8943。Table 6
表6
表6各生态区最佳播种密度水平下可获得产量及匹配系数
Table 6
生态区 Ecological region | 冬小麦Winter wheat | 夏玉米Summer maize | |||
---|---|---|---|---|---|
可获得产量 Available yield (kg·hm-2) | 匹配系数 Matching coefficient | 可获得产量 Available yield (kg·hm-2) | 匹配系数 Matching coefficient | ||
鲁东ES | 10049.86 | 0.9903 | 11531.94 | 0.9021 | |
鲁中CS | 8471.68 | 0.9879 | 10616.74 | 0.9092 | |
鲁西北NS | 9825.92 | 0.9904 | 11441.68 | 0.8820 | |
鲁西南SS | 8527.92 | 0.9873 | 10848.51 | 0.8943 |
新窗口打开|下载CSV
2.5 冬小麦、夏玉米生长季水资源利用分析
根据小麦产量与水分生产率关系计算山东省各生态区小麦全生育期需水量。按照农民习惯并结合相关试验,于播种、拔节、开花期进行灌溉,每次灌水量75 mm。山东省各生态区冬小麦季播种—拔节,拔节—开花,开花—成熟需水量及全生育期水资源利用系数如表7所示。鲁东地区冬小麦播种期至拔节期、拔节期至开花期、开花期至成熟阶段需水量分别为255.20、184.30、269.40 mm;鲁中地区分别为216.90、156.70、229.00 mm;鲁西北地区分别为251.20、181.40、265.20 mm;鲁西南地区分别为218.20、157.60、230.30 mm。各生育阶段水资源利用系数均小于1,即降水、灌水量不能满足各生育时期需水量。鲁东、鲁中、鲁西北和鲁西南地区冬小麦季水资源利用系数分别为0.5730、0.6880、0.5062、0.6841。Table 7
表7
表7山东省各生态区冬小麦季水资源利用系数
Table 7
生态区 Ecological region | 参数 Parameter | 生育阶段Growth stage | ||
---|---|---|---|---|
播种—拔节 Sowing-jointing stage | 拔节—开花 Jointing-flowering stage | 开花—成熟 Flowering-maturing stage | ||
鲁东ES | 降水量P (mm) 需水量ET (mm) 水资源利用系数WI | 89.5 255.2 | 24.5 184.3 0.5730 | 69.0 269.4 |
鲁中CS | 降水量P (mm) 需水量ET (mm) 水资源利用系数WI | 86.2 216.9 | 23.8 156.7 0.6880 | 83.0 229.0 |
鲁西北NS | 降水量P (mm) 需水量ET (mm) 水资源利用系数WI | 56.2 251.2 | 16.8 181.4 0.5062 | 55.0 265.2 |
鲁西南SS | 降水量P (mm) 需水量ET (mm) 水资源利用系数WI | 90.9 218.2 | 30.1 157.6 0.6841 | 69.0 230.3 |
新窗口打开|下载CSV
根据每生产100 kg玉米籽粒所需耗水量计算山东省各生态区夏玉米全生育期需水量。按照农民习惯夏玉米生长季一般不进行灌水。山东省各生态区夏玉米季播种—拔节,拔节—吐丝,吐丝—成熟需水量及全生育期水资源利用系数如表8所示。鲁东地区夏玉米播种期至拔节期、拔节期至吐丝期、吐丝期至成熟期需水量分别为125.0、264.0、305.8 mm;鲁中地区分别为116.0、245.0、283.8 mm;鲁西北地区分别为127.0、268.0、310.2 mm;鲁西南地区分别为120.0、253.0、293.3 mm。其中鲁中、鲁西北及鲁西南地区播种至拔节期水分利用系数均为1,阶段降水量满足其需水量。其余生育期阶段水分利用系数均小于1。鲁东、鲁中、鲁西北和鲁西南地区夏玉米季水资源利用系数分别为0.7131、0.8374、0.7193、0.9046。
Table 8
表8
表8山东省各生态区夏玉米季水资源利用系数
Table 8
生态区 Ecological region | 参数 Parameter | 生育阶段Growth stage | ||
---|---|---|---|---|
播种—拔节 Sowing-jointing stage | 拔节—吐丝 Jointing-silking stage | 吐丝—成熟 Silking-maturing stage | ||
鲁东ES | 降水量P (mm) 需水量ET (mm) | 117.0 125.0 | 145.0 264.0 | 200.0 305.8 |
水资源利用系数WI | 0.7131 | |||
鲁中CS | 降水量P (mm) 需水量ET (mm) | 161.0 116.0 | 108.0 245.0 | 194.0 283.8 |
水资源利用系数WI | 0.8374 | |||
鲁西北NS | 降水量P (mm) 需水量ET (mm) | 137.0 127.0 | 113.0 268.0 | 204.0 310.2 |
水资源利用系数WI | 0.7193 | |||
鲁西南SS | 降水量P (mm) 需水量ET (mm) | 198.0 120.0 | 107.0 253.0 | 188.0 293.3 |
水资源利用系数WI | 0.9046 |
新窗口打开|下载CSV
2.6 冬小麦、夏玉米生长季氮肥资源利用分析
根据每生产100 kg小麦、玉米籽粒需氮量计算山东省各生态区冬小麦夏玉米全生育期需氮量。冬小麦、夏玉米生长季农民常规施氮量平均为240 kg·hm-2左右。山东省鲁东、鲁中、鲁西北和鲁西南地区冬小麦季需氮量分别为288.3、265.4、286.0、271.2 kg·hm-2;夏玉米季各区域需氮量分别为305.2、259.4、300.5、261.0 kg·hm-2。鲁东、鲁中、鲁西北和鲁西南地区冬小麦、夏玉米两季养分资源利用系数均为1(表9)。即各地区冬小麦、夏玉米生长季内氮肥供给量均多于甚至远超过作物需氮量。Table 9
表9
表9山东省各生态区冬小麦季、夏玉米季养分资源利用系数
Table 9
生态区 Ecological region | 参数 Parameter | 冬小麦季 Winter wheat season | 夏玉米季 Summer maize season |
---|---|---|---|
鲁东ES | 土壤氮含量N0 (mg·kg-1) | 78.0 | |
作物需氮量NC (kg·hm-2) | 305.2 | 288.3 | |
养分资源利用系数FI | 1 | 1 | |
鲁中CS | 土壤氮含量N0 (mg·kg-1) | 91.7 | |
作物需氮量NC (kg·hm-2) | 259.4 | 265.4 | |
养分资源利用系数FI | 1 | 1 | |
鲁西北NS | 土壤氮含量N0 (mg·kg-1) | 81.0 | |
作物需氮量NC (kg·hm-2) | 300.5 | 286.0 | |
养分资源利用系数FI | 1 | 1 | |
鲁西南SS | 土壤氮含量N0(mg·kg-1) | 90.2 | |
作物需氮量NC (kg·hm-2) | 261.0 | 271.2 | |
养分资源利用系数FI | 1 | 1 |
新窗口打开|下载CSV
2.7 不同生态区农田资源利用限制因素
如图6所示,在冬小麦生长季,鲁东地区受光温资源、品种、水资源因素影响率分别为16.96%、20.68%、60.97%;鲁西北地区受上述三因素影响率分别为17.90%、19.11%、61.79%,表明小麦生长季鲁东、鲁西北地区农田资源利用主要受水分资源限制,其次是光温资源和品种的限制,栽培模式和氮肥资源影响不大。鲁中地区光温资源、品种、水资源因素影响率分别为37.72%、20.16%、40.55%;鲁西南地区分别为33.65%、23.80%、40.90%。鲁中、鲁西南地区主要受光温资源和水分资源的共同影响,其次受品种因素限制。在夏玉米生长季,鲁东地区受光温资源、品种、栽培模式、水资源、氮肥资源因素影响率分别为49.11%、9.07%、10.64%、31.18%和0;鲁中地区受各限制因素影响率分别为56.62%、10.86%、11.65%、20.87%和0;鲁西北地区受各限制因素影响率分别为43.01%、18.95%、11.26%、26.78%和0;鲁西南地区受各限制因素影响率分别为64.42%、5.44%、15.84%、14.30%和0。表明鲁东、鲁中、鲁西北及鲁西南地区均受光温资源因素影响最大,水资源次之,品种和栽培模式影响较小。图6
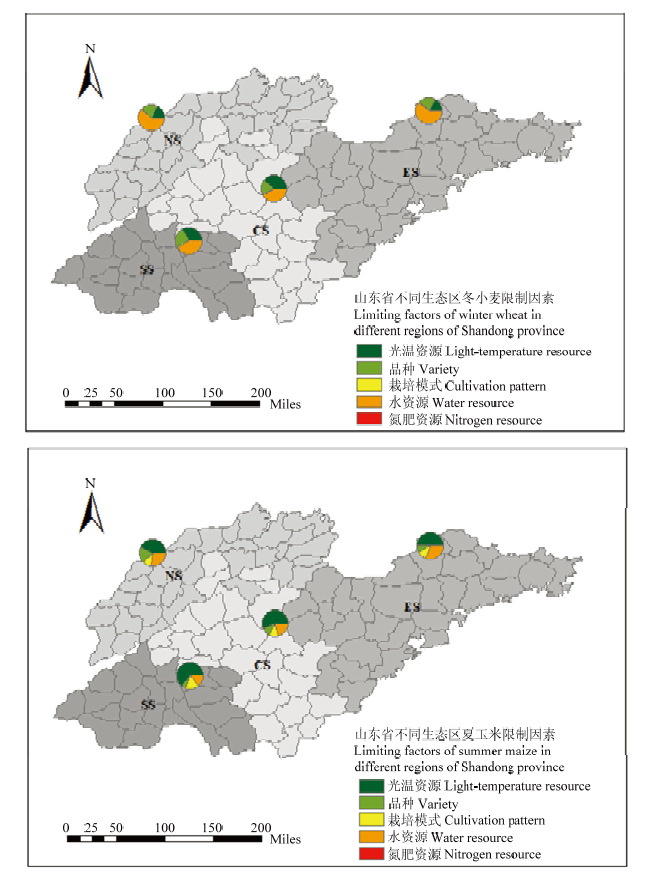
图6山东省不同生态区冬小麦夏玉米生长季影响农田资源利用限制因素
Fig. 6The limiting rate of various factors affecting the utilization of light and temperature resources in the growing wheat and maize season in different ecological areas of Shandong province
3 讨论
3.1 冬小麦-夏玉米周年资源利用的影响因素
作物生产是一个复杂的系统,作物产量形成与生态环境条件密切相关[30],光温水肥及各种栽培措施等因素均会影响作物生长发育。合理配置生长季间光温资源可显著提高冬小麦-夏玉米周年轮作系统产量和资源利用效率。播期、收获期是调节作物生育期内光温资源,改变作物生长发育环境的有效手段。还可以根据种植地的环境选择、培育适宜的品种以实现光温资源的高效利用,既能保证各季作物安全成熟,还能发挥其高产潜力。栽培措施也是影响作物光温资源利用效率的重要因素,其直接影响了作物冠层结构及光照环境。水是植物体内含量最多、作用最广泛的组成成分,参与或影响几乎所有的生物物理和生物化学过程。优化灌溉量以及灌溉时间可以有效提高作物水分利用效率和作物产量[31,32],合理的灌溉设计以及氮肥施用量对作物生长过程中的资源利用效率和产量的提高也具有重要作用。赵鑫等[33]对冬小麦-夏玉米一年两作的超高产途径进行研究,认为通过合理的灌溉设计可实现小麦生长过程中对水资源的高效利用和最终产量的提升。3.2 冬小麦-夏玉米周年不同生态区农田资源利用限制因素
山东省位于黄淮海平原东部,近年来受气候变化和生产条件影响,冬小麦-夏玉米两熟制播期、生育期、密度、作物品种等因素与光温水肥资源不匹配问题突出,限制了周年产量和资源利用效率的进一步提升[34,35]。明确周年气候资源分配与利用的定量特征,可为进一步优化季节间资源配置,提高不同生态区周年产量潜力与资源利用效率提供理论依据。本文利用APSIM模型,研究了光温资源、品种、栽培模式、水资源以及氮肥等不同因素对山东省鲁东、鲁中、鲁西北及鲁西南地区冬小麦、夏玉米生长季高产高效的影响,结果显示,冬小麦生长季鲁东、鲁西北两地区均主要受水分资源限制,其次是光温资源和品种的限制。山东省属季风气候,夏季炎热多雨,冬季寒冷干燥,年降水量分布不均,小麦生育期降水量只占全年降水量的25%—40%,仅能满足冬小麦全生育期需水量的1/3到1/5,冬小麦拔节至灌浆期是耗水高峰期,春旱缺水状况更为严重,加之农田灌溉量不足以及灌溉时间不合理,不能满足作物对水分的需求。鲁东、鲁西北地区可通过优化冬小麦生长季灌溉量和灌溉时间减少水分资源限制对农田资源利用影响。鲁中、鲁西南两地区则主要受光温资源和水分资源的共同影响,其次受品种因素限制。播期、收获期是调节作物生育时期,改善生育期内对光、热、水资源利用,改变作物生长发育环境的有效手段。调节冬小麦播期、收获期对光温资源进行优化配置,同时优化灌溉量以及灌溉时间,减少光温资源以及水分资源限制对鲁中、鲁西南两地区农田资源利用的影响。不同生态环境条件下选用适宜的作物品种,充分利用气候资源,发挥品种的生产潜力,可实现高产、高效、稳产的效果。近50年来黄淮海平原作物产量一直保持稳步增长趋势[36],与品种的改良密切相关。不同品种在不同环境条件下往往表现出不同的地域适应性,根据4个生态区环境特点选择适宜环境高产作物品种,对稳定提高区域作物产量也具有重要意义。对于华北地区的冬小麦和夏玉米,选择及培育适宜的品种可消除气候变暖大背景下引起的作物生育期缩短,保证开花前生长发育所需时间[37]。夏玉米生长季4个地区均受光温资源因素影响最大,水资源次之,品种和栽培模式影响较小。夏玉米具有高光能利用效率和光能生产效率,充分利用农闲期和冬小麦季冗余的光温资源,发挥夏玉米高光效的优势,可进一步调高周年产量和资源效率[38]。夏玉米生长季虽与夏季雨季同期,但同时其生育期处于高温季节,绝对耗水量很大[21],加之夏玉米季灌水量不足,难以满足作物水分需求。故调节夏玉米播期、收获期改善生育期内资源利用率;同时,选择紧凑型玉米品种,提高群体光能利用率,增加单位群体光合面积,充分利用光热资源。合理增加灌水量、明确灌水时间在提高水分利用效率的基础上减少水资源对产量提高的限制,综合提高夏玉米季资源利用率。
由于肥料技术的进步,施用肥料使作物产量大幅提升。山东省各生态区冬小麦季、夏玉米季氮肥资源供给量均可满足其需求,甚至可适当减少施氮量,避免因施氮过量造成氮素损失以及经济损失。
4 结论
冬小麦生长季鲁东、鲁西北地区农田资源主要受水分资源限制,其次是光温资源和品种的限制,栽培模式和氮肥资源影响不大。鲁中、鲁西南地区则主要受光温资源和水分资源的共同影响,其次受品种因素限制。夏玉米生长季4个地区均受光温资源因素影响最大,水资源次之,品种和栽培模式影响较小。根据不同区域限制因素及限制因素大小,通过调整冬小麦、夏玉米播期和收获期,选用适宜环境条件的作物品种,同时合理增加灌水量和灌水时期,可有效提高农田资源利用效率,达到稳产增产效果。参考文献 原文顺序
文献年度倒序
文中引用次数倒序
被引期刊影响因子
DOI:10.1038/ncomms2296URLPMID:23250423 [本文引用: 1]

In the coming decades, continued population growth, rising meat and dairy consumption and expanding biofuel use will dramatically increase the pressure on global agriculture. Even as we face these future burdens, there have been scattered reports of yield stagnation in the world's major cereal crops, including maize, rice and wheat. Here we study data from approximately 2.5 million census observations across the globe extending over the period 1961-2008. We examined the trends in crop yields for four key global crops: maize, rice, wheat and soybeans. Although yields continue to increase in many areas, we find that across 24-39% of maize-, rice-, wheat- and soybean-growing areas, yields either never improve, stagnate or collapse. This result underscores the challenge of meeting increasing global agricultural demands. New investments in underperforming regions, as well as strategies to continue increasing yields in the high-performing areas, are required.
DOI:10.3864/j.issn.0578-1752.2016.06.008URL [本文引用: 1]

【Objective】The purpose of this study is to reveal grain production potential and Cultivated land resources and provide a reference for national food security decisions.【Method】The time series forecasting method was used to forecast the arable land area, cropping index, food and non-food crops area ratio, crop planting structure in 2020 by using the cultivated land area and grain production series data in 1980/1996-2013 as sampled and based on the results of the second survey of land resources. Then the potential of the nation's grain yield increase was analyzed from the multi-angle of view the high-yield demonstration area, the unit-yield level in variety regional test. 【Result】By 2020, the national cultivated land area will be 1.32×108 hm2, grain crops and non-crops area will account for 66:34, grain crops acreage will be 1.12×108 hm2. The potential of national grain production will be 68.9% from high-yield demonstration area and 35.5% from regional trial variety. By 2020, the potential of national grain production will reach 6.34×108-6.53×108 t ,with 5.3%-8.5% increase compared to 2013.【Conclusion】In the future, the cultivated land and grain crops area will be decreased, but the national total grain production capacity will be continued show a upward trend with the pulling of crop yield increasing.
DOI:10.3864/j.issn.0578-1752.2016.06.008URL [本文引用: 1]

【Objective】The purpose of this study is to reveal grain production potential and Cultivated land resources and provide a reference for national food security decisions.【Method】The time series forecasting method was used to forecast the arable land area, cropping index, food and non-food crops area ratio, crop planting structure in 2020 by using the cultivated land area and grain production series data in 1980/1996-2013 as sampled and based on the results of the second survey of land resources. Then the potential of the nation's grain yield increase was analyzed from the multi-angle of view the high-yield demonstration area, the unit-yield level in variety regional test. 【Result】By 2020, the national cultivated land area will be 1.32×108 hm2, grain crops and non-crops area will account for 66:34, grain crops acreage will be 1.12×108 hm2. The potential of national grain production will be 68.9% from high-yield demonstration area and 35.5% from regional trial variety. By 2020, the potential of national grain production will reach 6.34×108-6.53×108 t ,with 5.3%-8.5% increase compared to 2013.【Conclusion】In the future, the cultivated land and grain crops area will be decreased, but the national total grain production capacity will be continued show a upward trend with the pulling of crop yield increasing.
DOI:10.3724/SP.J.1006.2012.01483URL [本文引用: 1]

APSIM-Wheat model was validated in regional level with crop data from agrometeorological observation stations in North China. Combining the daily climatic data during 1961–2007, the spatiotemporal distribution characteristics were analyzed in potential yield, water-restricted yield, and water-nitrogen-restricted yield of winter wheat to understand the influences of climatic factors on potential yield in different levels. The APSIM-Wheat model was proved to be applicable in North China. In the region, the potential yields of winter wheat in different levels showed a zonal distribution in space with a generally temporal decline; however, the differences of spatial distribution characteristics were distinct among yield levels. The decreasing trends were from northeast to southwest in potential yield of winter wheat, from southeast to northwest in water restricted yield, and from west to east before a decreasing from east to west in water and nitrogen restricted yield, with the maximum values in Jining, Shandong Province.Hebei Province was located in the zone of high values of potential yield and water and nitrogen restricted yield but low value of water restricted yield, where irrigation was the main factor to improve the yield of winter wheat. Shandong Province was in the zone of high values of potential yield and water restricted yield but low value of water and nitrogen restricted yield, where nitrogen fertilizer was the main factor to improve the yield of winter wheat. Henan Province was a zone of low value of potential yield, where radiation was a main restraint. The total radiation during the growing season was a major climatic factor that determined the spatiotemporal distribution characteristics of the simulated potential yield of winter wheat, and it was positively correlated with the potential yield (P < 0.01). Precipitation during the growing season was the most important climatic factor for distribution characteristics of water restricted yield, which was positively correlated with water restricted yield (P < 0.01). In contrast, the variance of water and nitrogen restricted yield explained by climate factors was only 0.48, suggesting that soil factors were determinative rather than climatic factors. Under the background of changed climate and unchanged varieties of winter wheat, the potential yields at different levels showed declining trends which resulted from the decrease of radiation and the increase of accumulated temperature during wheat growing season. The primary climatic factors for determining the distribution characteristics of winter wheat yield were total radiation and precipitation.
DOI:10.3724/SP.J.1006.2012.01483URL [本文引用: 1]

APSIM-Wheat model was validated in regional level with crop data from agrometeorological observation stations in North China. Combining the daily climatic data during 1961–2007, the spatiotemporal distribution characteristics were analyzed in potential yield, water-restricted yield, and water-nitrogen-restricted yield of winter wheat to understand the influences of climatic factors on potential yield in different levels. The APSIM-Wheat model was proved to be applicable in North China. In the region, the potential yields of winter wheat in different levels showed a zonal distribution in space with a generally temporal decline; however, the differences of spatial distribution characteristics were distinct among yield levels. The decreasing trends were from northeast to southwest in potential yield of winter wheat, from southeast to northwest in water restricted yield, and from west to east before a decreasing from east to west in water and nitrogen restricted yield, with the maximum values in Jining, Shandong Province.Hebei Province was located in the zone of high values of potential yield and water and nitrogen restricted yield but low value of water restricted yield, where irrigation was the main factor to improve the yield of winter wheat. Shandong Province was in the zone of high values of potential yield and water restricted yield but low value of water and nitrogen restricted yield, where nitrogen fertilizer was the main factor to improve the yield of winter wheat. Henan Province was a zone of low value of potential yield, where radiation was a main restraint. The total radiation during the growing season was a major climatic factor that determined the spatiotemporal distribution characteristics of the simulated potential yield of winter wheat, and it was positively correlated with the potential yield (P < 0.01). Precipitation during the growing season was the most important climatic factor for distribution characteristics of water restricted yield, which was positively correlated with water restricted yield (P < 0.01). In contrast, the variance of water and nitrogen restricted yield explained by climate factors was only 0.48, suggesting that soil factors were determinative rather than climatic factors. Under the background of changed climate and unchanged varieties of winter wheat, the potential yields at different levels showed declining trends which resulted from the decrease of radiation and the increase of accumulated temperature during wheat growing season. The primary climatic factors for determining the distribution characteristics of winter wheat yield were total radiation and precipitation.
[D].
[本文引用: 1]
[D].
[本文引用: 1]
DOI:10.1007/s10584-015-1579-8URL [本文引用: 1]
URLPMID:20709725 [本文引用: 1]
DOI:10.1016/j.eja.2013.09.020URL [本文引用: 1]

The detailed field experiment data from 1980 to 2009 at four stations in the North China Plain (NCP), together with a crop simulation model, were used to disentangle the relative contributions of cultivars renewal, fertilization management and climate change to winter wheat yield, as well as the relative impacts of different climate variables on winter wheat yield, in the past three decades. We found that during 1980-2009 cultivars renewal contributed to yield increase by 12.2-22.6%; fertilization management contributed to yield increase by 2.1-3.6%; and climate change contributed to yield generally by -3.0-3.0%, however by -15.0% for rainfed wheat in southern part of the NCP. Modern cultivars and agronomic management played dominant roles in yield increase in the past three decades, nevertheless the estimated impacts of climate change on yield accounted for as large as -23.8-25.0% of observed yield trends. During the study period, increase in temperature increased winter wheat yield by 3.0-6.0% in northern part of the NCP, however reduced rainfed winter wheat yield by 9.0-12.0% in southern part of the NCP. Decrease in solar radiation reduced wheat yield by 3.0-12.0% across the,stations. The impact of precipitation change on winter wheat yield was slight because there were no pronounced trends in precipitation. Our findings highlight that modern cultivars and agronomic management contributed dominantly to yield increase in the past three decades, nevertheless the impacts of climate change were large enough in some areas to affect a significant portion of observed yield trends in the NCP. (C) 2013 Elsevier B.V.
DOI:10.1890/08-0611.1URLPMID:20349832 [本文引用: 1]

Improving nitrogen use efficiency (NUE) in the major cereals is critical for more sustainable nitrogen use in high-input agriculture, but our understanding of the potential for NUE improvement is limited by a paucity of reliable on-farm measurements. Limited on-farm data suggest that agronomic NUE (AE(N)) is lower and more variable than data from trials conducted at research stations, on which much of our understanding of AE(N) has been built. The purpose of this study was to determine the magnitude and causes of variability in AE(N) across an agricultural region, which we refer to as the achievement distribution of AE(N). The distribution of simulated AE(N) in 80 farmers' fields in an irrigated wheat system in the Yaqui Valley, Mexico, was compared with trials at a local research center (International Wheat and Maize Improvement Center; CIMMYT). An agroecosystem simulation model WNMM was used to understand factors controlling yield, AE(N), gaseous N emissions, and nitrate leaching in the region. Simulated AE(N) in the Yaqui Valley was highly variable, and mean on-farm AE(N) was 44% lower than trials with similar fertilization rates at CIMMYT. Variability in residual N supply was the most important factor determining simulated AE(N). Better split applications of N fertilizer led to almost a doubling of AE(N), increased profit, and reduced N pollution, and even larger improvements were possible with technologies that allow for direct measurement of soil N supply and plant N demand, such as site-specific nitrogen management.
DOI:10.1111/geb.2010.19.issue-6URL [本文引用: 1]
DOI:10.1016/j.agsy.2010.02.004URL [本文引用: 1]
DOI:10.1016/j.fcr.2012.09.023URL [本文引用: 1]
[本文引用: 2]
DOI:10.1016/j.eja.2012.08.002URL [本文引用: 2]

For increasing rice production in West Africa, both expansion of rice harvested area and raising rice yield are required. Development of small-scale irrigation schemes is given high priority in national rice development plans. For realizing potential of the newly developed schemes, it is essential to understand yield level, farmers' crop management practices and production constraints. A series of field surveys were conducted in six small-scale irrigation schemes in Zou department, Benin during the dry season in 2010-2011 to assess variation in rice yields and identify factors affecting the variation. The schemes were established between 1969 and 2009. Rice yields ranged from 1.3 to 7.8 t ha(-1) with an average yield of 4.8 t ha(-1). The average yield was only 2.9 t ha(-1) for newer irrigation schemes developed in 2002 and 2009. Multiple regression analysis using farmers' crop management practices as well as abiotic and biotic stresses as independent variables revealed that 75% of the variation in yields could be explained by five agronomic factors (fallow residue management, ploughing method, water stress, rat damage and N application rate) and two edaphic factors (sloped surfaces and sand content in the soil). Removing fallow residue from the fields for land preparation reduced yields. Yields were lower in plots ploughed by hand than by machine. Sloped surface, water stress and rat damage reduced yields. Yield increase due to N application ranged from 0.8 to 1.6 t ha(-1). Higher sand content was associated with lowered yields. The low yields in new irrigation schemes caused by sub-optimal crop management practices suggest that farmer-to-farmer learning and extension of good agricultural principles and practices can increase yields. Organizational capacity is also important to ensure the use of common resources such as irrigation water and tractors for land preparation. (c) 2012 Elsevier B.V.
DOI:10.1038/nature19368URLPMID:27602513 [本文引用: 1]

Sustainably feeding the world's growing population is a challenge, and closing yield gaps (that is, differences between farmers' yields and what are attainable for a given region) is a vital strategy to address this challenge. The magnitude of yield gaps is particularly large in developing countries where smallholder farming dominates the agricultural landscape. Many factors and constraints interact to limit yields, and progress in problem-solving to bring about changes at the ground level is rare. Here we present an innovative approach for enabling smallholders to achieve yield and economic gains sustainably via the Science and Technology Backyard (STB) platform. STB involves agricultural scientists living in villages among farmers, advancing participatory innovation and technology transfer, and garnering public and private support. We identified multifaceted yield-limiting factors involving agronomic, infrastructural, and socioeconomic conditions. When these limitations and farmers' concerns were addressed, the farmers adopted recommended management practices, thereby improving production outcomes. In one region in China, the five-year average yield increased from 67.9% of the attainable level to 97.0% among 71 leading farmers, and from 62.8% to 79.6% countywide (93,074 households); this was accompanied by resource and economic benefits.
DOI:10.3390/su10020363URL [本文引用: 1]
URL [本文引用: 1]

A warming climate trend in the last three decades has been well documented around the global and this trend have had a considerable impact on agricultural productivity. Phenology is a plant growth progress that is largely driven by meteorological conditions. Phenological changes are vital indicators for changes in climate and other environmental conditions. In this study, the trends in winter wheat phenology for 1981-2009 were investigated based on phenological dates from 16 agro-experimental stations in the North China Plain (NCP). The study showed that the dates of sowing, emergence and dormancy delayed. On the other hand, the dates of green-up, anthesis and maturity advanced in most of the stations. The advance or delay of winter wheat phenology resulted in corresponding changes in the durations of the different growth stages. In most of the investigated stations, the durations from emergence to dormancy, dormancy to green-up, green-up to anthesis and in the entire period from emergence to maturity of winter wheat shortened during 1981-2009. However, the duration of anthesis to maturity slightly prolonged on the average by 0.9 days per decade. Observed changes in winter wheat phenology were functions of both climate and management practices, especially that of cultivated cultivar shift. The effect of crop cultivar shift was isolated from that of climate change on winter wheat phonological changes by comparing field observed phonological events in four stations with those simulated by the CERES (Crop Environment Resource Synthesis)-Wheat model. The results suggested that climate warming played a dominant role in phenological changes in winter wheat in the NCP. However, the effect of cultivar shift on winter wheat phenological changes was not entirely negligible. Moreover, correlation analysis on the durations of green-up to anthesis and anthesis to maturity against mean temperatures for the growth periods showed that temperature increase by 1 ℃ shortened the durations of green-up to anthesis and anthesis to maturity by 3.8 and 1.2 days, respectively. Understanding the response of crop development and phenology to climate change was critical for not only building in-depth insights into the impacts of climate change on crop development and productivity, but also on food security for the millions of people in the region and beyond.
URL [本文引用: 1]

A warming climate trend in the last three decades has been well documented around the global and this trend have had a considerable impact on agricultural productivity. Phenology is a plant growth progress that is largely driven by meteorological conditions. Phenological changes are vital indicators for changes in climate and other environmental conditions. In this study, the trends in winter wheat phenology for 1981-2009 were investigated based on phenological dates from 16 agro-experimental stations in the North China Plain (NCP). The study showed that the dates of sowing, emergence and dormancy delayed. On the other hand, the dates of green-up, anthesis and maturity advanced in most of the stations. The advance or delay of winter wheat phenology resulted in corresponding changes in the durations of the different growth stages. In most of the investigated stations, the durations from emergence to dormancy, dormancy to green-up, green-up to anthesis and in the entire period from emergence to maturity of winter wheat shortened during 1981-2009. However, the duration of anthesis to maturity slightly prolonged on the average by 0.9 days per decade. Observed changes in winter wheat phenology were functions of both climate and management practices, especially that of cultivated cultivar shift. The effect of crop cultivar shift was isolated from that of climate change on winter wheat phonological changes by comparing field observed phonological events in four stations with those simulated by the CERES (Crop Environment Resource Synthesis)-Wheat model. The results suggested that climate warming played a dominant role in phenological changes in winter wheat in the NCP. However, the effect of cultivar shift on winter wheat phenological changes was not entirely negligible. Moreover, correlation analysis on the durations of green-up to anthesis and anthesis to maturity against mean temperatures for the growth periods showed that temperature increase by 1 ℃ shortened the durations of green-up to anthesis and anthesis to maturity by 3.8 and 1.2 days, respectively. Understanding the response of crop development and phenology to climate change was critical for not only building in-depth insights into the impacts of climate change on crop development and productivity, but also on food security for the millions of people in the region and beyond.
DOI:10.1016/j.fcr.2016.05.007URL [本文引用: 1]
[本文引用: 1]
DOI:10.1016/j.eja.2014.04.007URL [本文引用: 1]

The yield gap (YG) between the potential yields (Yp) and the average on-farm yields (Ya) is an indicator of the potential improvement for crop production. Understanding how large the current gap is and how this gap has changed over the past few decades is essential for increasing wheat production to meet increased food demand in China. This paper describes a study conducted using an APSIM-Wheat model and farm-level crop yield to analyze the spatio-temporal distribution of the yield gap of winter wheat from 1981 to 2010 in the North China Plain. Nine varieties were calibrated and evaluated based on the data from 16 agro-meteorological experimental sites and then potential yields were estimated considering cultivar replacement. In addition, a trend pattern analysis of on-farm yields for the period 1981-2010 was conducted. Results revealed an estimated yield gap across the entire North China Plain region of 1140-6810 kg ha(-1), with a weight average of 3630 kg ha(-1) in 1981-2010. Expressed as a relative yield (yield gap % of potential yields), the range was 15-80%, and the weight average was 45%. Despite the negative effects of increasing temperature and decreasing radiation, the potential yields significantly increased by 45 kg ha-1 per year due to cultivar improvement. On-farm yields increased even more notably because of new cultivar selection, increased fertilizer application and other management improvements, but were stagnating in 32.3% of wheat areas, located mainly in Hebei province, Shandong province, Beijing and Tianjin. The improvement of on-farm yields have substantially contributed to yield gap spatio-temporal variation. As a result, the yield gap decreased from 4200 kg ha(-1) (56%) in 1981-1990 to 3000 kg ha(-1) (35%) in 2001-2010 at a rate of 69 kg ha(-1) per year. However, yields stagnation will expand to the northern Henan province without cultivar potential productivity improving, where yield gap was close to or less than 20% of the potential yields and proved difficult to reduce. To further improve the total production of winter wheat in the coming decades, efforts should be paid to break the potential ceiling and reduce the yield gap by breeding higher yield variety and introduction of new agricultural technology. (C) 2014 Elsevier B.V.
DOI:10.1016/j.fcr.2012.12.020URL [本文引用: 1]
[本文引用: 2]
[本文引用: 1]
URLPMID:26156832 [本文引用: 1]
DOI:10.4141/CJPS2011-266URL [本文引用: 1]

Zhang, Y., Feng, L., Wang, E., Wane, J. and Li, B. 2012. Evaluation of the APSIM-Wheat model in terms of different cultivars, management regimes and environmental conditions. Can. J. Plant Sci. 92: 937-949. Wheat is one of the most important crops in the world, and wheat models have been widely used to study yield responses to changes in management and climate. However, less information is available on how a wheat model performs in simulation of wheat. response to changes in varieties, sowing dates and planting densities across space. This study presents an evaluation of the APSIMWheat model using data from field experiments consisting of three sowing dates, two and three crop varieties and three planting densities in a split-split plot design at three ecological sites from 2008 to 2010 in the North China Plain. The results show that the APS1M-Wheat model could capture a large part of the variation in phenoloay, biomass and yield for the same variety across sites. However, errors of simulation in phenology and yield were increased with delay in sowing date, with the average absolute root mean square errors of 2 d, 3 d, and 3-4 d in phenology, and the normalized root mean square error (RMSEn) of 7-12 %, 11-16 %, 16-22 % in yield at early, medium, and late sowing dates, respectively. Simulation of yield achieved poor results with decreased planting density, with average RMSEn of 9-12 %, 11-12 %, and 16-19 % at high, medium, and low density, respectively. Additionally, the simulation behaved in a complex manner, and the errors varied greatly with different combinations of sowing dates and planting densities. These alerted us that the model should be used cautiously to simulate growth and yield over a wide range of sowing dates and planting densities. Improved modeling of the responses of wheat growth to extreme temperatures during winter and spring periods, and to varying planting densities is needed for better future prediction. Other areas of model improvements are also discussed.
DOI:10.1016/j.eja.2015.07.001URL [本文引用: 1]
DOI:10.1016/j.agwat.2015.11.005URL [本文引用: 1]
[本文引用: 1]
[本文引用: 1]
[本文引用: 1]
[本文引用: 1]
[本文引用: 1]
[本文引用: 1]
[本文引用: 1]
URL [本文引用: 1]

Winter wheat (Triticum aestivum L.) is the main cereal crop grown in the arid and semi-arid regions of the world. Average yields of winter wheat in many countries have increased by 40% over the past five decades due to the development of new cultivars, improvements of crop management practices and changes of favorable climate. However, water shortage is becoming an important factor limiting sustainable winter wheat production in many parts of the world. The greatest challenge for the winter wheat producers is to produce more wheat grain from limited water, and an available way to face the challenge is to improve winter wheat water productivity. Winter wheat water productivity had been significantly improved in the last 25 years, but there is still a big room for improving further. Selecting cultivars with more efficient water use is a key means to reduce water consumption in winter wheat production in the water-scarce regions. A field experiment was carried out during 2010 to 2011 and 2011 to 2012 growing seasons of winter wheat to clarify the variations in water consumption, grain yield, and water use efficiency (WUE), and their responses to water stress during the process of cultivar replacement in past decades. Seven cultivars of winter wheat released from 1950s to the current, in which each cultivar was once widely planted in north central Henan province during a certain decade, were taken as experimental materials. At the mean time, three irrigation regimes were designed including no irrigation after turning green (W0), irrigation applied only once at jointing (W1), and irrigation applied at jointing, and at filling, respectively (W2), to investigate dynamics of water consumption characteristics, yield components, harvest index, and WUE of winter wheat. Results showed that precipitation and timing of irrigation significantly impacted total water consumption and soil water extraction of winter wheat while different planting decades had insignificant effect on them. 1000-kernel weight during 1990s to the current kept more than 41 g, significantly higher than that during the earlier planting decades. During the 2010 to 2011 and 2011 to 2012 growing seasons, grain yields of winter wheat were increased by 396 and 362 kg/hm2, or 58.4 % and 41.8 % higher than the average yield across 1950s to the present, respectively; similarly, harvest indices were increased by 37.0 % and 18.0 %, an increase of 0.2 and 0.1 from the previous average indices; WUE was increased by 55.3 % and 40.8 %, an increase of 0.11 and 0.10 kg/m3, respectively. Improvement of grain yield is mainly attributable to the improved source to sink relationship, boosted 1000-kernel weight, and increased harvest index. Grain yield and WUE are significantly influenced by cultivar × soil water interaction, and can be significantly improved by supplemental irrigation applied at jointing and at filling stages of winter wheat.
URL [本文引用: 1]

Winter wheat (Triticum aestivum L.) is the main cereal crop grown in the arid and semi-arid regions of the world. Average yields of winter wheat in many countries have increased by 40% over the past five decades due to the development of new cultivars, improvements of crop management practices and changes of favorable climate. However, water shortage is becoming an important factor limiting sustainable winter wheat production in many parts of the world. The greatest challenge for the winter wheat producers is to produce more wheat grain from limited water, and an available way to face the challenge is to improve winter wheat water productivity. Winter wheat water productivity had been significantly improved in the last 25 years, but there is still a big room for improving further. Selecting cultivars with more efficient water use is a key means to reduce water consumption in winter wheat production in the water-scarce regions. A field experiment was carried out during 2010 to 2011 and 2011 to 2012 growing seasons of winter wheat to clarify the variations in water consumption, grain yield, and water use efficiency (WUE), and their responses to water stress during the process of cultivar replacement in past decades. Seven cultivars of winter wheat released from 1950s to the current, in which each cultivar was once widely planted in north central Henan province during a certain decade, were taken as experimental materials. At the mean time, three irrigation regimes were designed including no irrigation after turning green (W0), irrigation applied only once at jointing (W1), and irrigation applied at jointing, and at filling, respectively (W2), to investigate dynamics of water consumption characteristics, yield components, harvest index, and WUE of winter wheat. Results showed that precipitation and timing of irrigation significantly impacted total water consumption and soil water extraction of winter wheat while different planting decades had insignificant effect on them. 1000-kernel weight during 1990s to the current kept more than 41 g, significantly higher than that during the earlier planting decades. During the 2010 to 2011 and 2011 to 2012 growing seasons, grain yields of winter wheat were increased by 396 and 362 kg/hm2, or 58.4 % and 41.8 % higher than the average yield across 1950s to the present, respectively; similarly, harvest indices were increased by 37.0 % and 18.0 %, an increase of 0.2 and 0.1 from the previous average indices; WUE was increased by 55.3 % and 40.8 %, an increase of 0.11 and 0.10 kg/m3, respectively. Improvement of grain yield is mainly attributable to the improved source to sink relationship, boosted 1000-kernel weight, and increased harvest index. Grain yield and WUE are significantly influenced by cultivar × soil water interaction, and can be significantly improved by supplemental irrigation applied at jointing and at filling stages of winter wheat.
DOI:10.1016/j.fcr.2016.07.009URL [本文引用: 1]
[本文引用: 1]
[本文引用: 1]
DOI:10.1016/j.agrformet.2011.06.013URL [本文引用: 1]

Recent crop model projections have shown that crop production may benefit from warming, especially in the high latitudes, but hard evidence is limited. In this study we conducted correlation and regression analyses of climate records of seventy-two meteorological stations and records of corn yield over the period 1965-2008 in Northeast China. It was found that over these forty-four years. the diurnal mean, minimum and maximum temperatures during corn growing season increased on average by 0.31 degrees C, 0.42 degrees C and 0.23 degrees C every ten years, respectively. No significant change in precipitation was found, although differences between years were large. The daily minimum temperature was the dominant factor to corn production. Corn yield was significantly correlated with the daily minimum temperature in May and September. According to a regression analysis of the anomalies of corn yield and air temperature, a 1.0 degrees C increase in daily minimum temperature in May or September will lead to an increment of 303 kg ha(-1) or 284 kg ha(-1) in corn yield, respectively. Corn varieties with longer growth duration will profit most from the climatic changes but agronomic practices may have to be modified to address expected weather extremes such as droughts and periods with heavy rainfall. (C) 2011 Elsevier B.V.
DOI:10.1016/j.fcr.2013.01.003URL [本文引用: 1]

Environmental conditions greatly affect the growth of maize. To examine differences in phenological responses of maize (Zea mays L.) to climatic factors under different environmental conditions as induced by latitude, experiments were conducted from 2007 to 2010 at 34 sites in seven Chinese provinces located in the north spring maize region of China between latitudes 35 degrees 11' and 48 degrees 08'N in the cultivation of hybrid zhengdan958 (ZD958). Latitude is an important geographical factor which significantly affects temperature, sunshine hours, and the duration of crop growth. The findings of this study indicate that for every 10 increase in the latitude, northward, the growth durations of sowing to emergence and emergence to silking were significantly increased by 0.7 d and 1.25 d, respectively as a consequence of lowering temperatures (mean, maximum, and minimum temperatures). Reproductive growth duration (silking to maturity), which was significantly correlated with the precipitation, decreased by 0.8 d with each 1 degrees increase in latitude northward. At higher latitudes, the number of growing degree days (GDD) of maize vegetative growth duration (emergence to silking) was significantly higher, and the GDD of the reproductive growth duration were significantly lower. The average photoperiod during the photoperiod-sensitive phase of maize development across all the experimental sites was 14.9 h with a range of 13.7-15.6 h. Total leaf numbers increased from 18.7 to 23.7 with an average of 21.0 across all experimental sites. Significant and positive linear relationships were found to occur between both latitude and photoperiods and latitude and total leaf number. In the north China spring maize region, the mean growth duration of ZD958 was 143.73 d, which constituted 82.8% of the frost free period, the percentage increasing with higher latitude. These findings strongly indicate that in order to ensure high and stable production of maize in the north spring maize region of China, with its limited heat resources, especially in the high-latitude regions, there is a need to cultivate short-growth-duration cultivars. (C) 2013 Elsevier B.V.
URLPMID:26259458 [本文引用: 1]

Based on the multi-model datasets of three representative concentration pathway (RCP) emission scenarios from IPCC5, the response of yield and accumulative evapotranspiration (ET) of winter wheat to climate change in the future were assessed by VIP model. The results showed that if effects of CO2 enrichment were excluded, temperature rise would lead to a reduction in the length of the growing period for wheat under the three climate change scenarios, and the wheat yield and ET presented a decrease tendency. The positive effect of atmospheric CO2 enrichment could offset most negative effect introduced by temperature rising, indicating that atmospheric CO2 enrichment would be the prime reason of the wheat yield rising in future. In 2050s, wheat yield would increase 14.8% (decrease 2.5% without CO2 fertilization) , and ET would decrease 2.1% under RCP4.5. By adoption of new crop variety with enhanced requirement on accumulative temperature, the wheat yield would increase more significantly with CO2 fertilization, but the water consumption would also increase. Therefore, cultivar breeding new irrigation techniques and agronomical management should be explored under the challenges of climate change in the future.
URLPMID:26259458 [本文引用: 1]

Based on the multi-model datasets of three representative concentration pathway (RCP) emission scenarios from IPCC5, the response of yield and accumulative evapotranspiration (ET) of winter wheat to climate change in the future were assessed by VIP model. The results showed that if effects of CO2 enrichment were excluded, temperature rise would lead to a reduction in the length of the growing period for wheat under the three climate change scenarios, and the wheat yield and ET presented a decrease tendency. The positive effect of atmospheric CO2 enrichment could offset most negative effect introduced by temperature rising, indicating that atmospheric CO2 enrichment would be the prime reason of the wheat yield rising in future. In 2050s, wheat yield would increase 14.8% (decrease 2.5% without CO2 fertilization) , and ET would decrease 2.1% under RCP4.5. By adoption of new crop variety with enhanced requirement on accumulative temperature, the wheat yield would increase more significantly with CO2 fertilization, but the water consumption would also increase. Therefore, cultivar breeding new irrigation techniques and agronomical management should be explored under the challenges of climate change in the future.
[D].
[本文引用: 1]
[D].
[本文引用: 1]
[D].
[本文引用: 1]
[D].
[本文引用: 1]