1.
Introduction
The development of nanoscience and nanotechnology is based on synthesis methods producing different nanostructures, which increases the surface to volume ratio. In recent years, wide ranges of nanosized and nanoshaped materials have been formulated to enhance properties such as chemical, electrical, mechanical, and optical properties. Significant efforts have been made to synthesize TiO2 thin films, including sol gel and hydrothermal methods[1], thermal evaporation[2], chemical bath[3], spray pyrolysis, etc. The spray pyrolysis technique is one of the simplest and cheapest techniques for the preparation of thin films with a larger area. Titanium oxide (TiO2) is a versatile material, which has been extensively studied because of its wide range of applications such as in solar cells, photocatalysis, energy storage devices, optical coating for filters and waveguides, corrosion-protective coatings, gas sensors, etc.[4–11]. In the last decade, TiO2 has been extensively studied as a gas-sensing material due to its high temperature stability, catalytic properties, and harsh environment tolerance. TiO2 is also a promising material for humidity-sensing applications in thick film and thin film forms. The anatase phase has high conductance and response to adsorbents compared with other phases[12]. Thus, the formation of a thin film with porous anatase phase with maximum surface area can enhance the humidity- and gas-sensing properties of TiO2-based sensors. For the last two decades, TiO2 thin film based humidity sensors have been extensively studied because of their hydrophilic properties[13, 14]. In the literature, there are many reports on thick-film TiO2 humidity sensors, but fewer works on TiO2 thin film based humidity sensors have been reported, even though TiO2 thin films show lower response and recovery time than thick-film sensors. In the literature, pure TiO2 synthesis by using various methods shows lower sensitivity towards humidity, as was mentioned by many researchers. To enhance the sensitivity towards humidity, various elements and their oxides have been doped into TiO2, also compositions of TiO2 with other metal oxide or PANI (polyaniline) have been synthesized to obtain porous materials with large surface area for adsorption. In general, the effect of deposition temperature on the properties of TiO2 has been extensively studied. Less emphasis has been given to precursor concentration when using the spray pyrolysis technique for the deposition of TiO2 thin films.
The present paper reports the effect of concentration of precursor solution on the structural, optical, and humidity sensing properties of TiO2 thin films. The sensitivity of the material is correlated with surface morphology and electrical (resistance) change with the corresponding change in the relative humidity.
2.
Experimental details
TiO2 thin films were deposited onto glass substrates by using a modified spray pyrolysis technique. The standard TiCl3 solution (Sigma Aldrich) with standard molarity of 7.73 M was diluted in doubled distilled water to obtain three concentrations: 0.075 M, 0.1 M, and 0.125 M. The other spray parameters such as solution to air flow rate, deposition temperature (350 °C), distance between spray nozzle to substrate (30 cm), spray time (20 min), etc., remain constant during the deposition. The deposited films were annealed in air at 500 °C for 2 h for further oxidization and crystallization of the material. The surface properties such as the phase and crystallinity of the films were analyzed by X-ray diffractometry (XRD) by using a BRUKER D8 ADVANCE diffractometer and the surface morphology was studied by using scanning electron microscopy (SEM) with a JEOL-540LV microscope. The humidity sensing characterization was performed be measuring the equivalent resistance between the thin film and reference resistance of 10 MΩ. The film resistance can be calculated by evaluating equivalent resistance of the parallel combination of the two resistances. The film resistances were measured by monitoring the change in relative humidity. The relative change in humidity was recorded by using a humidity meter (MEXTECH, model no. M288CTHW).
3.
Results and discussion
3.1
Structural studies
The concentration of the precursor solution considerably affected the structural properties of the spray-deposited TiO2 thin films[15]. The effect of the precursor concentration on the distribution of the crystallographic orientation of the polycrystalline sample was investigated by calculating the texture coefficient. The texture coefficient for all the crystallite planes was estimated by using the following expression[16].
$${T_{ m{c}}}left( {hkl} ight) = frac{{Ileft( {hkl} ight)/{I_0}left( {hkl} ight)}}{{left( {1/N} ight)mathop sum nolimits^ Ileft( {hkl} ight)/{I_0}left( {hkl} ight)}},$$ ![]() | (1) |
where Tc(hkl) is the texture coefficient of the (hkl) plane, I(hkl) is the measured intensity from the (hkl) plane, I0(hkl) is JCPDS standard intensity of the (hkl) plane, and N is the number of diffraction peaks.
The mean crystallite size of the line broadening of the (101) plane was evaluated by using the Scherrer formula[17],
$$D = frac{{Klambda }}{{beta cos theta }},$$ ![]() |
where K = 0.9 is the shape factor, λ is the X-ray wavelength of CuKα radiation (1.5406 ?), θ is the Bragg’s angle, and β is the full width at half maximum of the peak.
The broadening of the diffraction line is due to microstresses on the crystallite. The shifting of the diffraction line is due to the macrostress on the crystallite. The broadening of the line due to microstress causes microstrain in the crystallite. The mean strain (ε) developed in the crystallite normal to the diffraction plane can be calculated by the relationship[18]
$$varepsilon = frac{{Delta d}}{{{d_0}}} = frac{{ {d-{d_0}} }}{{{d_0}}},$$ ![]() |
where d0 is the interplanar distance of the bulk material (TiO2 d0 = 3.520 ?), d is the experimentally predicted interplanar distance.
The maximum tensile or compressive strain along the diffraction plane is represented in terms of microstress present in the sample. The maximum microstress present in the sample can be obtained with the relationship[18],
$${sigma _{{ m{stress}}}}{ m{ = }}frac{varepsilon }{2}E,$$ ![]() |
where E is the Young’s modulus of the material (TiO2 E = 282.76 GPa)[19].
The dislocation density is the number of dislocated lines per unit area normal to the surface area. In crystalline materials, the dislocation density is the dislocated lines per unit volume of the crystallite. The value of dislocation density (δ) is calculated by using the following relationship[20, 21]
$$delta = n/{D^2},$$ ![]() |
where n is a factor, which when equal to unity gives the minimum dislocation density, and D is the crystallite size.
X-ray diffractograms of the films are given in Fig. 1. Films deposited with different precursor concentrations and annealed at a temperature of 500 °C for 2 h had a polycrystalline nature with anatase TiO2 crystalline phase. The crystalline planes oriented along anatase TiO2 (101), (112), (200), (105), (211), (204), (220), and (216) planes. With the increase in precursor concentration, the films showed increased crystallinity, which is observed by the corresponding increase in intensity and lower peak broadening in the diffractograms. The films exhibited tetragonal crystal structure with space group I41/amd, and unit cell parameters a = 3.7852 ?, c = 9.5139 ?. The peak intensities showed good agreement with the JCPDS data (#21-1272). The intensity and full width at half maximum of the diffraction peaks was observed to increase proportionally with the precursor concentration, which is in agreement with Ref. [15]. The structural parameters such as crystalline size, dislocation density, and microstrain were determined from the appropriate equations and are reported in Table 1. With the increase in precursor concentration from 0.075 M to 0.125 M, the size of the crystallites also showed an increase from 25.37 to 33.16 nm and is consistent with earlier reports[15].

class="figure_img" id="Figure1"/>
Download
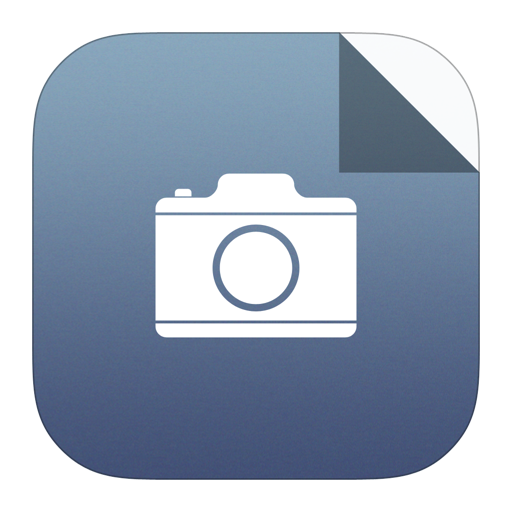
Larger image
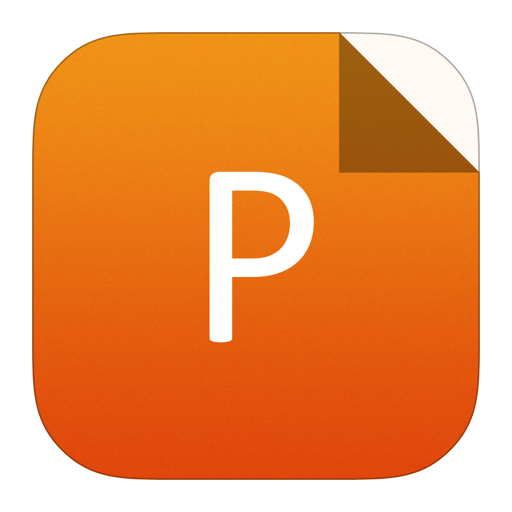
PowerPoint slide
Figure1.
(Color online) XRD spectra of TiO2 thin films deposited at different precursor concentrations and annealed at 500 °C for 2 h.
Sample | Precursor concentration (M) | Crystallite size (nm) | Texture coefficient | Micro-strain (10?3) | Micro-stress (108 Pa) | Dislocation density (1014 cm?2) |
C1 | 0.075 | 25.37 | 1.47 | 1.98 | 2.80 | 15.53 |
C2 | 0.1 | 31.21 | 1.56 | 1.67 | 2.37 | 10.26 |
C3 | 0.125 | 33.16 | 1.84 | 1.27 | 1.79 | 9.09 |
Table1.
Structural properties of TiO2 film deposited by spray pyrolysis with different precursor solution.
Table options
-->

Download as CSV
Sample | Precursor concentration (M) | Crystallite size (nm) | Texture coefficient | Micro-strain (10?3) | Micro-stress (108 Pa) | Dislocation density (1014 cm?2) |
C1 | 0.075 | 25.37 | 1.47 | 1.98 | 2.80 | 15.53 |
C2 | 0.1 | 31.21 | 1.56 | 1.67 | 2.37 | 10.26 |
C3 | 0.125 | 33.16 | 1.84 | 1.27 | 1.79 | 9.09 |
The microstrain in films is due to the defects in crystal lattices, dislocations, vacancies, staking faults, etc. With the decrease in precursor solution concentration, the imperfection (defects) in films were increased, as indicated by the increase in microstrain on the surface of the film, shown in Table. Thus, the corresponding change in microstress is of the tensile type and also increased with the decrease in precursor solution concentration[22]. The decreased in microstrain with increased crystallite size is a well-known phenomenon[23–25]. The texture is a preferred crystallographic orientation in a polycrystalline material. The texture coefficient determines the preferred orientation of the crystallites in a polycrystalline aggregate. The increase in the texture coefficient (Tc) of the (110) plane implies the improvement of crystalline quality[26].
3.2
Surface morphological studies
The surface micrographs of TiO2 thin films prepared at different precursor concentrations and annealed at 500 °C for 2 h are presented in Fig. 2. It is observed that the film prepared at a precursor concentration of 0.075 M is pinhole and pore free, whereas the films prepared at a precursor concentration of 0.1 M have nano or micropores and some pores are observed in the film deposited at a precursor concentration of 0.125 M. Therefore, the average diameter of the pores increases with the increasing concentration of the precursor solution. The diffusion and film growth rate depend on the species concentration and temperature of the deposition[27]. Therefore, the concentration of the precursor solution can control the morphology, grain size, and thickness of the films. With the increase in concentration, the grain size and thickness of the film increases, which causes some pores with small diameters. The film prepared at a precursor concentration of 0.1 M shows the formation of a uniform and dense interconnected web-like structure. Similar web-like morphology has been reported for TiO2 thin films prepared by the spray pyrolysis method[15]. The interconnected webs are of variable diameter, which may be due to the sequential growth of webs; one above another during the growth process.

class="figure_img" id="Figure2"/>
Download
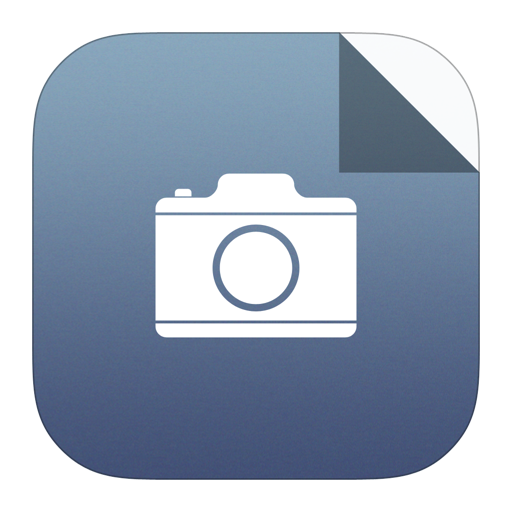
Larger image
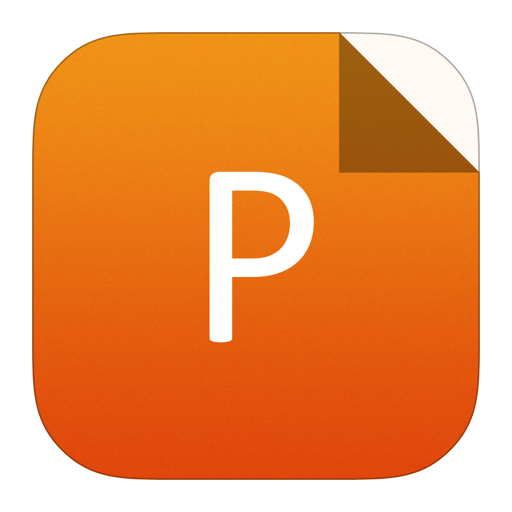
PowerPoint slide
Figure2.
SEM images of spray pyrolysis deposited TiO2 thin films with precursor concentrations from 0.075 M to 0.125 M.
3.3
Optical properties
The optical absorption spectra of pure TiO2 thin films deposited on glass substrates by using a modified spray pyrolysis technique were obtained by using a UV–vis spectrophotometer. Fig. 3 shows the optical absorption spectra of pure TiO2 thin films deposited at different precursor solution concentrations with deposition temperature of 350 °C. The absorption edge was found to be sharp and showed a shift towards lower wavelength region with an increase in precursor solution concentration. This may be due to the change in crystal orientation in the film. The optical absorption spectra of TiO2 films were investigated in the wavelength range 200–800 nm, at ambient temperature. The energy band gap of the films was evaluated from the relationship[18]

class="figure_img" id="Figure3"/>
Download
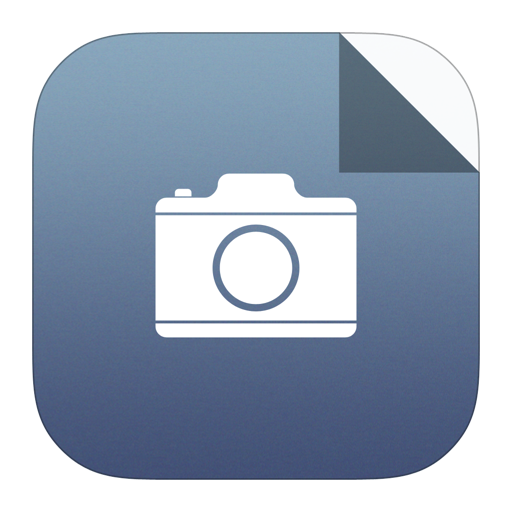
Larger image
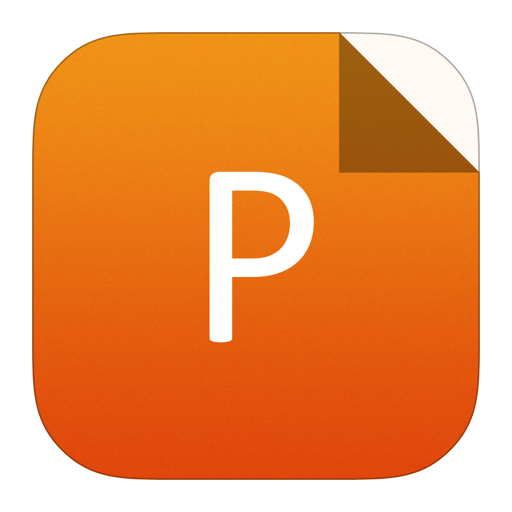
PowerPoint slide
Figure3.
(Color online) Optical absorption spectra of TiO2 films deposited with different precursor concentrations.
$${left( {alpha hnu } ight)^2} = A(hnu -{E_{ m{g}}}),$$ ![]() |
where A is the proportionality constant and Eg is the direct transition band gap, α is the optical absorption coefficient as a function of the wavelength.
The band gaps of the materials were estimated from the (absorbance)2 versus E plot, as shown in Fig. 4. The band gap values decreased from 3.50 to 3.40 eV with the decrease in precursor solution concentration as shown in Table 2. The band gap of the material depends upon the crystallite size, grain size, microstrain, dislocation density, etc., of the material. The decrease in band gap may be due to the increase in grain size corresponding to the precursor concentration and is consistent with the quantum confinement effect[21, 28]. The tunable band gap of the material can control the adsorption of water molecules[29].

class="figure_img" id="Figure4"/>
Download
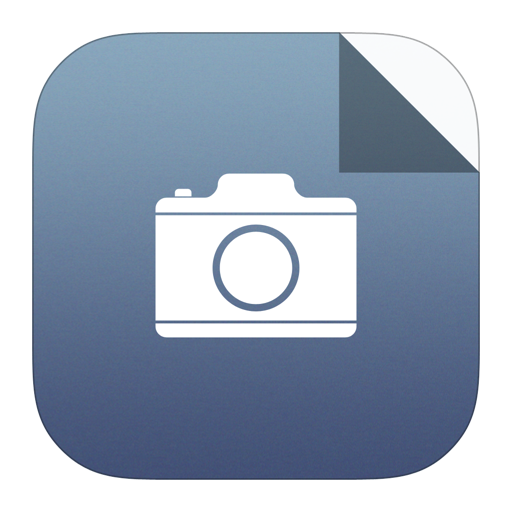
Larger image
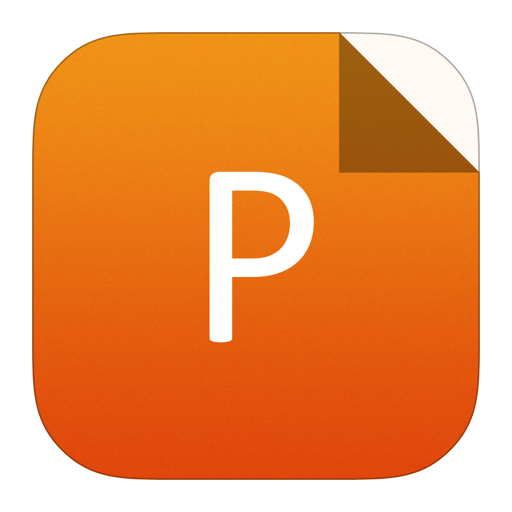
PowerPoint slide
Figure4.
(Color online) Optical band gap measurement of TiO2 films deposited with different precursor concentrations.
Sr. No. | Concentration (M) | Sample | Band gap (eV) |
1 | 0.075 | C1 | 3.40 |
2 | 0.1 | C2 | 3.44 |
3 | 0.125 | C3 | 3.50 |
Table2.
Optical properties – indirect band gap of samples with different precursor concentration.
Table options
-->

Download as CSV
Sr. No. | Concentration (M) | Sample | Band gap (eV) |
1 | 0.075 | C1 | 3.40 |
2 | 0.1 | C2 | 3.44 |
3 | 0.125 | C3 | 3.50 |
3.4
Humidity sensing properties
The humidity sensing properties of the TiO2 films deposited with various precursor concentrations were measured by monitoring the change in resistance with the corresponding change in relative humidity. The sensitivity toward humidity has been defined by the change in resistance (ΔR) of the material per unit change in relative humidity (RH%)[29]. Sensitivity can also be defined as the ratio of the resistance variations (ΔR between highest and lowest RH values) to the resistance values at the lowest RH levels as a percentage[30]
$$S = frac{{{R_{ m{H}}} - {R_{ m{D}}}}}{{{R_{ m{D}}}}} times 100.$$ ![]() |
The resistance of the sample decreases exponential with the increase in relative humidity. At lower relative humidity, the resistance decreases faster than at higher relative humidity as shown in Fig. 5. The resistance of the TiO2 sample decreases because the adsorbed water condensed on the surface of the materials forms a superficial liquid-like layer and protons will be conducted.

class="figure_img" id="Figure5"/>
Download
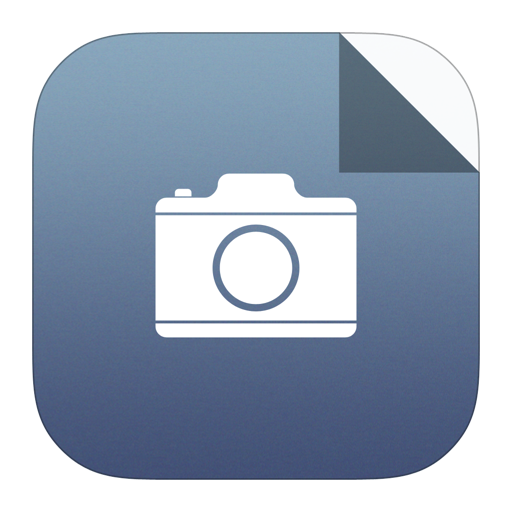
Larger image
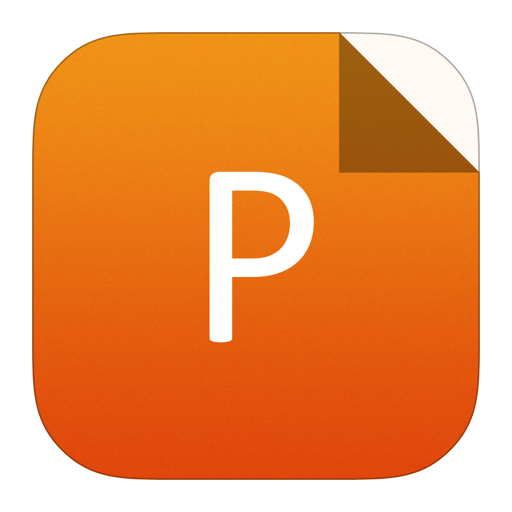
PowerPoint slide
Figure5.
(Color online) Variation of resistance of spray-deposited films for various concentrations with relative humidity.
The response time of the sensor is represented by the time taken to achieve 90% of the total resistance change for the adsorption process whereas the recovery time of the sensor is represented by the time taken to achieve 90% of the total resistance change for the desorption process as shown in Fig. 6. The TiO2 sample synthesis with the precursor concentration 0.1 M shows 77.5 s response time and a significantly lower 3 s recovery time as shown in Table 3. The response time for the sample with 0.125 M concentration is high due to the presence of pin holes but shows higher sensitivity than that of the samples deposited at 0.1 M and 0.075 M. The sensitivity of the samples increases with the increase in the precursor concentration (Fig. 7), thus the sensitivity depends on the particle size and morphology.

class="figure_img" id="Figure6"/>
Download
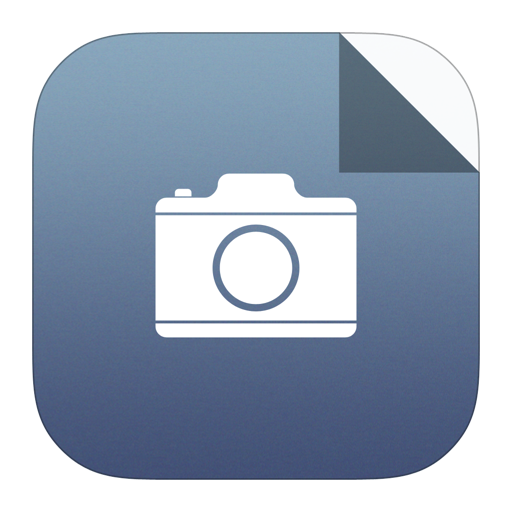
Larger image
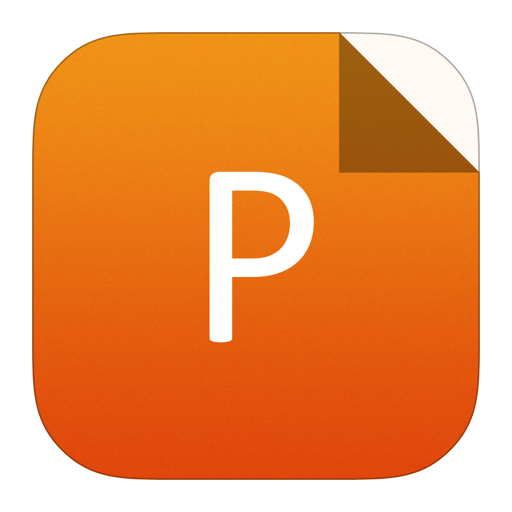
PowerPoint slide
Figure6.
(Color online) Response and recovery time estimation of spray-deposited films for various concentrations.

class="figure_img" id="Figure7"/>
Download
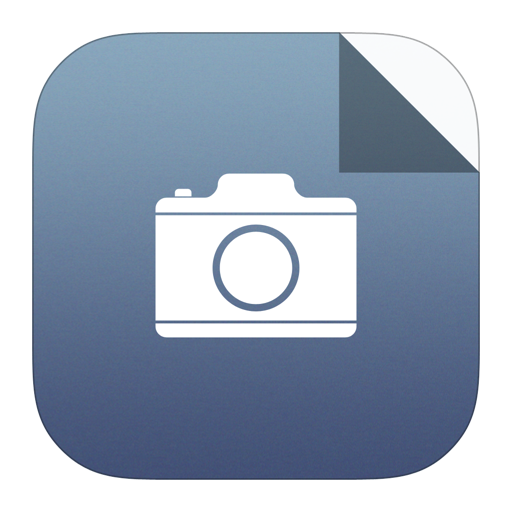
Larger image
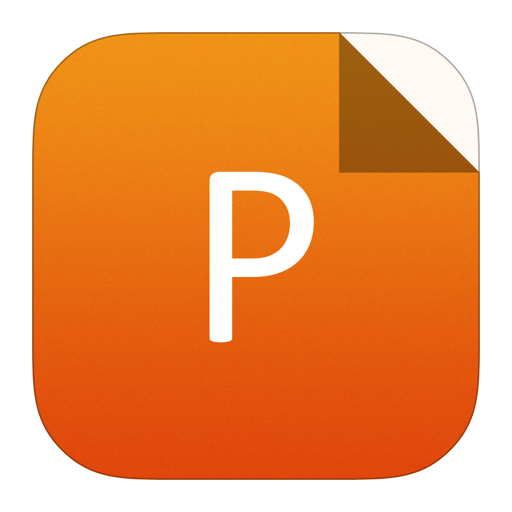
PowerPoint slide
Figure7.
(Color online) Sensitivity of the samples for different relative humidities.
Sample | Relative humidity (RH%) | Sensitivity (%) | Response time (s) | Recovery time (s) |
C1 | 90 | 70.47 | 149.5 | 4.5 |
50 | 10.60 | 105.5 | 4 | |
C2 | 90 | 80.85 | 77.5 | 3 |
50 | 20.86 | 69 | 2.5 | |
C3 | 90 | 84.54 | 193 | 5 |
50 | 17.27 | 183 | 4.5 |
Table3.
Summaries the humidity sensing properties of the synthesis sample.
Table options
-->

Download as CSV
Sample | Relative humidity (RH%) | Sensitivity (%) | Response time (s) | Recovery time (s) |
C1 | 90 | 70.47 | 149.5 | 4.5 |
50 | 10.60 | 105.5 | 4 | |
C2 | 90 | 80.85 | 77.5 | 3 |
50 | 20.86 | 69 | 2.5 | |
C3 | 90 | 84.54 | 193 | 5 |
50 | 17.27 | 183 | 4.5 |
The specific surface area can also contribute toward the humidity sensitivity. The specific surface area depends upon the crystallite size; it is inversely proportional to the crystallite size and density of the material[31]. Therefore, with the increase in crystallite size, the specific surface area decreases, which cause the increase in sensitivity at higher concentrations, that is, 0.125 M. The pores in the films could not contribute to the charge conduction unless and until it is completely filled with condensed water vapor. At lower humidity, larger sized pores will fill with water vapor, which condenses on its walls and then fills the empty area of the pore, which results in lower sensitivity of the sample at lower humidity and the higher response time. Therefore, at the higher concentration (0.125 M), the decreased in response time is due to the increase in average pore size. Therefore, nano- or micropores are essential for higher humidity sensitivity[32]. The thin-film sensor shows lower sensitivity and high hysteresis[33], but in the present work, we report the highest sensitivity of 87% and negligible hysteresis for the sample synthesized at the precursor concentration of 0.1 M as shown in Fig. 8.

class="figure_img" id="Figure8"/>
Download
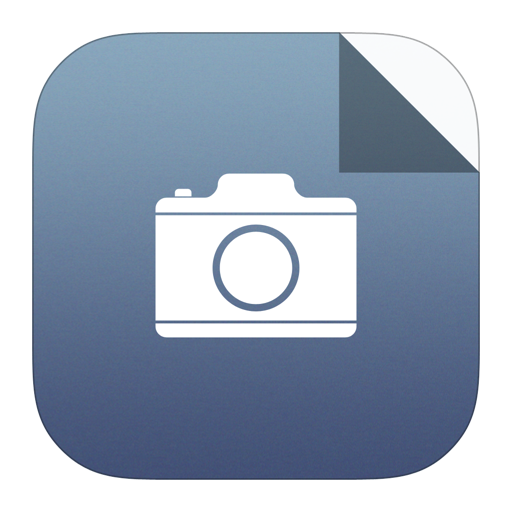
Larger image
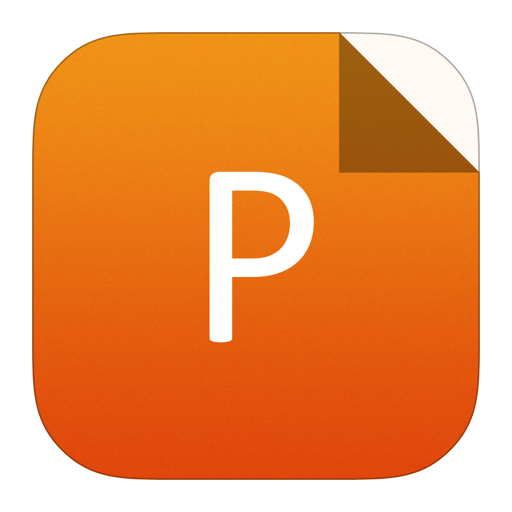
PowerPoint slide
Figure8.
(Color online) Change in equivalent resistance between parallel combination of film resistance and 10 MΩ reference resistance with increasing and decreasing relative humidity for various precursor concentrations.
In the literature, fewer reports are available on pure TiO2 thin film synthesis by using several methods and their humidity sensing applications. Sasikumar and Subiramaniyam[34] recorded a maximum sensitivity of about 64% at relative humidity of 90% with low response and recovery time of 55 and 20 s for sol gel synthesized TiO2. Chantarawong et al.[35] recorded a minimum response and recovery time of about 20 and 23 s for electrostatic spray synthesized TiO2 thin films. In the present study, we report a maximum sensitivity and low response time for pure TiO2 thin films. This is the most significant result from this study.
4.
Conclusion
Pure TiO2 thin films were successfully deposited onto glass substrates at 350 °C by using a spray pyrolysis technique and by varying the precursor concentration. XRD study revealed that the deposited TiO2 thin films have polycrystalline anatase phase with dominant (101) plane. The crystallite size of the film material was found to increase with increasing precursor concentration. The microstrain, microstress, and dislocation density of the films were decreased with increasing precursor concentration. The optical band gap of the TiO2 films was observed to increase from 3.40 to 3.50 eV as the precursor concentration was increased. The resistance of the TiO2 film decreases because of the adsorbed water condensed on the surface of the materials forms a superficial liquid-like layer and protons will be conducted. The sensitivity of the samples increases with the increase in the precursor concentration.
Acknowledgments
We thank our departmental students as well as all faculty members of department of Physics Sangamner College, Sangamner, for the synthesis and characterization of the materials. We are also thankful to the Thin and Thick Film Laboratory, Dept. of Electronics M.S.G. College, Malegaon Camp. We thank the Department of Science and Technology, New Delhi, for providing funds to the college under the DST–FIST scheme, which helped with the characterization and synthesis of materials. We also appreciate the technical support and characterization facility provided by the Department of Physics S.P. Pune University.