1.
Introduction
Vertical cavity surface emitting lasers (VCSELs) have a large number of applications in optical communication, optical sensors, optical storage, and optical interconnection due to their low threshold current, low power consumption, good beam quality, small far field divergence angle, high speed modulation, and high density two-dimensional integration[1–3]. VCSELs were the core devices of 3D sensing technology of facial recognition in the iPhone X, which means that VCSELs have been extensively applied in the field of consumer electronics. The mass fabrication cost of VCSELs can be reduced by using wet-etching and wet nitrogen oxidation.
As we all know, the oxidation confined layer with good insulation and low refractive index can be used to achieve electrical and optical mode confinement through transverse oxidation of Al0.98Ga0.02As[4–8]. Moreover, the oxidation confined layer can improve the quantum efficiency to obtain lower threshold current and higher power conversion efficiency. The general rule of the wet nitrogen oxidation with oxidation time, temperature and Al component has been studied in many previous articles[9–12]. However, the anisotropy caused by the wet nitrogen oxidation has not been studied yet.
In the paper, the effect of time and temperature on wet nitrogen oxidation is studied. Moreover, the anisotropy of the oxidation rate with three mesa shapes was studied. Furthermore, the devices with the same size of diamond side length and circular diameter were fabricated, and the differences in P–I–V characteristics and threshold currents were also compared.
2.
Device structure and experiment
The epitaxial structure of the VCSEL was grown by metal–organic chemical vapor deposition on a heavily N-doped GaAs substrate. The top and bottom distribute Bragg reflection (DBR) contain 20 and 34 pairs of Al0.9Ga0.1As/ Al0.12Ga0.88As respectively. The active region with an optical length of 1λ has three GaAs quantum wells separated by four Al0.3Ga0.4As barriers and phase matching layer. A 30-nm-thick Al0.98Ga0.02As oxidation confined layer above the active region can be oxidized by water vapor in a nitrogen atmosphere to form the insulating oxide film (AlxOy) with a low refractive index of 1.5. The wet nitrogen oxidation was realized by using a 5271 diffusion furnace, which was mainly composed of a 140 cm quartz furnace tube with a diameter of 8 cm, a quartz bottle with 3 L volume and a nitrogen bottle. In order to control the oxidation rate accurately, in each experiment the water temperature, the water level and the time of system stability were unified. The oxidation aperture can also be measured by a microscope, due to the remarkable color differences between oxidized and un-oxidized zones of the Al0.98Ga0.02As layer.
The essence of the wet nitrogen oxidation process is the chemical reaction between H2O carried by N2 and AlAs in Al0.98Ga0.02As. In the related literature, the products are a mixture of crystal products and amorphous products, including Al crystal oxide γ-Al2O3[13], amorphous Al(OH)3 and Al(OH), as well as chemical compound AsH3, As2O3, and so on[14–16].
3.
The effect of time and temperature on wet nitrogen oxidation rate and anisotropy
Diamond (45° of each side and two crystal orientation), square (sides parallel to two crystal orientation) and circular mesas were designed for studying the anisotropy of wet nitrogen oxidation. The side length of diamond and square mesa and the circular diameter were 84 μm. As shown in Fig. 1, at 430 °C under the condition of 9 L/min N2 flow rate and 90 °C water temperature, the oxidation depth increases gradually and the oxidation aperture decreases with the increase of oxidation time. The Al0.98Ga0.02As oxidation confined layer is actually dark red after oxidation. The oxidation confined layer in Fig. 1 is pale pink as it is covered by 60 nm thick Al0.12Ga0.88As. The oxide-apertures of the diamond mesa always maintain a diamond shape with different oxidation time, but the square and circular mesa appear as a rectangle and an ellipse, which is more obvious with the increase of the oxidation time.

class="figure_img" id="Figure1"/>
Download
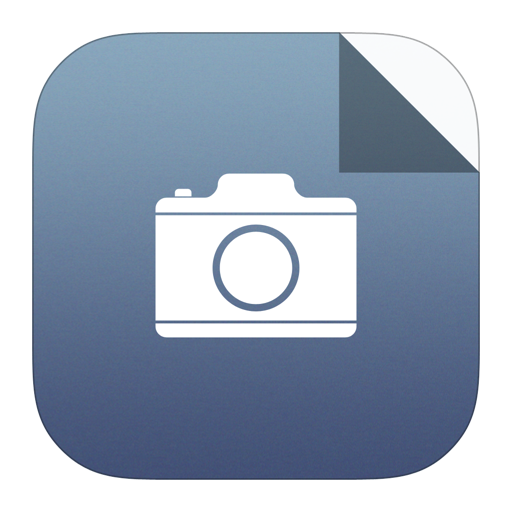
Larger image
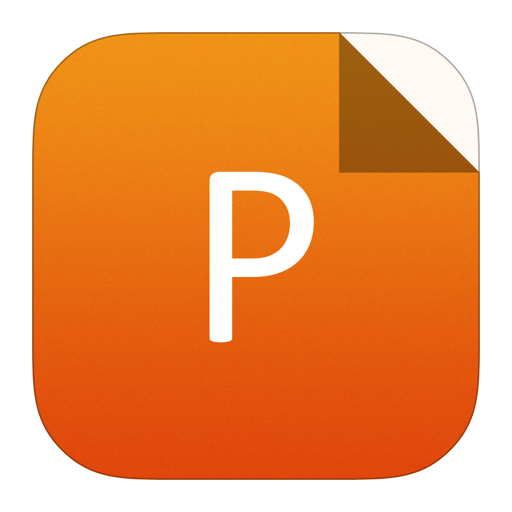
PowerPoint slide
Figure1.
(Color online) The oxidation aperture microscope images of three mesa shapes at different oxidation time at 430 °C. Un-oxidized zone is purple and oxidized zone is pink.
Five sizes of three mesa shapes, including the side length of diamond, the side length of square on [011] (square transverse), the side length of square on [0–11] (square longitudinal), the diameter of circular on [011] (circular transverse) and the diameter of circular on [0–11] (circular longitudinal) were further measured. The detailed oxidation depths and oxidation rates with different oxidation times are shown inFigs. 2(a) and 2(b), respectively. The oxidation rate of diamond mesa is ~ 0.175 μm/min at 410 °C and ~ 0.4 μm/min at 430 °C. The oxidation rate slightly increases from ~ 0.17 to ~ 0.187 μm/min at 410 °C and increases from ~ 0.38 to ~ 0.41 μm/min at 430 °C with the increase of time due to the water molecules concentration in unit area increasing with the decrease of the reaction area. The oxidation rate of square transverse and circular transverse are generally higher than that of diamond, while the oxidation rate of square longitudinal and circular longitudinal are lower than that of the diamond. This phenomenon is further obvious with the increase of the oxidation time, which is mainly due to the bond energy difference between [0–11] and [011] being amplified.

class="figure_img" id="Figure2"/>
Download
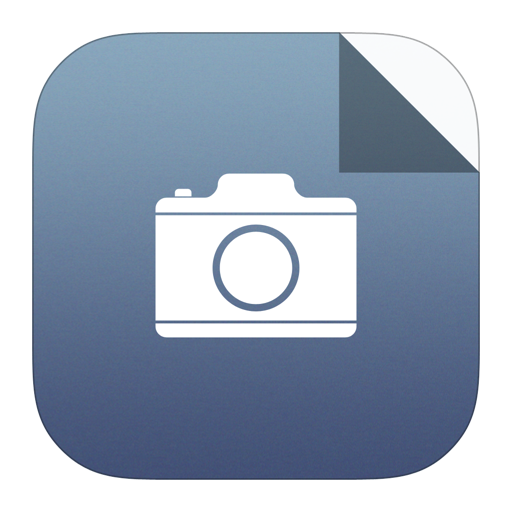
Larger image
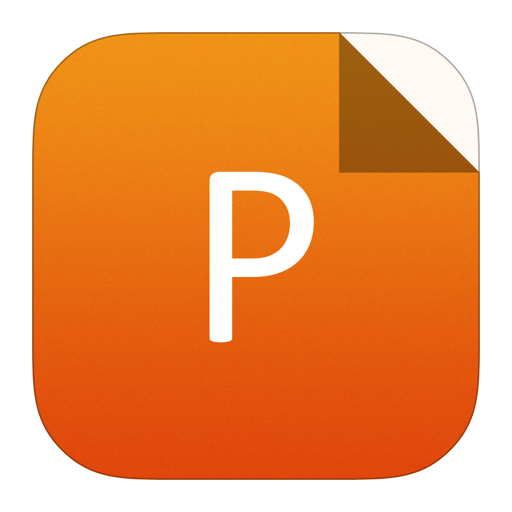
PowerPoint slide
Figure2.
(Color online) (a) Effect of oxidation time on oxidation depth at 430 and 410 °C. (b) Effect of oxidation time on oxidation rate at 430 and 410 °C.
The molecular activity and the collisional bonding probability of the reactants are intently affected by temperature. The temperature of wet nitrogen oxidation is generally within the range of 370–450 °C. When the temperature increases from 410 to 440 °C under the condition of 9 L/min N2 flow rate, 90 °C water temperature and 1 hour oxidation time, the oxidation rate of diamond mesa increases from ~ 0.175 to ~ 0.65 μm/min, as shown in Fig. 3. The oxidation rate of the square transverse and the circular transverse are generally higher than that of the diamond, while the oxidation rate of the square longitudinal and the circular longitudinal are lower than that of the diamond. The higher the temperature, the bigger the differences of the oxidation rate.

class="figure_img" id="Figure3"/>
Download
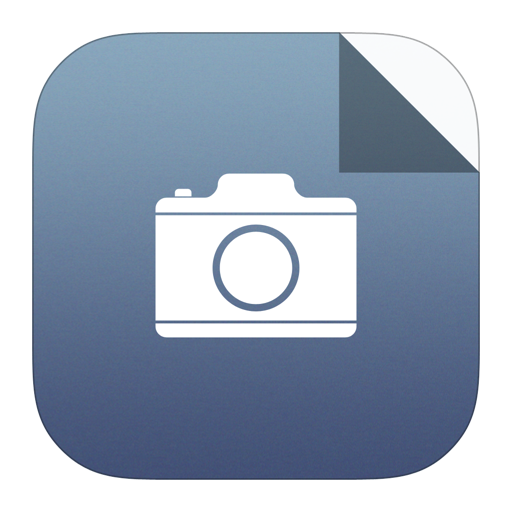
Larger image
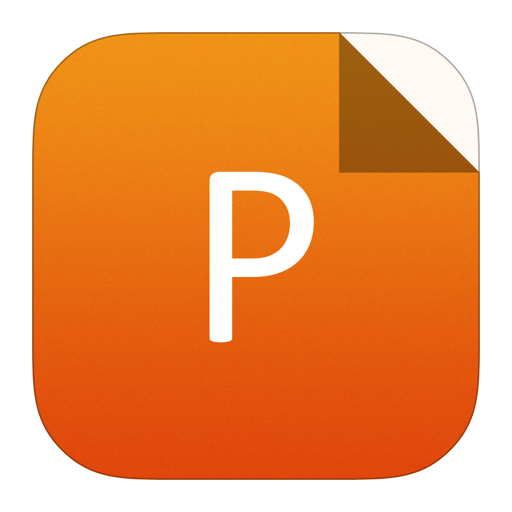
PowerPoint slide
Figure3.
(Color online) Effect of temperature on oxidation rate with different shapes and anisotropies of VCSELs. Inset: a close-up of the oxidation rate lines with different shapes and anisotropies of VCSELs.
In order to characterize the anisotropy of the oxidation rate, we define anisotropy coefficient γ as the ratio of the wet nitrogen oxidation rate between [0–11] (longitudinal direction in Fig. 1) and [011] (transverse direction in Fig. 1), and its expression is as follows:
$gamma = {{{nu _{[0 - 11]}}} over {{nu _{[011]}}}}.$ ![]() | (1) |
According to Eq. (1), the influence of the oxidation time on the anisotropy coefficient at 430 °C is shown in Fig. 4(a). The anisotropy coefficient of diamond mesa is 1 in the whole range of oxidation time. Owing to the existence of 45° of each side and two crystal orientations, the influence of two crystal orientations on the oxidation rate of each side length is consistent. However, for the circular and square mesa, the anisotropic coefficient decreases with the increase of oxidation time, which is caused by amplifying the difference of the bond energy of two crystal orientations. On the whole, the anisotropy coefficient of the circular mesa was lower than that of the square mesa, which was probably due to the radian of circular mesa. The temperature-influence on the anisotropy coefficient under 1 h oxidation time for three mesas is shown in Fig. 4(b), which is similar to the influence of oxidation time.

class="figure_img" id="Figure4"/>
Download
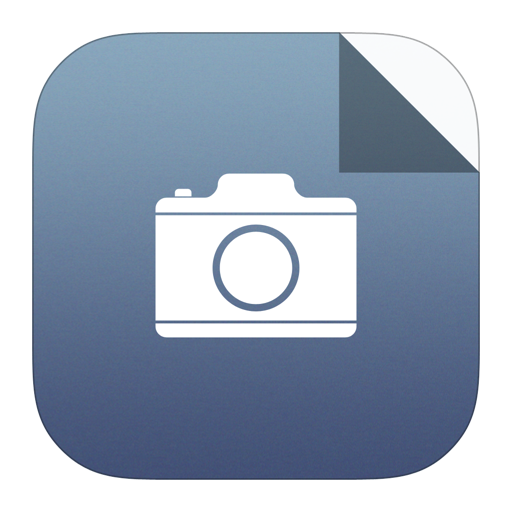
Larger image
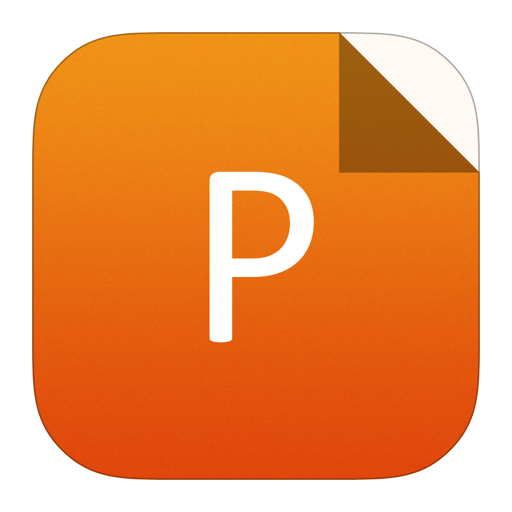
PowerPoint slide
Figure4.
(Color online) (a) The variation of anisotropy coefficient with oxidation time of three mesa shapes of diamond, round and square at 430 °C. (b) The variation of the anisotropy coefficient with the temperature of three mesa shapes of diamond, round and square in 1 h oxidation.
4.
The anisotropy effect on its performance
The side length of the diamond and the circular diameter were designed with 84 μm before oxidation. A diamond device with an aperture of 9 μm side length and a circular device with an aperture of 10.5 μm long axis on [0–11] and 7 μm short axis on [011] were fabricated under the condition of 420 °C, 9 L/min N2 flow rate, 90 °C water temperature and 110 min. The P–I–V characteristic curves of the VCSELs with diamond and circular oxide-apertures are shown in Fig. 5. The cut-in voltage is ~ 1.3 V, the threshold currents are ~ 1.4 and ~ 1 mA and differential resistance are ~ 345 and ~ 450 Ω, respectively, for the VCSELs with diamond and circular oxide-apertures. Function of the VCSEL output light power is described as[17]:

class="figure_img" id="Figure5"/>
Download
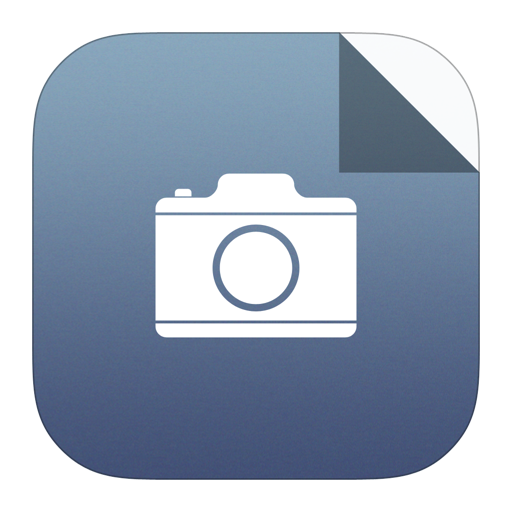
Larger image
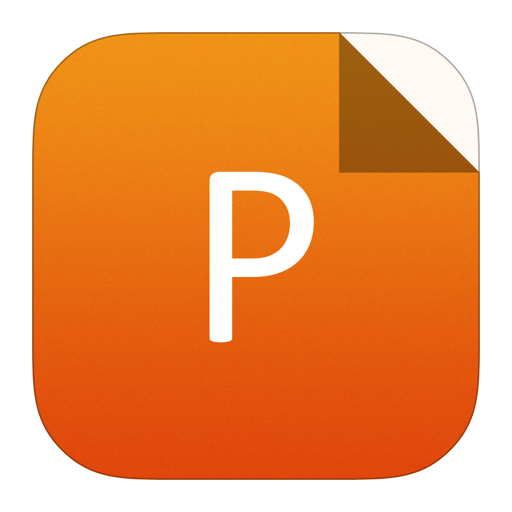
PowerPoint slide
Figure5.
(Color online) The P–I–V characteristic curves of diamond and circular devices.
$P = {eta _{ m {i}}}{eta _0}frac{{hnu }}{q}(I - {I_{ m {th}}}) I > {I_{ m {th}}}, $ ![]() | (2) |
wherein ηi and η0 are the respective internal and external quantum efficiency, h is the Planck constant and ν is frequency. The output light power of diamond-oxide-aperture VCSEL is higher than that of the circular under the same oxidation condition, due to smaller differential resistance, lower heat production and higher thermal saturation current.
The loss of the VCSEL is α = αi + αm, wherein, αi is internal loss and αm is mirror loss. The αm depends on the reflectivity of the top and bottom DBR, which is expressed as αm = ln(1/R1R2)/2L, wherein R1 and R2 are the reflectivity of the top and bottom DBR, respectively, and L is the equivalent cavity length. The threshold carrier concentration can be solved according to the generalized logarithmic relation of gain[17],
$alpha = g = {G_0}{ m {ln}}frac{{n + {n_0}}}{{{n_{ m tr}} + {n_0}}},$ ![]() | (3) |
wherein n is carrier concentration, ntr is transparent carrier concentration and n0 is fitting parameters.
${I_{ m {th}}} = frac{{Vq{n_{ m {th}}}}}{{{tau _{ m e}}eta }},$ ![]() | (4) |
wherein V is quantum well active region volume V = Sl, l is the sum of quantum well thickness, S is the cross section area of carriers injected into the active region, q is electron charge, nth is threshold carrier concentration, τe is carrier life and η is carrier injection efficiency of laser.
The threshold current is mainly influenced by the oxidation aperture. Ithd and Ithc are respectively defined as the threshold current of diamond and circular devices. xd is the oxidation depth of the diamond device. x1c and x2c are the oxidation depth of the circular device on [0–11] and [011] crystal orientation. The anisotropic coefficients of the diamond and circular mesa areγd and γc respectively, and a0 is assumed as the mesa size before oxidation, there were:
$I_{ m {th}}^{ m d} = frac{{{V^{ m d}}q{n_{ m {th}}}}}{{{tau _{ m e}}eta }} = frac{{lq{n_{ m {th}}}}}{{{tau _{ m e}}eta }}{({{ {a}}_0} - {x^{ m d}})^2}{gamma ^{ m d}},$ ![]() | (5) |
$I_{ m th}^{ m c} = frac{{{V^{ m c}}q{n_{ m {th}}}}}{{{tau _{ m e}}eta }} = frac{{lq{n_{ m {th}}}}}{{{tau _{ m e}}eta }} times frac{pi }{4}({{a}_0} - x_1^{ m c})({a_0} - x_2^{ m c}).$ ![]() | (6) |
From the anisotropy coefficient of circular device γc = x1c/x2c and the oxidation depth of diamond device xd = (x1c + x2c)/2, we got:
$x_1^{ m c} = frac{{2{x^{ m d}}{gamma ^{ m c}}}}{{{gamma ^{ m c}} + 1}}.$ ![]() | (7) |
$x_2^{ m c} = frac{{2{x^{ m d}}}}{{{gamma ^{ m c}} + 1}}.$ ![]() | (8) |
Based on Eqs. (7) and (8), Eqs. (5) and (6) can be written as:
$I_{ m {th}}^{ m d} = frac{{lq{n_{ m {th}}}}}{{{tau _{ m e}}eta }}[{a_0}^2 - 2{a_0}{x^{ m d}} + {({x^{ m d}})^2}]{gamma ^{ m d}},$ ![]() | (9) |
$I_{ m {th}}^{ m c} = frac{{lq{n_{ m {th}}}}}{{{tau _{ m e}}eta }} times frac{pi }{4}[{a_0}^2 - 2{a_0}{x^{ m d}} + frac{{4{gamma ^{ m c}}}}{{{{(1 + {gamma ^{ m c}})}^2}}}{({x^{ m d}})^2}].$ ![]() | (10) |
Taking a0 = 84 μm, xd = 37.5 μm, γc = 0.9545 and γd = 1 into Eqs. (9) and (10):
$frac{{I_{ m {th}}^{ m c}}}{{I_{ m {th}}^{ m d}}} = 0.785.$ ![]() | (11) |
As a result, the experimental threshold current ratio of ~ 0.714 is basically consistent with the theoretical calculated ratio of ~ 0.785.
5.
Conclusion
The impact of oxidation time and temperature on the oxidation rate was studied in this paper. The oxidation rates are approximately ~ 0.4 μm/min at 430 °C and ~ 0.175 μm/min at 410 °C, and they increased slightly with the increase of oxidation time due to the increase of water molecules concentration. When the temperature is increased from 410 to 440 °C with 1 h oxidation time, the molecular activity enhances and the collisional bonding probability of the reactants became larger, thus the oxidation rate increased from ~ 0.175 to ~ 0.65 μm/min. Diamond, circular and square mesas are designed to study the anisotropy of the oxidation rate between [0–11] and [011]. It was shown that the anisotropy coefficient decreased with the increase of the oxidation time and oxidation temperature. The relationship between the anisotropy coefficient and the threshold current is obtained. A diamond device with an aperture of 9μm side length and a circular device with an aperture of 10.5 μm long axis on [0–11] and 7 μm short axis on [011] were fabricated, whose threshold current ratio of ~ 0.714 was basically consistent with the theoretical calculated ratio of ~ 0.785. Our research on wet nitrogen oxidation and its anisotropy serves as an important reference in the batch fabrication of large-area VCSELs.