
Genome dominance and the breeding significance in Triticeae
LIU Deng-Cai
通讯作者:
收稿日期:2020-03-18接受日期:2020-07-2网络出版日期:2020-07-06
基金资助: |
Received:2020-03-18Accepted:2020-07-2Online:2020-07-06
Fund supported: |

摘要
关键词:
Abstract
Keywords:
PDF (4156KB)元数据多维度评价相关文章导出EndNote|Ris|Bibtex收藏本文
本文引用格式
刘登才, 张连全, 郝明, 黄林, 甯顺腙, 袁中伟, 姜博, 颜泽洪, 伍碧华, 郑有良. 小麦族的基因组显性及其育种学意义[J]. 作物学报, 2020, 46(10): 1465-1473. doi:10.3724/SP.J.1006.2020.01022
LIU Deng-Cai, ZHANG Lian-Quan, HAO Ming, HUANG Lin, NING Shun-Zong, YUAN Zhong-Wei, JIANG Bo, YAN Ze-Hong, WU Bi-Hua, ZHENG You-Liang.
小麦族(Triticeae)是禾本科早熟禾亚科的一个大族。基因组组成多样是小麦族植物的重要特征。小麦、大麦、黑麦、小黑麦等小麦族作物属于一年生。小麦族多数属种属于多年生, 多年生物种具有重要的生态经济价值, 是高寒草原、草甸、荒漠等植被区的重要组成部分, 也是重要牧草资源。近年来, 多年生物种中间偃麦草[Thinopyrum intermedium (Host) Barkworth & D. R. Dewey]已被驯化为具有生态和经济价值、小范围种植的多年生新作物, 商品名为Kernza[1]。小麦族基因组源于不同的二倍体属, 例如A (Triticum)、B/C/D/G/M/N/S/U (Aegilops)、E/J (Lophopyrum)、F (Eremopyrum)、H/I (Hordeum)、K (Crithopsis)、L (Festucopsis)、Ns (Psathyrostachys)、O (Henrardia)、P (Agropyron)、Q (Heteranthelium)、R (Secale)、St (Pseudoroegneria)、T (Amblyopyrum)、Ta (Taeniatherum)、V (Pseudosecale)、W (Australop- yrum)、Xp (Peridictyon)等[2,3,4,5]。小麦族的大多数物种是异源多倍体, 表明小麦族异源多倍体物种具有进化适应优势。
普通小麦(T. aestivum L., AABBDD)是世界三大粮食作物之一。普通小麦的起源涉及3个二倍体物种。其中, A基因组供体来自乌拉尔图小麦(T. urartu, AuAu)[6], D基因组供体来自节节麦(Aegilops tauschii, DD)[7]。但是, B基因组供体物种来源还有争论, 通常认为来自拟斯卑尔塔山羊草(Ae. speltoides, SsSs)或与之紧密相关的物种[8,9]。普通小麦的起源经历了2次异源多倍化过程。第1次, 形成具有AABB基因组的野生四倍体小麦(T. turgidum ssp. dicoccoides)。第2次, 以栽培型四倍体小麦与节节麦杂交, 形成异源六倍体小麦[7,10]。普通小麦的起源进化过程存在多倍化和驯化瓶颈[11]。通常认为, 现有普通小麦由少数的原始六倍体个体进化而来, 原始普通小麦的遗传多样性低。同时, 普通小麦的起源历史不长, 大约发生在1万年前[12], 自然变异的累积时间不长。尽管如此, 目前普通小麦的适应性广、分布范围广。因此, 探讨普通小麦进化成功的原因及其育种学价值是一个重要课题。
1 基因组显性现象
孟德尔提出了显性概念, 建立了表现型与基因型的联系。当2个具有相对性状的纯合亲本进行杂交, 杂合体中表现出来的性状叫显性性状, 未表现出来的性状叫隐性性状。对应显性性状的基因为显性基因, 对应隐性性状的为隐性基因。有的异源多倍体物种或种间杂种后代, 形态上更像其中的一个供体物种, 而与另一个供体物种存在明显不同。例如, 在株型、穗型等方面, 普通小麦与它的AB基因组供体物种(四倍体小麦)相像, 而与它的D基因组供体物种(节节麦)差异很大(图1)。仅根据形态学, 有时很难将普通小麦与栽培型的四倍体小麦区分开。异源多倍体的不同基因组对表型性状的非对称性影响现象[13], 被称为基因组显性[14]。图1

图1四倍体小麦、节节麦和普通小麦
普通小麦的株型穗型与它的AB基因组供体物种四倍体小麦相像, 而与它的D基因组供体物种节节麦差异很大。
Fig. 1Triticum turgidum, Aegilops tauschii, and common wheat
The plant and spike morphologies of common wheat are like those from T. turgidum, distinct from Ae. tauschii.
几百年前的形态学比较研究, 暗示了小麦属的A基因组显性现象。早期的研究聚焦于小麦属和山羊草属。瑞典博物学家林奈以Triticum aestivum L.为指定模式种建立了小麦属(Triticum L.), 以Aegilops ovata L.为指定模式种建立了山羊草属(Aegilops L.)。在1753年, 林奈出版的《植物种志》中, 他将二倍体小麦、四倍体小麦、普通小麦放在小麦属, 而其他物种放在山羊草属。后来的细胞学研究发现, 这些小麦属物种均具有A基因组, 而其他物种不具有A基因组。尽管以后的分类学家对小麦-山羊草复合群的物种归属有多样化的处理方式, 但是通常的意见仍然是将具有A基因组的所有物种放在小麦属, 而其他物种放在山羊草属[15]。传统分类主要依靠形态学差异, 这样的分类处理表明, 具有A基因组的物种具有类似的形态分类特征, 即A基因组显性。
基因组组成和表型的比较研究发现, 基因组显性是山羊草属物种的进化特征。具有CD、DN、DM、DDM、DMS、DMU等基因组组成的山羊草物种(图2), 它们具有共同的D基因组, 它们的穗型等形态特征更类似于二倍体物种节节麦(D), 而与其他二倍体供体物种差异很大。类似地, 具有MU、SU、UC/CU、UM、UMN等不同基因组组成的山羊草物种, 它们的形态特征更类似于U基因组的供体物种小伞山羊草(Ae. umbellulata)。根据基因组和形态分类特征的关系, 一些****将小麦-山羊草复合群分为A、D和U种簇(图2), 并提出了“轴心-分化基因组进化”(pivotal-differential genome evolution)假说[16,17,18]。在形态学方面, 异源多倍体物种的穗型、种子形态等特征更像它们的轴心基因组供体物种, 即轴心基因组表现显性。该假说认为, 异源多倍体物种分享一个比较保守的轴心基因组(A、D、U中的一个)和分化较大的其他1~2基因组。在染色体或基因组水平上的研究结果, 发现了异源多倍体物种不同基因组进化不一致的现象[19,20,21,22,23,24,25,26,27]。但是, 从新合成多倍体来看, 基因组对表型非对称贡献现象从合成新多倍体就表现出来了, 例如在新合成的具有AABBUU基因组的六倍体[28], 其穗型更像四倍体小麦亲本, 而与山羊草亲本小伞山羊草(Ae. umbellulata)差异大(图3)。因此, 亲本物种的基因组显性对表型的非对称贡献现象有重要作用。
图2
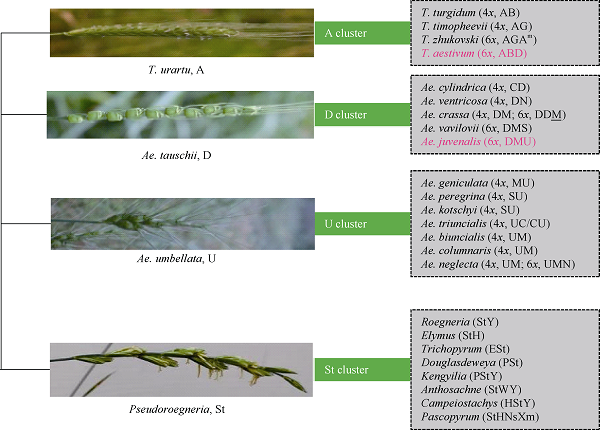
图2以A、D、U、St基因组形成的异源多倍体物种簇
Fig. 2Allopolyploid species clusters with A, D, U, or St as the pivotal genome
图3

图3人工合成新多倍体及其亲本物种的穗型
a: 新合成的六倍体(AABBUU、AABBMM、AABBCC)及其亲本四倍体小麦(AABB)、小伞山羊草(Ae. umbellulata, UU)、顶芒山羊草(Ae. comosa, MM)、尾状山羊草(Ae. caudata, CC)。b: 新合成的六倍体(AABBAuAu、AABBAmAm、AAGGDD)、八倍体(AABBDDAmAm、AABBDDDD)及其二倍体乌拉尔图小麦(AuAu)、栽培一粒小麦(AmAm)、节节麦(DD)、四倍体小麦(AABB)、提莫菲维小麦(AAGG)。
Fig. 3Spikes of nascent allopolyplods and the parent species
a: Nascent hexaploids (AABBUU, AABBMM, AABBCC) and T. trugidum (AABB), Ae. umbellulata (UU), Ae. comosa (MM), Ae. caudata (CC). b: Nascent hexaploids (AABBAuAu, AABBAmAm, AAGGDD), octaploids (AABBDDAmAm, AABBDDDD) and T. urartu (AuAu), T. monococcum (AmAm), Ae. tauschii (DD), T. trugidum (AABB), and T. timopheevii (AAGG).
小麦族多年生属种也存在基因组显性现象。在小麦族, St基因组参与了拟鹅观草属、鹅观草属、披碱草属、毛麦属、杜威草属、仲彬草属、花鳞草属、曲穗草属、牧场麦属等属的起源进化(图2), 涉及大量多年生物种[29,30,31,32]。传统的分类学与系统学主要以形态特征的鉴定为主, 含St基因组的物种处理, 一直比较混乱。其中, 一个主要原因是St基因组的显性作用。颜济和杨俊良[31]在《小麦族生物系统学》第四卷的序言部分认为, “由于St染色体组具有一系列的强显性基因, 因此, 使含St染色体组的不同的异源多倍体组合的属, 如披碱草属(HSt)、鹅观草属(StY)、弯穗草属(HStY)的形态性状趋同, 因此单纯从外表形态不可能认识其隐伏的差异, 只有进一步进行遗传学的检测才能看到它们在亲缘系统上的真正区别。找出区别以后, 再在众多的性状中筛选出区别于核基因组的特有关键性状(表型), 也就是反映染色体组特有的形态显性性状。用这些关键性状我们就可以比较准确地根据形态性状来区别一个分类群的归属。我们还要进一步指出, 由于显性性状的掩盖, 有那么一些在系统演化上完全不同的种, 用形态学来鉴定是无法区分的”。因此, 基因组显性现象也是长期困扰一些小麦族多年生属种分类的一个难题。
2 基因组显性的生物学意义及遗传基础
异源多倍体物种广泛存在, 表明异源多倍体具有进化适应优势。基因组显性可能是异源多倍体物种产生优势的原因之一。一个物种的基因组优先对一类性状表现显性, 而另一物种的基因组优先对另一些性状表现显性, 组合在一起可产生集合优势, 导致异源多倍体比亲本物种更有优势。以小麦为例, 普通小麦保持了四倍体小麦的高产潜力[33]。D基因组的加入, 使得普通小麦对春花和光周期要求的适应范围更广, 提高了对盐、低pH、铝、冻害的耐性, 增加了对一些病虫害的抗性, 并且可加工的食品类型更为丰富多样[33,34]。因此, 普通小麦既高产、又广适优质。另一个例子, 来自世界上第一个人造新作物小黑麦。原始的四倍体(AARR)、六倍体(AABBRR)、八倍体小黑麦(AABBDDRR)分别用二倍体、四倍体、六倍体小麦与黑麦(RR)杂交创制。小黑麦将小麦的高产潜力和黑麦的生长优势、不良环境的适应能力聚集在一起。在贫瘠土壤和气候环境不利于小麦生长的地方, 小黑麦表现较高的产量潜力[35,36,37]。小黑麦的生物产量和籽粒产量高, 除了用作食品加工和饲草应用, 也用作能源作物[38,39,40]。在遗传上, 四倍体小麦的A基因组优先控制形态发育, 而B基因组有更多的基因控制适应性状[13,17]。在普通小麦定位发掘的株型、穗型等产量相关QTL中, 来自A基因组的位点更多[41]。最近的系统分析表明, 发掘出的控制普通小麦产量三要素(穗数、穗粒数、千粒重)的QTL位点优先分布在A基因组[42]。在利用四倍体小麦与节节麦杂交人工创制的新六倍体小麦, 也有类似的现象, 定位的多数产量相关位点来自A基因组[43], 但是适应性状在B和D基因组更多[44]。在转录组水平, 四倍体亲本基因组的表达显性基因主要贡献于人工合成新小麦的发育, 二倍体亲本基因组表达显性基因主要贡献于新小麦的适应性[45,46]。近年来, 对其他物种进行的研究表明, 基因组表达显性是异源多倍体物种比较普遍的遗传特征[47,48]。不同的假说用来解释基因组表达显性现象。其中, 转座子的非对称分布是一个比较流行的假说, 即不同基因组的转座子分布数量和位置存在明显差异, 从而影响了转座子附近的基因表达[49,50]。
在小麦族物种, 轴心(显性)基因组参与形成了大量异源多倍体物种。但是, 基因组显性与基因组的组成有关。例如, 小麦-山羊草复合群A、D和U基因组是小麦属和山羊草属物种的3个轴心(显性)基因组(图2)。普通小麦具有其中的2个(A、D)。但是, 普通小麦形态学上更像具有A基因组的二倍体物种, 因此放在A簇。类似地, 山羊草物种Ae. juvenalis (基因组DDMMUU)具有D、U两个轴心基因组。但是, 该物种更像它的D基因组二倍体物种节节麦, 而与具有U染色体的二倍体山羊草物种Ae. umbellulata差异大, 因此Ae. juvenalis属于D簇物种[16-18,51]。目前还不清楚产生这种特殊进化现象的遗传机制[51]。深入研究轴心基因组的形成原因及其与基因组组成的关系, 对于进一步阐释基因组显性现象的遗传基础具有重要意义。这样的研究结果, 可为作物育种设计及新作物创造提供指导依据。
3 基因组显性与新多倍体作物研制
小麦族属种具有丰富的基因组资源有待开发利用。有的小麦族植物具有特殊或极端的环境适应性, 如高海拔(接近5000 m)、戈壁滩、沙丘、高盐环境。利用它们研发粮食、饲草、生态草等用途的新作物, 可以作为未来应对全球气候变化、生态环境保护等重要问题的技术策略。小麦族可组配的基因组组成类型很多。以20个具有不同基因组的二倍体杂交为例, 在不考虑正反交(细胞质不同)的情况下, 就可以形成190种四倍体基因组组合。如果考虑细胞质和其他倍性物种的杂交, 基因组组合的数量将是巨量的。利用具有不同基因组的物种杂交, 已人工创制了大量的新型多倍体植物(双二倍体)。其中, 小黑麦将小麦和黑麦基因组的遗传优势聚集在一起, 已被改良成一个多用途的新多倍体作物。研制新型多倍体作物仍是未来育种的一个重要方向。新型多倍体作物育种的优势, 在于改良复杂性状。复杂性状的遗传基础复杂, 受多个基因位点控制。最近, 全基因组模型(omnigenic model)用于解释一些复杂性状的遗传调控[52]。多倍体物种如小麦, 由于存在大量重复基因, 基因组缓冲性、可塑性高, 单个基因拷贝的变化经常仅有较小的遗传效应[33, 53]。通过单个基因或单个染色体片段的导入, 对多倍体物种复杂性状的改良效果有限。但是, 多个基因的同时导入, 可能产生明显效果。由于长期适应进化, 一个物种的基因组不同位置聚集了适应特殊环境的系列遗传变异。新型多倍体作物育种涉及整个基因组导入, 可能在改良复杂性状方面具有优势。例如, 小黑麦通过黑麦R基因组的导入, 改良了对贫瘠土壤和不利气候环境的适应能力[35,36,37]。
基因组显性影响多倍体新作物的开发潜力。多倍体新作物研制涉及两个主要阶段。一是通过远缘杂交, 创制多倍体新植物, 该工作比较容易。二是对新多倍体植物进行驯化改良。虽然新发展的基因编辑等技术能显著提高驯化效率, 但是仍涉及大量繁琐的遗传改良工作。因此, 预测新多倍体的改良潜力是必要的基础工作。基因组显性强烈影响新多倍体的表型, 因此可以用作优良基因组组合预测的参考依据。以四倍体小麦与山羊草物种创制的3个新六倍体为例[28,54], 新合成的具有AABBMM和AABBUU基因组的六倍体, 穗型更像四倍体小麦, 而与顶芒山羊草(Ae. comosa, M)、小伞山羊草(Ae. umbellulata)差异大(图3)。但是, 具有AABBCC基因组的六倍体, 它的穗型更像它的山羊草亲本尾状山羊草(Ae. caudata, CC), 表明C基因组表现显性。与具有AABBCC基因组的六倍体相比, 具有AABBMM基因组的新六倍体具有四倍体亲本的多小花特征, 驯化成高产粮食作物的可能性更大。
小麦栽培种包括二倍体、四倍体和六倍体。其中, 栽培一粒小麦(T. monococcum, AmAm)是人类在1万年前最早驯化的作物之一, 四倍体具有AABB基因组或AAGG基因组(T. timopheevii), 六倍体为普通小麦(AABBDD)和茹可夫斯基小麦(T. zhukovski, AAAmAmGG)。利用四倍体小麦和乌拉尔图小麦(AuAu)、栽培一粒小麦(AmAm)、节节麦(DD)创制的六倍体新类型(AABBAuAu、AABBAmAm、AAGGDD), 均具有利于高产的穗型特征[55,56]。国际玉米小麦改良中心利用普通小麦与栽培一粒小麦、节节麦创制的八倍体新类型(AABBDDAmAm、AABBDDDD)也具有高产的穗型特征(图3)。其中, 具有AAGGDD、AABBAmAm、AABBDDAmAm的多倍体, 具有比较正常的减数分裂行为, 可以尝试对其驯化改良。考虑到A(Am)基因组对穗型发育的显性影响以及基因表达剂量效应[57], 值得优先对同时具有A与Am基因组的小麦新多倍体进行驯化改良尝试, 以探索它们的高产潜力。
4 基因组显性与小麦-外源易位设计
拓宽遗传多样性是当前育种的一项重要工作。小麦族属种繁多、生境多样, 为小麦育种提供了丰富的外源基因源。模拟普通小麦起源, 通过四倍体小麦与节节麦杂种的染色体加倍, 有目的地人工创制六倍体小麦, 并以此为桥梁, 通过同源重组, 可快速将四倍体小麦和节节麦的遗传变异导入普通小麦[43,58-59]。但是, 绝大多数外源物种与小麦没有同源染色体, 无法实现同源重组, 通常需要借助染色体工程技术, 以小麦-外源染色体易位的方式, 实现外源基因转移[60]。黑麦、偃麦草、簇毛麦、冰草等小麦族属种已先后用于小麦育种, 拓展了我国小麦品种的遗传基础[61,62,63,64]。如果易位发生在共线性程度高的小麦-外源染色体区段间, 则遗传补偿性好, 这样的易位系称为平衡易位[65]。平衡易位是育种利用的主要方式, 例如育种广泛利用的1BL·1RS和6AL·6VS是臂间平衡易位[66,67]。基因组学的快速发展[68,69,70,71], 加速了分子标记和原位杂交探针开发, 提升了小麦-外源易位系的定向创制效率。目前, 利用ph1b突变体作为染色体操作工具, 创制平衡的小片段易位系已变得比较容易[72,73,74]。小麦-外源易位系的创制过程中, 基因组显性应作为一个参考因素。目前的易位系主要用于将外源物种的单基因控制性状转移到小麦, 绝大多数工作涉及外源抗病基因[75,76]。普通小麦的染色体组包含7个部分同源群, 每个同源群具有3对在遗传上类似的部分同源染色体。但是, 在减数分裂中期I, 只有同源染色体发生配对[77], 表明部分同源染色体间存在明显的遗传分化。在普通小麦易位系培育中, 如果不考虑染色体间的结构变化、育性和减数分裂等关键基因的影响[78,79], 可以将外源染色体片段易位到3个部分同源染色体的相应位置, 形成3种类型的平衡易位。以黑麦染色体长臂7RL的抗病基因导入为例, 可以形成3种平衡易位类型(7AS·7RL、7BS·7RL和7DS·7RL)。在这些易位系中, 7RL分别替换了7AL、7BL、7DL, 影响了小麦基因组的完整。因此, 在实际操作中, 需要考虑创制哪种易位类型更有利于育种的问题。由于A基因组显性, 小麦的穗型、种子形态等有利于高产的表型性状优先受A基因组上的基因控制[17, 34]。如果保持A基因组的完整性, 更有利于高产, 则应优先考虑创制7BS·7RL、7DS·7RL易位。这样的设想需要利用在同一遗传背景、涉及3种部分同源染色体臂的成套易位材料来验证。但是, 目前还缺乏这样的材料。这样的材料可用于研究外源导入对基因组A、B、D的影响, 也可用于研究不同亚基因组与外源染色体区段的互作影响。
致谢
感谢国际玉米小麦改良中心(CIMMYT)的Masahiro Kishii博士提供具有AABBDDAmAm、AABBDDDD基因组的八倍体小麦材料, 四川农业大学张海琴教授提供拟鹅观草的穗型图片。参考文献 原文顺序
文献年度倒序
文中引用次数倒序
被引期刊影响因子
[本文引用: 1]
[本文引用: 1]
In: Gustafson J P, ed. Gene Manipulation in Plant Improvement.
[本文引用: 1]
[本文引用: 1]
DOI:10.1270/jsbbs.59.513URL [本文引用: 1]
DOI:10.1139/g93-004URLPMID:18469969 [本文引用: 1]

Cytogenetic work has shown that the tetraploid wheats, Triticum turgidum and T. timopheevii, and the hexaploid wheat T. aestivum have one pair of A genomes, whereas hexaploid T. zhukovskyi has two. Variation in 16 repeated nucleotide sequences was used to identify sources of the A genomes. The A genomes of T. turgidum, T. timopheevii, and T. aestivum were shown to be contributed by T. urartu. Little divergence in the repeated nucleotide sequences was detected in the A genomes of these species from the genome of T. urartu. In T. zhukovskyi one A genome was contributed by T. urartu and the other was contributed by T. monococcum. It is concluded that T. zhukovskyi originated from hybridization of T. timopheevii with T. monococcum. The repeated nucleotide sequence profiles in the A genomes of T. zhukovskyi showed reduced correspondence with those in the genomes of both ancestral species, T. urartu and T. monococcum. This differentiation is attributed to heterogenetic chromosome pairing and segregation among chromosomes of the two A genomes in T. zhukovskyi.
[本文引用: 2]
DOI:10.1016/j.ympev.2006.01.023URLPMID:16504543 [本文引用: 1]

Common wheat (Triticum aestivum) has for decades been a textbook example of the evolution of a major crop species by allopolyploidization. Using a sophisticated extension of the PCR technique, we have successfully isolated two single-copy nuclear genes, DMC1 and EF-G, from each of the three genomes found in hexaploid wheat (BA(u)D) and from the two genomes of the tetraploid progenitor Triticum turgidum (BA(u)). By subjecting these sequences to phylogenetic analysis together with sequences from representatives of all the diploid Triticeae genera we are able for the first time to provide simultaneous and strongly supported evidence for the D genome being derived from Aegilops tauschii, the A(u) genome being derived from Triticum urartu, and the hitherto enigmatic B genome being derived from Aegilops speltoides. Previous problems of identifying the B genome donor may be associated with a higher diversification rate of the B genome compared to the A(u) genome in the polyploid wheats. The phylogenetic hypothesis further suggests that neither Triticum, Aegilops, nor Triticum plus Aegilops are monophyletic.
DOI:10.1093/molbev/msl151URLPMID:17053048 [本文引用: 1]

The origin of modern wheats involved alloploidization among related genomes. To determine if Aegilops speltoides was the donor of the B and G genomes in AABB and AAGG tetraploids, we used a 3-tiered approach. Using 70 amplified fragment length polymorphism (AFLP) loci, we sampled molecular diversity among 480 wheat lines from their natural habitats encompassing all S genome Aegilops, the putative progenitors of wheat B and G genomes. Fifty-nine Aegilops representatives for S genome diversity were compared at 375 AFLP loci with diploid, tetraploid, and 11 nulli-tetrasomic Triticum aestivum wheat lines. B genome-specific markers allowed pinning the origin of the B genome to S chromosomes of A. speltoides, while excluding other lineages. The outbreeding nature of A. speltoides influences its molecular diversity and bears upon inferences of B and G genome origins. Haplotypes at nuclear and chloroplast loci ACC1, G6PDH, GPT, PGK1, Q, VRN1, and ndhF for approximately 70 Aegilops and Triticum lines (0.73 Mb sequenced) reveal both B and G genomes of polyploid wheats as unique samples of A. speltoides haplotype diversity. These have been sequestered by the AABB Triticum dicoccoides and AAGG Triticum araraticum lineages during their independent origins.
[本文引用: 1]
In: Tsunewaki K, ed. Frontiers of Wheat Bioscience, the 100th Memorial Issue of Wheat Information Service.
[本文引用: 1]
DOI:10.1073/pnas.072223799URLPMID:12060759 [本文引用: 1]

The classic wheat evolutionary history is one of adaptive radiation of the diploid Triticum/Aegilops species (A, S, D), genome convergence and divergence of the tetraploid (Triticum turgidum AABB, and Triticum timopheevii AAGG) and hexaploid (Triticum aestivum, AABBDD) species. We analyzed Acc-1 (plastid acetyl-CoA carboxylase) and Pgk-1 (plastid 3-phosphoglycerate kinase) genes to determine phylogenetic relationships among Triticum and Aegilops species of the wheat lineage and to establish the timeline of wheat evolution based on gene sequence comparisons. Triticum urartu was confirmed as the A genome donor of tetraploid and hexaploid wheat. The A genome of polyploid wheat diverged from T. urartu less than half a million years ago (MYA), indicating a relatively recent origin of polyploid wheat. The D genome sequences of T. aestivum and Aegilops tauschii are identical, confirming that T. aestivum arose from hybridization of T. turgidum and Ae. tauschii only 8,000 years ago. The diploid Triticum and Aegilops progenitors of the A, B, D, G, and S genomes all radiated 2.5-4.5 MYA. Our data suggest that the Acc-1 and Pgk-1 loci have different histories in different lineages, indicating genome mosaicity and significant intraspecific differentiation. Some loci of the S genome of Aegilops speltoides and the G genome of T. timophevii are closely related, suggesting the same origin of some parts of their genomes. None of the Aegilops genomes analyzed is a close relative of the B genome, so the diploid progenitor of the B genome remains unknown.
DOI:10.1093/jxb/ers192URL [本文引用: 2]

The evolvement of duplicated gene loci in allopolyploid plants has become the subject of intensive studies. Most duplicated genes remain active in neoallopolyploids contributing either to a favourable effect of an extra gene dosage or to the build-up of positive inter-genomic interactions when genes or regulation factors on homoeologous chromosomes are divergent. However, in a small number of loci (about 10%), genes of only one genome are active, while the homoeoalleles on the other genome(s) are either eliminated or partially or completely suppressed by genetic or epigenetic means. For several traits, the retention of controlling genes is not random, favouring one genome over the other(s). Such genomic asymmetry is manifested in allopolyploid wheat by the control of various morphological and agronomical traits, in the production of rRNA and storage proteins, and in interaction with pathogens. It is suggested that the process of cytological diploidization leading to exclusive intra-genomic meiotic pairing and, consequently, to complete avoidance of inter-genomic recombination, has two contrasting effects. Firstly, it provides a means for the fixation of positive heterotic inter-genomic interactions and also maintains genomic asymmetry resulting from loss or silencing of genes. The possible mechanisms and evolutionary advantages of genomic asymmetry are discussed.
DOI:10.1105/tpc.114.127183URLPMID:24838977 [本文引用: 1]
[本文引用: 1]
[本文引用: 1]
DOI:10.1508/cytologia.19.336URL [本文引用: 2]
DOI:10.1111/evo.1962.16.issue-1URL [本文引用: 3]
DOI:10.1073/pnas.85.23.9106URLPMID:16594002 [本文引用: 2]

The numerical analysis of meiosis in hybrids between a wild allotetraploid and an autotetraploid of one of its putative diploid progenitors allows the identification of which genomes are pairing and also the verification of pivotal-differential evolution. This type of analysis should be applicable to all genera in which allopolyploid series exist.
DOI:10.1111/evo.1965.19.issue-4URL [本文引用: 1]
DOI:10.1002/j.1537-2197.1996.tb12813.xURL [本文引用: 1]
DOI:10.1111/nph.12731URL [本文引用: 1]

Transposable elements (TEs) are expectedly central to genome evolution. To assess the impact of TEs in driving genome turnover, we used allopolyploid genomes, showing considerable deviation from the predicted additivity of their diploid progenitors and thus having undergone major restructuring. Genome survey sequencing was used to select 17 putatively active families of long terminal repeat retrotransposons. Genome-wide TE insertions were genotyped with sequence-specific amplified polymorphism (SSAP) in diploid progenitors and their derived polyploids, and compared with changes in random sequences to assess restructuring of four independent Aegilops allotetraploid genomes. Generally, TEs with different evolutionary trajectories from those of random sequences were identified. Thus, TEs presented family-specific and species-specific dynamics following polyploidy, as illustrated by Sabine showing proliferation in particular polyploids, but massive elimination in others. Contrasting with that, only a few families (BARE1 and Romani) showed proliferation in all polyploids. Overall, TE divergence between progenitors was strongly correlated with the degree of restructuring in polyploid TE fractions. TE families present evolutionary trajectories that are decoupled from genome-wide changes after allopolyploidy and have a pervasive impact on their restructuring.]]>
DOI:10.1104/pp.112.205161URL [本文引用: 1]

Cycles of whole-genome duplication (WGD) and diploidization are hallmarks of eukaryotic genome evolution and speciation. Polyploid wheat (Triticum aestivum) has had a massive increase in genome size largely due to recent WGDs. How these processes may impact the dynamics of gene evolution was studied by comparing the patterns of gene structure changes, alternative splicing (AS), and codon substitution rates among wheat and model grass genomes. In orthologous gene sets, significantly more acquired and lost exonic sequences were detected in wheat than in model grasses. In wheat, 35% of these gene structure rearrangements resulted in frame-shift mutations and premature termination codons. An increased codon mutation rate in the wheat lineage compared with Brachypodium distachyon was found for 17% of orthologs. The discovery of premature termination codons in 38% of expressed genes was consistent with ongoing pseudogenization of the wheat genome. The rates of AS within the individual wheat subgenomes (21%-25%) were similar to diploid plants. However, we uncovered a high level of AS pattern divergence between the duplicated homeologous copies of genes. Our results are consistent with the accelerated accumulation of AS isoforms, nonsynonymous mutations, and gene structure rearrangements in the wheat lineage, likely due to genetic redundancy created by WGDs. Whereas these processes mostly contribute to the degeneration of a duplicated genome and its diploidization, they have the potential to facilitate the origin of new functional variations, which, upon selection in the evolutionary lineage, may play an important role in the origin of novel traits.
DOI:10.1111/tpj.12366URL [本文引用: 1]

Bread wheat derives from a grass ancestor structured in seven protochromosomes followed by a paleotetraploidization to reach a 12 chromosomes intermediate and a neohexaploidization (involving subgenomes A, B and D) event that finally shaped the 21 modern chromosomes. Insights into wheat syntenome in sequencing conserved orthologous set (COS) genes unravelled differences in genomic structure (such as gene conservation and diversity) and genetical landscape (such as recombination pattern) between ancestral as well as recent duplicated blocks. Contrasted evolutionary plasticity is observed where the B subgenome appears more sensitive (i.e. plastic) in contrast to A as dominant (i.e. stable) in response to the neotetraploidization and D subgenome as supra-dominant (i.e. pivotal) in response to the neohexaploidization event. Finally, the wheat syntenome, delivered through a public web interface PlantSyntenyViewer at http://urgi.versailles.inra.fr/synteny-wheat, can be considered as a guide for accelerated dissection of major agronomical traits in wheat.
DOI:10.1111/j.1095-8312.2004.00349.xURL [本文引用: 1]
DOI:10.1111/j.1469-8137.2005.01433.xURLPMID:15998412 [本文引用: 1]

Hybridization and polyploidy can induce rapid genomic changes, including the gain or loss of DNA, but the magnitude and timing of such changes are not well understood. The homoploid hybrid system in Helianthus (three hybrid-derived species and their two parents) provides an opportunity to examine the link between hybridization and genome size changes in a replicated fashion. Flow cytometry was used to estimate the nuclear DNA content in multiple populations of three homoploid hybrid Helianthus species (Helianthus anomalus, Helianthus deserticola, and Helianthus paradoxus), the parental species (Helianthus annuus and Helianthus petiolaris), synthetic hybrids, and natural hybrid-zone populations. Results confirm that hybrid-derived species have 50% more nuclear DNA than the parental species. Despite multiple origins, hybrid species were largely consistent in their DNA content across populations, although H. deserticola showed significant interpopulation differences. First- and sixth-generation synthetic hybrids and hybrid-zone plants did not show an increase from parental DNA content. First-generation hybrids differed in DNA content according to the maternal parent. In summary, hybridization by itself does not lead to increased nuclear DNA content in Helianthus, and the evolutionary forces responsible for the repeated increases in DNA content seen in the hybrid-derived species remain mysterious.
DOI:10.1105/tpc.114.129841URL [本文引用: 1]

rRNA genes consist of long tandem repeats clustered on chromosomes, and their products are important functional components of the ribosome. In common wheat (Triticum aestivum), rDNA loci from the A and D genomes were largely lost during the evolutionary process. This biased DNA elimination may be related to asymmetric transcription and epigenetic modifications caused by the polyploid formation. Here, we observed both sets of parental nucleolus organizing regions (NORs) were expressed after hybridization, but asymmetric silencing of one parental NOR was immediately induced by chromosome doubling, and reversing the ploidy status could not reactivate silenced NORs. Furthermore, increased CHG and CHH DNA methylation on promoters was accompanied by asymmetric silencing of NORs. Enrichment of H3K27me3 and H3K9me2 modifications was also observed to be a direct response to increased DNA methylation and transcriptional inactivation of NOR loci. Both A and D genome NOR loci with these modifications started to disappear in the S4 generation and were completely eliminated by the S7 generation in synthetic tetraploid wheat. Our results indicated that asymmetric epigenetic modification and elimination of rDNA sequences between different donor genomes may lead to stable allopolyploid wheat with increased differentiation and diversity.
DOI:10.1016/j.pbi.2017.01.001URLPMID:28182971 [本文引用: 1]

Following the triplication reported in Brassiceae approximately 10million years ago, and at the basis of rosids approximately 100million years ago, bias in organization and regulation, known as subgenome dominance, has been reported between the three post-polyploidy compartments referenced to as less fractionated (LF), medium fractionated (MF1) and more fractionated (MF2), that have been proposed to derive from an hexaploidization event involving ancestors of 7-14-21 chromosomes. Modern bread wheat experienced similar paleohistory during the last half million year of evolution opening a new hypothesis where the wheat genome is at the earliest stages on the road of diploidization through subgenome dominance driving asymmetry in gene content, gene expression abundance, transposable element content as dynamics and epigenetic control between the A, B and D subgenomes.
DOI:10.1017/S1479262118000254URL [本文引用: 2]
DOI:10.1360/aps040073URL [本文引用: 1]
[本文引用: 1]
[本文引用: 1]
[本文引用: 2]
[本文引用: 2]
[本文引用: 1]
[本文引用: 1]
DOI:10.1126/science.1143986URLPMID:17600208 [本文引用: 3]

Wheat was domesticated about 10,000 years ago and has since spread worldwide to become one of the major crops. Its adaptability to diverse environments and end uses is surprising given the diversity bottlenecks expected from recent domestication and polyploid speciation events. Wheat compensates for these bottlenecks by capturing part of the genetic diversity of its progenitors and by generating new diversity at a relatively fast pace. Frequent gene deletions and disruptions generated by a fast replacement rate of repetitive sequences are buffered by the polyploid nature of wheat, resulting in subtle dosage effects on which selection can operate.
DOI:10.1534/genetics.112.146316URL [本文引用: 2]

The wheat group has evolved through allopolyploidization, namely, through hybridization among species from the plant genera Aegilops and Triticum followed by genome doubling. This speciation process has been associated with ecogeographical expansion and with domestication. In the past few decades, we have searched for explanations for this impressive success. Our studies attempted to probe the bases for the wide genetic variation characterizing these species, which accounts for their great adaptability and colonizing ability. Central to our work was the investigation of how allopolyploidization alters genome structure and expression. We found in wheat that allopolyploidy accelerated genome evolution in two ways: (1) it triggered rapid genome alterations through the instantaneous generation of a variety of cardinal genetic and epigenetic changes (which we termed "revolutionary" changes), and (2) it facilitated sporadic genomic changes throughout the species' evolution (i.e., evolutionary changes), which are not attainable at the diploid level. Our major findings in natural and synthetic allopolyploid wheat indicate that these alterations have led to the cytological and genetic diploidization of the allopolyploids. These genetic and epigenetic changes reflect the dynamic structural and functional plasticity of the allopolyploid wheat genome. The significance of this plasticity for the successful establishment of wheat allopolyploids, in nature and under domestication, is discussed.
In: The Canadian Encyclopedia.
URL [本文引用: 2]
DOI:10.1016/j.fcr.2011.05.020URL [本文引用: 2]
DOI:10.1016/j.fcr.2012.01.003URL [本文引用: 2]
In: Home Grown Cereals Authority Project Report (No. 434).
[本文引用: 1]
[本文引用: 1]
DOI:10.1016/j.fcr.2010.12.003URL [本文引用: 1]
DOI:10.1111/j.1744-7909.2010.00967.xURL [本文引用: 1]

Triticum aestivum L.) in the available published reports. We then carried out a meta-QTL (MQTL) analysis to identify the major and consistent QTLs for these traits. In total, 55 MQTLs were identified, of which 12 significant MQTLs were located on wheat chromosomes 1A, 1B, 2A, 2D, 3B, 4A, 4B, 4D and 5A. Our study showed that the genetic control of yield and its components in common wheat involved the important genes such as Rht and Vrn. Furthermore, several significant MQTLs were found in the chromosomal regions corresponding to several rice genomic locations containing important QTLs for yield related traits. Our results demonstrate that meta-QTL analysis is a powerful tool for confirming the major and stable QTLs and refining their chromosomal positions in common wheat, which may be useful for improving the MAS efficiency of yield related traits.Zhang LY, Liu DC, Guo XL, Yang WL, Sun JZ, Wang DW, Zhang A (2010) Genomic distribution of quantitative trait loci for yield and yield-related traits in common wheat J. Integr. Plant Biol. 52(11), 996–1007]]>
DOI:10.1007/s00122-020-03659-0URLPMID:32803378 [本文引用: 1]

KEY MESSAGE: Quantitation of leaf surface wax on a population of switchgrass identified three significant QTL present across six environments that contribute to leaf glaucousness and wax composition and that show complex genetic x environmental (G x E) interactions. The C4 perennial grass Panicum virgatum (switchgrass) is a native species of the North American tallgrass prairie. This adaptable plant can be grown on marginal lands and is useful for soil and water conservation, biomass production, and as a forage. Two major switchgrass ecotypes, lowland and upland, differ in a range of desirable traits, and the responsible underlying loci can be localized efficiently in a pseudotestcross design. An outbred four-way cross (4WCR) mapping population of 750 F2 lines was used to examine the genetic basis of differences in leaf surface wax load between two lowland (AP13 and WBC) and two upland (DAC and VS16) tetraploid cultivars. The objective of our experiments was to identify wax compositional variation among the population founders and to map underlying loci responsible for surface wax variation across environments. GCMS analyses of surface wax extracted from 4WCR F0 founders and F1 hybrids reveal higher levels of wax in lowland genotypes and show quantitative differences of beta-diketones, primary alcohols, and other wax constituents. The full mapping population was sampled over two seasons from four field sites with latitudes ranging from 30 to 42 degrees N, and leaf surface wax was measured. We identified three high-confidence QTL, of which two displayed significant G x E effects. Over 50 candidate genes underlying the QTL regions showed similarity to genes in either Arabidopsis or barley known to function in wax synthesis, modification, regulation, and transport.
DOI:10.1007/s00122-019-03354-9URLPMID:31049633 [本文引用: 2]

KEY MESSAGE: Introgressing one-eighth of synthetic hexaploid wheat genome through a double top-cross plus a two-phase selection is an effective strategy to develop high-yielding wheat varieties. The continued expansion of the world population and the likely onset of climate change combine to form a major crop breeding challenge. Genetic advances in most crop species to date have largely relied on recombination and reassortment within a relatively narrow gene pool. Here, we demonstrate an efficient wheat breeding strategy for improving yield potentials by introgression of multiple genomic regions of de novo synthesized wheat. The method relies on an initial double top-cross (DTC), in which one parent is synthetic hexaploid wheat (SHW), followed by a two-phase selection procedure. A genotypic analysis of three varieties (Shumai 580, Shumai 969 and Shumai 830) released from this program showed that each harbors a unique set of genomic regions inherited from the SHW parent. The first two varieties were generated from very small populations, whereas the third used a more conventional scale of selection since one of bread wheat parents was a pre-breeding material. The three varieties had remarkably enhanced yield potential compared to those developed by conventional breeding. A widely accepted consensus among crop breeders holds that introducing unadapted germplasm, such as landraces, as parents into a breeding program is a risky proposition, since the size of the breeding population required to overcome linkage drag becomes too daunting. However, the success of the proposed DTC strategy has demonstrated that novel variation harbored by SHWs can be accessed in a straightforward, effective manner. The strategy is in principle generalizable to any allopolyploid crop species where the identity of the progenitor species is known.
DOI:10.1007/s11032-016-0541-4URL [本文引用: 1]
DOI:10.1105/tpc.114.124388URL [本文引用: 1]

Nascent allohexaploid wheat may represent the initial genetic state of common wheat (Triticum aestivum), which arose as a hybrid between Triticum turgidum (AABB) and Aegilops tauschii (DD) and by chromosome doubling and outcompeted its parents in growth vigor and adaptability. To better understand the molecular basis for this success, we performed mRNA and small RNA transcriptome analyses in nascent allohexaploid wheat and its following generations, their progenitors, and the natural allohexaploid cultivar Chinese Spring, with the assistance of recently published A and D genome sequences. We found that nonadditively expressed protein-coding genes were rare but relevant to growth vigor. Moreover, a high proportion of protein-coding genes exhibited parental expression level dominance, with genes for which the total homoeolog expression level in the progeny was similar to that in T. turgidum potentially participating in development and those with similar expression to that in Ae. tauschii involved in adaptation. In addition, a high proportion of microRNAs showed nonadditive expression upon polyploidization, potentially leading to differential expression of important target genes. Furthermore, increased small interfering RNA density was observed for transposable element-associated D homoeologs in the allohexaploid progeny, which may account for biased repression of D homoeologs. Together, our data provide insights into small RNA-mediated dynamic homoeolog regulation mechanisms that may contribute to heterosis in nascent hexaploid wheat.
DOI:10.1016/j.molp.2015.02.016URLPMID:25747845 [本文引用: 1]

Bread wheat (or common wheat, Triticum aestivum) is an allohexaploid (AABBDD, 2n = 6x = 42) that arose by hybridization between a cultivated tetraploid wheat T. turgidum (AABB, 2n = 4x = 28) and the wild goatgrass Aegilops tauschii (DD, 2n = 2x = 14). Polyploidization provided niches for rigorous genome modification at cytogenetic, genetic, and epigenetic levels, rendering a broader spread than its progenitors. This review summarizes the latest advances in understanding gene regulation mechanisms in newly synthesized allohexaploid wheat and possible correlation with polyploid growth vigor and adaptation. Cytogenetic studies reveal persistent association of whole-chromosome aneuploidy with nascent allopolyploids, in contrast to the genetic stability in common wheat. Transcriptome analysis of the euploid wheat shows that small RNAs are driving forces for homoeo-allele expression regulation via genetic and epigenetic mechanisms. The ensuing non-additively expressed genes and those with expression level dominance to the respective progenitor may play distinct functions in growth vigor and adaptation in nascent allohexaploid wheat. Further genetic diploidization of allohexaploid wheat is not random. Regional asymmetrical gene distribution, rather than subgenome dominance, is observed in both synthetic and natural allohexaploid wheats. The combinatorial effects of diverged genomes, subsequent selection of specific gene categories, and subgenome-specific traits are essential for the successful establishment of common wheat.
DOI:10.1016/j.pbi.2016.02.006URLPMID:26943938 [本文引用: 1]

The formation of an allopolyploid species involves the merger of genomes with separate evolutionary histories and thereby different genomic legacies. Contrary to expectations from theory, genes from one are often lost preferentially in allopolyploids - there is biased fractionation. Here, we provide an overview of two ways in which the genomic legacies of the progenitors may impact the fate of duplicated genes in allopolyploids. Specifically, we discuss the role of homeolog expression biases in setting the stage for biased fractionation, and the evidence for transposable element silencing as a possible mechanism for homeolog expression biases. Finally, we highlight how differences between the progenitors with respect to accumulation of deleterious variation may affect trajectories of duplicate gene evolution in allopolyploids.
DOI:10.1016/j.gde.2018.01.004URLPMID:29438956 [本文引用: 1]

We consider the rapidly advancing discipline of plant evolutionary genomics, with a focus on the evolution of polyploid genomes. In many lineages, polyploidy is followed by 'biased fractionation', the unequal loss of genes from ancestral progenitor genomes. Mechanistically, it has been proposed that biased fractionation results from changes in the epigenetic landscape near genes, likely mediated by transposable elements. These epigenetic changes result in unequal gene expression between duplicates, establishing differential fitness that leads to biased gene loss with respect to ancestral genomes. We propose a unifying conceptual framework and a set of testable hypotheses based on this model, relating genome size, the proximity of transposable elements to genes, epigenetic reprogramming, chromatin accessibility, and gene expression.
DOI:10.1111/nph.15256URLPMID:29882360 [本文引用: 1]

Contents Summary 87 I. Introduction 87 II. Evolution in action: subgenome dominance within newly formed hybrids and polyploids 88 III. Summary and future directions 90 Acknowledgements 92 References 92 SUMMARY: The merger of divergent genomes, via hybridization or allopolyploidization, frequently results in a 'genomic shock' that induces a series of rapid genetic and epigenetic modifications as a result of conflicts between parental genomes. This conflict among the subgenomes routinely leads one subgenome to become dominant over the other subgenome(s), resulting in subgenome biases in gene content and expression. Recent advances in methods to analyze hybrid and polyploid genomes with comparisons to extant parental progenitors have allowed for major strides in understanding the mechanistic basis for subgenome dominance. In particular, our understanding of the role that homoeologous exchange might play in subgenome dominance and genome evolution is quickly growing. Here we describe recent discoveries uncovering the underlying mechanisms and provide a framework to predict subgenome dominance in hybrids and allopolyploids with far-reaching implications for agricultural, ecological, and evolutionary research.
DOI:10.1016/j.tplants.2018.01.002URLPMID:29433919 [本文引用: 1]

The classical example of nonadditive contributions of the two parents to allopolyploids is nucleolar dominance, which entails silencing of one parental set of ribosomal RNA genes. This has been observed for many other loci. The prevailing explanation for this genome-wide expression disparity is that the two merged genomes differ in their transposable element (TE) complement and in their level of TE-mediated repression of gene expression. Alternatively, and not exclusively, gene expression dominance may arise from mismatches between trans effectors and their targets. Here, we explore quantitative models of regulatory mismatches leading to gene expression dominance. We also suggest that, when pairs of merged genomes are similar from one allopolyploidization event to another, gene-level and genome dominance patterns should also be similar.
DOI:10.1016/j.tplants.2017.06.003URLPMID:28648886 [本文引用: 2]

An interesting and possibly unique pattern of genome evolution following polyploidy can be observed among allopolyploids of the Triticum and Aegilops genera (wheat group). Most polyploids in this group are presumed to share a common unaltered (pivotal) subgenome (U, D, or A) together with one or two modified (differential) subgenomes, a status that has been referred to as 'pivotal-differential' genome evolution. In this review we discuss various mechanisms that could be responsible for this evolutionary pattern, as well as evidence for and against the putative evolutionary mechanisms involved. We suggest that, in light of recent advances in genome sequencing and related technologies in the wheat group, the time has come to reopen the investigation into pivotal-differential genome evolution.
DOI:10.1016/j.cell.2017.05.038URLPMID:28622505 [本文引用: 1]

A central goal of genetics is to understand the links between genetic variation and disease. Intuitively, one might expect disease-causing variants to cluster into key pathways that drive disease etiology. But for complex traits, association signals tend to be spread across most of the genome-including near many genes without an obvious connection to disease. We propose that gene regulatory networks are sufficiently interconnected such that all genes expressed in disease-relevant cells are liable to affect the functions of core disease-related genes and that most heritability can be explained by effects on genes outside core pathways. We refer to this hypothesis as an
[本文引用: 1]
DOI:10.1139/gen-2019-0199URLPMID:32552081 [本文引用: 1]

The subterranean islands hypothesis for calcretes of the Yilgarn region in Western Australia applies to many stygobitic (subterranean-aquatic) species that are
DOI:10.1017/S1479262118000175URL [本文引用: 1]
DOI:10.1007/s10722-018-0649-yURL [本文引用: 1]
DOI:10.1186/s12870-020-2309-6URLPMID:32131739 [本文引用: 1]

BACKGROUND: In contrast to most animal species, polyploid plant species are quite tolerant of aneuploidy. Here, the global transcriptome of four aneuploid derivatives of a synthetic hexaploid wheat line was acquired, with the goal of characterizing the relationship between gene copy number and transcript abundance. RESULTS: For most of the genes mapped to the chromosome involved in aneuploidy, the abundance of transcripts reflected the gene copy number. Aneuploidy had a greater effect on the strength of transcription of genes mapped to the chromosome present in a noneuploid dose than on that of genes mapped elsewhere in the genome. Overall, changing the copy number of one member of a homeologous set had little effect on the abundance of transcripts generated from the set of homeologs as a whole, consistent with the tolerance of aneuploidy exhibited by allopolyploids, whether in the form of a chromosomal deficit (monosomy) or chromosomal excess (trisomy). CONCLUSIONS: Our findings shed new light on the genetic regulation of homeoallele transcription and contribute to a deeper understanding of allopolyploid genome evolution, with implications for the breeding of polyploid crops.
DOI:10.1007/BF00126756URL [本文引用: 1]
DOI:10.1016/S1673-8527(08)60145-9URL [本文引用: 1]

AbstractSynthetic hexaploid wheat (Triticum turgidum × Aegilops tauschii) was created to explore for novel genes from T. turgidum and Ae. tauschii that can be used for common wheat improvement. In the present paper, research advances on the utilization of synthetic hexaploid wheat for wheat genetic improvement in China are reviewed. Over 200 synthetic hexaploid wheat (SHW) accessions from the International Maize and Wheat Improvement Centre (CIMMYT) were introduced into China since 1995. Four cultivars derived from these, Chuanmai 38, Chuanmai 42, Chuanmai 43 and Chuanmai 47, have been released in China. Of these, Chuanmai 42, with large kernels and resistance to stripe rust, had the highest average yield (> 6 t/ha) among all cultivars over two years in Sichuan provincial yield trials, outyielding the commercial check cultivar Chuanmai 107 by 22.7%. Meanwhile, by either artificial chromosome doubling via colchicine treatment or spontaneous chromosome doubling via a union of unreduced gametes (2n) from T. turgidum-Ae. tauschii hybrids, new SHW lines were produced in China. Mitotic-like meiosis might be the cytological mechanism of spontaneous chromosome doubling. SHW lines with genes for spontaneous chromosome doubling may be useful for producing new SHW-alien amphidiploids and double haploid in wheat genetic improvement.]]>
In: 4th Stadler Genetics Symposium,
[本文引用: 1]
DOI:10.1007/BF00223930URLPMID:24170007 [本文引用: 1]

Several Triticum aestivum L.-Haynaldia villosa disomic 6VS/6AL translocation lines with powdery mildew resistance were developed from the hybridization between common wheat cultivar Yangmai 5 and alien substitution line 6V(6A). Mitotic and meiotic C-banding analysis, aneuploid analysis with double ditelosomic stocks, in situ hybridization, as well as the phenotypic assessment of powdery mildew resistance, were used to characterize these lines. The same translocated chromosome, with breakpoints near the centromere, appears to be present in all the lines, despite variation among the lines in their morphology and agronomic characteristics. The resistance gene, conferred by H. villosa and designated as Pm21, is a new and promising source of powdery mildew resistance in wheat breeding.
[本文引用: 1]
DOI:10.1016/S1673-8527(08)60062-4URLPMID:18721781 [本文引用: 1]

Wheat is a staple food crop in the world as well as in China. Because of the progress of wheat breeding and other agricultural scitechnologies, the wheat grain yield per unit area has increased more than five folds from 1952 to 2006 in China. The first part of this article briefly reviews the history of wheat breeding in China. Second, the establishment of
DOI:10.1038/s41598-019-46197-6URLPMID:31273296 [本文引用: 1]

Agropyron cristatum (L.) Gaertn. (P genome) is cultivated as pasture fodder and can provide many desirable genes for wheat improvement. With the development of genomics and fluorescence in situ hybridization (FISH) technology, probes for identifying plant chromosomes were also developed. However, there are few reports on A. cristatum chromosomes. Here, FISH with the repeated sequences pAcTRT1 and pAcpCR2 enabled the identification of all diploid A. cristatum chromosomes. An integrated idiogram of A. cristatum chromosomes was constructed based on the FISH patterns of five diploid A. cristatum individuals. Structural polymorphisms of homologous chromosomes were observed not only among different individuals but also within individuals. Moreover, seventeen wheat-A. cristatum introgression lines containing different P genome chromosomes were identified with pAcTRT1 and pAcpCR2 probes. The arrangement of chromosomes in diploid A. cristatum was determined by identifying correspondence between the P chromosomes in these genetically identified introgression lines and diploid A. cristatum chromosomes. The two probes were also effective for discriminating all chromosomes of tetraploid A. cristatum, and the differences between two tetraploid A. cristatum accessions were similar to the polymorphisms among individuals of diploid A. cristatum. Collectively, the results provide an effective means for chromosome identification and phylogenetic studies of P genome chromosomes.
DOI:10.1105/tpc.113.114553URL [本文引用: 1]

Rye (Secale cereale) is closely related to wheat (Triticum aestivum) and barley (Hordeum vulgare). Due to its large genome (similar to 8 Gb) and its regional importance, genome analysis of rye has lagged behind other cereals. Here, we established a virtual linear gene order model (genome zipper) comprising 22,426 or 72% of the detected set of 31,008 rye genes. This was achieved by high-throughput transcript mapping, chromosome survey sequencing, and integration of conserved synteny information of three sequenced model grass genomes (Brachypodium distachyon, rice [Oryza sativa], and sorghum [Sorghum bicolor]). This enabled a genome-wide high-density comparative analysis of rye/barley/model grass genome synteny. Seventeen conserved syntenic linkage blocks making up the rye and barley genomes were defined in comparison to model grass genomes. Six major translocations shaped the modern rye genome in comparison to a putative Triticeae ancestral genome. Strikingly dissimilar conserved syntenic gene content, gene sequence diversity signatures, and phylogenetic networks were found for individual rye syntenic blocks. This indicates that introgressive hybridizations (diploid or polyploidy hybrid speciation) and/or a series of whole-genome or chromosome duplications played a role in rye speciation and genome evolution.
In: Proceedings of the 4th International Wheat Genetics Symposium.
[本文引用: 1]
DOI:10.1073/pnas.1016981108URLPMID:21508323 [本文引用: 1]

Powdery mildew resistance gene Pm21, located on the chromosome 6V short arm of Haynaldia villosa and transferred to wheat as a 6VS.6AL translocation (T6VS.6AL), confers durable and broad-spectrum resistance to wheat powdery mildew. Pm21 has become a key gene resource for powdery mildew resistance breeding all over the world. In China, 12 wheat varieties containing Pm21 have been planted on more than 3.4 million hectares since 2002. Pm21 has been intractable to molecular genetic mapping because the 6VS does not pair and recombine with the 6AS. Moreover, all known accessions of H. villosa are immune to powdery mildew fungus. Pm21 is still defined by cytogenetics as a locus. In the present study, a putative serine and threonine protein kinase gene Stpk-V was cloned and characterized with an integrative strategy of molecular and cytogenetic techniques. Stpk-V is located on the Pm21 locus. The results of a single cell transient expression assay showed that Stpk-V could decrease the haustorium index dramatically. After the Stpk-V was transformed into a susceptible wheat variety Yangmai158, the characterized transgenic plants showed high and broad-spectrum powdery mildew resistance similar to T6VS.6AL. Silencing of the Stpk-V by virus-induced gene silencing in both T6VS.6AL and H. villosa resulted in their increased susceptibility. Stpk-V could be induced by Bgt and exogenous H(2)O(2), but it also mediated the increase of endogenous H(2)O(2), leading to cell death and plant resistance when the plant was attacked by Bgt.
DOI:10.1038/nature11997URL [本文引用: 1]

Bread wheat (Triticum aestivum, AABBDD) is one of the most widely cultivated and consumed food crops in the world. However, the complex polyploid nature of its genome makes genetic and functional analyses extremely challenging. The A genome, as a basic genome of bread wheat and other polyploid wheats, for example, T. turgidum (AABB), T. timopheevii (AAGG) and T. zhukovskyi (AAGGA(m)A(m)), is central to wheat evolution, domestication and genetic improvement(1). The progenitor species of the A genome is the diploid wild einkorn wheat T. urartu(2), which resembles cultivated wheat more extensively than do Aegilops speltoides (the ancestor of the B genome(3)) and Ae. tauschii (the donor of the D genome(4)), especially in the morphology and development of spike and seed. Here we present the generation, assembly and analysis of a whole-genome shotgun draft sequence of the T. urartu genome. We identified protein-coding gene models, performed genome structure analyses and assessed its utility for analysing agronomically important genes and for developing molecular markers. Our T. urartu genome assembly provides a diploid reference for analysis of polyploid wheat genomes and is a valuable resource for the genetic improvement of wheat.
DOI:10.1038/nature12028URL [本文引用: 1]

About 8,000 years ago in the Fertile Crescent, a spontaneous hybridization of the wild diploid grass Aegilops tauschii (2n = 14; DD) with the cultivated tetraploid wheat Triticum turgidum (2n = 4x = 28; AABB) resulted in hexaploid wheat (T. aestivum; 2n = 6x = 42; AABBDD)(1,2). Wheat has since become a primary staple crop worldwide as a result of its enhanced adaptability to a wide range of climates and improved grain quality for the production of baker's flour(2). Here we describe sequencing the Ae. tauschii genome and obtaining a roughly 90-fold depth of short reads from libraries with various insert sizes, to gain a better understanding of this genetically complex plant. The assembled scaffolds represented 83.4% of the genome, of which 65.9% comprised transposable elements. We generated comprehensive RNA-Seq data and used it to identify 43,150 protein-coding genes, of which 30,697 (71.1%) were uniquely anchored to chromosomes with an integrated high-density genetic map. Whole-genome analysis revealed gene family expansion in Ae. tauschii of agronomically relevant gene families that were associated with disease resistance, abiotic stress tolerance and grain quality. This draft genome sequence provides insight into the environmental adaptation of bread wheat and can aid in defining the large and complicated genomes of wheat species.
DOI:10.1126/science.1251788URLPMID:25035500 [本文引用: 1]

An ordered draft sequence of the 17-gigabase hexaploid bread wheat (Triticum aestivum) genome has been produced by sequencing isolated chromosome arms. We have annotated 124,201 gene loci distributed nearly evenly across the homeologous chromosomes and subgenomes. Comparative gene analysis of wheat subgenomes and extant diploid and tetraploid wheat relatives showed that high sequence similarity and structural conservation are retained, with limited gene loss, after polyploidization. However, across the genomes there was evidence of dynamic gene gain, loss, and duplication since the divergence of the wheat lineages. A high degree of transcriptional autonomy and no global dominance was found for the subgenomes. These insights into the genome biology of a polyploid crop provide a springboard for faster gene isolation, rapid genetic marker development, and precise breeding to meet the needs of increasing food demand worldwide.
[本文引用: 1]
DOI:10.1139/g77-063URL [本文引用: 1]
DOI:10.2135/cropsci2017.03.0192URL [本文引用: 1]
DOI:10.3389/fpls.2020.00252URLPMID:32211007 [本文引用: 1]

Breeding progress in most crops has relied heavily on the exploitation of variation within the species' primary gene pool, a process which is destined to fail once the supply of novel variants has been exhausted. Accessing a crop's secondary gene pool, as represented by its wild relatives, has the potential to greatly expand the supply of usable genetic variation. The crop in which this approach has been most strongly championed is bread wheat (Triticum aestivum), a species which is particularly tolerant of the introduction of chromosomal segments of exotic origin thanks to the genetic buffering afforded by its polyploid status. While the process of introgression can be in itself cumbersome, a larger problem is that linkage drag and/or imperfect complementation frequently impose a yield and/or quality penalty, which explains the reluctance of breeders to introduce such materials into their breeding populations. Thanks to the development of novel strategies to induce introgression and of genomic tools to facilitate the selection of desirable genotypes, introgression breeding is returning as a mainstream activity, at least in wheat. Accessing variation present in progenitor species has even been able to drive genetic advance in grain yield. The current resurgence of interest in introgression breeding can be expected to result in an increased deployment of exotic genes in commercial wheat cultivars.
[本文引用: 1]
DOI:10.3389/fpls.2019.00585URLPMID:31143197 [本文引用: 1]

Aegilops species have significantly contributed to wheat breeding despite the difficulties involved in the handling of wild species, such as crossability and incompatibility. A number of biotic resistance genes have been identified and incorporated into wheat varieties from Aegilops species, and this genus is also contributing toward improvement of complex traits such as yield and abiotic tolerance for drought and heat. The D genome diploid species of Aegilops tauschii has been utilized most often in wheat breeding programs. Other Aegilops species are more difficult to utilize in the breeding because of lower meiotic recombination frequencies; generally they can be utilized only after extensive and time-consuming procedures in the form of translocation/introgression lines. After the emergence of Ug99 stem rust and wheat blast threats, Aegilops species gathered more attention as a form of new resistance sources. This article aims to update recent progress on Aegilops species, as well as to cover new topics around their use in wheat breeding.
DOI:10.1146/annurev.ge.10.120176.000335URLPMID:797311 [本文引用: 1]
DOI:10.1007/BF00036700URL [本文引用: 1]
DOI:10.1007/s10577-006-1108-8URL [本文引用: 1]

Sears (1956) pioneered plant chromosome engineering 50years ago by directed transfer of a leaf rust resistance gene from an alien chromosome to a wheat chromosome using X-ray irradiation and an elegant cytogenetic scheme. Since then many other protocols have been reported, but the one dealing with induced homoeologous pairing and recombination is the most powerful, and has been extensively used in wheat. Here, we briefly review the current status of homoeologous recombination-based chromosome engineering research in plants with a focus on wheat, and demonstrate that integrated use of cytogenetic stocks and molecular resources can enhance the efficiency and precision of homoeologus-based chromosome engineering. We report the results of an experiment on homoeologous recombination-based transfer of virus resistance from an alien chromosome to a wheat chromosome, its characterization, and the prospects for further engineering by a second round of recombination. A proposal is presented for genome-wide, homoeologous recombination-based engineering for efficient mining of gene pools of wild relatives for crop improvement.]]>