
Bioinformatics analysis and response to nitrate-cadmium stress of NRT1.5 and NRT1.8 family genes in Brassica napus
LIANG Gui-Hong1,2, HUA Ying-Peng1,2, ZHOU Ting1,2, LIAO Qiong1,2, SONG Hai-Xing1,2, ZHANG Zhen-Hua
通讯作者:
收稿日期:2018-07-18接受日期:2018-10-8网络出版日期:2018-11-02
基金资助: |
Received:2018-07-18Accepted:2018-10-8Online:2018-11-02
Fund supported: |
作者简介 About authors
E-mail:ghliang1119@163.com。

摘要
关键词:
Abstract
Keywords:
PDF (5517KB)元数据多维度评价相关文章导出EndNote|Ris|Bibtex收藏本文
本文引用格式
梁桂红, 华营鹏, 周婷, 廖琼, 宋海星, 张振华. 甘蓝型油菜NRT1.5和NRT1.8家族基因的生物信息学分析及其对氮-镉胁迫的响应[J]. 作物学报, 2019, 45(3): 365-380. doi:10.3724/SP.J.1006.2019.84099
LIANG Gui-Hong, HUA Ying-Peng, ZHOU Ting, LIAO Qiong, SONG Hai-Xing, ZHANG Zhen-Hua.
氮(nitrogen, N)是植物生长发育所必需的大量营养元素, 广泛参与生物体蛋白质和核酸等大分子化合物的合成, 在生物体的物质和能量代谢过程中起重要作用[1]。铵态氮(NH4+-N)和硝态氮(NO3--N)是作物氮素吸收利用的主要形态, 其中硝态氮是旱地作物吸收利用的主要氮源[2]。植物从土壤中吸收硝酸盐是一个主动运输的过程, 其能量主要来源于质子电化学势梯度, 协助硝酸盐转运的载体称为硝酸盐转运体(nitrate transporters, NRTs)[3], 包括低亲和硝酸盐转运体NRT1 (nitrate transporter 1)和高亲和硝酸盐转运体NRT2 (nitrate transporter 2) 2个家族[4,5]。
硝酸盐被植物根系细胞吸收后, 在植物体内的分配主要有硝酸盐短途分配(液泡硝酸盐分配)和长途转运(硝酸盐在根部和地上部的转运)两种途径, 它们共同调控着硝酸盐的利用效率, 进而影响作物的氮素利用率(Nitrogen Use Efficiency, NUE)[6]。大部分的硝酸盐在蒸腾拉力的作用下经木质部长途转运, 将硝酸盐分配到地上部的不同组织和器官中被同化和利用。该过程主要由向上运输的木质部和向下运输的韧皮部介导[7]。有研究证明, 拟南芥木质部介导的硝酸盐长途转运及其在根和地上部的分配, 主要受NRT1家族的2个成员NRT1.5和NRT1.8的协同调控, 且二者的表达都受到硝酸盐的强烈诱导[8,9,10,11,12]。AtNRT1.5主要在根部原生木质部的中柱鞘细胞中表达, 负责将根部细胞质中的硝酸盐装载进入木质部以运往地上部分。当其功能缺失后, 突变体木质部伤流液中的硝酸盐含量降低, 植物向上转运硝酸盐的能力减弱[8]。AtNRT1.8在木质部薄壁细胞表达, 通过调控硝酸盐在木质部的卸载进而影响硝酸盐从根向地上部分的运输[10]。由此可见, NRT1.5和NRT1.8基因的表达模式相反, 但共同影响硝酸盐通过木质部向地上部分的长途转运, 进而影响作物的产量和氮素利用率。此外, NRT1.5和NRT1.8通过调控硝酸盐在地上部和根部的分配, 从而影响植物对镉胁迫的耐受能力。研究发现镉处理条件下, AtNRT1.8基因的表达显著上调, 抑制AtNRT1.5的表达, 使更多的硝酸盐从地上部运输到根部, 根部较高浓度的硝酸盐有利于增强植株对镉胁迫的耐受能力[10,13]。
在我国, 油菜作为一种重要的油料作物, 种植面积和总产量约占世界的1/3[14], 在有效供给油脂和改善生活结构等方面起着重要作用。甘蓝型油菜(Brassica napus L., AnAnCnCn, 约1345 Mb, 2n = 4x = 38)属于十字花科芸薹属[15], 由白菜(B. rapa, ArAr, ~485 Mb, 2n = 2x = 20)[16]和甘蓝(B. oleracea, CoCo, ~630 Mb, 2n = 2x = 18)[17]这2个二倍体基本种在7500年前通过天然远缘杂交形成, 其全基因组包含约十万多个蛋白编码基因[18,19]。甘蓝型油菜进化、遗传的复杂性形成了其复杂的基因组, 在一定程度上影响了各种功能基因在甘蓝型油菜生长和发育中的作用。
硝酸盐转运蛋白负责植物根系对硝酸盐的吸收和转运, 一直是植物营养学领域研究的热点之一。AtNRT1家族有53个家族成员, 目前, 其他作物中对于NRT1与硝酸盐长途转运的关系研究主要集中在小麦和水稻中, 但由于非模式植物研究的突变体材料和克隆基因技术的限制, 只在小麦中研究了NRT1.5和NRT1.8基因对氮胁迫的响应[20], 以及水稻中OsNRT1.5过表达材料的氮素生理特性[21], 对NRT1.5和NRT1.8基因的结构功能及发育进化分析较少[22], 尤其在经济作物甘蓝型油菜中的研究更少。
本研究利用AtNRT1.5和AtNRT1.8的基因序列和蛋白序列, 对甘蓝型油菜中NRT1.5和NRT1.8基因进行鉴定和分析, 通过对基因生物信息数据的挖掘和整理, 分析该基因在白菜、甘蓝和甘蓝型油菜中的系统进化关系和保守基序特征, 预测其蛋白质的理化性质等, 为进一步系统研究NRT1.5和NRT1.8基因在其他物种中的功能提供参考依据。
1 材料与方法
1.1 试验材料
水培试验所用的甘蓝型油菜(B. napus L.)品种为“湘油15号”, 油菜种子来自国家油料作物改良中心湖南分中心官春云院士团队。1.2 白菜、甘蓝及甘蓝型油菜NRT1.5和NRT1.8基因序列的数据来源及检索
以拟南芥NRT1.5 (AT1G32450)和NRT1.8 (AT4G21680)的基因序列为种子序列, 利用BLAST搜索白菜、甘蓝和甘蓝型油菜中NRT1.5和NRT1.8的目的基因序列, 同时下载筛选到的基因序列对应编码的氨基酸序列, 将其保存为FASTA格式。本研究所用的数据库包括拟南芥信息资源(TAIR)(https:// www.arabidopsis.org/)。白菜(B. rapa)、甘蓝(B. oleracea)及甘蓝型油菜(B. napus)基因组全长DNA序列、CDS序列、氨基酸序列及其注释信息均从BRAD (http://brassicadb.org/brad/)数据库下载[23]。胡杨的氨基酸序列从NCBI (https://www.ncbi.nlm.nih.gov/)在线数据库下载。高粱、水稻、粟、玉米等单子叶植物的氨基酸序列从JGI (https://phytozome.jgi.doe. gov/pz/portal.html#)在线数据库下载。1.3 白菜、甘蓝及甘蓝型油菜的基因命名
对三种芸薹属作物基因的命名以物种名称+染色体(后缀.)+拟南芥中的同源基因。例如, BnaA5.NRT1.5代表甘蓝型油菜中定位在A5染色体上的NRT1.5基因。1.4 白菜、甘蓝及甘蓝型油菜NRT1.5和NRT1.8蛋白的分子特性分析
采用ExPASy ProtoParam (https://web.expasy. org/protparam/)[24]在线软件预测白菜、甘蓝和甘蓝型油菜NRT1.5和NRT1.8蛋白的氨基酸数目和组成、相对分子量(MW)、理论等电点(pI)、蛋白质的亲水性(GRAVY)及稳定性等理化性质。1.5 白菜、甘蓝及甘蓝型油菜NRT1.5和NRT1.8基因的内含子-外显子结构特征
从拟南芥信息资源(TAIR)和BRAD数据库中下载拟南芥、白菜、甘蓝和甘蓝型油菜的全长基因组DNA序列和编码序列(CDS), 利用在线软件GSDS 2.0 (http://gsds.cbi.pku.edu.cn/)描绘内含子和外显子结构[25]。1.6 白菜、甘蓝及甘蓝型油菜NRT1.5和NRT1.8基因的染色体定位分析
利用BLAST分别确定NRT1.5和NRT1.8在白菜、甘蓝和甘蓝型油菜中每条染色体上的起始位置, 并在GBrowse (http://brassicadb.org/cgi-bin/gbrowse/ Brassica/)中查找4个物种的各条染色体全长。利用软件MapInspect v. 2010 (http://www.softsea.com/ review/MapInspect.html)绘制NRT1.5和NRT1.8在白菜、甘蓝及甘蓝型油菜中染色体的分布情况。一般认为, 在100-kb基因组范围内排列有2个或以上基因, 我们定义其为串联重复基因[26]。1.7 白菜、甘蓝及甘蓝型油菜NRT1.5和NRT1.8的系统发育关系
提取3种芸薹属作物NRT1.5和NRT1.8蛋白的氨基酸序列, 利用软件MEGA 7.0 (http://www. megasoftware.net/)[27]分别进行多重比对分析, 去除冗余序列[28]。采用邻接法(Neighbor Joining, NJ)[29]对白菜、甘蓝和甘蓝型油菜NRT1.5和NRT1.8的比对蛋白序列构建系统发育树, 并进行自检, 设定Bootstrap重复为1000, 去除bootstrap支持率低于50%的节点。1.8 白菜、甘蓝及甘蓝型油菜NRT1.5和NRT1.8蛋白的进化选择压力分析
为了研究NRT1.5和NRT1.8蛋白在物种进化过程中是否涉及到达尔文的选择, 分别对拟南芥和白菜、甘蓝和甘蓝型油菜中NRT1.5和NRT1.8的CDS序列和蛋白序列进行比对, 利用在线软件PAL2NAL (http://www.bork.embl.de/pal2nal/)计算核苷酸的同义突变频率(synonymous, Ks)和非同义突变频率(non-synonymous, Ka), 及其比值Ka/Ks。1.9 白菜、甘蓝及甘蓝型油菜NRT1.5和NRT1.8蛋白的保守基序和跨膜结构域预测分析
为了研究拟南芥和3种芸薹属作物中NRT1.5和NRT1.8蛋白的结构差异, 利用软件MEME v. 4.12.0 (http://meme-suite.org/tools/meme)[30]对蛋白质的保守基序进行预测和分析。所用默认参数, 最佳基序宽度为6~50 bp, 基序最大数为15。利用在线软件NovoPro (http://www.novopro.cn/tools/color_align_ prop.html)进行蛋白保守序列分析。同时利用在线软件TMpred Server (https://embnet.vital-it.ch/software/ TMPRED_form.html)对甘蓝型油菜的NRT1.5和NRT1.8蛋白序列进行跨膜结构域的预测和分析[31]。1.10 甘蓝型油菜NRT1.5和NRT1.8基因在氮-镉胁迫条件下的表达分析
1.10.1 甘蓝型油菜的培养条件 油菜苗期培养试验在光照培养室中, 其温度22℃, 光照周期14 h (光照)/10 h (黑暗), 光照强度300~320 μmol m-2 s-1, 湿度60%~75%。选取大小一致的油菜种子, 使用75%的酒精杀菌处理10 min, 用超纯水将种子表面冲洗干净后, 4℃下用无菌水浸泡24 h, 期间换水5~6次。将浸泡后的种子均匀播种在塑料育苗盘表面固定的纱布上, 育苗盘中加适量超纯水。育苗7 d后, 将长势一致的幼苗移栽到盛有4 L营养液的黑色塑料盒中。营养液浓度为Hoagland完全营养液[32]的1/4。1.10.2 低氮处理高通量转录组测序试验 油菜幼苗以硝态氮为唯一氮源, 在6.0 mmol L-1 NO3-中培养10 d后, 转移至0.3 mmol L-1 NO3-条件下处理0、3和72 h, 对地上部(S)和根(R)取样, 将每个样品单独分装到干净的离心管中, 并作好标记, 在液氮中速冻, -80℃冰箱中保存待测, 每个处理作3个独立的生物学重复。测序平台为Illumina Hiseq 4000[33], 每个样品产生6.0 Gb测序数据, 两端配对末端(PE)读数, 由RNA-seq数据确定[34]转录组丰度(FPKM值)。
1.10.3 镉处理高通量转录组测序试验 油菜幼苗在无镉的正常营养液中培养10 d后, 将油菜样品分成两组, 一组继续在正常营养液中培养, 另一组转移至含10 μmol L-1镉的营养液中处理6 h, 分别对两组样品的地上部(S)和根(R)取样, 将每个样品单独分装到干净的离心管中, 作好标记。样品的高通量转录组测序试验参照步骤1.10.2。
2 结果与分析
2.1 白菜、甘蓝及甘蓝型油菜NRT1.5和NRT1.8基因/蛋白的分子特性
利用AtNRT1.5和AtNRT1.8数据在甘蓝型油菜全基因组数据库中分别检索到8条NRT1.5和8条NRT1.8同源基因序列(表1和表2)。白菜、甘蓝和甘蓝型油菜的NRT1.5蛋白均由22种氨基酸组成, 其中Leu和Ser的含量最高。基因编码区长度在1236 bp (BolC5.NRT1.5b)~1863 bp (BnaA9.NRT1.5)之间, 编码411~620个氨基酸, 预测蛋白质的理论分子量为45.80~69.41 kD。8个NRT1.5蛋白中酸性氨基酸(Asp+Glu)个数均多于碱性氨基酸(Arg+Lys), 等电点(pI)范围在5.63~6.35, 为酸性蛋白。Guruprasad等[35]认为, 蛋白质的不稳定系数大于40时为不稳定蛋白。NRT1.5蛋白的不稳定性系数在27.54~34.68间, 均小于40, 属于稳定性蛋白。氨基酸的亲水性指数(GRAVY)是氨基酸的化学特性, 是描述其支链的亲水性或疏水性程度的值, 根据亲水性指数介于-0.5~0.5为两性蛋白(GRAVY为负值表示亲水性, 正值表示疏水性)的原则[36], 氨基酸的亲水性指数在-0.006 (BolC5.NRT1.5b)~0.096 (BraA5.NRT1.5)之间, 表明NRT1.5主要为两性蛋白。脂肪指数在86.13 (BolC5.NRT1.5b)~89.77 (BnaC5.NRT1.5b)之间。Table 1
表1
表1白菜、甘蓝和甘蓝型油菜NRT1.5和NRT1.8基因的分子特性
Table 1
基因名 Gene name | 基因编号 Gene ID | 分区 Block | 亚类 Subgenome | 物理位置 Physical position | 编码区长度 CDS (bp) | 外显子/内含子Exon/intron |
---|---|---|---|---|---|---|
NRT1.5 | ||||||
BraA5.NRT1.5 | Bra010165 | B | MF2 | 15109908-15113350 | 1815 | 5/4 |
BraA9.NRT1.5 | Bra023267 | B | LF | 20166714-20170510 | 1833 | 5/4 |
BolC5.NRT1.5a | Bol020904 | B | MF2 | 13227461-13230901 | 1812 | 5/4 |
BolC5.NRT1.5b | Bol022226 | B | LF | 10183303-10186752 | 1236 | 5/4 |
BnaA5.NRT1.5 | BnaA05g35790D | B | MF2 | 1269482-1274007 | 1845 | 6/5 |
BnaA9.NRT1.5 | BnaA09g24330D | B | LF | 17085147-17089456 | 1863 | 6/5 |
BnaC5.NRT1.5a | BnaC05g28620D | B | MF2 | 26898087-26903213 | 1845 | 6/5 |
BnaC5.NRT1.5b | BnaC05g24580D | B | LF | 19037761-19041900 | 1860 | 6/5 |
NRT1.8 | ||||||
BraA1.NRT1.8 | Bra013547 | U | LF | 6243271-6245298 | 1752 | 4/3 |
BraA3.NRT1.8 | Bra038763 | U | MF1 | 24230588-24232594 | 1752 | 4/3 |
BolC1.NRT1.8 | Bol028440 | U | LF | 8086050-8088075 | 1752 | 4/3 |
BolC6.NRT1.8 | Bol024295 | U | MF1 | 42081659-42082729 | 897 | 3/2 |
BnaA1.NRT1.8 | BnaA01g11510D | U | LF | 5715790-5718126 | 1752 | 4/3 |
BnaA3.NRT1.8 | BnaA03g44820D | U | MF1 | 22774854-22776862 | 1752 | 4/3 |
BnaC7.NRT1.8 | BnaC07g36810D | U | MF1 | 38737319-38739325 | 1752 | 4/3 |
BnaCn.NRT1.8 | BnaCnng78690D | U | LF | 80520183-80521816 | 1167 | 3/2 |
新窗口打开|下载CSV
Table 2
表2
表2白菜、甘蓝和甘蓝型油菜NRT1.5和NRT1.8蛋白的分子特性
Table 2
基因名 Gene name | 氨基酸数Amino acids | 主要氨基酸 Major amino acids | 碱性氨基酸Arg+Lys | 酸性氨基酸Asp+Glu | 分子量MW(kD) | 等电点pI | 不稳定系数Instability index | 亲水性GRAVY | 脂肪指数Aliphatic index |
---|---|---|---|---|---|---|---|---|---|
NRT1.5 | |||||||||
BraA5.NRT1.5 | 604 | Leu, Ser | 58 | 63 | 67.64 | 5.93 | 34.13 | 0.096 | 88.79 |
BraA9.NRT1.5 | 610 | Leu, Ser | 59 | 62 | 68.17 | 6.29 | 31.20 | 0.073 | 89.18 |
BolC5.NRT1.5a | 603 | Leu, Ser | 58 | 63 | 67.45 | 5.93 | 33.33 | 0.094 | 88.77 |
BolC5.NRT1.5b | 411 | Leu, Ser | 38 | 44 | 45.80 | 5.63 | 27.54 | -0.006 | 86.13 |
BnaA5.NRT1.5 | 614 | Leu, Ser | 59 | 64 | 68.72 | 5.93 | 34.82 | 0.094 | 88.62 |
BnaA9.NRT1.5 | 620 | Leu, Ser | 60 | 64 | 69.41 | 6.14 | 32.08 | 0.082 | 89.47 |
BnaC5.NRT1.5a | 614 | Leu, Ser | 59 | 64 | 68.71 | 5.93 | 34.68 | 0.094 | 88.45 |
BnaC5.NRT1.5b | 619 | Leu, Ser | 59 | 62 | 69.35 | 6.35 | 33.07 | 0.094 | 89.77 |
NRT1.8 | |||||||||
BraA1.NRT1.8 | 583 | Leu, Ser | 48 | 51 | 64.72 | 6.22 | 27.23 | 0.209 | 91.66 |
BraA3.NRT1.8 | 583 | Leu, Ala | 51 | 51 | 64.55 | 7.06 | 29.40 | 0.218 | 94.70 |
BolC1.NRT1.8 | 583 | Leu, Ser | 48 | 52 | 64.67 | 6.05 | 28.13 | 0.211 | 92.16 |
BolC6.NRT1.8 | 298 | Leu, Ala | 26 | 24 | 32.84 | 8.18 | 18.64 | 0.088 | 88.36 |
BnaA1.NRT1.8 | 583 | Leu, Ser | 48 | 51 | 64.60 | 6.22 | 27.84 | 0.211 | 91.17 |
BnaA3.NRT1.8 | 583 | Leu, Ala | 52 | 51 | 64.60 | 7.52 | 27.69 | 0.208 | 94.70 |
BnaC7.NRT1.8 | 583 | Leu, Ser | 53 | 50 | 64.67 | 8.18 | 27.88 | 0.207 | 94.85 |
BnaCn.NRT1.8 | 388 | Leu, Ser | 37 | 38 | 43.81 | 6.60 | 32.07 | 0.110 | 87.96 |
新窗口打开|下载CSV
NRT1.8蛋白在白菜、甘蓝和甘蓝型油菜中均由22种氨基酸组成。其中除了BraA3.NRT1.8、BolC6.NRT1.8和BnaA3.NRT1.8三种蛋白的Leu和Ala含量较高外, 其余五种蛋白均是Leu和Ser的含量较高。除BolC6.NRT1.8 (897 bp)和BnaCn.NRT1.8 (1167 bp)外, NRT1.8的基因编码区均为1752 bp, 编码583个氨基酸, 分子量分布在64.55 kD (BraA3. NRT1.8)~64.72 kD (BraA1.NRT1.8)之间。3个NRT1.8蛋白(BolC6.NRT1.8、BnaA3.NRT1.8和BnaC7.NRT1.8)中Arg+Lys>Asp+Glu, pI分别为8.18、7.52和8.18, 为碱性蛋白; BraA3.NRT1.8中Arg+Lys和Asp+Glu均为51, pI为7.06, 为中性蛋白; 其余4个NRT1.8蛋白中Asp+Glu>Arg+Lys, 为酸性蛋白。蛋白的不稳定性系数在18.64 (BolC6.NRT1.8)~ 32.07 (BnaCn.NRT1.8)之间, 为稳定性蛋白。GRAVY介于0.088 (BolC6.NRT1.8)~0.218 (BraA3.NRT1.8)之间, 为双亲和蛋白。脂肪指数在87.96 (BnaCn. NRT1.8)~94.85 (BnaC7.NRT1.8)之间。本研究中3种芸薹属作物NRT1.5和NRT1.8蛋白中Leu的含量均较高, 均为稳定的双亲和蛋白。
2.2 白菜、甘蓝、甘蓝型油菜及拟南芥NRT1.5和NRT1.8的基因拷贝数变异、染色体定位及基因结构分析
以拟南芥NRT1.5和NRT1.8的基因序列为基础序列, 对在油菜全基因组数据库中检索到的8个NRT1.5和8个NRT1.8同源基因的拷贝数变异、染色体定位及基因结构特征进行如下分析。拷贝数变异(copy number variations, CNVs)是由基因组发生重排所致。为了比较白菜、甘蓝及甘蓝型油菜NRT1.5和NRT1.8基因的进化多样性, 分别以AtNRT1.5和AtNRT1.8为查询对象, 在NCBI数据库中进行BLAST搜索。图1显示, 在异源四倍体甘蓝型油菜中鉴定了4个NRT1.5和4个NRT1.8同源基因, 明显多于拟南芥、甘蓝和白菜所具有的NRT1.5和NRT1.8基因数量。甘蓝型油菜中NRT1.5基因的数量与甘蓝和白菜中NRT1.5的总数相同, NRT1.8基因的数量等同于甘蓝和白菜中NRT1.8的总数, 这意味着甘蓝型油菜NRT1.5和NRT1.8基因在异源多倍体自然加倍过程中没有损失。
图1
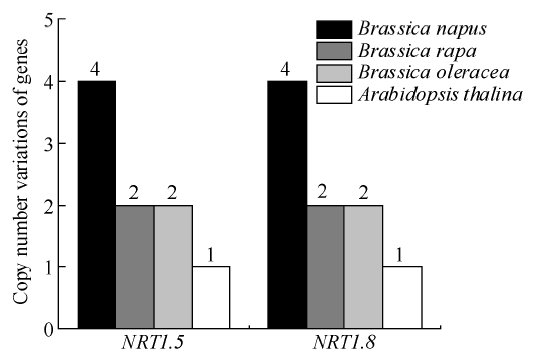
图1白菜、甘蓝、甘蓝型油菜及拟南芥NRT1.5和NRT1.8基因的拷贝数变异
柱状图顶部数值为该物种拷贝的基因数目。
Fig. 1Copy number variations of NRT1.5 and NRT1.8 genes in B. rapa, B. oleracea, B. napus, and A. thaliana
The number at the top of the histogram is the number of genes copied for that species.
利用MapInspect软件绘制出NRT1.5和NRT1.8基因在拟南芥、白菜、甘蓝及甘蓝型油菜染色体上的定位图(附图1)。表明NRT1.5和NRT1.8基因分布在不同的染色体上。NRT1.5基因在染色体上的分布是不均匀的, 每条染色体上有1~2个NRT1.5基因。Ar5、Ar9、An5和An9各分布1个; Co5和Cn5各分布2个NRT1.5串联重复基因。异源四倍体甘蓝型油菜中的A染色体全部来源于亲本二倍体白菜, C染色体全部来源于亲本二倍体甘蓝, 说明NRT1.5蛋白在自然加倍的进化过程中相对保守。与NRT1.5基因在染色体上的分布相比, NRT1.8基因在芸薹属作物染色体上的分布相对均匀, Ar1和Ar3、Co1和Co6以及An1、An3和Cn7每条染色体上各有一个NRT1.8基因。
附图1
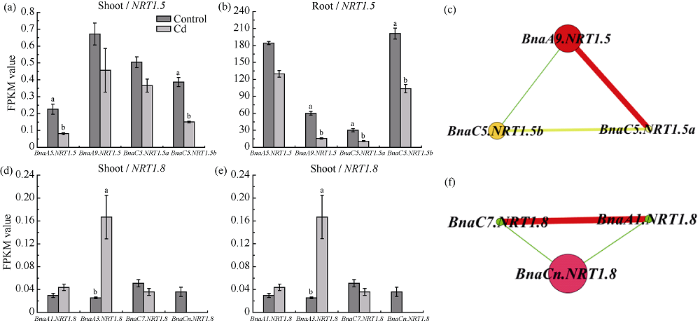
附图1NRT1.5和NRT1.8基因在白菜、甘蓝、甘蓝型油菜及拟南芥中的染色体定位
图a表示NRT1.5基因在拟南芥、甘蓝型油菜、甘蓝及白菜中的染色体定位;图b表示NRT1.8基因在拟南芥、甘蓝型油菜、甘蓝及白菜中的染色体定位。
Fig. 10Supplementary fig. 1 Chromosomal localization of NRT1.5 and NRT1.8 genes in B. rapa, B. oleracea, B. napus, and A. thaliana.
Fig. a shows the chromosomal localization of NRT1.5 genes in A. thaliana, B. napus, B. oleracea, and B. rapa. Fig. b shows the chromosomal localization of NRT1.8 genes in A. thaliana, B. napus, B. oleracea, and B. rapa.
外显子-内含子结构的数目是某些基因家族中典型的进化印记[37]。因此, 利用GSDS 2.0软件对芸薹属作物和拟南芥中NRT1.5和NRT1.8的基因结构进行分析(图2)。白菜和甘蓝的NRT1.5基因均有4个内含子, 甘蓝型油菜有5个内含子, 与拟南芥的基因结构类似。除BolC6.NRT1.8和BnaCn.NRT1.8有2个内含子外, 3种芸薹属作物NRT1.8基因均含3个内含子。同源基因中内含子个数的不同在一定程度上表明了即使是直系同源基因也有可能经历基因结构的多样化。
图2
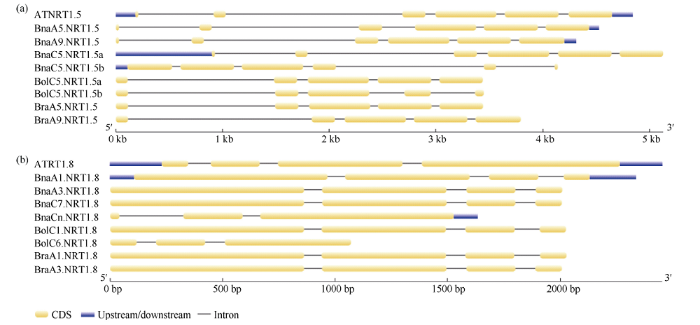
图2白菜、甘蓝、甘蓝型油菜及拟南芥NRT1.5和NRT1.8的基因结构特征
图a表示拟南芥、白菜、甘蓝及甘蓝型油菜NRT1.5的基因结构特征; 图b表示拟南芥、白菜、甘蓝及甘蓝型油菜NRT1.8的基因结构特征。其中, 黄色部分代表CDS序列, 蓝色部分代表上游基因和下游基因, 黑色细线代表基因的内含子。
Fig. 2Gene structure characteristics of NRT1.5 and NRT1.8 in B. rapa, B. oleracea, B. napus, and A. thaliana.
Fig. a and Fig. b show the gene structure characteristics of NRT1.5 and NRT1.8 respectively in A. thaliana, B. rapa, B. oleracea, and B. napus. The yellow parts represent the CDS sequence and the blue parts represent the upstream and downstream genes. The black lines represent the intron of genes.
2.3 不同物种NRT1.5和NRT1.8蛋白的系统进化分析
为了比较NRT1.5和NRT1.8蛋白在不同物种发育进化过程中的分子进化规律和系统发生关联, 分别对拟南芥、甘蓝型油菜、胡杨等5个双子叶物种和水稻、玉米、高粱等5个单子叶物种的32个NRT1.5和33个NRT1.8蛋白序列进行同源性比对, 利用邻接法构建系统进化树(图3)。图3所示, NRT1.5和NRT1.8家族成员均分为双子叶植物和单子叶植物两个分支, 表明NRT1.5和NRT1.8这两种蛋白均在生物形态发生后开始出现差异[36]。白菜、甘蓝和油菜中NRT1.5和NRT1.8两种蛋白均位于双子叶的分支, 且非常紧密地聚集在一起, 同源性较高。图3
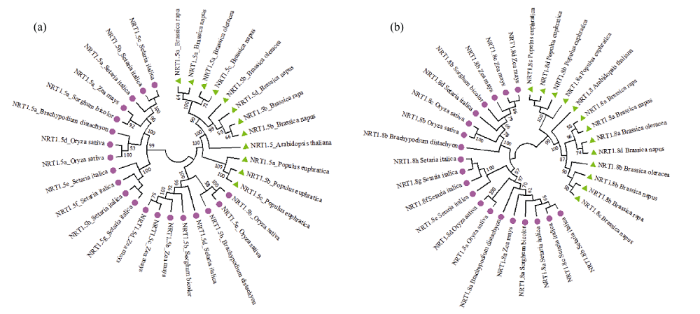
图3不同物种NRT1.5和NRT1.8蛋白的系统发育关系
图a表示在双子叶和单子叶植物中NRT1.5蛋白的系统发育关系; 图b表示在双子叶和单子叶植物中NRT1.8蛋白的系统发育关系。其中, 绿色部分代表双子叶植物, 包括拟南芥、白菜、甘蓝、甘蓝型油菜和胡杨, 红色部分代表单子叶植物, 包括高粱、水稻、小米、玉米和二穗短柄草。
Fig. 3Phylogenetic relationships of the NRT1.5 and NRT1.8 proteins in diverse species.
Fig.a and Fig.b show the phylogenetic relationships of NRT1.5 and NRT1.8 proteins respectively in dicots and monocots. The green parts represent the dicots, including Arabidopsis thaliana, Brassica rapa, Brassica oleracea, Brassica napus, and Populus euphratica. The red parts represent the monocots, including Sorghum bicolor, Oryza sativa, Setaria italica, Zea mays, and Brachypodium distachyon.
2.4 白菜、甘蓝及甘蓝型油菜NRT1.5和NRT1.8蛋白的进化选择压力分析
十字花科芸薹属作物全基因组可分为LF, MF1和MF2[38]3个亚基因组。表1显示, NRT1.5蛋白定位在B分区, 其中50%为LF亚基因组, 50%为MF2亚基因组。NRT1.8蛋白定位在U分区, 分为LF和MF1两个亚基因组。为了研究NRT1.5和NRT1.8蛋白在物种进化过程中是否受到选择压力, 分别对拟南芥和3种芸薹属作物NRT1.5和NRT1.8的CDS序列及蛋白序列进行比对, 分别计算Ka、Ks和Ka/Ks。在遗传学中认为, 用Ka/Ks可以判断这个基因在进化中是否受到选择压力。当Ka/Ks>1.0时, 认为有正选择效应; 当Ka/Ks=1.0时, 认为该蛋白存在中性选择; 当Ka/Ks<1.0时, 则认为存在纯化选择作用[39]。图4所示, NRT1.5和NRT1.8在3种芸薹属作物中的Ka/Ks值均小于1.0, 表明这两种蛋白在物种进化过程中保留其重要功能而存在纯化选择作用。
图4
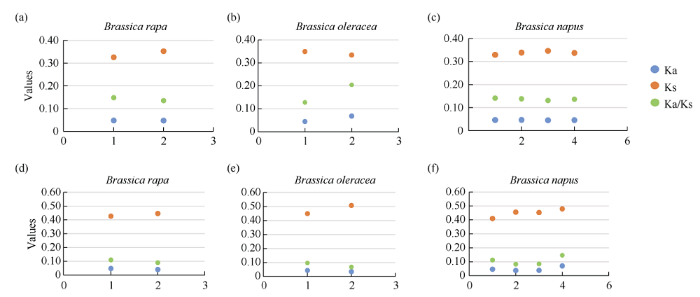
图4白菜、甘蓝及甘蓝型油菜中NRT1.5和NRT1.8蛋白的同义突变频率(Ks)和非同义突变频率(Ka)
图a, b, c分别表示白菜、甘蓝及甘蓝型油菜NRT1.5蛋白的同义突变频率和非同义突变频率; 图d, e, f分别表示白菜、甘蓝及甘蓝型油菜NRT1.8蛋白的同义突变频率和非同义突变频率。
Fig. 4Synonymous nucleotide substitution rates (Ks) and non-synonymous nucleotide substitution rates (Ka) of NRT1.5 and NRT1.8 proteins in B. rapa, B. oleracea, and B. napus
Fig. a, Fig. b, and Fig. c show synonymous nucleotide substitution rates and non-synonymous nucleotide substitution rates of NRT1.5 proteins in B. rapa, B. oleracea, and B. napus, respectively. Fig. d, Fig. e, and Fig. f show synonymous nucleotide substitution rates and non-synonymous nucleotide substitution rates of the NRT1.8 proteins in B. rapa, B. oleracea, and B. napus, respectively.
2.5 白菜、甘蓝、甘蓝型油菜及拟南芥NRT1.5和NRT1.8蛋白的保守基序分析
不同物种硝酸盐转运体的蛋白序列高度同源, 具有相似的保守序列。在拟南芥中, NRT1家族属于MFS (major facilitator superfamily)的小肽转运体PTR (peptide transporter)家族。PTR转运蛋白一般含有450~600个氨基酸和12个跨膜区。在第5跨膜区有一个PTR家族的保守序列(F-Y-x-x-x-N-x-G-S- L)[40]。对3种芸薹属作物及拟南芥NRT1.5和NRT1.8的氨基酸序列比对发现, 4个物种NRT1.5和NRT1.8蛋白的氨基酸序列相似性很高, 蛋白结构保守, 均含有PTR家族的保守基序(F-Y-L-A-L-N-L-G-S- L)(图5-a和图5-b蓝色虚线区域)。利用在线软件MEME对芸薹属作物及拟南芥NRT1.5和NRT1.8蛋白的保守基序进行分析, 不同颜色的盒子表示不同的保守基序, 灰色的线条表示没有检测到基序的蛋白质区域。图6-a显示, NRT1.5蛋白在4个物种中相对保守, 保守基序为10~15个。其中, 甘蓝型油菜和拟南芥中NRT1.5蛋白非常保守, 保守基序均为15个; BraA9.NRT1.5、BraA5.NRT1.5和BolC5.NRT1.5a缺失Motif 1, 含有14个保守基序; BolC5.NRT1.5b有10个保守基序, 缺失Motif 1、Motif 9、Motif 10、Motif 13及Motif 14。图6-b所示, 4个物种NRT1.8蛋白的保守基序为7~15个, 均含有保守基序Motif 6和Motif 7。其中BraA1.NRT1.8、BraA3.NRT1.8、BnaA1.NRT1.8、BnaA3.NRT1.8、BnaC7.NRT1.8及BolC1.NRT1.8的保守基序为15个; AtNRT1.8、BnaCn.NRT1.8和BolC6.NRT1.8保守基序分别为14、10和7个。从分子进化的角度看, 氨基酸残基在进化过程中的保守性, 可以认为其在功能或结构上具有重要性[41]。图5
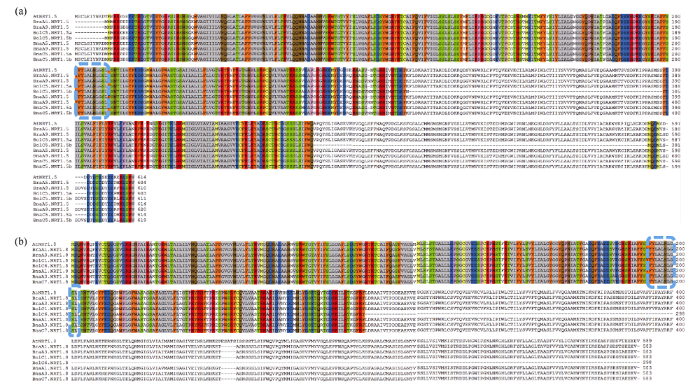
图5白菜、甘蓝、甘蓝型油菜及拟南芥NRT1.5和NRT1.8蛋白的保守序列比对
图a表示拟南芥、白菜、甘蓝及甘蓝型油菜NRT1.5蛋白的保守序列比对; 图b表示拟南芥、白菜、甘蓝及甘蓝型油菜NRT1.8蛋白的保守序列比对。其中, 蓝色虚线部分表示PTR家族的保守序列(F-Y-L-A-L-N-L-G-S-L)。
Fig. 5Conservative sequence alignment of NRT1.5 and NRT1.8 proteins in B. rapa, B. oleracea, B. napus, and A. thaliana.
Fig. a and Fig. b show the conservative sequence alignment of NRT1.5 and NRT1.8 proteins respectively in A. thaliana, B. rapa, B. oleracea, and B. napus. The blue parts show the conservative sequence (F-Y-L-A-L-N-L-G-S-L) of peptide transporter.
2.6 甘蓝型油菜NRT1.5和NRT1.8蛋白跨膜结构域分析
拟南芥NRT1家族的蛋白含12个跨膜区, 且在第6、7跨膜区之间有一个由约100个氨基酸组成的大亲水环相连, 将12个跨膜区分成两部分[42]。BnaNRT1.5和BnaNRT1.8家族蛋白的跨膜结构域如图7所示, 除了BnaCn.NRT1.8含有7个跨膜结构域外(图7-h), NRT1.5和NRT1.8均含有12个跨膜结构域, 在第6~7跨膜区间由一个大的亲水环相连, 与拟南芥中NRT1家族的结构特点相似。NRT1.5蛋白跨膜区主要分布在第41~580氨基酸残基间, 12个跨膜结构域长度为17~29个氨基酸。BnaA1.NRT1.8、BnaA3.NRT1.8和BnaC7.NRT1.8蛋白的跨膜区位于第35~565个氨基酸残基间, 跨膜结构域长度为17~27个氨基酸。BnaCn.NRT1.8蛋白的跨膜区分布在260~370氨基酸之间, 跨膜结构域长度为17~22个氨基酸。图6
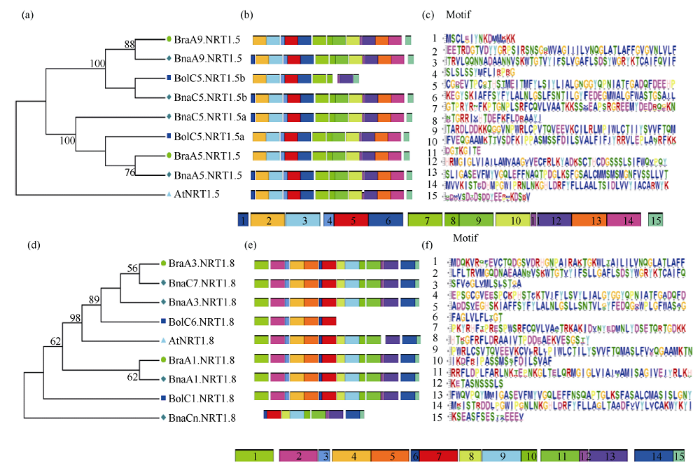
图6白菜、甘蓝、甘蓝型油菜及拟南芥NRT1.5和NRT1.8蛋白的保守基序特征
图a, d分别表示NRT1.5和NRT1.8蛋白在拟南芥、白菜、甘蓝及甘蓝型油菜中的系统进化关系; 图b, e分别表示NRT1.5和NRT1.8蛋白在拟南芥、白菜、甘蓝及甘蓝型油菜的保守基序; 图c, f分别表示NRT1.5和NRT1.8蛋白中每个保守基序的序列。
Fig. 6Characterization of conserved motifs in the NRT1.5 and NRT1.8 proteins in B. rapa, B. oleracea, B. napus, and A. thaliana.
Fig. a and Fig. d show the phylogenetic relationships of NRT1.5 and NRT1.8 proteins respectively in A. thaliana, B. rapa, B. oleracea, and B. napus. Fig. b and Fig. e show the motives of NRT1.5 and NRT1.8 proteins respectively in A. thaliana, B. rapa, B. oleracea, and B. napus. Fig. c and Fig. f show the sequence of conserved motif of NRT1.5 and NRT1.8 proteins.
图7
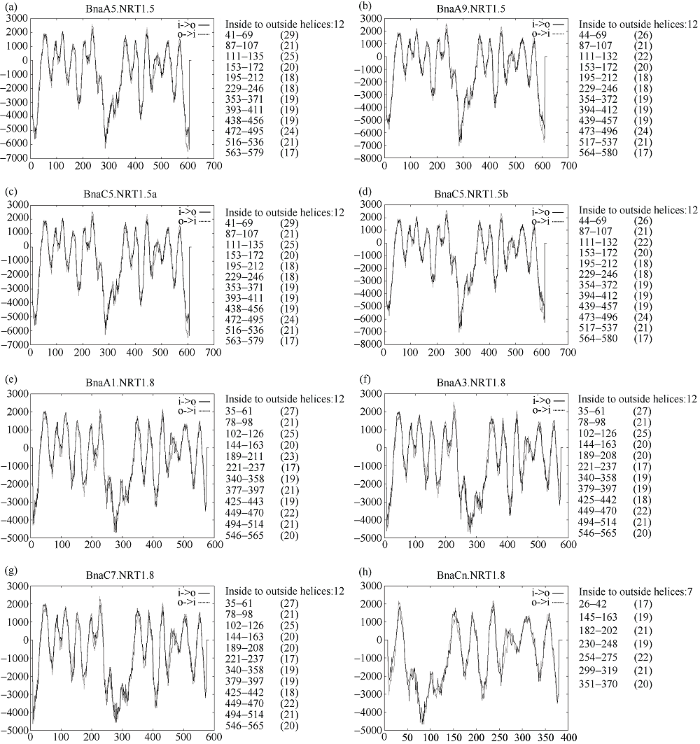
图7甘蓝型油菜NRT1.5和NRT1.8蛋白的跨膜结构域
图a~d表示甘蓝型油菜中4个NRT1.5蛋白的跨膜结构域, 其中a表示Bna.A5.NRT1.5, b表示Bna.A9.NRT1.5, c表示Bna.C5.NRT1.5a, d表示Bna.C5.NRT1.5b。图e~h表示甘蓝型油菜中4个NRT1.8蛋白的跨膜结构域, 其中a表示Bna.A1.NRT1.8, f表示Bna.A3.NRT1.8, g表示Bna.C7.NRT1.8, h表示Bna.Cn.NRT1.8。
Fig. 7Transmembrane domains of NRT1.5 and NRT1.8 proteins in B. napus
Fig. a to Fig. d show four transmembrane domains of NRT1.5 protein in B. napus. Fig. a, Fig. b, Fig. c, and Fig. d show the protein of Bna.A5.NRT1.5, Bna.A9.NRT1.5, Bna.C5.NRT1.5a, and Bna.C5.NRT1.5b, respectively. Fig. e to Fig. h show four transmembrane domains of NRT1.8 protein in B. napus. Fig. e, Fig.f, Fig. g, and Fig. h show the protein of Bna.A1.NRT1.8, Bna.A3.NRT1.8, Bna.C7.NRT1.8, and Bna.Cn.NRT1.8, respectively.
2.7 油菜NRT1.5和NRT1.8基因在氮-镉胁迫条件下基因表达丰度和基因共表达网络分析
硝酸盐不仅是重要的营养物质, 同时也是一种重要的信号分子, 对硝酸盐转运体发挥功能起重要的调控作用[43]。为了确定BnaNRT1.5和BnaNRT1.8对低氮胁迫是否存在响应, 对短期(3 h)和长期(72 h)低氮处理的甘蓝型油菜地上部和根进行了高通量转录组分析(图8)。表明低氮处理0~72 h后, 甘蓝型油菜中NRT1.5和NRT1.8基因在根部的表达均受到显著影响, 地上部差异不大。低氮处理后NRT1.5基因在根部的表达丰度上调, 且在72 h时达到最大值。其中, BnaA9.NRT1.5和BnaC5.NRT1.5a表达量在0~3 h内上调, 3~72 h内趋于平缓。BnaA5.NRT1.5和BnaC5.NRT1.5b表达量高, 且在0~72 h内持续上调。从甘蓝型油菜NRT1.5基因的共表达分析网络中可以看出, BnaA5.NRT1.5、BnaC5.NRT1.5a、BnaC5.NRT1.5b在响应低氮胁迫中均起到调控作用, 其中BnaA5.NRT1.5基因的调控占主导作用, 且与BnaC5.NRT1.5a的功能互作较强。低氮处理同样会影响BnaNRT1.8的表达。根部低氮处理0~72 h, 其基因表达量呈下调的趋势, 在0~3 h内急剧下调, 3~72 h内下调较缓慢, 在72 h表达量最低。其中, 低氮诱导相同的时间, BnaA3.NRT1.8、BnaC7.NRT1.8和BnaCn.NRT1.8的表达量均高于BnaA1.NRT1.8。基因共表达网络分析也表明, BnaA3.NRT1.8和BnaC7.NRT1.8在响应低氮胁迫中均起调控作用, 其中前者起主导作用。图8
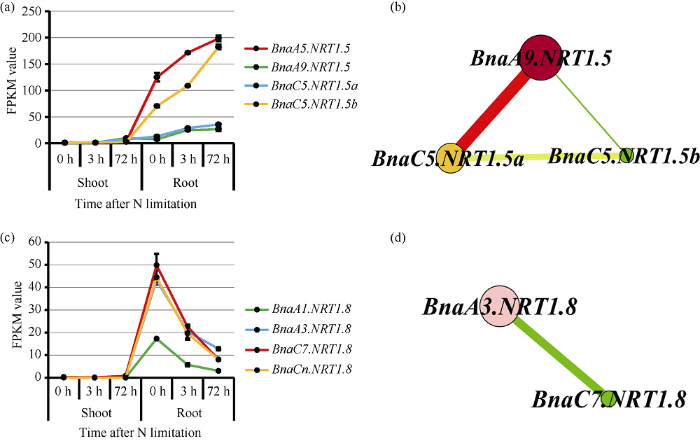
图8甘蓝型油菜NRT1.5和NRT1.8基因对低氮的响应及基因共表达网络分析
图a表示甘蓝型油菜地上部和根部NRT1.5家族基因在低氮处理0 h、3 h、72 h 时的基因表达丰度; 图b表示NRT1.5家族基因在低氮处理时的基因共表达网络分析。图c表示甘蓝型油菜地上部和根部NRT1.8家族基因在低氮处理0 h、3 h、72 h 时的基因表达丰度; 图d表示NRT1.8家族基因在低氮处理时的基因共表达网络分析; FPKM表示每千个碱基转录每百万映射读取的fragments。
Fig. 8Response to low concentration nitrate and co-expression network analysis of NRT1.5 and NRT1.8 genes in B. napus
Fig. a shows the gene expression abundance of NRT1.5 family genes in shoots and roots of B. napus with low nitrate treatment for 0 h, 3 h, 72 h. Fig. b shows the co-expression network analysis of NRT1.5 family genes with low nitrate treatment. Fig. c shows the gene expression abundance of NRT1.8 family genes in shoots and roots of B. napus with low nitrate treatment for 0 h, 3 h, 72 h. Fig. d shows the co-expression network analysis of NRT1.8 family genes with low nitrate treatment. FPKM means the fragments per kilobase of exon model per million mapped reads.
甘蓝型油菜湘油15号在10 μmol L-1的镉胁迫条件下, 根部和地上部BnaNRT1.5基因的表达均受到抑制(图9-a, b), 基因共表达网络分析表明(图9-c), BnaA9.NRT1.5、BnaC5.NRT1.5a和BnaC5.NRT1.5b在镉胁迫条件下均起到调控作用, 其中BnaA9. NRT1.5起主导调控作用。根部BnaNRT1.8在镉胁迫下受到显著诱导, BnaCn.NRT1.8、BnaA1.NRT1.8和BnaC7.NRT1.8在此过程中均起到调控作用, 其中BnaCn.NRT1.8起主要作用, BnaA1.NRT1.8和BnaC7.NRT1.8之间的功能互作较强(图9-d, e, f)。
图9
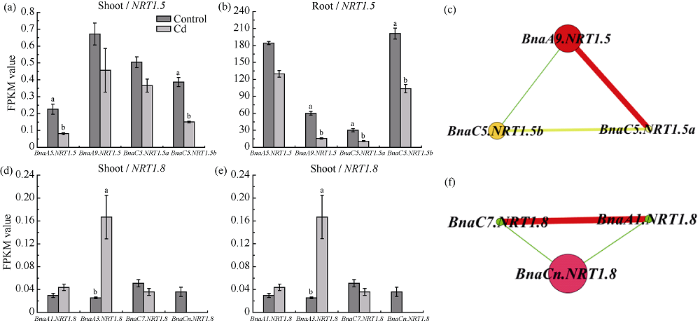
图9甘蓝型油菜NRT1.5和NRT1.8基因对镉胁迫的响应及基因共表达网络分析
图a和图b分别表示甘蓝型油菜地上部和根部NRT1.5家族基因在镉处理时的基因表达丰度; 图c表示NRT1.5家族基因在镉处理时的基因共表达网络分析。图d和图e分别表示甘蓝型油菜地上部和根部NRT1.8家族基因在镉处理时的基因表达丰度; 图f表示NRT1.8家族基因在镉处理时的基因共表达网络分析。FPKM表示每千个碱基转录每百万映射读取的fragments。图中所示显著性差异是单个基因对照和镉处理两两对比, 未达到显著性差异的图中未标记。误差线代
Fig. 9Response to cadmium stress and co-expression network analysis of NRT1.5 and NRT1.8 genes
Fig. a and Fig. b show the gene expression abundance of NRT1.5 family genes in shoots and roots of B. napus with cadmium stress. Fig. c shows the co-expression network analysis of NRT1.5 family genes with cadmium stress. Fig. d and Fig. e show the gene expression abundance of NRT1.8 family genes in shoots and roots of B. napus with cadmium stress. Fig. f shows the co-expression network analysis of NRT1.8 family genes with cadmium stress. FPKM means the fragments per kilobase of exon model per million mapped reads. The significant difference shown in the figure is the pairwise comparison between control and cadmium treatment, and the figure without significant difference is not marked. Data are shown as means±SE (n = 3) and the different lowercases above text are used to show statistical significance (Ρ < 0.05).
甘蓝型油菜根部NRT1.5和NRT1.8基因在低氮和镉胁迫条件下的表达量均受到不同程度的诱导。低氮胁迫条件下, NRT1.5基因的表达丰度上调抑制NRT1.8基因的表达, 使更多的硝酸盐从根部运输到地上部被作物同化和利用, 从而提高植物的氮素利用率。植物受到镉胁迫时, NRT1.8基因的表达上调抑制NRT1.5基因的表达, 从而使更多的硝酸盐运输到根部, 根部高浓度的硝酸盐有利于提高植物的胁迫防御能力。
3 讨论
植物吸收和转运硝酸盐需要硝酸盐转运体(NRTs)的协助完成。在拟南芥中, NRT1家族有53个成员, 目前鉴定有8个成员(NRT1.1、NRT1.2、NRT1.4~1.8)在硝酸盐吸收和转运中各自发挥着独特的作用[3,8,42,44-45], 其中NRT1.5和NRT1.8在调控硝酸盐长途转运及其根系和地上部的分配中起着重要的作用。白菜、甘蓝和甘蓝型油菜作为十字花科芸薹属中3种重要的经济作物, 对其NRT1.5和NRT1.8基因的结构功能及进化分析研究具有重要的实际意义。拟南芥NRT1家族属于MFS (major facilitator superfamily)超家族的小肽转运体PTR (peptide transporter)家族。PTR转运蛋白是一类可以转运氨基酸、寡肽以及硝酸盐等含氮化合物的膜转运蛋白, 广泛存在于动物、植物和微生物中[46], 一般含450~ 600个氨基酸, 12个跨膜区, 在第6、第7跨膜区之间由一个约100个氨基酸组成的亲水环相连, 在第5跨膜区有一个PTR家族的保守基序(F-Y-x-x-x-N- x-G-S-L)。3种芸薹属作物中大部分NRT1.5和NRT1.8蛋白含411~620个氨基酸, 跨膜结构域分析显示有12个跨膜区, 且在第6~7跨膜区间有一个亲水环。蛋白保守序列比对和保守基序分析发现, 2个蛋白均含有PTR家族的保守基序(F-Y-L-A-L-N-L- G-S-L), 与拟南芥NRT1家族基因相似。一般认为, 含有多个内含子的基因比较保守, 而不含有内含子的基因保守性较差[47,48]。3种芸薹属作物NRT1.5和NRT1.8基因结构含有2~5个内含子, 分别属于B分区不同亚类(LF、MF2)和U分区不同亚类(LF、MF1)。系统进化树和Ka/Ks表明蛋白在不同物种发育进化过程中的同源性和进化过程中是否受到选择压力[39]。芸薹属作物NRT1.5蛋白同源性较高, Ka/Ks值均小于1.0, 表明NRT1.5在3个物种进化过程中存在纯化选择作用且发挥相似的功能。NRT1.8蛋白Ka/Ks值均小于1.0, 但同源性较低, 表明NRT1.8在物种系统进化中受到纯化选择压力, 但在不同物种进化过程中其功能可能存在一定差异。
硝酸盐是氮素储存和运输的重要形式, 同时作为一种重要的信号物质对硝酸盐转运体起到重要的调控作用。植物通过硝酸盐转运体感知土壤中硝酸盐的浓度, 诱导硝酸盐转运体家族的不同成员发挥作用[43]。Wang等[49]指出, 在不同氮水平下小麦根系硝酸盐转运蛋白的不同成员基因表达存在差异, 从而使植株更有效地利用养分。此外, 硝酸盐转运体在植物胁迫防御方面发挥着重要作用。研究表明, 在逆境胁迫条件下, NRT1.5和NRT1.8通过调控硝酸盐在根部和地上部的分配从而影响植物对逆境胁迫的耐受能力。乙烯/茉莉酸-硝酸盐转运体介导的信号途径通过促进NRT1.8表达上调而抑制NRT1.5的表达, 从而使更多的硝酸盐从地上部运输到根部, 进而提高植物抗逆境胁迫的能力[10,11]。本研究中, 油菜湘油15号根部在低氮处理0~72 h时, NRT1.5基因的表达丰度显著上调, 而NRT1.8基 因的表达丰度呈现下调趋势, 由此可见, NRT1.5和 NRT1.8两个基因在响应低氮条件下诱导产生的基因表达模式相反, 但共同影响硝酸盐通过木质部向地上部的长途转运, 进而影响作物的产量和氮素利用率。在10 μmol L-1镉处理条件下, 甘蓝型油菜中NRT1.8表达上调抑制NRT1.5基因的表达, 这与Li等[10]研究的拟南芥NRT1.8基因对镉胁迫的响应一致。AtNRT1.8显著上调使更多的硝酸盐从地上部分运输到根部, 根部较高浓度的硝酸盐有利于根系适应镉胁迫。Li等[10]的研究也表明, 随着培养液中镉浓度的适当增加, atnrt1.8中的硝酸盐含量较野生型显著降低, 这说明在根部运输硝酸盐的过程中, AtNRT1.8基因的表达受镉的正向调控。基因共表达网络分析发现, 油菜在适应低氮胁迫时是BnaA5.NRT1.5和BnaA3. NRT1.8起主要调控作用, 镉胁迫时发挥主导作用的是BnaA9.NRT1.5和BnaCn.NRT1.8, 这可能是因为BnaNRT1.5和BnaNRT1.8基因对氮和镉胁迫的响应程度不同, 由此可以推断这2个基因家族在抵抗非生物胁迫过程中可能扮演着不同的角色, 但不可否认的是NRT1.5和NRT1.8基因在植物适应氮-镉胁迫方面发挥着重要的调控作用。因此, 深入全面地了解NRT1.5和NRT1.8的基因结构和进化关系对更好地发挥其功能具有重要的意义, 同时也为这2个家族基因在其他物种中的功能研究提供依据。
参考文献 原文顺序
文献年度倒序
文中引用次数倒序
被引期刊影响因子
URL [本文引用: 1]

硝酸根不仅是植物的主要氮源,而且作为重要的信号分子,在植物的生长发育及逆境响应过程中发挥了重要作用。本文重点介绍了硝酸根作为信号分子在基因表达,根系、叶片发育,种子休眠以及逆境响应调控中作用机理的最新研究进展。
URL [本文引用: 1]

硝酸根不仅是植物的主要氮源,而且作为重要的信号分子,在植物的生长发育及逆境响应过程中发挥了重要作用。本文重点介绍了硝酸根作为信号分子在基因表达,根系、叶片发育,种子休眠以及逆境响应调控中作用机理的最新研究进展。
[本文引用: 1]
DOI:10.1105/tpc.109.067603URLPMID:19734434 [本文引用: 2]

Several quantitative trait locus analyses have suggested that grain yield and nitrogen use efficiency are well correlated with nitrate storage capacity and efficient remobilization. This study of the Arabidopsis thaliana nitrate transporter NRT1.7 provides new insights into nitrate remobilization. Immunoblots, quantitative RT-PCR, beta-glucuronidase reporter analysis, and immunolocalization indicated that NRT1.7 is expressed in the phloem of the leaf minor vein and that its expression levels increase coincidentally with the source strength of the leaf. In nrt1.7 mutants, more nitrate was present in the older leaves, less (15)NO(3)(-) spotted on old leaves was remobilized into N-demanding tissues, and less nitrate was detected in the phloem exudates of old leaves. These data indicate that NRT1.7 is responsible for phloem loading of nitrate in the source leaf to allow nitrate transport out of older leaves and into younger leaves. Interestingly, nrt1.7 mutants showed growth retardation when external nitrogen was depleted. We conclude that (1) nitrate itself, in addition to organic forms of nitrogen, is remobilized, (2) nitrate remobilization is important to sustain vigorous growth during nitrogen deficiency, and (3) source-to-sink remobilization of nitrate is mediated by phloem.
DOI:10.1104/pp.106.085209URLPMID:17012411 [本文引用: 1]

The identification of a family of NAR2-type genes in higher plants showed that there was a homolog in Arabidopsis (Arabidopsis thaliana), AtNAR2.1. These genes encode part of a two-component nitrate high-affinity transport system (HATS). As the Arabidopsis NRT2 gene family of nitrate transporters has been characterized, we tested the idea that AtNAR2.1 and AtNRT2.1 are partners in a two-component HATS. Results using the yeast split-ubiquitin system and Xenopus oocyte expression showed that the two proteins interacted to give a functional HATS. The growth and nitrogen (N) physiology of two Arabidopsis gene knockout mutants, atnrt2.1-1 and atnar2.1-1, one for each partner protein, were compared. Both types of plants had lost HATS activity at 0.2 mM nitrate, but the effect was more severe in atnar2.1-1 plants. The relationship between plant N status and nitrate transporter expression revealed a pattern that was characteristic of N deficiency that was again stronger in atnar2.1-1. Plants resulting from a cross between both mutants (atnrt2.1-1 atnar2.1-1) showed a phenotype like that of the atnar2.1-1 mutant when grown in 0.5 mM nitrate. Lateral root assays also revealed growth differences between the two mutants, confirming that atnar2.1-1 had a stronger phenotype. To show that the impaired HATS did not result from the decreased expression of AtNRT2.1, we tested if constitutive root expression of a tobacco (Nicotiana plumbaginifolia) gene, NpNRT2.1, previously been shown to complement atnrt2.1-1, can restore HATS to the atnar2.1-1 mutant. These plants did not recover wild-type nitrate HATS. Taken together, these results show that AtNAR2.1 is essential for HATS of nitrate in Arabidopsis.
DOI:10.1111/j.1365-3040.2011.02396.xURLPMID:21736591 [本文引用: 1]

Cereals are major crops worldwide, and improvement of their nitrogen use efficiency is a crucial challenge. In this study proteins responding to N supply in barley roots and shoots were analysed using a proteomics approach, to provide insight into mechanisms of N uptake and assimilation. Control plants grown hydroponically for 33 d with 5 mm nitrate, plants grown under N deficiency (0.5 mm nitrate, 33 d) or short-term N starvation (28 d with 5 mm nitrate followed by 5 d with no N source) were compared. N deficiency caused changes in C and N metabolism and ascorbate-glutathione cycle enzymes in shoots and roots. N starvation altered proteins of amino acid metabolism in roots. Both treatments caused proteome changes in roots that could affect growth. Shoots of plants grown with ammonium as N source (28 d with 5 mm nitrate followed by 5 d with 5 mm ammonium) showed responses similar to N deficient shoots, characterized by turnover of ribulose 1路5-bisphosphate carboxylase/oxygenase (Rubisco) and increases in proteins of the chloroplastic transcription and translation machinery. Identified proteins in 67 and 49 varying spots in roots and shoots respectively, corresponded to 62 functions and over 80 gene products, considerably advancing knowledge of N responses in barley.
DOI:10.11674/zwyf.15357URL [本文引用: 1]

【目的】铵态氮(NH_4~+)和硝态氮(NO_3~–)是作物氮素吸收利用的主要形态,旱作作物NO_3~–的累积与利用是氮素营养研究的主要组成部分,关系到理解作物NO_3~–的转运和利用关系及作物体内NO_3~–含量和氮素利用效率(nitrogen utilization efficiency,NUE)高低的问题。【主要进展】作物吸收的NO_3~–分为被作物直接利用、分泌到根外、储存在液泡和向地上部分运输四种途径。其中NO_3~–短途分配(液泡NO_3~–分配)和长途转运(地上、地下部NO_3~–的转运)共同调控着NO_3~–的利用效率,进而影响作物的NUE。液泡NO_3~–不能被作物直接利用,只有分配到液泡外细胞质中的NO_3~–才能被作物迅速代谢和利用;同时有更大比例的NO_3–分配到地上部分,使得作物可以充分利用太阳光能进行NO_3–代谢和能量转换,从而提高了作物的NUE。此外,液泡对NO_3–起到分隔作用,储存在液泡中的NO_3–并不能对NO_3–转运相关基因(如NR、NO_3–长途转运基因NRT1.5和NRT1.8)起到诱导效果;只有分配在液泡外原生质体中的NO_3–才能对NO_3–诱导基因产生强烈的诱导。因此,作物细胞原生质体中液泡内、外NO_3–的分配不仅影响了NO_3–的同化利用,而且直接影响了NO_3–的长途转运。【展望】本文对植物原生质体中液泡内、外NO_3–的短途分配和地上、地下部间NO_3–的长途转运机制进行了总结,为进一步深入研究作物地上、地下部NO_3–长途转运和液泡NO_3–短途分配的关系,以及更好地揭示作物NUE对NO_3–转运和利用的响应机理提供参考。
DOI:10.11674/zwyf.15357URL [本文引用: 1]

【目的】铵态氮(NH_4~+)和硝态氮(NO_3~–)是作物氮素吸收利用的主要形态,旱作作物NO_3~–的累积与利用是氮素营养研究的主要组成部分,关系到理解作物NO_3~–的转运和利用关系及作物体内NO_3~–含量和氮素利用效率(nitrogen utilization efficiency,NUE)高低的问题。【主要进展】作物吸收的NO_3~–分为被作物直接利用、分泌到根外、储存在液泡和向地上部分运输四种途径。其中NO_3~–短途分配(液泡NO_3~–分配)和长途转运(地上、地下部NO_3~–的转运)共同调控着NO_3~–的利用效率,进而影响作物的NUE。液泡NO_3~–不能被作物直接利用,只有分配到液泡外细胞质中的NO_3~–才能被作物迅速代谢和利用;同时有更大比例的NO_3–分配到地上部分,使得作物可以充分利用太阳光能进行NO_3–代谢和能量转换,从而提高了作物的NUE。此外,液泡对NO_3–起到分隔作用,储存在液泡中的NO_3–并不能对NO_3–转运相关基因(如NR、NO_3–长途转运基因NRT1.5和NRT1.8)起到诱导效果;只有分配在液泡外原生质体中的NO_3–才能对NO_3–诱导基因产生强烈的诱导。因此,作物细胞原生质体中液泡内、外NO_3–的分配不仅影响了NO_3–的同化利用,而且直接影响了NO_3–的长途转运。【展望】本文对植物原生质体中液泡内、外NO_3–的短途分配和地上、地下部间NO_3–的长途转运机制进行了总结,为进一步深入研究作物地上、地下部NO_3–长途转运和液泡NO_3–短途分配的关系,以及更好地揭示作物NUE对NO_3–转运和利用的响应机理提供参考。
DOI:10.1093/jxb/erq409URLPMID:21193579 [本文引用: 1]

Under temperate climates and in cultivated soils, nitrate is the most important source of nitrogen (N) available for crops and, before its reduction and assimilation into amino acids, must enter the root cells and then move in the whole plant. The aim of this review is to provide an overall picture of the numerous membrane proteins that achieve these processes by being localized in different compartments and in different tissues. Nitrate transporters (NRT) from the NRT1 and NRT2 families ensure the capacity of root cells to take up nitrate, through high- and low-affinity systems (HATS and LATS) depending on nitrate concentrations in the soil solution. Other members of the NRT1 family are involved subsequently in loading and unloading of nitrate to and from the xylem vessels, allowing its distribution to aerial organs or its remobilization from old leaves. Once in the cell, nitrate can be stored in the vacuole by passing through the tonoplast, a step that involves chloride channels (CLC) or a NRT2 member. Finally, with the exception of one NRT1 member, the transport of nitrite towards the chloroplast is still largely unknown. All these fluxes are controlled by key factors, the 0900major tour operators0964 like the internal nutritional status of the plant but also by external abiotic factors.
DOI:10.1105/tpc.108.060244URLPMID:18780802 [本文引用: 3]

Abstract Little is known about the molecular and regulatory mechanisms of long-distance nitrate transport in higher plants. NRT1.5 is one of the 53 Arabidopsis thaliana nitrate transporter NRT1 (Peptide Transporter PTR) genes, of which two members, NRT1.1 (CHL1 for Chlorate resistant 1) and NRT1.2, have been shown to be involved in nitrate uptake. Functional analysis of cRNA-injected Xenopus laevis oocytes showed that NRT1.5 is a low-affinity, pH-dependent bidirectional nitrate transporter. Subcellular localization in plant protoplasts and in planta promoter-beta-glucuronidase analysis, as well as in situ hybridization, showed that NRT1.5 is located in the plasma membrane and is expressed in root pericycle cells close to the xylem. Knockdown or knockout mutations of NRT1.5 reduced the amount of nitrate transported from the root to the shoot, suggesting that NRT1.5 participates in root xylem loading of nitrate. However, root-to-shoot nitrate transport was not completely eliminated in the NRT1.5 knockout mutant, and reduction of NRT1.5 in the nrt1.1 background did not affect root-to-shoot nitrate transport. These data suggest that, in addition to that involving NRT1.5, another mechanism is responsible for xylem loading of nitrate. Further analyses of the nrt1.5 mutants revealed a regulatory loop between nitrate and potassium at the xylem transport step.
DOI:10.1104/pp.112.199257URLPMID:22685171 [本文引用: 1]

Nitrate reallocation to plant roots occurs frequently under adverse conditions and was recently characterized to be actively regulated by Nitrate Transporter1.8 (NRT1.8) in Arabidopsis (Arabidopsis thaliana) and implicated as a common response to stresses. However, the underlying mechanisms remain largely to be determined. In this study, characterization of NRT1.5, a xylem nitrate-loading transporter, showed that the mRNA level of NRT1.5 is down-regulated by salt, drought, and cadmium treatments. Functional disruption of NRT1.5 enhanced tolerance to salt, drought, and cadmium stresses. Further analyses showed that nitrate, as well as Na + and Cd 2+ levels, were significantly increased in nrt1.5 roots. Important genes including Na + /H + exchanger1, Salt overly sensitivel, Pyrroline-5-carboxylate synthase1, Responsive to desiccation29A, Phytochelatin synthase1, and NRT1.8 in stress response pathways are steadily up-regulated in nrt1.5 mutant plants. Interestingly, altered accumulation of metabolites, including proline and malondialdehyde, was also observed in nrt1.5 plants. These data suggest that NRT1.5 is involved in nitrate allocation to roots and the consequent tolerance to several stresses, in a mechanism probably shared with NRT1.8.
DOI:10.1105/tpc.110.075242URLPMID:20501909 [本文引用: 6]

Long-distance transport of nitrate requires xylem loading and unloading, a successive process that determines nitrate distribution and subsequent assimilation efficiency. Here, we report the functional characterization of NRT1.8, a member of the nitrate transporter (NRT1) family in Arabidopsis thaliana. NRT1.8 is upregulated by nitrate. Histochemical analysis using promoter-β-glucuronidase fusions, as well as in situ hybridization, showed that NRT1.8 is expressed predominantly in xylem parenchyma cells within the vasculature. Transient expression of the NRT1.8:enhanced green fluorescent protein fusion in onion epidermal cells and Arabidopsis protoplasts indicated that NRT1.8 is plasma membrane localized. Electrophysiological and nitrate uptake analyses using Xenopus laevis oocytes showed that NRT1.8 mediates low-affinity nitrate uptake. Functional disruption of NRT1.8 significantly increased the nitrate concentration in xylem sap. These data together suggest that NRT1.8 functions to remove nitrate from xylem vessels. Interestingly, NRT1.8 was the only nitrate assimilatory pathway gene that was strongly upregulated by cadmium (Cd0562) stress in roots, and the nrt1.8-1 mutant showed a nitrate-dependent Cd0562-sensitive phenotype. Further analyses showed that Cd0562 stress increases the proportion of nitrate allocated to wild-type roots compared with the nrt1.8-1 mutant. These data suggest that NRT1.8-regulated nitrate distribution plays an important role in Cd0562 tolerance.
DOI:10.1105/tpc.114.129296URLPMID:25326291 [本文引用: 2]

Abstract Stresses decouple nitrate assimilation and photosynthesis through stress-initiated nitrate allocation to roots (SINAR), which is mediated by the nitrate transporters NRT1.8 and NRT1.5 and functions to promote stress tolerance. However, how SINAR communicates with the environment remains unknown. Here, we present biochemical and genetic evidence demonstrating that in Arabidopsis thaliana, ethylene (ET) and jasmonic acid (JA) affect the crosstalk between SINAR and the environment. Electrophoretic mobility shift assays and chromatin immunoprecipitation assays showed that ethylene response factors (ERFs), including OCTADECANOID-RESPONSIVE ARABIDOPSIS AP2/ERF59, bind to the GCC boxes in the NRT1.8 promoter region, while ETHYLENE INSENSITIVE3 (EIN3) binds to the EIN3 binding site motifs in the NRT1.5 promoter. Genetic assays showed that cadmium and sodium stresses initiated ET/JA signaling, which converged at EIN3/EIN3-Like1 (EIL1) to modulate ERF expression and hence to upregulate NRT1.8. By contrast, ET and JA signaling mediated the downregulation of NRT1.5 via EIN3/EIL1 and other, unknown component(s). SINAR enhanced stress tolerance and decreased plant growth under nonstressed conditions through the ET/JA-NRT1.5/NRT1.8 signaling module. Interestingly, when nitrate reductase was impaired, SINAR failed to affect either stress tolerance or plant growth. These data suggest that SINAR responds to environmental conditions through the ET/JA-NRT signaling module, which further modulates stress tolerance and plant growth in a nitrate reductase-dependent manner. 2014 American Society of Plant Biologists. All rights reserved.
DOI:10.1016/j.tplants.2013.08.008URLPMID:24055139 [本文引用: 1]

Members of the plant NITRATE TRANSPORTER 1/PEPTIDE TRANSPORTER (NRT1/PTR) family display protein sequence homology with the SLC15/PepT/PTR/POT family of peptide transporters in animals. In comparison to their animal and bacterial counterparts, these plant proteins transport a wide variety of substrates: nitrate, peptides, amino acids, dicarboxylates, glucosinolates, IAA, and ABA. The phylogenetic relationship of the members of the NRT1/PTR family in 31 fully sequenced plant genomes allowed the identification of unambiguous clades, defining eight subfamilies. The phylogenetic tree was used to determine a unified nomenclature of this family named NPF, for NRT1/PTR FAMILY. We propose that the members should be named accordingly: NPFX.Y, where X denotes the subfamily and Y the individual member within the species.
DOI:10.3969/J.ISSN.1000-5137.2017.05.019URL [本文引用: 1]

植物硝酸盐转运蛋白不仅能够吸收、运转硝态氮,而且在植物其他生理过程中也发挥 重要作用.重点介绍了硝酸盐转运蛋白在氮素吸收运转、硝酸盐积累、侧根发育、激素运转以及 逆境响应调控等方面的最新研究进展,并概括了硝酸盐转运蛋白的表达调控模式.
DOI:10.3969/J.ISSN.1000-5137.2017.05.019URL [本文引用: 1]

植物硝酸盐转运蛋白不仅能够吸收、运转硝态氮,而且在植物其他生理过程中也发挥 重要作用.重点介绍了硝酸盐转运蛋白在氮素吸收运转、硝酸盐积累、侧根发育、激素运转以及 逆境响应调控等方面的最新研究进展,并概括了硝酸盐转运蛋白的表达调控模式.
DOI:10.3969/j.issn.1008-0864.2010.03.03URL [本文引用: 1]

目前,我国食用植物油供给形势严峻,国内油料作物仅提供植物油消费总量的40.6%,对国外的依存度越来越高,安全隐患日益严重。菜籽油是国产食用植物油的第一大来源,在我国食用油市场中具有举足轻重的地位,目前已占我国油料作物产油量的57.2%。因此,在不与粮争地的前提下,大力挖掘油菜生产潜力,是提高油菜产量和保障我国食用植物油供给安全的核心举措。概述了我国油菜生产现状,分析了我国油菜在扩大面积、提高单产、提高含油量三个方面的生产潜力,并在此基础上提出了挖掘油菜生产潜力的有效途径是科技创新和政策保障。
DOI:10.3969/j.issn.1008-0864.2010.03.03URL [本文引用: 1]

目前,我国食用植物油供给形势严峻,国内油料作物仅提供植物油消费总量的40.6%,对国外的依存度越来越高,安全隐患日益严重。菜籽油是国产食用植物油的第一大来源,在我国食用油市场中具有举足轻重的地位,目前已占我国油料作物产油量的57.2%。因此,在不与粮争地的前提下,大力挖掘油菜生产潜力,是提高油菜产量和保障我国食用植物油供给安全的核心举措。概述了我国油菜生产现状,分析了我国油菜在扩大面积、提高单产、提高含油量三个方面的生产潜力,并在此基础上提出了挖掘油菜生产潜力的有效途径是科技创新和政策保障。
DOI:10.4141/cjps2011-002URL [本文引用: 1]

Increased demand for biodiesel feedstock has encouraged greater napus canola (Brassica napus L.) production, but there may be a need for greater production of other oilseed crops for this purpose. A multi-site field study was conducted to determine the oil yield potential of various crops relative to that of napus canola in the semi-arid, short-season environment of the Canadian prairies. Oilse...
[本文引用: 1]
[本文引用: 1]
DOI:10.1111/pbi.12742URLPMID:5698052 [本文引用: 1]

As an increasing number of plant genome sequences become available, it is clear that gene content varies between individuals, and the challenge arises to predict the gene content of a species. However, genome comparison is often confounded by variation in assembly and annotation. Differentiating between true gene absence and variation in assembly or annotation is essential for the accurate identification of conserved and variable genes in a species. Here, we present thede novoassembly of theB.02napuscultivar Tapidor and comparison with an improved assembly of theBrassica02napuscultivar Darmor‐bzh. Both cultivars were annotated using the same method to allow comparison of gene content. We identified genes unique to each cultivar and differentiate these from artefacts due to variation in the assembly and annotation. We demonstrate that using a common annotation pipeline can result in different gene predictions, even for closely related cultivars, and repeat regions which collapse during assembly impact whole genome comparison. After accounting for differences in assembly and annotation, we demonstrate that the genome of Darmor‐bzhcontains a greater number of genes than the genome of Tapidor. Our results are the first step towards comparison of the true differences betweenB.02napusgenomes and highlight the potential sources of error in future production of aB.02napuspangenome.
[本文引用: 1]
DOI:10.7606/j.issn.1009-1041.2014.08.01 [本文引用: 1]

硝酸盐转运蛋白NRT1(Nitrate transporter 1)和NRT2(Nitrate transporter 2)家族基因在高等植物氮转运过程中发挥着重要作用。为探讨NRT1和NRT2家族基因在小麦幼苗响应氮饥饿中的作用,本研究测定了氮饥饿处理过程中小麦幼苗的生长参数和叶片中叶绿素、可溶性蛋白、硝酸盐和丙二醛(MDA)的含量,并利用荧光实时定量PCR(qPCR)技术分析了叶片中NRT1(7个)和NRT2(5个)家族基因的转录水平。结果表明,氮饥饿明显抑制小麦幼苗生长,其株高、干鲜重显著下降,叶片叶绿素含量、蛋白质含量和硝酸盐含量均显著降低,而MDA含量却显著升高。在氮饥饿处理后所有测定时间点(2d、4d、6d和8d),小麦幼苗叶片中TaNRT1.1、TaNRT1.2、TaNRT1.7、TaNRT2.3和TaNRT2.4的表达均受到显著抑制;然而,氮饥饿2d时,TaNRT1.4、TaNRT1.8和TaNRT2.1的表达量显著升高;氮饥饿4d时,TaNRT1.3、TaNRT1.5和TaNRT2.5的表达量也显著升高,推测TaNRT1.3、TaNRT1.4、TaNRT1.5、TaNRT1.8、TaNRT2.1和TaNRT2.5可能在小麦幼苗响应氮饥饿中发挥着重要作用。
DOI:10.7606/j.issn.1009-1041.2014.08.01 [本文引用: 1]

硝酸盐转运蛋白NRT1(Nitrate transporter 1)和NRT2(Nitrate transporter 2)家族基因在高等植物氮转运过程中发挥着重要作用。为探讨NRT1和NRT2家族基因在小麦幼苗响应氮饥饿中的作用,本研究测定了氮饥饿处理过程中小麦幼苗的生长参数和叶片中叶绿素、可溶性蛋白、硝酸盐和丙二醛(MDA)的含量,并利用荧光实时定量PCR(qPCR)技术分析了叶片中NRT1(7个)和NRT2(5个)家族基因的转录水平。结果表明,氮饥饿明显抑制小麦幼苗生长,其株高、干鲜重显著下降,叶片叶绿素含量、蛋白质含量和硝酸盐含量均显著降低,而MDA含量却显著升高。在氮饥饿处理后所有测定时间点(2d、4d、6d和8d),小麦幼苗叶片中TaNRT1.1、TaNRT1.2、TaNRT1.7、TaNRT2.3和TaNRT2.4的表达均受到显著抑制;然而,氮饥饿2d时,TaNRT1.4、TaNRT1.8和TaNRT2.1的表达量显著升高;氮饥饿4d时,TaNRT1.3、TaNRT1.5和TaNRT2.5的表达量也显著升高,推测TaNRT1.3、TaNRT1.4、TaNRT1.5、TaNRT1.8、TaNRT2.1和TaNRT2.5可能在小麦幼苗响应氮饥饿中发挥着重要作用。
DOI:10.7666/d.Y2038161URL [本文引用: 1]

氮素(N)是农作物产量的重要限制因子,铵态氮(NH4+)和硝态氮(N03-)是植物从土壤中吸收的主要矿质氮源。水稻(Oryza sativa L.)不仅是世界上主要的粮食作物之一,而且是单子叶作物研究的重要模式植物。在淹水条件下,水稻根系实际上处于铵硝混合营养中,越来越多的人关注N03-的研究,发现N03-对水稻的生长发育有重要的影响作用。在模式植物拟南芥中,研究发现CHL1、AtNRT1.2的功能是与硝酸盐吸收有关的。AtNRT1.4参与硝酸盐在叶柄的储存,AtNRT1.7促进了硝酸盐从老叶向嫩叶的转运和再利用,AtNRT1.6促进了硝酸盐向种子转运,AtNRT1.5参与了硝酸盐通过木质部从根系向地上部的长距离运输。然而关于水稻是如何吸收硝态氮生理与分子机制这方面报道还很少。 本研究从水稻基因组中找到AtNRT1.2、AtNRT1.5的同源基因,命名为:OsNRT1.2、OsNRT1.5,利用农杆菌介导转化水稻武运粳7号和日本晴,对OsNRT1.2、OsNRT1.5进行获得性功能验证,构建了OsNRT1.2、OsNRT1.5基因的超量表达、RNAi基因沉默、MicroRNAs、蛙卵异源表达载体,转化水稻武运粳7号、日本晴和蛙卵;选取OsNRT1.2的武运粳7号超量表达株系W1.2-OE1、W1.2-OE2、W1.2-OE8;日本晴转基因株系1.2-OE1、1.2-OE5、1.2-OE6及OsNRT1.5的武运粳7号超量表达株系W1.5-OE2、W1.5-OE7、W1.5-OE8;日本晴转基因株系1.5-OE1、1.5-OE9、1.5-OE16,以野生型武运粳7号(WWT)、日本晴(WT)作为对照,进一步研究了基因OsNRT1.2和OsNRT1.5的表达调控和功能。主要研究结果为: 1)通过生物信息学预测,OsNRT1.2序列全长为2178 bp,编码阅读框为1781 bp,编码593个氨基酸;OsNRT1.5全长为2240 bp,编码阅读框为1833 bp,编码610个氨基酸。通过氨基酸序列预测,OsNRT1.2和OsNRT1.5可能是硝酸盐运输蛋白,含有12次跨膜域,以及一个由24个氨基酸组成的中心环。OsNRT1.2和OsNRT1.5均由4个外显子和3个内含子,组织定位可能在细胞质膜上。 2)半定量RT-PCR检测了OsNRT1.2和OsNRT1.5的时空表达模式。结果表明,OsNRT1.2、OsNRT1.5在武运粳7号、日本晴,表达存在器官特异性;OsNRT1.2主要在根系表达,地上部分几乎不表达。OsNRT1.5主要在地上部分表达,根系表达很少。 3)采用农杆菌介导转化水稻分别超量表达OsNRT1.2和OsNRT1.5。分析检测结果表明:与野生型相比,在mRNA水平OsNRT1.2、OsNRT1.5在武运粳7号、日本晴超量转基因材料表达均上调并且在不同氮素水平处理下无显著差异;在转基因植株地上部分和根系,低亲和组成型OsNRT1.1和高亲和OsNRT2.1、OsNRT2.2的表达均没有变化。 4) OsNRT1.2武运粳7号超量表达植株在水培试验中,用5.0 mM NO3-处理,株高与WWT有显著差异(除W1.2-OE2外),比WWT增加了3.1~3.7 cm;根长、根系全氮含量显著降低,W1.2-OE1、W1.2-OE2、W1.2-OE8分别比WWT减少了3.3、2.5、4.5 cm和11.3%、10.9%、14.6%;氮吸收量比WWT分别增加了39.5%、33.9%、97.8%; W1.2-OE1、W1.2-OE2与WWT地上部分全氮含量没有显著差异,而W1.2-OE8是WWT的1.12倍。在0.2 mM NO3-供氮水平上,转基因植株与WWT的株高、根长(除W1.2-OE1外)、地上部分及根系的氮吸收量、全氮含量均无显著差异。在0.2 mMNO3-、5.0mM NO3-供氮水平上,转基因植株比WWT的生物量分别增加9.4%~31.1%和12.5%~43.8%。在缺氮的田间试验中,转基因植株地上部干重、根系干重比WWT,分别显著增加35.4%~57.2%和79.3%~120.9%。OsNRT1.2在武运粳7号过量表达可能受高浓度硝酸盐影响,促进植株对硝酸盐的吸收、生物量增加和根系生长。 5) OsNRT1.2日本晴超量表达植株水培试验中,在0.2mM NH4+、5.0mM NH4+、0.2mM NO3-、5.0mM NO3-、2.5mM NH4NO3供氮水平上,与WT的根系长度变化无显著差异。在2.5 mM NH4NO3供氮水平上,WT、1.2-OE1、1.2-OE5、1.2-OE6株高分别增加了7.8 cm、20.2 cm、18.6 cm、27.2 cm; 1.2-OE1、1.2-OE5、1.2-OE6地上部分全氮含量比WT显著增加,分别增加了27%、18%、23%和15.6%、9.5%、19.2%;生物量分别是WT的1.9、1.3、2.2倍和1.8、1.7、2.7倍。在缺氮的田间试验中,转基因植株的株高、分蘖数、有效穗数、单株产量、千粒重、结实率、地上部分干重(除1.2-OE6外)、根系干重与WT无显著差异。 6) OsNRT1.5武运粳7号转基因植株水培试验中,在5.0 mM NH4+、2.5 mM NH4NO3供氮水平上处理30天,W1.5-OE2、W1.5-OE7、W1.5-OE8干物质量比WWT分别增加了54.8%、74.6%、36.7%和68.5%、24.8%、31.5%;生长中期在5.0 mM NO3-、2.5 mM NH4NO3、5.0 mM NH4+供氮时,比野生型先分蘖,且根系粗壮。在缺氮的田间试验中,与WWT相比,武运粳7号转基因植株的单株产量(除W1.5-OE7外)、结实率、单穗粒数显著增加;株高、分蘖数(除W1.5-OE2外)、有效穗数(除W1.5-OE2外)、千粒重无显著差异。转基因植株提前分蘖,从而导致生育期提前,比野生型早成熟。OsNRT1.5在武运粳7号过量表达可能促使分蘖期提前,促进植株根系生长,从而促进植株生长、单株产量增加。OsNRTl.5在武运粳7号过量表达可能促进了氮素向籽粒的转运。 7)OsNRT1.5日本晴转基因植株水培试验中,在5.0 mM NH4+供氮水平上,1.5-OE9比WT地上部分全氮含量显著增加了13.3%;在2.5 mM NH4NO3供氮水平上,1.5-OE1、1.5-OE9、1.5-OE16比WT地上部分全氮含量显著增加了14.5%-38.2%;在5.0 mM NH4+、2.5 mM NH4NO3供氮水平上,1.5-OE1、1.5-OE9干物质量显著增加,分别是WT的1.3、1.2、倍和1.9、2.1倍;在0.2 mM NO3-、5.0 mM NO3-、0.2 mM NH4+供氮水平上,地上部分全氮含量与WT无显著差异。在缺氮的田间试验中,转基因植株株高显著降低(除1.5-OE9外),结实率和单穗粒数显著增加(除1.5-OE16外),单株产量、分蘖数(除1.5-OE1外)、有效穗数、千粒重、地上部分干重(除1.5-OE9外)没有显著差异。
DOI:10.7666/d.Y2038161URL [本文引用: 1]

氮素(N)是农作物产量的重要限制因子,铵态氮(NH4+)和硝态氮(N03-)是植物从土壤中吸收的主要矿质氮源。水稻(Oryza sativa L.)不仅是世界上主要的粮食作物之一,而且是单子叶作物研究的重要模式植物。在淹水条件下,水稻根系实际上处于铵硝混合营养中,越来越多的人关注N03-的研究,发现N03-对水稻的生长发育有重要的影响作用。在模式植物拟南芥中,研究发现CHL1、AtNRT1.2的功能是与硝酸盐吸收有关的。AtNRT1.4参与硝酸盐在叶柄的储存,AtNRT1.7促进了硝酸盐从老叶向嫩叶的转运和再利用,AtNRT1.6促进了硝酸盐向种子转运,AtNRT1.5参与了硝酸盐通过木质部从根系向地上部的长距离运输。然而关于水稻是如何吸收硝态氮生理与分子机制这方面报道还很少。 本研究从水稻基因组中找到AtNRT1.2、AtNRT1.5的同源基因,命名为:OsNRT1.2、OsNRT1.5,利用农杆菌介导转化水稻武运粳7号和日本晴,对OsNRT1.2、OsNRT1.5进行获得性功能验证,构建了OsNRT1.2、OsNRT1.5基因的超量表达、RNAi基因沉默、MicroRNAs、蛙卵异源表达载体,转化水稻武运粳7号、日本晴和蛙卵;选取OsNRT1.2的武运粳7号超量表达株系W1.2-OE1、W1.2-OE2、W1.2-OE8;日本晴转基因株系1.2-OE1、1.2-OE5、1.2-OE6及OsNRT1.5的武运粳7号超量表达株系W1.5-OE2、W1.5-OE7、W1.5-OE8;日本晴转基因株系1.5-OE1、1.5-OE9、1.5-OE16,以野生型武运粳7号(WWT)、日本晴(WT)作为对照,进一步研究了基因OsNRT1.2和OsNRT1.5的表达调控和功能。主要研究结果为: 1)通过生物信息学预测,OsNRT1.2序列全长为2178 bp,编码阅读框为1781 bp,编码593个氨基酸;OsNRT1.5全长为2240 bp,编码阅读框为1833 bp,编码610个氨基酸。通过氨基酸序列预测,OsNRT1.2和OsNRT1.5可能是硝酸盐运输蛋白,含有12次跨膜域,以及一个由24个氨基酸组成的中心环。OsNRT1.2和OsNRT1.5均由4个外显子和3个内含子,组织定位可能在细胞质膜上。 2)半定量RT-PCR检测了OsNRT1.2和OsNRT1.5的时空表达模式。结果表明,OsNRT1.2、OsNRT1.5在武运粳7号、日本晴,表达存在器官特异性;OsNRT1.2主要在根系表达,地上部分几乎不表达。OsNRT1.5主要在地上部分表达,根系表达很少。 3)采用农杆菌介导转化水稻分别超量表达OsNRT1.2和OsNRT1.5。分析检测结果表明:与野生型相比,在mRNA水平OsNRT1.2、OsNRT1.5在武运粳7号、日本晴超量转基因材料表达均上调并且在不同氮素水平处理下无显著差异;在转基因植株地上部分和根系,低亲和组成型OsNRT1.1和高亲和OsNRT2.1、OsNRT2.2的表达均没有变化。 4) OsNRT1.2武运粳7号超量表达植株在水培试验中,用5.0 mM NO3-处理,株高与WWT有显著差异(除W1.2-OE2外),比WWT增加了3.1~3.7 cm;根长、根系全氮含量显著降低,W1.2-OE1、W1.2-OE2、W1.2-OE8分别比WWT减少了3.3、2.5、4.5 cm和11.3%、10.9%、14.6%;氮吸收量比WWT分别增加了39.5%、33.9%、97.8%; W1.2-OE1、W1.2-OE2与WWT地上部分全氮含量没有显著差异,而W1.2-OE8是WWT的1.12倍。在0.2 mM NO3-供氮水平上,转基因植株与WWT的株高、根长(除W1.2-OE1外)、地上部分及根系的氮吸收量、全氮含量均无显著差异。在0.2 mMNO3-、5.0mM NO3-供氮水平上,转基因植株比WWT的生物量分别增加9.4%~31.1%和12.5%~43.8%。在缺氮的田间试验中,转基因植株地上部干重、根系干重比WWT,分别显著增加35.4%~57.2%和79.3%~120.9%。OsNRT1.2在武运粳7号过量表达可能受高浓度硝酸盐影响,促进植株对硝酸盐的吸收、生物量增加和根系生长。 5) OsNRT1.2日本晴超量表达植株水培试验中,在0.2mM NH4+、5.0mM NH4+、0.2mM NO3-、5.0mM NO3-、2.5mM NH4NO3供氮水平上,与WT的根系长度变化无显著差异。在2.5 mM NH4NO3供氮水平上,WT、1.2-OE1、1.2-OE5、1.2-OE6株高分别增加了7.8 cm、20.2 cm、18.6 cm、27.2 cm; 1.2-OE1、1.2-OE5、1.2-OE6地上部分全氮含量比WT显著增加,分别增加了27%、18%、23%和15.6%、9.5%、19.2%;生物量分别是WT的1.9、1.3、2.2倍和1.8、1.7、2.7倍。在缺氮的田间试验中,转基因植株的株高、分蘖数、有效穗数、单株产量、千粒重、结实率、地上部分干重(除1.2-OE6外)、根系干重与WT无显著差异。 6) OsNRT1.5武运粳7号转基因植株水培试验中,在5.0 mM NH4+、2.5 mM NH4NO3供氮水平上处理30天,W1.5-OE2、W1.5-OE7、W1.5-OE8干物质量比WWT分别增加了54.8%、74.6%、36.7%和68.5%、24.8%、31.5%;生长中期在5.0 mM NO3-、2.5 mM NH4NO3、5.0 mM NH4+供氮时,比野生型先分蘖,且根系粗壮。在缺氮的田间试验中,与WWT相比,武运粳7号转基因植株的单株产量(除W1.5-OE7外)、结实率、单穗粒数显著增加;株高、分蘖数(除W1.5-OE2外)、有效穗数(除W1.5-OE2外)、千粒重无显著差异。转基因植株提前分蘖,从而导致生育期提前,比野生型早成熟。OsNRT1.5在武运粳7号过量表达可能促使分蘖期提前,促进植株根系生长,从而促进植株生长、单株产量增加。OsNRTl.5在武运粳7号过量表达可能促进了氮素向籽粒的转运。 7)OsNRT1.5日本晴转基因植株水培试验中,在5.0 mM NH4+供氮水平上,1.5-OE9比WT地上部分全氮含量显著增加了13.3%;在2.5 mM NH4NO3供氮水平上,1.5-OE1、1.5-OE9、1.5-OE16比WT地上部分全氮含量显著增加了14.5%-38.2%;在5.0 mM NH4+、2.5 mM NH4NO3供氮水平上,1.5-OE1、1.5-OE9干物质量显著增加,分别是WT的1.3、1.2、倍和1.9、2.1倍;在0.2 mM NO3-、5.0 mM NO3-、0.2 mM NH4+供氮水平上,地上部分全氮含量与WT无显著差异。在缺氮的田间试验中,转基因植株株高显著降低(除1.5-OE9外),结实率和单穗粒数显著增加(除1.5-OE16外),单株产量、分蘖数(除1.5-OE1外)、有效穗数、千粒重、地上部分干重(除1.5-OE9外)没有显著差异。
URL [本文引用: 1]

钾和氮是植物生长发育所必需的矿质营养元素。在农作物生产中,钾和氮的供给水平直接影响农作物的产量和品质。然而,我国耕地土壤普遍缺钾和氮,农作物生产中需大量施用钾肥和氮肥。已有的研究报道显示,植物可以协同吸收利用钾和氮。长期的农业生产实践早已证明,按比例施用钾肥和氮肥可以显著提高肥料的吸收利用效率。然而,长久以来植物协同吸收利用钾和氮的分子机制仍不清楚。本实验室前期筛选拟南芥EMS诱变群体获得低钾敏感突变体lks2。图位克隆结果显示,lks2中NPF家族成员基因NRT1.5/NPF7.3发生单碱基突变,导致氨基酸序列的第209位甘氨酸突变为谷氨酸。本论文工作以此为基础,深入研究了NRT1.5参与钾离子(K+)在木质部装载的分子机制,探讨了K+和NO3-在木质部协同运输的分子调控机制。低钾移苗实验结果显示,与野生型相比,lks2和nrtl.5突变体表现出冠部提前发黄、根部持续生长的低钾敏感表型。回补材料lks2/NRT1.5, nrtl.5/NRT1.5能将突变体的低钾敏感表型恢复至野生型水平,表明Iks2、nrtl.5的低钾敏感表型是由NRT1.5的功能缺失导致。钾离子含量测定结果显示,无论在正常条件还是低钾条件下,nrtl.5、lks2冠部的K+含量都较野生型显著降低,而根部K+含量则较野生型显著升高,说明K+在突变体根部积累而不能被转运至冠部。硝酸根含量测定结果显示,在突变体中N03-的分布也呈现出根部高冠部低的特点。进一步检测发现,nrt1.5和lks2突变体木质部伤流液中K+和N03-含量都显著低于野生型,表明NRT1.5可能参与调控K+和NO3-由根部向冠部协同运输的生理过程。离子依赖性实验结果显示,nrt1.5和lks2突变体冠部发黄的敏感表型只依赖于外界的低钾条件,而并不依赖于外界的N03-浓度。植株体内的阳离子含量测定结果显示,nrtl.5和lks2突变体的Ca2+和Mg2+的分布并未受到显著影响,说明NRT1.5特异地调控K+由根部向冠部的转运,而不影响其它阳离子的转运。利用非洲爪蟾卵母细胞异源表达系统分析NRT1.5的离子转运功能,结果显示NRT1.5可以直接介导细胞内的K+向细胞外转运,并且NRT1.5向细胞外转运K+的活性依赖于胞外的酸性条件,而不依赖于胞内外的NO3-浓度,说明NRT1.5作为一个H+/K+反向转运体向细胞外转运K+。进一步研究显示,来自拟南芥、玉米和水稻中的NRT1.5同源蛋白都具有外向转运K+的活性。本论文工作的研究结果表明,拟南芥NRT1.5作为K+外向转运体介导K+在木质部的装载,并调控K+从根部到冠部的长距离运输,同时也影响N03-在根冠的分配。本论文研究结果还提示,NRT1.5及其同源蛋白可能是植物中一类新的K+转运蛋白,并可能参与调控植物体内K+长距离运输的生理过程。本论文的研究结果对于认知植物长距离转运钾的分子调控机制具有重要意义,也为将来利用分子遗传手段改良作物钾和氮的养分吸收利用效率提供了重要的理论依据。
URL [本文引用: 1]

钾和氮是植物生长发育所必需的矿质营养元素。在农作物生产中,钾和氮的供给水平直接影响农作物的产量和品质。然而,我国耕地土壤普遍缺钾和氮,农作物生产中需大量施用钾肥和氮肥。已有的研究报道显示,植物可以协同吸收利用钾和氮。长期的农业生产实践早已证明,按比例施用钾肥和氮肥可以显著提高肥料的吸收利用效率。然而,长久以来植物协同吸收利用钾和氮的分子机制仍不清楚。本实验室前期筛选拟南芥EMS诱变群体获得低钾敏感突变体lks2。图位克隆结果显示,lks2中NPF家族成员基因NRT1.5/NPF7.3发生单碱基突变,导致氨基酸序列的第209位甘氨酸突变为谷氨酸。本论文工作以此为基础,深入研究了NRT1.5参与钾离子(K+)在木质部装载的分子机制,探讨了K+和NO3-在木质部协同运输的分子调控机制。低钾移苗实验结果显示,与野生型相比,lks2和nrtl.5突变体表现出冠部提前发黄、根部持续生长的低钾敏感表型。回补材料lks2/NRT1.5, nrtl.5/NRT1.5能将突变体的低钾敏感表型恢复至野生型水平,表明Iks2、nrtl.5的低钾敏感表型是由NRT1.5的功能缺失导致。钾离子含量测定结果显示,无论在正常条件还是低钾条件下,nrtl.5、lks2冠部的K+含量都较野生型显著降低,而根部K+含量则较野生型显著升高,说明K+在突变体根部积累而不能被转运至冠部。硝酸根含量测定结果显示,在突变体中N03-的分布也呈现出根部高冠部低的特点。进一步检测发现,nrt1.5和lks2突变体木质部伤流液中K+和N03-含量都显著低于野生型,表明NRT1.5可能参与调控K+和NO3-由根部向冠部协同运输的生理过程。离子依赖性实验结果显示,nrt1.5和lks2突变体冠部发黄的敏感表型只依赖于外界的低钾条件,而并不依赖于外界的N03-浓度。植株体内的阳离子含量测定结果显示,nrtl.5和lks2突变体的Ca2+和Mg2+的分布并未受到显著影响,说明NRT1.5特异地调控K+由根部向冠部的转运,而不影响其它阳离子的转运。利用非洲爪蟾卵母细胞异源表达系统分析NRT1.5的离子转运功能,结果显示NRT1.5可以直接介导细胞内的K+向细胞外转运,并且NRT1.5向细胞外转运K+的活性依赖于胞外的酸性条件,而不依赖于胞内外的NO3-浓度,说明NRT1.5作为一个H+/K+反向转运体向细胞外转运K+。进一步研究显示,来自拟南芥、玉米和水稻中的NRT1.5同源蛋白都具有外向转运K+的活性。本论文工作的研究结果表明,拟南芥NRT1.5作为K+外向转运体介导K+在木质部的装载,并调控K+从根部到冠部的长距离运输,同时也影响N03-在根冠的分配。本论文研究结果还提示,NRT1.5及其同源蛋白可能是植物中一类新的K+转运蛋白,并可能参与调控植物体内K+长距离运输的生理过程。本论文的研究结果对于认知植物长距离转运钾的分子调控机制具有重要意义,也为将来利用分子遗传手段改良作物钾和氮的养分吸收利用效率提供了重要的理论依据。
[本文引用: 1]
[本文引用: 1]
DOI:10.1093/bioinformatics/btu817URLPMID:25504850 [本文引用: 1]

Summary: Visualizing genes’ structure and annotated features helps biologists to investigate their function and evolution intuitively. The Gene Structure Display Server (GSDS) has been widely used ...
DOI:10.1016/0022-2836(81)90087-5URLPMID:7265238 [本文引用: 1]

Smith TF, Waterman MS.
DOI:10.1093/molbev/mst197URL [本文引用: 1]
DOI:10.1093/bioinformatics/btm404URLPMID:17846036 [本文引用: 1]

SUMMARY: The Clustal W and Clustal X multiple sequence alignment programs have been completely rewritten in C++. This will facilitate the further development of the alignment algorithms in the future and has allowed proper porting of the programs to the latest versions of Linux, Macintosh and Windows operating systems. AVAILABILITY: The programs can be run on-line from the EBI web server: http://www.ebi.ac.uk/tools/clustalw2. The source code and executables for Windows, Linux and Macintosh computers are available from the EBI ftp site ftp://ftp.ebi.ac.uk/pub/software/clustalw2/
[本文引用: 1]
DOI:10.1093/nar/gkp335URLPMID:19458158 [本文引用: 1]

The MEME Suite web server provides a unified portal for online discovery and analysis of sequence motifs representing features such as DNA binding sites and protein interaction domains. The popular MEME motif discovery algorithm is now complemented by the GLAM2 algorithm which allows discovery of motifs containing gaps. Three sequence scanning algorithms AST, FIMO and GLAM2SCAN llow scanning numerous DNA and protein sequence databases for motifs discovered by MEME and GLAM2. Transcription factor motifs (including those discovered using MEME) can be compared with motifs in many popular motif databases using the motif database scanning algorithm Tomtom. Transcription factor motifs can be further analyzed for putative function by association with Gene Ontology (GO) terms using the motif-GO term association tool GOMO. MEME output now contains sequence LOGOS for each discovered motif, as well as buttons to allow motifs to be conveniently submitted to the sequence and motif database scanning algorithms (MAST, FIMO and Tomtom), or to GOMO, for further analysis. GLAM2 output similarly contains buttons for further analysis using GLAM2SCAN and for rerunning GLAM2 with different parameters. All of the motif-based tools are now implemented as web services via Opal. Source code, binaries and a web server are freely available for noncommercial use athttp://meme.nbcr.net.
[本文引用: 1]
DOI:10.1016/S0140-6736(00)73482-9URL [本文引用: 1]

This is a revised edition of a popular account issued in 1938 [H.A., 10: 28] based on the investigations of the two authors. Since then, experience in the U.S.A. and elsewhere has failed, in the authors' opinion, to support the early exaggerated claims for the value of the technique. Their experience leads to the conclusion that for its successful operation a knowledge of plant physiology is es...
[本文引用: 1]
DOI:10.1007/s11104-017-3204-2URL [本文引用: 1]

Boron (B) is a micronutrient essential for plant normal growth and development. Brassica napus L., one of the leading oil-crop species, is extremely susceptible to deficient and excessive B stresses.
DOI:10.1093/protein/4.2.155URL [本文引用: 1]
DOI:10.3969/j.issn.2095-1736.2014.04.068URL [本文引用: 2]

LEC1是调控植物种子发育过程和油脂产量的重要基因。本文选择功能已知的拟南芥LEC1基因为参考序列,对麻风树LEC1基因开展了蛋白组分和理化性质分析,蛋白结构和功能预测,系统进化分析等生物信息学研究,为进一步研究麻风树LEC1基因的生物学功能奠定了基础。
DOI:10.3969/j.issn.2095-1736.2014.04.068URL [本文引用: 2]

LEC1是调控植物种子发育过程和油脂产量的重要基因。本文选择功能已知的拟南芥LEC1基因为参考序列,对麻风树LEC1基因开展了蛋白组分和理化性质分析,蛋白结构和功能预测,系统进化分析等生物信息学研究,为进一步研究麻风树LEC1基因的生物学功能奠定了基础。
DOI:10.1007/s11104-018-3652-3URL [本文引用: 1]

Abstract Background Nitrogen (N) is a macronutrient that is essential for optimal plant growth and seed yield. Allotetraploid rapeseed (AnAnCnCn, 2n=4x=38) has a higher requirement for N fertilizers whereas exhibiting a lower N use efficiency (NUE) than cereal crops. N limitation adaptation (NLA) is pivotal for enhancing crop NUE and reducing N fertilizer use in yield production. Therefore,... [Show full abstract]
[本文引用: 1]
DOI:10.1101/gr.200901URL [本文引用: 2]
DOI:10.1016/S0005-2736(00)00140-1URLPMID:10748256 [本文引用: 1]

Physiological studies have established that plants acquire their NO 61 3 from the soil through the combined activities of a set of high- and low-affinity NO 61 3 transport systems, with the influx of NO 61 3 being driven by the H + gradient across the plasma membrane. Some of these NO 61 3 transport systems are constitutively expressed, while others are NO 61 3-inducible and subject to negative feedback regulation by the products of NO 61 3 assimilation. Here we review recent progress in the characterisation of the two families of NO 61 3 transporters that have so far been identified in plants, their structure and their regulation, and consider the evidence for their roles in NO 61 3 acquisition. We also discuss what is currently known about the genetic basis of NO 61 3 induction and feedback repression of the NO 61 3 transport and assimilatory pathway in higher plants.
DOI:10.1016/j.jmb.2007.08.036URLPMID:2605514 [本文引用: 1]

The rapid increase in the amount of protein sequence data has created a need for automated identification of sites that determine functional specificity among related subfamilies of proteins. A significant fraction of subfamily specific sites are only marginally conserved, which makes it extremely challenging to detect those amino acid changes that lead to functional diversification. To address this critical problem we developed a method named SPEER (specificity prediction using amino acids' properties, entropy and evolution rate) to distinguish specificity determining sites from others. SPEER encodes the conservation patterns of amino acid types using their physico-chemical properties and the heterogeneity of evolutionary changes between and within the subfamilies. To test the method, we compiled a test set containing 13 protein families with known specificity determining sites. Extensive benchmarking by comparing the performance of SPEER with other specificity site prediction algorithms has shown that it performs better in predicting several categories of subfamily specific sites.
DOI:10.1016/j.febslet.2007.04.047URLPMID:17481610 [本文引用: 2]

In higher plants, two types of nitrate transporters, NRT1 and NRT2, have been identified. In Arabidopsis, there are 53 NRT1 genes and 7 NRT2 genes. NRT2 are high-affinity nitrate transporters, while most members of the NRT1 family are low-affinity nitrate transporters. The exception is CHL1 (AtNRT1.1), which is a dual-affinity nitrate transporter, its mode of action being switched by phosphorylation and dephosphorylation of threonine 101. Two of the NRT1 genes, CHL1 and AtNRT1.2, and two of the NRT2 genes, AtNRT2.1 and AtNRT2.2, are known to be involved in nitrate uptake. In addition, AtNRT1.4 is required for petiole nitrate storage. On the other hand, some members of the NRT1 family are dipeptide transporters, called PTRs, which transport a broad spectrum of di/tripeptides. In barley, HvPTR1, expressed in the plasma membrane of scutellar epithelial cells, is involved in mobilizing peptides, produced by hydrolysis of endosperm storage protein, to the developing embryo. In higher plants, there is another family of peptide transporters, called oligopeptide transporters (OPTs), which transport tetra/pentapeptides. In addition, some OPTs transport GSH, GSSH, GSH conjugates, phytochelatins, and metals.
DOI:10.3969/j:issn.2095-1191.2012.04.425URL [本文引用: 2]

NO3^-不仅是植物从土壤中吸收的重要无机氮素形式,还是在植物体内转移的氮素形式,植物 依赖硝酸盐转运体(Nitrate transporters,NRTs)参与吸收和转运NO3^-。目前,许多****主要对NRT1.1、NRT1.2、NRT2.1进行大量研究,而对其他 硝酸盐转运体的功能及调控机制研究甚少。植物体作为一个整体,吸收、转运硝酸盐是一个连续的过程,在此过程中,各硝酸盐转运体间如何相互补充、相互协调, 仍有待进一步研究。文章通过对NRTs蛋白的结构、生物学功能和调控机制进行综述,旨在阐明植物吸收、转运NO3^-的生理机制,为通过基因工程手段提高 作物氮素利用效率的研究提供理论依据。
DOI:10.3969/j:issn.2095-1191.2012.04.425URL [本文引用: 2]

NO3^-不仅是植物从土壤中吸收的重要无机氮素形式,还是在植物体内转移的氮素形式,植物 依赖硝酸盐转运体(Nitrate transporters,NRTs)参与吸收和转运NO3^-。目前,许多****主要对NRT1.1、NRT1.2、NRT2.1进行大量研究,而对其他 硝酸盐转运体的功能及调控机制研究甚少。植物体作为一个整体,吸收、转运硝酸盐是一个连续的过程,在此过程中,各硝酸盐转运体间如何相互补充、相互协调, 仍有待进一步研究。文章通过对NRTs蛋白的结构、生物学功能和调控机制进行综述,旨在阐明植物吸收、转运NO3^-的生理机制,为通过基因工程手段提高 作物氮素利用效率的研究提供理论依据。
URL [本文引用: 1]

硝酸盐转运蛋白(NRT)是植物吸收和利用硝态氮的一种关键蛋白。运用RACE技术从茶树中扩增出NRT基因的cDNA,并利用实时荧光定量PCR检测了CsNRT基因在不同茶树器官与品种之间的差异表达。结果表明:CsNRT基因的cDNA全长2 061 bp,开放阅读框为1 818 bp,编码含由605个氨基酸组成的蛋白质,GenBank登录号为KJ160503,属于NRT2基因家族。CsNRT为组成型基因,对不同处理的水培茶苗进行定量表达分析显示,该基因在根、茎、叶中都有表达,其中在根部的表达水平最高,1.0 mmol·L-1的NO3-可诱导其表达量上升7.53倍。不同茶树品种中CsNRT基因的表达也有较大差异,‘龙井长叶’和‘凫早2号’的表达量较高,前者强烈响应0.5和1.0 mmol·L-1 NO3-的诱导,后者的响应浓度为1.0和2.0mmol·L-1,而‘舒茶早’在各浓度下的表达差异不明显。
URL [本文引用: 1]

硝酸盐转运蛋白(NRT)是植物吸收和利用硝态氮的一种关键蛋白。运用RACE技术从茶树中扩增出NRT基因的cDNA,并利用实时荧光定量PCR检测了CsNRT基因在不同茶树器官与品种之间的差异表达。结果表明:CsNRT基因的cDNA全长2 061 bp,开放阅读框为1 818 bp,编码含由605个氨基酸组成的蛋白质,GenBank登录号为KJ160503,属于NRT2基因家族。CsNRT为组成型基因,对不同处理的水培茶苗进行定量表达分析显示,该基因在根、茎、叶中都有表达,其中在根部的表达水平最高,1.0 mmol·L-1的NO3-可诱导其表达量上升7.53倍。不同茶树品种中CsNRT基因的表达也有较大差异,‘龙井长叶’和‘凫早2号’的表达量较高,前者强烈响应0.5和1.0 mmol·L-1 NO3-的诱导,后者的响应浓度为1.0和2.0mmol·L-1,而‘舒茶早’在各浓度下的表达差异不明显。
[本文引用: 1]
DOI:10.3321/j.issn:1008-505X.2004.04.018URL [本文引用: 1]

植物吸收NO-3分为高亲和力(HATS,high affinitynitratetransportsystem)和低亲和力(LATS,low affinitynitratetransportsystem)转运系统。这两个系统的编码基因均已被克隆,其中HATS由NRT2基因家族和NAR2基因家族共同编码,LATS由NRT1基因家族编码。本文比较详细地介绍了这些转运蛋白的结构和功能以及在这方面的最新进展,概要性地介绍了这些基因的表达调控;同时摘要点评了该研究领域中还没有解决的一些问题。
DOI:10.3321/j.issn:1008-505X.2004.04.018URL [本文引用: 1]

植物吸收NO-3分为高亲和力(HATS,high affinitynitratetransportsystem)和低亲和力(LATS,low affinitynitratetransportsystem)转运系统。这两个系统的编码基因均已被克隆,其中HATS由NRT2基因家族和NAR2基因家族共同编码,LATS由NRT1基因家族编码。本文比较详细地介绍了这些转运蛋白的结构和功能以及在这方面的最新进展,概要性地介绍了这些基因的表达调控;同时摘要点评了该研究领域中还没有解决的一些问题。
DOI:10.1023/A:1006332105728URL [本文引用: 1]
DOI:10.1016/j.gene.2004.12.014URLPMID:15777618 [本文引用: 1]

MIKC-type proteins represent a class of MADS-domain transcription factors and are defined by a unique domain structure: in addition to the highly conserved DNA-binding MADS-domain, they have three other domains (‘I’, ‘K’ and ‘C’), with the keratin-like K-domain being the most highly conserved and characteristic one. The number and functional diversity of MIKC-type proteins increased considerably during land plant evolution, culminating in higher flowering plants, where they dominate the control of reproductive development from early to late stages. We wonder how one special class of proteins became important in the control of essentially all stages of a morphogenetic process. All MADS-domain proteins appear to bind to DNA as homo- or heterodimers and may function as part of ternary transcription factor complexes involving non-MADS-domain proteins. Only MIKC-type proteins, however, generate complex intrafamily interaction networks. These are based on the special potential of MIKC-type proteins to form complexes involving more than two homologous proteins constituting transcriptional regulators. We speculate that the potential to form heteromultimers of homologous proteins was achieved by the acquisition of the K-domain during evolution. There is emerging evidence that organismal complexity arises from progressively more elaborate regulation of gene expression. We hypothesize that combinatorial multimer formation of MIKC-type MADS-domain proteins facilitated an unusually efficient and rapid functional diversification based on gene duplication, sequence divergence and fixation. This ‘networking’ may have enabled a more sophisticated transcriptional control of target genes which was recruited for controlling increasingly complex and diverse developmental pathways during the rapid origin and diversification of plant reproductive structures. Therefore, MIKC-type proteins may owe their evolutionary ‘success’ and present-day developmental importance in part to their modular domain structure. Investigating the evolution of MIKC-type genes may thus help to better understand origin and diversification of gene regulatory networks.
DOI:10.1016/S1671-2927(11)60052-7URL [本文引用: 1]

The objective of this study was to understand the morphological,physiological,and molecular responses of wheat roots to nitrate supply at seedling stage.Two wheat genotypes,Jimai 22 and Shannong 15,were grown in Hoagland's nutrient solution with different nitrate levels at seedling stage.Results indicated that the plant dry weight and N accumulation increased with the increase of nitrate supply.The number of axial root,total uptake area (TUA),and active uptake area (AUA) increased with more nitrate supply.Correlation analysis indicated that significant positive correlations existed between N accumulation and dry weight,N accumulation and AUA,and N accumulation and AUA/TUA.Although,the expressions of NRT2.1,NRT2.2,and NRT2.3 decreased with nitrate supply increased,the expressions of NRT1,NRT2.1,and NRT2.3 could maintain high level at N3 treatment.The free amino acid and NO3- content in shoot also increased with the increased nitrate application,but no significant difference was found in root among the treatments.These results implied that the increase of N uptake by nitrate supply was due to the morphological and physiological responses of wheat roots and the high expression level of TaNRT genes.Similarly,the contribution of morphological,physiological,and molecular parameters was different between two genotypes of wheat.