


Research progress on genetic regulatory mechanism of seed color in soybean (Glycine max)
QIU Hong-Mei


通讯作者: * 邱丽娟, E-mail:qiulijuan@caas.cn;王跃强, E-mail:825164942@qq.com
收稿日期:2021-01-24接受日期:2021-05-17网络出版日期:2021-05-26
基金资助: |
Corresponding authors: * E-mail:qiulijuan@caas.cn;E-mail:825164942@qq.com
Received:2021-01-24Accepted:2021-05-17Published online:2021-05-26
Fund supported: |
作者简介 About authors
E-mail:qhm2001-2005@163.com

摘要
大豆种子颜色是重要的形态标记和进化性状, 在驯化过程中种皮从黑色逐渐演变成黄、绿、褐及双色, 子叶从绿色进化出黄色。深色种子中含有较多的天然色素——花色素, 具有药用和营养价值。因此, 种子颜色的遗传调控机制研究对进化理论和实际应用均具有重要意义。种子中色素含量及组分构成导致多样的种皮颜色, 其分子调控机制复杂。本文主要阐述了控制大豆种子颜色的遗传位点、相关基因与调控机制、类黄酮生物合成途径三方面的研究进展。具体介绍了9个经典遗传位点I、R、T、O、W1、K1、G、D1、D2和相关分子标记, 以及位点间的相互作用; 23个调控种子颜色的相关基因, 与部分基因等位变异的调控机制; 相关基因参与的类黄酮生物合成途径和主要代谢产物的生理功能。通过综述归纳了大豆种皮、种脐、子叶颜色的遗传调控研究进展, 利用遗传位点、基因、等位基因调控机制及类黄酮代谢途径绘制出调控网路, 以期为种子外观品质及花色苷组分遗传改良等研究提供参考。
关键词:
Abstract
The color of soybean seeds is an important morphological marker and evolutionary trait. During the process of domestication, seed coat has gradually evolved from black to yellow, green, black, brown, and bicolor, and cotyledons has evolved from green to yellow. The dark seed coat contains anthocyanins, which are natural pigments with medicinal and nutritional values. Therefore, it is of great importance to study the genetic regulation mechanism of seed color for evolutionary theory, variety breeding, and practical application. The pigment content and composition of seeds result in diverse seed coat colors through complex molecular regulatory mechanisms. In this paper, we described the research progress on genetic loci, related genes, regulatory mechanisms, and flavonoid biosynthesis pathways that controlling the color of soybean seeds. Specifically, we introduced the 9 classical genetic loci I, R, T, O, W1, K1, G, D1, D2, and related molecular markers, as well as the interactions between the loci; 22 related genes that controlling seed color, and the regulatory mechanisms of some allelic variants; as well as the physiological functions of the flavonoid biosynthesis pathways and major metabolites involved in the related genes. The progress of researches on genetic regulation of the color of seed coat, seed hilum, and cotyledon in soybean was reviewed, in addition the regulatory network was mapped with genetic loci, genes, allelic regulatory mechanisms, and flavonoid metabolic pathways, in order to provide references for the quality of seed appearance and genetic improvement of anthocyanin components.
Keywords:
PDF (2315KB)元数据多维度评价相关文章导出EndNote|Ris|Bibtex收藏本文
本文引用格式
邱红梅, 陈亮, 侯云龙, 王新风, 陈健, 马晓萍, 崔正果, 张玲, 胡金海, 王跃强, 邱丽娟. 大豆种子颜色遗传调控机制研究进展. 作物学报, 2021, 47(12): 2299-2313 DOI:10.3724/SP.J.1006.2021.14022
QIU Hong-Mei, CHEN Liang, HOU Yun-Long, WANG Xin-Feng, CHEN Jian, MA Xiao-Ping, CUI Zheng-Guo, ZHANG Ling, HU Jin-Hai, WANG Yue-Qiang, QIU Li-Juan.
大豆种皮色是基本的生物学性状, 也是重要的农艺性状, 可用来描述品种特性, 同时也是一种进化性状[1]。野生大豆一般为黑色种皮, 当前大豆品种多为黄色种皮[2]。在进化过程中, 大豆种皮从黑色逐渐演变成黄、绿、褐、双色[3]。每种颜色类型按深浅的不同又可细分若干种, 如黄色种皮有白黄、淡黄、浓黄、暗黄等。绿色种皮有淡绿、绿、深绿, 统称为青豆[4]。褐色种皮有茶色、淡褐、褐、深褐及红褐等。双色种皮分为虎斑和鞍挂2种, 底色一般为黄、褐或绿, 虎斑是种皮上有如老虎的条纹, 鞍挂是种脐两侧有如马鞍状的色斑[5,6]。大豆种皮色多样性高, 表型易于观察, 是大豆中较早开展遗传研究的性状之一[7]。1910年, Piper和Morss[8]首次报道大豆种皮色在杂交子代有分离现象, 但并未明确其遗传规律。1918年, Terao[9]报道子叶颜色与黄、绿种皮色的遗传关系。1928年, Owen[10]报道了黑色种皮与棕色茸毛位点连锁。
迄今, 种皮色从经典遗传到分子标记再到功能基因等研究都取得了显著进展[11,12]。此外, 由于黑豆的保健功能, 种皮中化学物质构成也备受关注[13]。研究表明, 深色种皮中主要含有花色苷和原花青素, 二者的组成比例和分布决定了种皮的着色程度[14]。花色苷属多酚类化合物, 来源类黄酮生物合成途径, 具有酚羟基结构。它对活性氧等自由基具有很强的捕捉能力, 因此抗氧化能力强, 具有药用价值。青豆种皮中主要含有叶绿素, 叶绿素含量决定了绿色程度[15]。控制绿色种皮的基因存在于野生大豆中, 属于古基因, 但野生大豆种皮的花色苷含量更高, 使种皮显现黑色。大豆经驯化后, 黄种皮的以食用为主; 黑豆列入中药名录, 常入中药[16,17,18]。随着人们生活水平的提高和健康意识的加强, 黑豆和青豆也逐渐受到市场欢迎[19]。鉴于种皮色、子叶色与外观品质及内在营养价值密切相关, 本文归纳了控制大豆种子颜色的遗传位点、相关基因与调控机制、类黄酮生物合成途径三方面的研究进展, 以期总结大豆种子颜色的遗传调控机制, 为种子外观品质及营养组分遗传改良等研究提供参考。
在已发表的综述中, 总结了控制大豆种皮色的5个经典遗传位点与基因的相关研究进展[20]。本综述汇总了控制大豆种子颜色的9个经典遗传位点, 并将位点-基因-等位基因的调控机制关联起来, 从点到线绘制了种子颜色的调控机制。此外将调控种皮色的相关基因与主要显色物质类黄酮代谢途径相
结合, 又从代谢通路绘制了花色苷含量的遗传调控途径, 最终以基因为节点, 将遗传位点与代谢产物关联起来, 绘制出大豆种子颜色遗传调控网路。
1 控制大豆种子颜色的遗传位点
大豆种皮色类型多样, 子叶颜色也有黄绿之分, 利用经典遗传学方法, 明确了9个遗传位点I、R、T、O、W1、K1、G、D1、D2, 分别位于8、9、6、8、13、11、1、1与11号染色体上(表1)[12,21-22]。此外, 还发现一个细胞质遗传位点CytG, 位于叶绿体内[9,23]。其中I和O位于同一染色体上, 但不存在连锁关系; G和D1位点连锁, 其他位点分属于不同染色体。这些位点间存在着相互作用, 遗传基础复杂[24,25]。I、R、T为控制种皮色的主效位点, I位点决定种皮是否含有花色素[26]。据报道I位点有4个等位基因, 分别为I、ii、ik、i, 显性关系顺序为I>ii>ik>i [27]。I基因型时, 种皮呈黄色, 我国北方育成品种多为该基因型。ii基因型时, 为黄种皮黑脐。ik基因型时, 种脐色素向外延伸至鞍区, 种皮为鞍挂(图1-c)。i基因型时, 种皮含有花色素, 呈现黑或褐种皮[28]。R位点有4个等位基因, 分别为R、R*、r、rm, 在i基因型背景下, 种皮分别显现黑色、黑色、褐色、虎斑(图1-d~f), 显性关系顺序为R>R*>rm>r [29]。T位点主要控制茸毛着色, 同时调控种皮的着色程度, 含有3个等位基因, 分别为T、t、tm, 表型分别为深褐种皮、棕色茸毛和浅褐种皮、灰色茸毛, 及同一株同时存在棕色和灰色茸毛、深褐和褐色种皮[30,31]。I、R、T位点间不同等位基因组合, 产生不同种皮颜色。IRT和IRt时, 种皮为黄色。ikRT和ikRt时, 种皮为鞍挂, 分别显现黑鞍和褐鞍。iRT和iRt时, 种皮为黑色与褐色。irT和irt时, 种皮为深褐色与褐色。Table 1
表1
表1控制种子颜色经典遗传位点信息
Table 1
遗传位点 Genetic locus | 连锁群 Linkage group | 染色体 Chromosome | 控制性状 Character | 等位 基因 Allele | 表型 Phenotype | 参考文献 Reference |
---|---|---|---|---|---|---|
I | A2 | 8 | 种皮、种脐是否含有花色素 The seed coat and hilum were controlled to contain anthocyanins. | I | 黄种皮黄脐 Fully yellow seed coat and hilum | Terao[9], Todd et al.[26] |
ii | 黄种皮黑脐 Yellow seed coat with black hilum | Terao[9], Tuteja et al.[27] | ||||
ik | 鞍挂Black saddle | Terao[9], Tuteja et al.[27] | ||||
i | 黑种皮或褐种皮 Black or brown seed coat | Terao[9], Clough et al.[28] | ||||
R | K | 9 | 种皮为黑色或褐色 The seed coat was black or brown. | R | 黑种皮Black seed coat | Owen[10], Song et al.[29] |
R* | 黑种皮Black seed coat | Zabala et al.[57] | ||||
r | 褐种皮Brown seed coat | Zabala et al.[57] | ||||
rm | 虎斑Black spots or concentric rings of black pigment superimposed on an otherwise brown seed coat | Zabala et al.[57] | ||||
T | C2 | 6 | 种皮的着色程度及茸毛颜色 Control the pigmentation degree of seed coat and the color of trichomes. | T | 深褐种皮、棕色茸毛 Dark brown seed coat, tawny trichomes | Owen[10], Zabala et al.[29,30] |
t | 褐种皮、灰色茸毛 Brown seed coat, gray trichomes | Owen[10], Zabala et al.[29,30] | ||||
tm | 深褐和褐色种皮、棕和灰色茸毛 Dark brown and brown seed coat, tawny and gray trichomes | Zabala et al.[29,30] | ||||
O | A2 | 8 | 种皮的褐色程度 Control the brown degree of seed coat. | O | 褐种皮Brown seed coat | Owen[10], Yang et al.[32] |
o | 红褐种皮Red-brown seed coat | Owen[10], Yang et al.[32] | ||||
W1 | G | 13 | 种皮着色程度及花的颜色 Control the pigmentation degree of seed coat and the color of flowers. | W1 | 黑种皮、紫花 Black seed coat and purple flowers | Owen[10], Zabala et al.[33] |
w1 | 浅褐种皮、白花 Buff seed coat and white flowers | Owen[10], Zabala et al.[33] | ||||
K1 | B1 | 11 | 控制色素在鞍区的分布 Control the distribution of pigment in saddle region. | K1 | 黄种皮Yellow seed coat | Cho et al.[34] |
k1 | 鞍挂Black saddle | Cho et al.[34] | ||||
G | D1a | 1 | 种皮、子叶是否含有叶绿素 The seed coat and cotyledon were controlled to contain chlorophyll. | G | 绿种皮、绿子叶 Green seed coat and cotyledon | Owen[10], Song et al.[21] |
g | 黄种皮、黄子叶 Yellow seed coat and cotyledon | Owen[10], Song et al.[21] | ||||
D1 | D1a | 1 | 种皮、子叶是否含有叶绿素 The seed coat and cotyledon were controlled to contain chlorophyll. | D1 | 黄种皮、黄子叶 Yellow seed coat and cotyledon | Fang et al.[35], Song J [12] |
d1 | 绿种皮、绿子叶 Green seed coat and cotyledon | Fang et al.[35], Song J [12] | ||||
D2 | B1 | 11 | 种皮、子叶是否含有叶绿素 The seed coat and cotyledon were controlled to contain chlorophyll. | D2 | 黄种皮、黄子叶 Yellow seed coat and cotyledon | Fang et al.[35], Song J [12] |
d2 | 绿种皮、绿子叶 Green seed coat and cotyledon | Fang et al.[35], Song J [12] | ||||
CytG | — | — | 种皮、子叶是否含有叶绿素 The seed coat and cotyledon were controlled to contain chlorophyll. | CytG | 黄种皮、黄子叶 Yellow seed coat and cotyledon | Guiamét et al.[39] |
cytG | 绿种皮、绿子叶 Green seed coat and cotyledon | Guiamét et al.[39] |
新窗口打开|下载CSV
图1
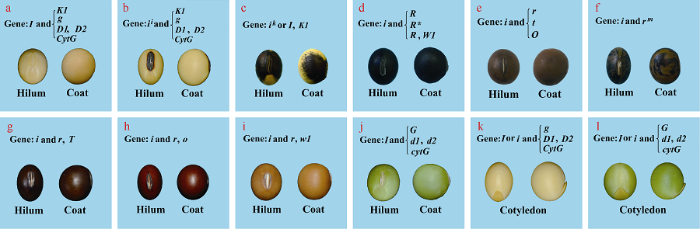
图1不同基因型种子颜色
a: 黄种皮黄脐; b: 黄种皮黑脐; c: 鞍挂; d: 黑种皮; e: 褐种皮; f: 虎斑; g: 深褐种皮; h: 红褐种皮; i: 淡褐种皮; j: 绿种皮; k: 黄色子叶; l: 绿色子叶。
Fig. 1Seed color of different genotypes
a: fully yellow seed coat and hilum; b: yellow seed coat with black hilum; c: black saddle; d: black seed coat; e: brown seed coat; f: black spots or concentric rings of black pigment superimposed on an otherwise brown seed coat; g: dark brown seed coat; h: red-brown seed coat; i: buff seed coat; j: green seed coat; k: yellow cotyledon; l: green cotyledon.
O、W1位点只有在纯合隐性基因型i r背景下才影响大豆种皮的颜色[7]。O位点主要控制种皮的褐色程度, 显性O等位基因为褐色种皮, 隐性o等位基因为红褐色种皮(图1-h)[32]。W1位点同时控制种皮色和花色, 显性W1除控制紫花外又可使种皮为黑色, 隐性w1可部分抑制R基因的作用, 呈现淡褐色种皮[33]。K1与I位点存在典型的上位效应, 显性K1等位基因不会影响种皮花色素分布和含量, 隐性k1在表观上克服显性I和ii等位基因对种皮着色的抑制, 使种皮脐部、鞍区或更大区域含有花色素[34]。基因型IK1、iiK1、ikK1、iK1, 分别与基因型I、ii、ik、i种皮色一致(图1-a~d)。Ik1、iik1的种皮色分别为黑色和鞍挂(黄底黑鞍), Ik1的种子在脱水前完全膨大期种皮外缘有一窄条的非黑色区域, 表明鞍区花色苷向更大的区域扩展, 而导致成熟的种子显现黑色, 其与i基因型黑种皮遗传机制不同。I基因型时, 种皮、子叶一般为黄色, 显性G等位基因使种皮显现绿色, 隐性g等位基因使种皮为黄色[21]。D1、D2位点同时为隐性基因型时, 种皮、子叶才呈现绿色[35,36,37]。CytG位点为隐性时, 种皮、子叶均为绿色[38,39]。Kohzuma等[40]研究发现, 日本大豆绿子叶资源99.5%由cytG基因控制, 而中国大豆绿子叶资源均为d1和d2基因型。
近年, 伴随生物技术发展, 获得了控制种子颜色分子标记位点30余个, 分别位于1、3、5、6、7、8、9、10、11、12、13、18、19、20号染色体上(图2), 这些标记位点表明调控种皮、子叶颜色的遗传基础复杂[41,42]。其中1、6、8、9、11、13号染色体的一些分子标记, 锚定到控制种皮、子叶色经典遗传位点区域。王吴彬等利用野生大豆染色体代换系, 在1 号染色体发现分子标记Sat_160与绿色种皮紧密连锁, 该区域位于G位点[43,44,45]。宋健[12]利用全基因组关联分析也获得G位点连锁的SNP分子标记, 并利用遗传群体, 精细定位该区域, 最终实现候选基因克隆。此外, 宋健[12]利用BSA法定位了2个控制子叶色位点, qCC1和qCC2, 分别包括经典遗传位点D1和D2。T位点位于6号染色体分子标记Satt207和Satt589之间, 同时获得与之关联的SNP标记1个[46]。O位点位于8号染色体分子标记Satt286和Satt365之间, 同时发现与之关联的SNP标记1个。I位点位于8号染色体分子标记A454.p2和AW132402之间, 与Sat_162、SSR53和BARCSO YSSR_08_0539连锁, 同时得到与之关联的SNP标记6个[47]。目前报道的与I位点连锁的分子标记最多, 表明该位点主效应显著, 易被检测到。R位点位于9号染色体分子标记A668-1和K387-1 之间, 与BARCSOYSSR_09_1506和BARCSOYSSR_09_1535连锁, 同时发现与之关联的SNP标记1个[48,49]。W1位点位于13号染色体分子标记Satt348和Satt160之间, 同时获得与之关联的SNP标记1个[50]。这些分子标记的发现为目标性状候选基因克隆及分子调控机制研究奠定了基础。
图2
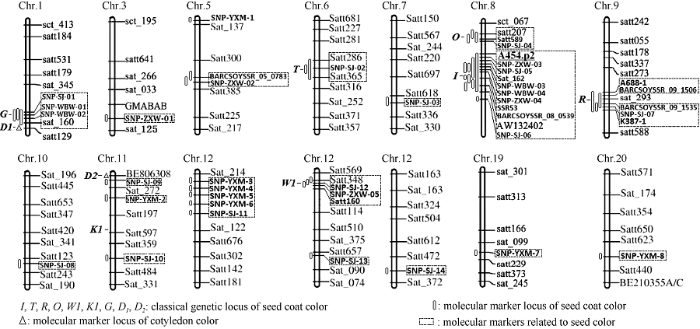
图2控制种子颜色遗传位点和相关分子标记
本图是在Song等[29]发表的遗传图谱基础上进行了修改。
Fig. 2Genetic loci and molecular markers of controlling seed coat color
This map was modified on the basis of the genetic map published by Song et al. [29]
2 大豆种子颜色相关基因及调控机制
目前已报道调控种皮颜色相关基因20个, 控制子叶颜色基因3个, 详细信息见表2。I位点内有3个基因, O位点内有2个基因, 其他经典遗传位点内均为1个基因。其中4、5、6、11号为花色素合成酶基因的调控基因, 12、13、14、23号为叶绿素调控基因, 其余15个基因均为类黄酮代谢途径酶基因。查尔酮合成酶(chalcon synthase, CHS)基因在大豆中含有9个同源基因, 其编码产物是类黄酮合成途径中的关键酶[51,52]。I位点内CHS1、3、4均有2个拷贝, 排列方式为CHS1-3-4和CHS4-3-1, 其中CHS1和CHS3可构成长倒置重复序列(long inverted repeats, LIR)[53]。该区域通过RNA干扰(RNAi)以组织特异性的方式沉默CHS基因的表达, 是自然界发生RNAi的典型例子之一[54,55]。I或ii等位基因, 可形成长倒置重复序列, 进而产生siRNAs, 使CHS基因沉默, 种皮为黄色[56]。ii基因型时CHS基因的拷贝数平均为10.6个, 较I基因型多3个拷贝数, 导致种脐含有花色素。隐性i基因型时, 位点内发生大片段缺失, 不能产生siRNAs, 丧失对CHS基因表达的抑制作用, 种皮、种脐为深色[57]。I位点对种皮色的调控机制复杂, 不仅有特殊的结构, 还发现3个靶向调控基因AGO5、DCL2a、DCL2b。AGO5位于K1位点, 是Argonaute基因家族的一员, 可靶向切割CHS基因的长倒置重复序列, 产生siRNAs调控种皮颜色[58]。通过转录组和基因组重测序发现, Clark18a中隐性k1的ago5基因存在129 bp的缺失, 导致转录本中第7个外显子缺失[59]。该基因丧失产生siRNAs的功能, 使种皮鞍区CHS的mRNA富集, 形成黑鞍。对不同资源材料的隐性k1位点进行克隆测序发现, 不同的缺失长度导致种脐两侧色斑形状差异, 但具体机制未见报道。DCL2a/2b编码产物为内啡肽酶同源物, 与AGO5基因的作用机制相同, 也可靶向切割CHS基因的长倒置重复序列, 产生22个碱基的siRNAs[60]。当DCL2a和DCL2b被编辑突变后, 丧失对长重复序列的切割功能, 减少对CHS基因的抑制, 使种皮为褐色。Table 2
表2
表2调控种子颜色的相关基因信息
Table 2
序号 No. | 基因编号(v2.1) Gene ID (v2.1) | 基因名称 Gene name | 英文缩写 Abbreviation | 遗传位点 Genetic locus | 编码产物注释 Annotation of coding products | 参考文献 Reference |
---|---|---|---|---|---|---|
1 | Glyma.08G109500 | 查尔酮合成酶1 Chalcone synthase 1 | CHS1 | I | 类黄酮合成途径酶:合成柚皮苷查尔酮 Flavonoid biosynthesis pathway enzyme: synthesis of naringin chalcone | Akada et al.[51] |
2 | Glyma.08G110300 | 查尔酮合成酶3 Chalcone synthase 3 | CHS3 | I | 类黄酮合成途径酶:合成柚皮苷查尔酮 Flavonoid biosynthesis pathway enzyme: synthesis of naringin chalcone | Senda et al.[52] Cho et al.[53] |
3 | Glyma.08G109200 | 查尔酮合成酶4 Chalcone synthase 4 | CHS4 | I | 类黄酮合成途径酶:合成柚皮苷查尔酮 Flavonoid biosynthesis pathway enzyme: synthesis of naringin chalcone | Liu et al.[54] Tuteja et al.[55] |
4 | Glyma.11G190900 | Argonaute 5蛋白 Argonaute 5 protein | AGO5 | K1 | 调控CHS siRNAs在种皮的分布 Regulation of CHS siRNAs distribution in seed coat | Rogers et al.[58] Song et al.[59] |
5 | Glyma.09G025300 | 内啡肽酶同源物2a Endorphinase homolog 2a | DCL2a | — | 切割含长LIR的转录本,生成22nt siRNA LIR-derived transcripts are predominately processed by GmDCL2 into 22-nt siRNAs | Jia et al.[60] |
6 | Glyma.09G025400 | 内啡肽酶同源物2b Endorphinase homolog 2a | DCL2b | — | 切割含长LIR的转录本,生成22nt siRNA LIR-derived transcripts are predominately processed by GmDCL2 into 22-nt siRNAs | Jia et al.[60] |
7 | Glyma.06G202300 | 黄烷酮3’-羟化酶 Flavanone 3’-hydroxylase | F3’H | T | 类黄酮合成途径酶:催化黄烷酮羟基化 Flavonoid biosynthesis pathway enzymes: catalyzing the hydroxylation of flavanones | Toda et al.[61] Guo et al.[62] |
8 | Glyma.13G072100 | 黄烷酮3’,5’-羟化酶 Flavanone 3’,5’-hydroxylase | F3’5’H | W1 | 类黄酮合成途径酶:催化黄烷酮羟基化 Flavonoid biosynthesis pathway enzymes: catalyzing the hydroxylation of flavanones | Zabala et al.[33] |
9 | Glyma.08G062000 | 花青素还原酶 Anthocyanidin reductase | ANR1 | O | 类黄酮合成途径酶:形成原花青素 Flavonoid biosynthesis pathway enzymes: procyanidins production | Yang et al.[32] |
10 | Glyma.08G062100 | 花青素还原酶 Anthocyanidin reductase | ANR2 | O | 类黄酮合成途径酶:形成原花青素 Flavonoid biosynthesis pathway enzymes: procyanidins production | Yang et al.[32] |
11 | Glyma.09G235100 | R2R3 MYB转录因子 Transcripion foctor R2R3 MYB | R2R3 MYB | R | 转录因子:调控ANS基因的表达 Transcription factors: regulation of ANS gene expression | Yan et al.[63] Zabala et al.[64] |
12 | Glyma.01G198500 | CAAX自身免疫蛋白酶 CAAX protease self-immunity | CPSI | G | 防止叶绿素降解使成熟种皮保持绿色 Prevent chlorophyll degradation to keep the mature seed coat green | Xie et al.[65] Wang et al.[66] |
13 | Glyma.01g214600 | 保持绿色蛋白1 Protein stay-green 1 | SGR1 | D1 | 使成熟种子保持绿色 Mature seeds stay-green | Fang et al.[34] 宋健[12] Fang et al.[34] Song J[12] |
14 | Glyma.11g027400 | 保持绿色蛋白2 Protein stay-green 2 | SGR2 | D2 | 使成熟种子保持绿色 Mature seeds stay-green | Fang et al.[34] 宋健[12] Fang et al.[34] Song J[12] |
15 | Glyma.20G241500 | 查尔酮异构酶1A Chalcone isomerase 1A | CHI1A | — | 类黄酮合成途径酶:催化分子内环化反应 Flavonoid synthesis pathway enzymes: catalyzing intramolecular cyclization | Kovinich et al.[63] |
16 | Glyma.20G241700 | 查尔酮异构酶2 Chalcone isomerase 2 | CHI2 | — | 类黄酮合成途径酶:催化分子内环化反应 Flavonoid synthesis pathway enzymes: catalyzing intramolecular cyclization | Kovinich et al.[63] |
17 | Glyma.02G048400 | 黄烷酮3-羟化酶 Naringenin 3-dioxygenase | F3H | Wp | 类黄酮合成途径酶:催化黄烷酮羟基化 Flavonoid biosynthesis pathway enzymes: catalyzing the hydroxylation of flavanones | Zabala et al.[64] |
18 | Glyma.14G072700 | 二氢黄酮醇还原酶1 Dihydroflavonol 4-reductase 1 | DFR1 | W3 | 类黄酮合成途径酶:形成无色花青素 Flavonoid synthesis pathway enzymes: formation of colorless anthocyanins | Xu et al.[65] |
19 | Glyma.17G252200 | 二氢黄酮醇还原酶2 Dihydroflavonol 4-reductase 2 | DFR2 | W4 | 类黄酮合成途径酶:形成无色花青素 Flavonoid synthesis pathway enzymes: formation of colorless anthocyanins | Xu et al.[65] |
20 | Glyma.01G214200 | 花青素合成酶2 Anthocyanidin synthase 2 | ANS2 | — | 类黄酮合成途径酶:形成花色素 Flavonoid biosynthesis pathway enzymes: anthocyanin formation | Kovinich et al.[68] |
21 | Glyma.11G027700 | 花青素合成酶3 Anthocyanidin synthase 3 | ANS3 | — | 类黄酮合成途径酶:形成花色素 Flavonoid biosynthesis pathway enzymes: anthocyanin formation | Kovinich et al.[68] |
22 | Glyma.08G066800 | UDP类黄酮葡萄糖基转移酶 UDP-flavonoid 3-O-glucosyltransferase | UF3GT | — | 类黄酮合成途径酶:形成花色苷 Flavonoid biosynthesis pathway enzymes: anthocyanin formation | Kovinich et al.[68] |
23 | — | 光系统Ⅱ反应中心M亚基 Photosystem II reaction center M subunit | Psbm | — | 具有叶绿素b降解功能 Degradation of chlorophyll b | Kohzuma et al.[40] |
新窗口打开|下载CSV
F3’H (flavanone-3’-hydroxylase)基因位于T位点, 编码类黄酮-3’-羟基化酶, 是细胞色素P450依赖的单加氧酶[61]。具有保守结构域GGEK, 能与血红素结合催化黄烷酮羟基化。隐性t位点, 含有3种等位基因, 分别有单碱基缺失和外显子区非同义突变, 最终导致基因功能缺失, 形成褐色种皮、灰色茸毛[62]。tm等位基因时, F3’H基因中插入一个20.5 kb-CACTA转座子。该转座子在生育期间的一些细胞中跳出, 恢复T基因型。导致同一株即有棕色又有灰色茸毛, 种皮也分别显现深褐和褐色[31]。F3’5’H (flavanone-3’,5’-hydroxylase)基因位于W1位点, 编码类黄酮-3’,5’-羟基化酶, 也是细胞色素P450依赖的单加氧酶, 催化黄烷酮羟基化[33]。隐性w1基因型时, F3’5’H基因的第3个外显子插入53 bp短序列, 导致移码突变缺失羟基化功能, 表型为白花、淡褐种皮。ANR1/2 (anthocyanidin reductase)位于O位点内, 在染色体上串联排列, 编码产物为花青素还原酶, 可合成原花青素, 通过RNAi干涉验证了它改变种皮色的功能, 但遗传调控机制未见报道[32]。R2R3 MYB基因为转录因子, 位于R位点, 可调控花青素合成酶(anthocyanidin synthase, ANS)基因的表达[63]。r基因型时, R2R3 MYB基因有一个碱基C的缺失, 导致移码翻译产物提前终止, 比成熟的完整蛋白质短152个氨基酸[64]。R2R3 MYB基因的第2个内含子插入13 kb-CACTA转座子, 根据表型又分为2种等位基因型: R*和rm。R*基因型时, 转座子的CACTA序列具有更高的甲基化水平, 转座酶无法与之结合, 缺乏转座活性, R2R3 MYB基因的转录拼接得以正常进行, 尽管转录水平较低, 足够刺激花青素的产生, 从而形成黑色种皮。rm基因型时, CACTA区的甲基化水平较低, 转座酶可与之结合, 阻止R2R3 MYB基因的内含子剪接, 导致棕色种皮背景; 同时在一些细胞中转座子被切除, R2R3 MYB得以转录调控ANS基因产生花色素, 形成黑色条纹。
CPSI基因编码CAAX自身免疫蛋白酶, 位于G位点, 可防止叶绿素降解使成熟种皮为绿色[65]。隐性g基因第8内含子的末端发生A-G碱基突变, 使基因翻译提前终止, 种皮为黄色[66]。G基因也可以调控种子的休眠, 在驯化过程中受到选择, 表明种皮色调控基因也存在其他重要功能。SGR1/2基因编码保持绿色蛋白, 位于D1和D2位点内。SGR基因通过转录或转录后水平, 调控叶绿素降解酶而参与叶绿素降解[67]。显性D1D2具有调控叶绿素降解功能, 子叶呈黄色。隐性d1d2由于单碱基的缺失及大片段插入, 缺失调控叶绿素降解功能, 使子叶保持绿色[12]。Psbm基因编码光系统II反应中心M亚基, 位于CytG位点内, 为母系遗传基因。隐性psbm基因中蛋白编码区有5 bp的碱基插入, 产生移码突变导致基因翻译提前终止, 使叶绿素b降解受阻。显性Psbm基因使成熟种子为黄皮黄子叶, 隐性psbm基因使成熟种子为绿皮绿子叶[40]。表2中15~22号为类黄酮合成酶基因, 分别为查尔酮异构酶1A/2 (chalconce isomerase, CHI1A/2)基因、黄烷酮-3-羟化酶(flavanone-3-hydroxylase, F3H)基因、二氢黄酮醇还原酶1/2 (dihydroflavonol 4-reductase, DFR1/2)基因、花青素合成酶2/3 (anthyocyanidin synthase, ANS2/3)基因、UDP类黄酮葡萄糖基转移酶(UDP- flavonoid 3-O-glucosyltransferase, UF3GT)基因[68]。其中F3H、DFR1、DFR2分别位于控制花色的Wp、W3、W4的位点内。这些基因在不同颜色的种皮中差异表达, 并且随着种子发育时期而变化, 进而调控种皮色[69,70]。综上可知, 种子颜色由多基因调控, 大部分基因的遗传调控机制已经解析(表3), 但仍有部分基因的分子调控机制未知。
Table 3
表3
表3控制种子颜色等位基因的调控机制
Table 3
基因/编号 Gene ID | 突变基因型 Mutant genotype | 种子颜色 Seed color | 变异方式 Variation | 检测方法 Detection method | 参考文献 Reference |
---|---|---|---|---|---|
I Glyma.08G109500 Glyma.08G110300 Glyma.08G109200 | ii | 黄种皮黑脐 Yellow seed coat with black hilum | CHS基因的拷贝数增加 The copy number of CHS gene increased | BAC重测序 BAC resequencing | Cho et al.[53] |
ik | 鞍挂 Black saddle | 基因间序列缺失 Deletion of intergenic sequencesl | BAC重测序 BAC resequencing | Cho et al.[53] | |
i | 深色种皮 Dark pigment seed coat | 基因及基因间序列大片段缺失 Large deletions of genes and intergenic sequences | BAC重测序 BAC resequencing | Cho et al.[53] | |
R Glyma.09G235100 | R* | 黑种皮 Black seed coat | 第2内含子插入CACTA转座子及CACTA序列的甲基化 Insertion of the second intron into the CACTA transposon and methylation of the CACTA sequence | 长片段PCR扩增、表达谱分析、甲基化检测 Long fragment PCR amplification, expression profile analysis, methylation detection | Zabala et al.[64] |
r | 褐种皮 Brown seed coat | 第2外显子单碱基C缺失 Single base C deletion in exon 2 | 克隆测序 Cloning and sequencing | Zabala et al.[64] | |
rm | 虎斑 Black spots or concentric rings of black pigment superimposed on an otherwise brown seed coat | 第2内含子插入CACTA转座子 Insertion of the second intron into the CACTA transposon | 长片段PCR扩增、表达谱分析、甲基化检测 Long fragment PCR amplification, expression profile analysis, methylation detection | Zabala et al.[64] | |
T Glyma.06G202300 | t | 褐种皮 Brown seed coat | 第3外显子单碱基A的缺失或非同义突变或单碱基C的缺失 Deletion of single base A or non synonymous mutation or deletion of single base C in exon 3 | PCR测序 PCR sequencing | Guo et al.[62] |
tm | 深褐和褐色种皮 Dark brown and brown seed coat, | 第1内含子插入CACTA转座子 The first intron was inserted into the CACTA transposon | 长片段PCR扩增 Long fragment PCR amplification | Zabala et al.[31] | |
W1 Glyma.13G072100 | w1 | 淡褐种皮 Buff seed coat | 第3外显子插入53 bp The third exon was inserted 53 bp sequence | 克隆测序 Cloning and sequencing | Guo et al.[62] |
K1 Glyma.11G190900 | k1 | 鞍挂 Black saddle | 第7外显子129 bp的缺失 Deletion of 129 bp sequence in exon 7 | 转录组与小RNA测序、PCR测序、全基因组深度重测序 Ranscriptome and micro RNA sequencing, PCR sequencing, whole genome deep resequencing | Cho et al.[34] |
G Glyma.01G198500 | g | 黄种皮 Yellow seed coat | 第8内含子末端发生碱基突变 A base mutation occurred at the end of intron 8 | 克隆测序 Cloning and sequencing | Wang et al.[66] |
D1 Glyma.01g214600 | d1 | 绿种皮、绿子叶 Green seed coat and cotyledon | 第2外显子单碱基T缺失 Single base T deletion in exon 2 | 基因组重测序、克隆测序 Genome resequencing, cloning and sequencing | Fang et al.[35], Song J[12] |
D2 Glyma.11g027400 | d2 | 绿种皮、绿子叶 Green seed coat and cotyledon | 322 bp重复插入 322 bp sequence repeated insertion | 基因组重测序、克隆测序 Genome resequencing, cloning and sequencing | Fang et al.[35], Song J[12] |
CytG | cytG | 绿种皮、绿子叶 Green seed coat and cotyledon | 5 bp插入 5 bp sequence insertion | 对叶绿体基因组广泛的测序 Extensively sequenced the chloroplast genome | Kohzuma et al.[40] |
新窗口打开|下载CSV
3 大豆类黄酮生物合成途径及主要产物的生理功能
迄今, 已报道23个种子颜色调控基因, 除G、D1、D2、CytG基因外, 其他19个均在类黄酮合成途径通路上。因此充分了解类黄酮生物合成途径, 可更好地明晰种皮色的调控机制。类黄酮是次生代谢产物中重要的一类, 其核心结构是由15个碳原子构成的苯丙烷[71]。其中2个六碳芳香环(A环和B环)被1个三碳杂环(C环)所连接, 在代谢通路上通过对C环的重排、氧化、烷化、糖基化等最终形成稳定的原花青素或花色苷[72]。二者为有色化合物, 使种皮、花和茸毛着色[73]。类黄酮生物合成属于次生代谢, 易受环境等因素影响, 调控机制非常复杂, 目前仍存在着未知代谢通路[74]。通过归纳总结已有研究成果, 将已报道的19个基因和相关代谢产物关联, 绘制出种皮色相关基因调控的大豆类黄酮生物合成途径(图3)。图3
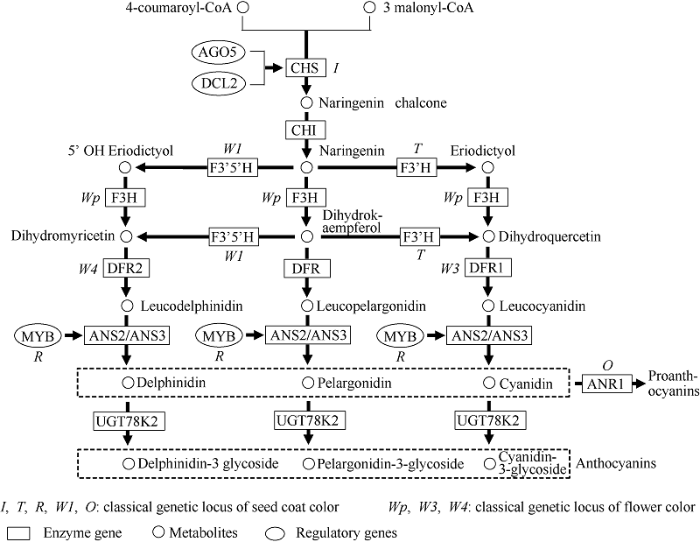
图3种皮颜色相关基因调控的大豆类黄酮生物合成途径
修改自Zabala等[64]。
Fig. 3Flavonoid biosynthesis pathway regulated by seed coat color related genes in legumes
The above figure was modified from Zabala et al. [64]
具体过程如下, 1分子香豆酰辅酶A (4-coumaroyl-CoA)和3分子丙二酰辅酶A (3 malonyl-CoA)在查尔酮合成酶(CHS)的作用下形成柚皮苷查尔酮(naringenin chalcone)。DCL2a/b和AGO5基因通过调节CHS基因的mRNA含量, 进而调控查尔酮合成酶的含量和活性, 由于该酶是类黄酮合成途径第1个酶, 对整个代谢途径都有限速调控功能。柚皮苷查尔酮在查尔酮异构酶(CHI)的催化下形成无色的三羟基黄烷酮(Naringenin)。三羟基黄烷酮之后产生3个分支, 分别在黄烷酮3’-羟化酶(F3’H)、黄烷酮3-羟化酶(F3H)、黄烷酮3’,5’-羟化酶(F3’5’H)的催化形成四羟基黄烷酮(eriodictyol)、二氢山萘酚(dihydrokaempferol)、五羟基黄烷酮(5’ OH Eriodictyol)[75]。四羟和五羟基黄烷酮在F3H的催化下形成二氢槲皮素(dihydroquercetin)和二氢杨梅素(dihydromyricetin), 二氢山萘酚在F3’H和F3’5’H的催化下也可形成二氢槲皮素和二氢杨梅素。二氢槲皮素、二氢山萘酚和二氢杨梅素在二氢黄酮醇还原酶(DFR)的催化下进一步还原形成不稳定的无色花色素, 然后再在花青素合成酶(ANS)的作用下形成花色素, 分别为矢车菊素(cyanidin)、天竺葵花素(pelargonidin)、翠雀花素(delphinidin)[76]。R位点的MYB转录因子基因调控ANS2/3基因的表达, 进而调控ANS酶的含量和活性, 调节花色素的含量, 影响种皮色[77]。花色素作为前体, 在花青素还原酶(ANR)和UDP类黄酮葡萄糖基转移酶(UF3GT)的催化下分别形成原花青素(proanthocyanins)和花色苷(anthocyanins)。UF3GT基因在种子成熟过程中表达量不断升高, 在种子成熟的中后期达到峰值。类黄酮生物合成途径的两大产物分别为原花青素和花色苷。花色苷含量高于原花青素时, 种皮呈黑色, 反之成褐色。当原花青素高时, 二者比例决定褐色程度[78]。
原花青素和花色苷, 是植物中广泛存在的水溶性天然色素[79]。近年, 天然色素的功能和重要性逐渐凸显, 目前已应用于腊肠、果汁饮品、牙膏、再造烟叶等的染色上[80,81,82,83]。黑豆种皮富含原花青素和花色苷, 有研究报道将其应用于蚕丝织物染色[84]。关于黑豆皮的药用研究已有千年历史, 《名医别录》载, 黑豆皮中药称“料豆衣”。料豆衣味甘性凉, 能解毒利尿, 经常服食对增强造血功能、强健骨骼等大有裨益[85]。现代医学研究表明, 花色苷通过清除自由基, 具有明显的抑制高血脂、动脉硬化等心血管病的效果[86]。徐金瑞[87]通过体外试验证实, 黑豆皮中的花色苷具有显著改善生物体内脂质代谢, 降低氧化应激水平, 减少血管内皮细胞的过氧化损伤, 并具有间接舒张血管的作用。张继曼[88]研究表明, 黑豆红花色苷还具有降低糖尿病小鼠高血糖的作用。综上可知, 大豆种皮中的花色素可作为天然染料, 更具药用价值。因此充分解析种皮色遗传调控机制, 可依据多元化市场需求分子设计改良大豆种子颜色, 以期最大发挥大豆的价值。
4 结语与展望
历经100多年, 种皮及子叶色的遗传规律基本明确[89], 相关位点、基因及互作机制也逐步被解析。遗传学分析发现控制大豆种皮色的位点有I、R、T、O、W1、K1、G、D1、D2、CytG[21]。利用遗传群体连锁分析和自然群体的关联分析, 获得控制种皮色的分子标记位点30余个, 除K1、CytG其他8个经典遗传学位点均有与之连锁的分子标记[41,42]。T位点的GmF3’H基因首个被克隆, 随后通过图位克隆、转录组和基因组重测序等方法, 相继获得其他9个位点的候选基因[61]。这些基因都与色素的代谢有关, 其中I、T、W1、O位点内为类黄酮生物合成途径关键酶基因, R和K1位点内分别为类黄酮生物合成途径酶基因的转录因子及调控基因, G、D1、D2、CytG位点内为叶绿素调控基因。此外通过基因编辑及目标基因的差异表达分析发现, 经典遗传位点外其他基因也有调控种皮色的功能, 如DCL2、CHI1A/2、F3H、DFR1/2、ANS2/3、UF3GT基因等[32,60,68]。这些基因的编码产物均参与类黄酮合成途径, 表明类黄酮代谢与种皮色密切相关, 代谢产物含量与组成比例决定了种皮颜色。纵览种子颜色的遗传调控研究历史, 20世纪以经典遗传研究为主, 21世纪以分子调控机制及网络解析为主。位点间互作、基因间的调控以及类黄酮代谢通路, 共同构成种皮色调控网络[72]。此网络包含的调控基因数目众多, 调控机制复杂。不仅有传统遗传调控, 还有表观遗传调控。如I位点存在基因结构变异, 同时存在RNA干涉现象[51,53]。此位点更是重要的驯化位点, 在进化过程中出现大片段反转、重复插入等, 导致种皮色从黑到黄的变化[54]。T位点有等位基因的序列变化[62], 同时存在转座子插入调控, 导致同株不同色现象[63]。R位点调控机制更为复杂, 有单碱基缺失、有转座子插入、更有甲基化程度的改变[64]。由于受遗传背景的影响, 同一个性状如黄色的遗传也不同, 既有显性[26], 也有隐性[21]。I基因型时, 在G基因控制下绿色为显性, 黄色为隐性[65]。此外G基因还有调控种子休眠的功能, 在驯化过程中受到选择[66]。由此可知调控种皮色的基因, 也有可能存在其他重要的未知功能。与种子颜色遗传研究相比, 我国开展黑大豆的药用功能研究更早, 距今已有千年历史[85]。现代医学研究证实, 黑豆皮中的花色苷具有促进脂类代谢良性循环、预防心脑血管疾病、降血糖等功能[86,87,88]。近年来, 由于生物染料具有安全健康等优势, 黑豆花色素也应用于蚕丝等织物的染色[84]。研究表明深色大豆种子含有多种花色苷, 种质资源间花色苷组分含量差异显著, 抗氧化能力也不尽相同[90]。大豆中花色苷含量受多基因调控, 表现出数量性状的遗传特征。这与本文关于种子颜色遗传调控归纳总结结果一致, 目前已知近20个基因参与调控类黄酮代谢途径。还需要进一步筛选挖掘调控种子颜色的结构基因、转录因子等, 完善相关基因的功能和分子调控机制, 补充绘制调控网络。随着技术的发展以及研究的深入, 更多遗传调控机制会被发现, 不仅能进一步完善已有种子颜色的调控网络, 也可利用大数据, 构建新种子颜色的代谢网络[91]。进而实现种子颜色的定向分子设计与改良, 创制出如黑子叶、紫种皮等更具药用、营养价值的大豆, 为满足多样化消费需求提供科技支撑。
参考文献 原文顺序
文献年度倒序
文中引用次数倒序
被引期刊影响因子
[本文引用: 1]
[本文引用: 1]
DOIURL [本文引用: 1]
[本文引用: 1]
[本文引用: 1]
[本文引用: 1]
[本文引用: 1]
DOIURL [本文引用: 1]
DOIPMID [本文引用: 1]

The R gene of soybean is involved in anthocyanin synthesis in the seed coat, and its r-m allele conditions a variegated distribution of black spots and/or concentric rings of pigment superimposed on an otherwise brown seed coat. We describe an unusual feature of r-m that causes expression at the R locus to switch between active and inactive phases both somatically and germinally. Non-heritable somatic changes of the allele produce single plants containing mixtures of seed with different coat colors (black+striped or brown+striped). Heritable changes of the r-m allele are manifested in progeny plants which produce all black seed or all brown seed. Surprisingly, subsequent generations from revertant sublines show continued instability of the allele such that brown revertants (r*/r*) or homozygous black seed revertants (R*/R*) can give rise to striped or striped+black-seeded plants. Thus, the revertants produced by the r-m allele are not stable but interconvert between all three forms (R*, r*, and r-m) at detectable frequencies. Mutability of the r-m allele in a different genetic background has also been found after inter-crossing various soybean genotypes.
[本文引用: 2]
[本文引用: 2]
[本文引用: 1]
[本文引用: 6]
PMID [本文引用: 10]
PMID [本文引用: 1]

The dominant I gene inhibits accumulation of anthocyanin pigments in epidermal cells of the soybean seed coat. We compared saline-soluble proteins extracted from developing seed coats and identified a 35-kilodalton protein that was abundant in Richland (genotype I/I, yellow) and much reduced in an isogenic mutant line T157 (genotype i/i, imperfect black seed coats). We purified the 35-kilodalton protein by a novel procedure using chromatography on insoluble polyvinylpolypyrrolidone. The 35-kilodalton protein was composed primarily of proline, hydroxyproline, valine, tyrosine, and lysine. Three criteria (N-terminal amino acid sequence, amino acid composition, and sequence of a cDNA) proved that the seed coat 35-kilodalton protein was PRP1, a member of a proline-rich gene family expressed in hypocotyls and other soybean tissues. The levels of soluble PRP1 polypeptides and PRP1 mRNA were reduced in young seed coats with the recessive i/i genotype. These data demonstrated an unexpected and novel correlation between an anthocyanin gene and the quantitative levels of a specific, developmentally regulated cell wall protein. In contrast, PRP2, a closely related cell wall protein, was synthesized later in seed coat development and was not affected by the genotype of the I locus.
[本文引用: 15]
[本文引用: 15]
[本文引用: 1]
[本文引用: 1]
PMID [本文引用: 1]

The dominant I gene inhibits accumulation of anthocyanin pigments in the epidermal layer of soybean (Glycine max) seed coats. Seed-coat color is also influenced by the R locus and by the pubescence color alleles (T, tawny; t, gray). Protein and RNA from cultivars with black (i,R,T) and brown (i,r,T) seed coats are difficult to extract. To determine the nature of the interfering plant products, we examined seed-coat extracts from Clark isogenic lines for flavonoids, anthocyanins, and possible proanthocyanidins by thin-layer chromatography. We show that yellow seed-coat varieties (I) do not accumulate anthocyanins (anthocyanidin glycosides) or proanthocyanidins (polymeric anthocyanidins). Mature, black (i,R,T) and imperfect-black (i,R,t) seed coats contained anthocyanins, whereas mature, brown (i,r,T) and buff (i,r,t) seed coats did not contain anthocyanins. In contrast, all colored (i) genotypes tested positive for the presence of proanthocyanidins by butanol/ HCl and 0.5% vanillin assays. Immature, black (i,R,T) and brown (i,r,T) seed coats contained significant amounts of procyanidin, a 3[prime],4[prime]-hydroxylated proanthocyanidin. Immature, black (i,R,T) or brown (i,r,T) seed-coat extracts also tested positive for the ability to precipitate proteins in a radial diffusion assay and to bind RNA in vitro. Imperfect-black (i,R,t) or buff (i,r,t) seed coats contained lesser amounts of propelargonidin, a 4[prime]-hydroxylated proanthocyanidin. Seed-coat extracts from these genotypes did not have the ability to precipitate protein or bind to RNA. In summary, the dominant I gene controls inhibition of not only anthocyanins but also proanthocyanidins in soybean seed coats. In homozygous recessive i genotypes, the T-t gene pair determines the types of proanthocyanidins present, which is consistent with the hypothesis that the T locus encodes a microsomal 3[prime]-flavonoid hydroxylase.
DOIURL [本文引用: 1]
[本文引用: 1]
[本文引用: 1]
[本文引用: 1]
[本文引用: 1]
[本文引用: 1]
[本文引用: 1]
DOIPMID [本文引用: 1]

In yellow soybean, seed coat pigmentation is inhibited by post-transcriptional gene silencing (PTGS) of chalcone synthase (CHS) genes. A CHS cluster named GmIRCHS (Glycine max inverted-repeat CHS pseudogene) is suggested to cause PTGS in yellow-hilum cultivars. Cold-induced seed coat discoloration (CD), a commercially serious deterioration of seed appearance, is caused by an inhibition of this PTGS upon exposure to low temperatures. In the highly CD-tolerant cultivar Toyoharuka, the GmIRCHS structure differs from that of other cultivars. The aim of this study was to determine whether the variation of GmIRCHS structure among cultivars is related to variations in CD tolerance. Using two sets of recombinant inbred lines between Toyoharuka and CD-susceptible cultivars, we compared the GmIRCHS genotype and CD tolerance phenotype during low temperature treatment. The GmIRCHS genotype was related to the phenotype of CD tolerance. A QTL analysis around GmIRCHS showed that GmIRCHS itself or a region located very close to it was responsible for CD tolerance. The variation in GmIRCHS can serve as a useful DNA marker for marker-assisted selection for breeding CD tolerance. In addition, QTL analysis of the whole genome revealed a minor QTL that also affected CD tolerance.
[本文引用: 1]
[本文引用: 1]
DOIURL [本文引用: 6]
DOIURL [本文引用: 1]
[本文引用: 1]
PMID [本文引用: 1]

The I locus controls inhibition of anthocyanin accumulation in the epidermal cells of the soybean seed coat and affects abundance of PRP1, a proline-rich cell wall protein in the seed coat. Saline-soluble PRP1 is abundant in the developing seed coats of cultivar Richland (homozygous I, yellow), while it is significantly decreased in the pigmented isogenic mutant T157 (homozygous i, imperfect black). In this report, we examined soluble PRP1 in several cultivars containing alleles of the I locus which affect spatial distribution of pigmentation in the seed coat. We also characterized PRP1 in isolines with allelic variants of several other loci involved in seed coat pigmentation, including T and Im. The T gene is pleiotropic and affects both pubesence color and seed coat pigmentation and structure. Soluble PRP1 was abundant in the developing seed coats of lines with yellow seed (I or ii alleles) regardless of pubescence color, just as in Richland. Likewise, soluble PRP1 was decreased in pigmented seed coats (ik or i alleles) with grey (t) pubescence, as in T157. However, the total seed coat proteins were not extractable from pigmented seed coats with tawny pubescence (i, T genotypes) because they have proanthocyanidins that exhibit tannin properties. The dominant Im allele inhibits seed coat mottling (irregular patches of pigmentation) that occurs if plants are infected with soybean mosaic virus. PRP1 was 35 kDa in mottled (im) isolines and 34 kDa in non-mottled (Im) isolines. PRP2, which is expressed later in seed coat development and in the hypocotyl hooks of soybean seedlings, was also smaller in Im isolines. In summary, some of the anthocyanin mutations affect the quantity of soluble PRP1 polypeptides. while others correlate with structural changes in developmentally regulated proline-rich proteins.
[本文引用: 1]
DOIURL [本文引用: 3]
DOIURL [本文引用: 3]
[本文引用: 2]
PMID [本文引用: 7]

A total of 391 simple sequence repeat (SSR) markers designed from genomic DNA libraries, 24 derived from existing GenBank genes or ESTs, and five derived from bacterial artificial chromosome (BAC) end sequences were developed. In contrast to SSRs derived from EST sequences, those derived from genomic libraries were a superior source of polymorphic markers, given that the mean number of tandem repeats in the former was significantly less than that of the latter ( P<0.01). The 420 newly developed SSRs were mapped in one or more of five soybean mapping populations: "Minsoy" x "Noir 1", "Minsoy" x "Archer", "Archer" x "Noir 1", "Clark" x "Harosoy", and A81-356022 x PI468916. The JoinMap software package was used to combine the five maps into an integrated genetic map spanning 2,523.6 cM of Kosambi map distance across 20 linkage groups that contained 1,849 markers, including 1,015 SSRs, 709 RFLPs, 73 RAPDs, 24 classical traits, six AFLPs, ten isozymes, and 12 others. The number of new SSR markers added to each linkage group ranged from 12 to 29. In the integrated map, the ratio of SSR marker number to linkage group map distance did not differ among 18 of the 20 linkage groups; however, the SSRs were not uniformly spaced over a linkage group, clusters of SSRs with very limited recombination were frequently present. These clusters of SSRs may be indicative of gene-rich regions of soybean, as has been suggested by a number of recent studies, indicating the significant association of genes and SSRs. Development of SSR markers from map-referenced BAC clones was a very effective means of targeting markers to marker-scarce positions in the genome.
DOIURL [本文引用: 4]
DOIURL [本文引用: 3]
DOIURL [本文引用: 7]
[本文引用: 5]
DOIURL [本文引用: 8]
DOIURL [本文引用: 5]
DOIURL [本文引用: 1]
DOIURL [本文引用: 1]
PMID [本文引用: 1]

Soybean plants (Glycine max [L.] Merr. cv Clark) carrying nuclear and cytoplasmic "stay-green" mutations, which affect senescence, were examined. Normally, the levels of chlorophyll (Chl) a and b decline during seedfill and the Chl a/b ratio decreases during late pod development in cv Clark. Plants homozygous for both the d(1) and d(2) recessive alleles, at two different nuclear loci, respectively, retained most (64%) of their Chl a and b and exhibited no change in their Chl a/b ratio. Combination of G (a dominant nuclear allele in a third locus causing only the seed coat to stay green during senescence) with d(1)d(2) further inhibited the loss of Chl in the leaf. Whereas the thylakoid proteins seem to be degraded in normal Clark leaves during late pod development, they were not substantially diminished in d(1)d(2) and Gd(1)d(2) leaves. In plants carrying a cytoplasmic mutation, cytG, Chl declined in parallel with normal cv Clark; however, the cytG leaves had a much higher level of Chl b, and somewhat more Chl a, remaining at abscission, enough to color the leaves green. In cytG, most thylakoid proteins were degraded, but the Chl a/b-binding polypeptides of the light-harvesting complex in photosystem II (LHCII), and their associated Chl a and b molecules, were not. Thus, the combination of d(1) and d(2) causes broad preservation of the thylakoid proteins, whereas cytG appears to selectively preserve LHCII. The cytG mutation may be useful in elucidating the sequence of events involved in the degradation of LHCII proteins and their associated pigments during senescence.
PMID [本文引用: 3]

The 'stay-green' mutation cytG in soybean (Glycine max) partially inhibits the degradation of the light-harvesting complex II (LHCII) and the associated chlorophyll during monocarpic senescence. cytG did not alter the breakdown of the cytochrome b6/f complex, thylakoid ATP synthase or components of Photosystem I. In contrast, cytG accelerated the loss of oxygen evolution activity and PSII reaction-centre proteins. These data suggest that LHCII and other thylakoid components are degraded by separate pathways. In leaves induced to senesce by darkness, cytG inhibited the breakdown of LHCII and chlorophyll, but it did not enhance the loss of PSII-core components, indicating that the accelerated degradation of PSII reaction centre proteins in cytG was light dependent. Illumination of mature and senescent leaves of wild-type soybean in the presence of an inhibitor (lincomycin) of chloroplast protein synthesis revealed that senescence per se did not affect the rate of photoinhibition in leaves. Likewise, mature leaves of the cytG mutant did not show more photoinhibition than wild-type leaves. However, in senescent cytG leaves, photoinhibition proceeded more rapidly than in the wild-type. We conclude that the cytG mutation enhances photoinhibition in senescing leaves, and photoinhibition causes the rapid loss of PSII reaction-centre proteins during senescence in cytG.
DOIPMID [本文引用: 4]

Chlorophyll degradation plays important roles in leaf senescence including regulation of degradation of chlorophyll-binding proteins. Although most genes encoding enzymes of the chlorophyll degradation pathway have been identified, the regulation of their activity has not been fully understood. Green cotyledon mutants in legume are stay-green mutants, in which chlorophyll degradation is impaired during leaf senescence and seed maturation. Among them, the soybean () green cotyledon gene is unique because it is maternally inherited. To isolate, we extensively sequenced the soybean chloroplast genome, and detected a 5-bp insertion causing a frame-shift in, which encodes one of the small subunits of photosystem II. Mutant tobacco plants () with a disrupted generated using a chloroplast transformation technique had green senescent leaves, confirming that encodes PsbM. The phenotype of was very similar to that of mutant of chlorophyll reductase catalyzing the first step of chlorophyll degradation. In fact, chlorophyll -degrading activity in dark-grown and -knockout seedlings was significantly lower than that of wild-type plants. Our results suggest that PsbM is a unique protein linking photosynthesis in presenescent leaves with chlorophyll degradation during leaf senescence and seed maturation. Additionally, we discuss the origin of, which may have been selected during domestication of soybean.© 2017 American Society of Plant Biologists. All Rights Reserved.
[本文引用: 2]
[本文引用: 2]
[本文引用: 2]
[本文引用: 2]
[本文引用: 1]
[本文引用: 1]
[本文引用: 1]
[本文引用: 1]
DOIURL [本文引用: 1]
[本文引用: 1]
[本文引用: 1]
DOIPMID [本文引用: 1]

Background: Although modern soybean cultivars feature yellow seed coats, with the only color variation found at the hila, the ancestral condition is black seed coats. Both seed coat and hila coloration are due to the presence of phenylpropanoid pathway derivatives, principally anthocyanins. The genetics of soybean seed coat and hilum coloration were first investigated during the resurgence of genetics during the 1920s, following the rediscovery of Mendel's work. Despite the inclusion of this phenotypic marker into the extensive genetic maps developed for soybean over the last twenty years, the genetic basis behind the phenomenon of brown seed coats (the R locus) has remained undetermined until now.;Results: In order to identify the gene responsible for the r gene effect (brown hilum or seed coat color), we utilized bulk segregant analysis and identified recombinant lines derived from a population segregating for two phenotypically distinct alleles of the R locus. Fine mapping was accelerated through use of a novel, bioinformatically determined set of Simple Sequence Repeat (SSR) markers which allowed us to delimit the genomic region containing the r gene to less than 200 kbp, despite the use of a mapping population of only 100 F6 lines. Candidate gene analysis identified a loss of function mutation affecting a seed coat-specific expressed R2R3 MYB transcription factor gene (Glyma09g36990) as a strong candidate for the brown hilum phenotype. We observed a near perfect correlation between the mRNA expression levels of the functional R gene candidate and an UDP-glucose: flavonoid 3-O-glucosyltransferase (UF3GT) gene, which is responsible for the final step in anthocyanin biosynthesis. In contrast, when a null allele of Glyma09g36990 is expressed no upregulation of the UF3GT gene was found.;Conclusions: We discovered an allelic series of four loss of function mutations affecting our R locus gene candidate. The presence of any one of these mutations was perfectly correlated with the brown seed coat/hilum phenotype in a broadly distributed survey of soybean cultivars, barring the presence of the epistatic dominant I allele or gray pubescence, both of which can mask the effect of the r allele, resulting in yellow or buff hila. These findings strongly suggest that loss of function for one particular seed coat-expressed R2R3 MYB gene is responsible for the brown seed coat/hilum phenotype in soybean.
[本文引用: 1]
DOIURL [本文引用: 1]
PMID [本文引用: 3]

Chalcone synthase (CHS; EC 2.3.1.74), the first committed enzyme of the multibranched pathway of flavonoid/isoflavonoid biosynthesis is encoded by a multigene family in soybean, (Glycine max L. Merrill). Our results suggest that this gene family comprises at least seven members, some of which are clustered. We have identified four chs clusters in the allo-tetraploid G. max genome and chs5, a newly characterized member of the chs gene family is present in two of them. We describe the complete nucleotide sequence of chs5, the identification of its immediate neighbors and the organization of the four hitherto identified chs clusters in the Gm genome.
PMID [本文引用: 2]

Seed coat color in soybean is controlled by the classically defined I ( Inhibitor) locus. The seeds of most commercial soybean varieties are yellow due to the presence of a dominant allele of the I locus ( I: yellow seed coat, or i(i) : pigmented hilum and yellow seed coat), which inhibits seed coat pigmentation. Analysis of spontaneous mutations from I (yellow seed coat) to i (pigmented seed coat) has shown that these mutations are correlated with the deletion of a duplicated chalcone synthase gene-1 ( CHS1) region. In the current study, we isolated the duplicated CHS1 region from a soybean cultivar with a I/I genotype (cv Miyagi shirome) and determined its structure. The results showed that the duplicated CHS1 contained intact regulatory and coding regions. We designated the duplicated CHS1 as ICHS1. In the hypocotyls of Miyagi shirome, the cDNA derived from ICHS1 mRNA was identified by reverse transcriptase-polymerase chain reaction (RT-PCR) analysis, whereas in the immature seed coats it was suggested that the amount of transcripts from ICHS1 and/or another type of CHS1 ( CHS1.1) was very low. Interestingly, in the Miyagi shirome genome with a I/I genotype, ICHS1 was closely linked to the truncated CHS3, and sequence comparison showed that this cluster probably arose from the CHS1-CHS3 cluster by a 1.8-kb deletion event.
[本文引用: 6]
DOIURL [本文引用: 3]
DOIURL [本文引用: 2]
[本文引用: 1]
PMID [本文引用: 4]

The seed of all wild Glycine accessions have black or brown pigments because of the homozygous recessive i allele in combination with alleles at the R and T loci. In contrast, nearly all commercial soybean (Glycine max) varieties are yellow due to the presence of a dominant allele of the I locus (either I or i) that inhibits pigmentation in the seed coats. Spontaneous mutations to the recessive i allele occur in these varieties and result in pigmented seed coats. We have isolated a clone for a soybean dihydroflavonol reductase (DFR) gene using polymerase chain reaction. We examined expression of DFR and two other genes of the flavonoid pathway during soybean seed coat development in a series of near-isogenic isolines that vary in pigmentation as specified by combinations of alleles of the I, R, and T loci. The expression of phenylalanine ammonia-lyase and DFR mRNAs was similar in all of the gene combinations at each stage of seed coat development. In contrast, chalcone synthase (CHS) mRNA was barely detectable at all stages of development in seed coats that carry the dominant I allele that results in yellow seed coats. CHS activity in yellow seed coats (I) was also 7- to 10-fold less than in the pigmented seed coats that have the homozygous recessive i allele. It appears that the dominant I allele results in reduction of CHS mRNA, leading to reduction of CHS activity as the basis for inhibition of anthocyanin and proanthocyanin synthesis in soybean seed coats. A further connection between CHS and the I locus is indicated by the occurrence of multiple restriction site polymorphisms in genomic DNA blots of the CHS gene family in near-isogenic lines containing alleles of the I locus.
DOIURL [本文引用: 2]
DOIURL [本文引用: 2]
[本文引用: 4]
DOIURL [本文引用: 3]
DOIURL [本文引用: 5]
DOIURL [本文引用: 5]
DOIURL [本文引用: 9]
DOIURL [本文引用: 5]
DOIPMID [本文引用: 4]

Domesticated species often exhibit convergent phenotypic evolution, termed the domestication syndrome, of which loss of seed dormancy is a component. To date, dormancy genes that contribute to parallel domestication across different families have not been reported. Here, we cloned the classical stay-green G gene from soybean and found that it controls seed dormancy and showed evidence of selection during soybean domestication. Moreover, orthologs in rice and tomato also showed evidence of selection during domestication. Analysis of transgenic plants confirmed that orthologs of G had conserved functions in controlling seed dormancy in soybean, rice, and Arabidopsis. Functional investigation demonstrated that G affected seed dormancy through interactions with NCED3 and PSY and in turn modulated abscisic acid synthesis. Therefore, we identified a gene responsible for seed dormancy that has been subject to parallel selection in multiple crop families. This may help facilitate the domestication of new crops.
DOIURL [本文引用: 1]
DOIPMID [本文引用: 5]

Background: The R locus controls the color of pigmented soybean (Glycine max) seeds. However information about its control over seed coat biochemistry and gene expressions remains limited. The seed coats of nearly-isogenic black (iRT) and brown (irT) soybean (Glycine max) were known to differ by the presence or absence of anthocyanins, respectively, with genes for only a single enzyme (anthocyanidin synthase) found to be differentially expressed between isolines. We recently identified and characterized a UDP-glycose:flavonoid-3-O-glycosyltransferase (UGT78K1) from the seed coat of black (iRT) soybean with the aim to engineer seed coat color by suppression of an anthocyanin-specific gene. However, it remained to be investigated whether UGT78K1 was overexpressed with anthocyanin biosynthesis in the black (iRT) seed coat compared to the nearly-isogenic brown (irT) tissue.;In this study, we performed a combined analysis of transcriptome and metabolite data to elucidate the control of the R locus over seed coat biochemistry and to identify pigment biosynthesis genes. Two differentially expressed late-stage anthocyanin biosynthesis isogenes were further characterized, as they may serve as useful targets for the manipulation of soybean grain color while minimizing the potential for unintended effects on the plant system.;Results: Metabolite composition differences were found to not be limited to anthocyanins, with specific proanthocyanidins, isoflavones, and phenylpropanoids present exclusively in the black (iRT) or the brown (irT) seed coat. A global analysis of gene expressions identified UGT78K1 and 19 other anthocyanin, (iso)flavonoid, and phenylpropanoid isogenes to be differentially expressed between isolines. A combined analysis of metabolite and gene expression data enabled the assignment of putative functions to biosynthesis and transport isogenes. The recombinant enzymes of two genes were validated to catalyze late-stage steps in anthocyanin biosynthesis in vitro and expression profiles of the corresponding genes were shown to parallel anthocyanin biosynthesis during black (iRT) seed coat development.;Conclusion: Metabolite composition and gene expression differences between black (iRT) and brown (irT) seed coats are far more extensive than previously thought. Putative anthocyanin, proanthocyanidin, (iso) flavonoid, and phenylpropanoid isogenes were differentially-expressed between black (iRT) and brown (irT) seed coats, and UGT78K2 and OMT5 were validated to code UDP-glycose: flavonoid-3-O-glycosyltransferase and anthocyanin 3'-O-methyltransferase proteins in vitro, respectively. Duplicate gene copies for several enzymes were overexpressed in the black (iRT) seed coat suggesting more than one isogene may have to be silenced to engineer seed coat color using RNA interference.
DOIURL [本文引用: 1]
DOI [本文引用: 1]

Active endogenous transposable elements, useful tools for gene isolation, have not been reported from any legume species. An active transposable element was suggested to reside in the W4 locus that governs flower color in soybean. Through biochemical and molecular analyses of several revertants of the w4-m allele, we have shown that the W4 locus encodes dihydroflavonol-4-reductase 2 (DFR2). w4-m has arisen through insertion of Tgm9, a 20,548-bp CACTA-like transposable element, into the second intron of DFR2. Tgm9 showed high nucleic acid sequence identity to Tgmt*. Its 5' and 3' terminal inverted repeats start with conserved CACTA sequence. The 39 subterminal region is highly repetitive. Tgm9 carries TNP1- and TNP2-like transposase genes that are expressed in the mutable line, T322 (w4-m). The element excises at a high frequency from both somatic and germinal tissues. Following excision, reinsertions of Tgm9 into the DFR2 promoter generated novel stable alleles, w4-dp (dilute purple flowers) and w4-p (pale flowers). We hypothesize that the element is fractured during transposition, and truncated versions of the element in new insertion sites cause stable mutations. The highly active endogenous transposon, Tgm9, should facilitate genomics studies specifically that relate to legume biology.
[本文引用: 1]
[本文引用: 1]
DOIURL [本文引用: 2]
PMID [本文引用: 1]

This review describes biochemistry, molecular biology and regulation of anthocyanin biosynthesis, with particular emphasis on mechanistic features and late steps of anthocyanin biosynthesis including glycosylation and vacuolar sequestration. The literature from 1997 to the beginning of 2002 is reviewed, and 163 references are cited.
DOIURL [本文引用: 1]
PMID [本文引用: 1]

Anthocyanidin synthase (ANS), an enzyme of the biosynthetic pathway to anthocyanin, has been postulated to catalyze the reaction(s) from the colorless leucoanthocyanidins to the colored anthocyanidins. Although cDNAs have been isolated that encode putative ANS, which exhibits significant similarities in amino acid sequence with members of a family of 2-oxoglutarate-dependent oxygenases, no biochemical evidence has been presented which identifies the actual reaction that is catalyzed by ANS. Here we show that anthocyanidins are formed in vitro through 2-oxoglutarate-dependent oxidation of leucoanthocyanidins catalyzed by the recombinant ANS and subsequent acid treatment. A cDNA encoding ANS was isolated from red and green formas of Perilla frutescens by differential display of mRNA. Recombinant ANS tagged with maltose-binding-protein (MBP) was purified, and the formation of anthocyanidins from leucoanthocyanidins was detected by the ANS-catalyzed reaction in the presence of ferrous ion, 2-oxoglutarate and ascorbate, being followed by acidification with HCI. Equimolar stoichiometry was confirmed for anthocyanidin formation and liberation of CO2 from 2-oxoglutarate. The presumptive two-copy gene of ANS was expressed in leaves and stems of the red forma of P. frutescens but not in the green forma plant. This corresponds to the accumulation pattern of anthocyanin. The mechanism of the reaction catalyzed by ANS is discussed in relation to the molecular evolution of a family of 2-oxoglutarate-dependent oxygenases.
DOIURL [本文引用: 1]
URL [本文引用: 1]
DOIURL [本文引用: 1]
[本文引用: 1]
[本文引用: 1]
[本文引用: 1]
[本文引用: 1]
[本文引用: 1]
[本文引用: 1]
[本文引用: 1]
[本文引用: 1]
[本文引用: 1]
[本文引用: 1]
[本文引用: 2]
[本文引用: 2]
[本文引用: 2]
[本文引用: 2]
[本文引用: 2]
[本文引用: 2]
[本文引用: 2]
[本文引用: 2]
[本文引用: 2]
[本文引用: 2]
[本文引用: 1]
[本文引用: 1]
[本文引用: 1]
[本文引用: 1]
DOIURL [本文引用: 1]