1.
Introduction
Semiconductor lasers, due to their portability, efficiency, simplicity of structure and easy-tuning characteristic, are widely used in many fields, such as atomic clocks, quantum measurement, highly stabilized microwave oscillators, and high precision spectroscopy and sensors. Semiconductor lasers were invented in 1962[1, 2], and they were successfully used in optical communication systems in 1978[3]. Fully functioning semiconductor lasers are not independent components but are composed of several inter-related parts such as laser diode and driver. The core part of a laser is the diode, which generates a stimulated emission light source with a small amount of stable injecting current provided by the driver. In addition, three terms are required in producing stimulated emission in semiconductor lasers, which are population inversion, resonant cavity, and gain medium. With an appropriate forward bias voltage on both ends of a PN junction, carriers reverse their distributions, and electrons in higher energy bands are more than those in lower ones. Photons can be emitted when electrons transit downward, then amplified, and the frequency is selected by the resonant cavity, forming a strong light beam with the same direction of propagation, frequency, and phase, that is, laser light[4].
Free-running semiconductor lasers are influenced by many factors, such as changes of atmospheric pressure, temperature fluctuations, external magnetic field, and mechanical vibration. For instance, if the change of working temperature is one degree Celsius, the wavelength of lasers in 1550 nm would change 3 × 10?10 m[5]. Similarly, if the change of driving current is one ampere, the wavelength of the same lasers would change 3 × 10?15 m. The frequency stabilization of semiconductor lasers is also affected by spontaneous emission and carrier density fluctuations[6].
In practice, the range of frequency fluctuation of semiconductor lasers is larger than the natural line width, resulting in broadening of the laser line-width and decrease of frequency stability. Therefore, the establishment of an appropriate frequency stabilization system can improve the frequency stability and reduce the frequency fluctuation of output for narrowing the line-width of lasers.
In this paper, several techniques for improving the frequency stabilization of semiconductor lasers are reviewed. In Section 2, basic proxies of evaluating the degree of stability and the basic system of active frequency stabilization are presented. In Section 3, the discussion is focused on three promising methods for achieving frequency stabilization, and experimental results on three subjects are presented. Section 4 introduces the development tendency of novel frequency stabilization. The methods of frequency stabilization will be used in combination rather than using alone. In addition, PID electronic control for optimizing the servo system will be added in these methods of frequency stabilization. Advantages and disadvantages of these three methods are also summarized.
2.
Principle
Frequency stability and repeatability are two proxies generally used to evaluate the degree of stability and determine the quality of frequency stability of lasers.
Laser frequency stability is the ratio of the average frequency (


$${{S}} = frac{{bar upsilon }}{{{ m{Delta upsilon }}}}.$$ ![]() | (1) |
The larger the frequency variation (

$${{{S}}^{ - 1}} = frac{{{ m{Delta upsilon }}}}{{bar upsilon }}.$$ ![]() | (2) |
According to the length of the sampling time, frequency stability is specified as long-term and short-term stability. Generally, the sampling time of the former is larger than 1 s, the latter is smaller than 1 s.
Frequency repetition describes the accuracy of repetition or reproduction of lasers at different times and locations, that is:
$${R} = frac{{{ m{delta upsilon }}}}{{bar upsilon }},$$ ![]() | (3) |
where


The fluctuation of laser frequency, like the frequency stability, can be quantitatively evaluated too. According to the principle of lasers, frequency oscillation is influenced by the frequency of the atomic transition spectral line νm and optical resonant frequency νc. If the atomic transition linewidth is Δνm, the width of resonant cavity is Δνc, then lasers oscillation frequency is expressed as:
$${upsilon = }frac{{{upsilon _{ m m}}{upsilon _{ m c}}left( {Delta {upsilon _{ m c}} + Delta {upsilon _m}} ight)}}{{{upsilon _{ m c}}Delta {upsilon _{ m c}} + {upsilon _{ m m}}Delta {upsilon _{ m m}}}},$$ ![]() | (4) |
where the Doppler linewidth of
m m}}$

m c}}$

$${upsilon = }{upsilon _{ m c}} + left( {{upsilon_{ m m}} - {upsilon_{ m c}}} ight)frac{{Delta {upsilon_{ m c}}}}{{Delta {upsilon_{ m m}}}}.$$ ![]() | (5) |
As shown in the above equation, the frequency of the laser is determined by the frequency of atomic transition spectrum and the resonant frequency of the resonator. The effect of the spectral line on the frequency of the laser is caused by frequency traction, which is described by the second item in the right side of the equation. This effect is generally so small (its magnitude is one percent of the first item) that it can be ignored. Thus, the stability of laser frequency is mainly determined by the stability of the resonant frequency of the resonator, which is easily affected by the environment.
Neglecting the effect of the atomic transition spectrum frequency, the frequency of free-running lasers operating at the base mode is
$${upsilon = }{{q}}frac{c}{{2nL}},$$ ![]() | (6) |
where n is the average refractive index of the resonant cavity, L is the cavity length of the resonator, c is the speed of light in a vacuum, and Q is the order of the mode. If the length of the cavity L or the average refractive index n changes, the frequency of lasers oscillation will change.
$$Delta {upsilon} = - {{qc}}left( {frac{{Delta L}}{{2n{L^2}}} + frac{{Delta n}}{{2L{n^2}}}} ight) = - {{q}}frac{c}{{2nL}}left( {frac{{Delta L}}{L} + frac{{Delta n}}{n}} ight).$$ ![]() | (7) |
That is:
$$left| {frac{{Delta {upsilon} }}{{upsilon} }} ight| = frac{{Delta L}}{L} + frac{{Delta n}}{n}.$$ ![]() | (8) |
So, in order to keep the laser frequency stable, it is necessary to maintain the stability of the length or the refractive index of the cavity. This is the fundament of designing semiconductor lasers with good stability in frequency.
The main method of frequency stabilization of lasers can be divided into passive frequency stabilization and active frequency stabilization according to the existence of the frequency discrimination system. Passive frequency stabilization is mainly achieved by improving the external environment of lasers, such as stabilizing input current of lasers, isolating vibration and fixing the temperature. With this method, the frequency stability can reach 10?8 but it is difficult to achieve better results. So active frequency stabilization technology became of use.
Active frequency stabilization needs a specific external reference frequency to set up a frequency stabilization system and to complete the closed loop of frequency stabilization. When external factors deviate the output frequency of lasers from the reference frequency, the frequency discriminator will give an error signal to the frequency stabilization system, and the servo control circuit sends feedback to lasers on the piezoelectric ceramic which is pasted on the surface of the laser cavity. Then the piezoelectric ceramic is slightly deformable as the result of the converse piezoelectric effect. The piezoelectric ceramic controls the length of the laser cavity through the change of voltage, so that the laser frequency goes back to the reference frequency. Fig. 1 is the basic system structure of frequency stabilization of semiconductor lasers.

class="figure_img" id="Figure1"/>
Download
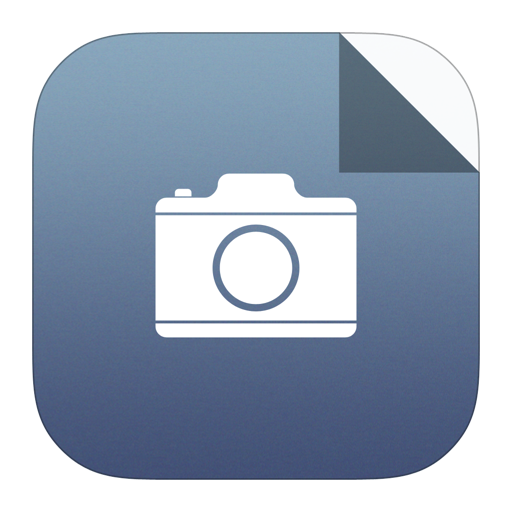
Larger image
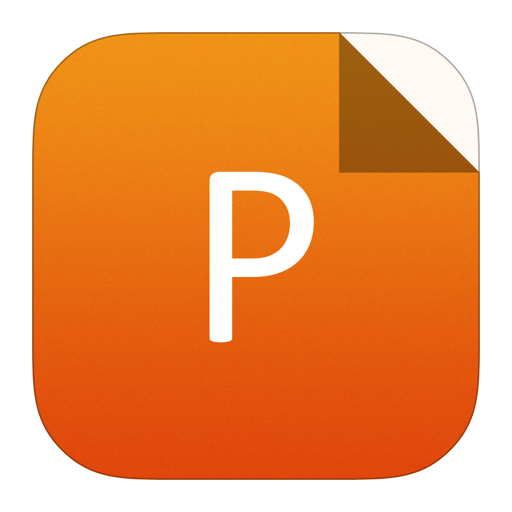
PowerPoint slide
Figure1.
(Color?online)?Basic system of active frequency stabilization.
The light emitted by the semiconductor laser is separated into two parts, one as the output light, and the other one is input to the discriminator. When the laser frequency deviates from the reference frequency, the frequency discriminator receives signals from the frequency discrimination, and transmits them to the servo control system. The frequency of these signals matches the output frequency of the laser with the reference frequency and processes of frequency stabilization are accomplished.
3.
Frequency stabilization methods
Active frequency stabilization, based on the differences of frequency discrimination curves, can be specified in three types: harmonic frequency stabilization, PDH frequency stabilization, and curve subtraction frequency stabilization.
3.1
Harmonic frequency stabilization
The harmonic frequency stabilization is also called derivative spectrum stabilization. This system utilizes the odd order differential locking technology to lock transmitted light[7] that travels through the absorption cell or the F–P cavity, which represent the two main harmonic frequency stabilization methods.
3.1.1
Molecular absorption harmonic frequency stabilization
Fig. 2 is a typical structure diagram of a system of molecular absorption harmonic frequency stabilization. As it shows, a sine wave modulation is added in the control voltage of the piezoelectric ceramic, which is on the laser chip or in the driving current of the laser. The curve of odd-order differential quotient of the modulated output light, which is obtained through the gas cell, is distributed anti-symmetrically[8]. For instance, on each side of the reference frequency, the curve distributes on the positive or negative half axis of the vertical coordinate, respectively. Thus, the position of laser frequency whether it is in the reference frequency’s left or right side can be distinguished according to the curve. Then, the offset of laser frequency is set to the servo-feedback-control system to control the length of the cavity for realizing frequency stabilization[9].

class="figure_img" id="Figure2"/>
Download
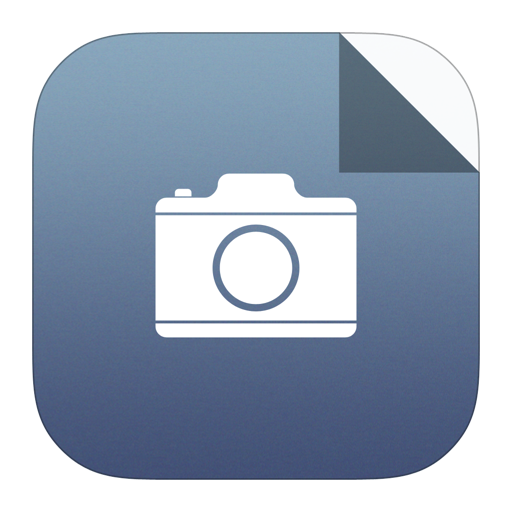
Larger image
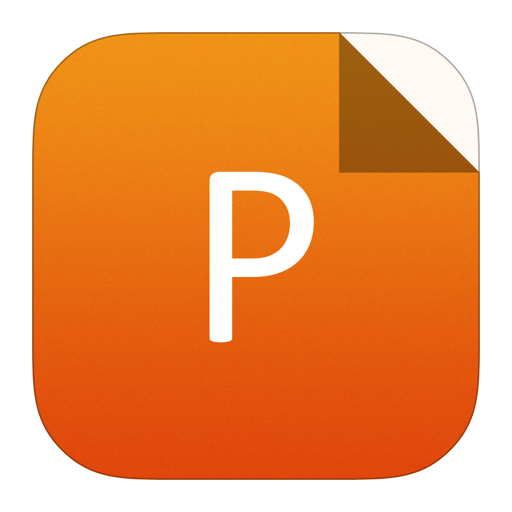
PowerPoint slide
Figure2.
(Color?online)?Molecular absorption harmonic frequency stabilization system structure. LD: laser diode, PZT: piezoelectric ceramic transducer, Ω: modulation frequency.

class="figure_img" id="Figure3"/>
Download
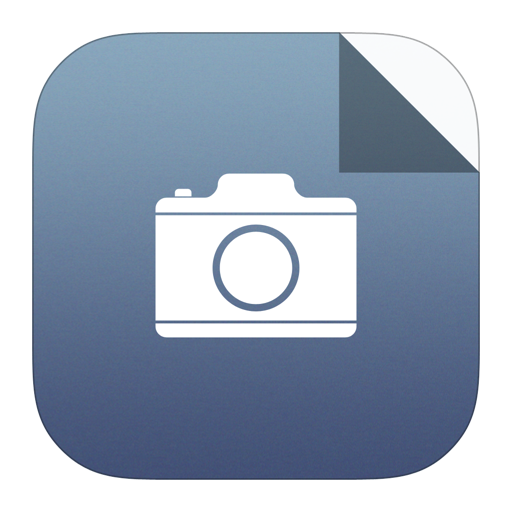
Larger image
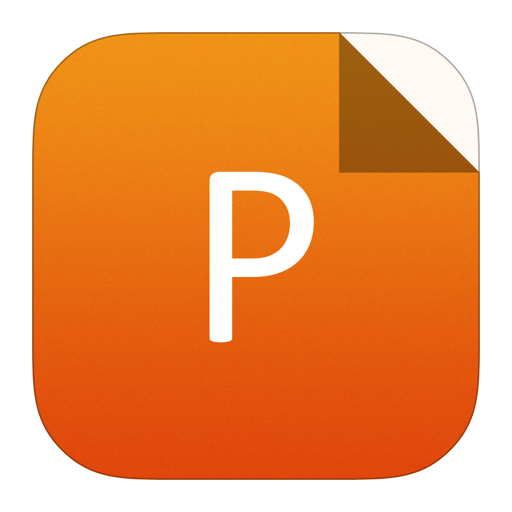
PowerPoint slide
Figure3.
(Color?online)?Harmonic frequency stabilization based on F–P system structure. EOM: electro-optic modulator, F–P: Fabry-Perot, PD: photoelectric detector.
This method is a traditional frequency stabilization scheme. It is relatively more complex and expensive, but it can achieve higher stability of frequency. In Ref. [10], an iodine molecule Doppler-broadened absorption technology is combined with the digital proportion integration differentiation (PID) feedback control system to stabilize the frequency of laser, where MgO:PPLN is used as the frequency doubling crystal. The Nd:YAG laser frequency shift is stable at 1.2 MHz/h with a stability of 4.26 × 10?9, which can be further improved if the temperature of iodine pool can be controlled to reduce the saturated vapor pressure of iodine. However, this operation will increase the complexity of the system.
3.1.2
Harmonic frequency stabilization based on F–P cavity
Harmonic frequency stabilization based on the F–P cavity system is similar to the system with an absorption cell. The intensity of the light which transmits through the F–P etalon is related to the light frequency. Thus, the curve of the odd-order differential quotient of the transmission intensity could be used as the frequency discrimination curve. Compared with the absorption cell system, the F–P etalon system is insensitive to air pressure.
The transmission light intensity can be derived from the incident light intensity[11]:
$${I_t} = frac{1}{{1 + {F{ m {sin}}^2}frac{varphi }{2}}}{I_0},$$ ![]() | (9) |
where I0 is the intensity of incident light; It is the intensity of transmission light; φ is a phase factor φ = 2ndω0/c;
ight)}} = frac{4}{pi }{f^2}$

The curve of frequency discrimination is the derivation of transmission light intensity It.
When the F–P free spectral range (FSR) is 375 MHz and the fineness f is 400, the obtained curve of frequency discrimination is as Fig. 4 shows. In such a case, the control range is relatively wide, that is greater than 100 MHz. However, the signal is rather weak and the ability of noise suppression is poor. Thus, the signal of frequency discrimination needs to be intensified by an amplifying circuit. In this case, the noise will also be amplified and the frequency stability will be affected at the same time.

class="figure_img" id="Figure4"/>
Download
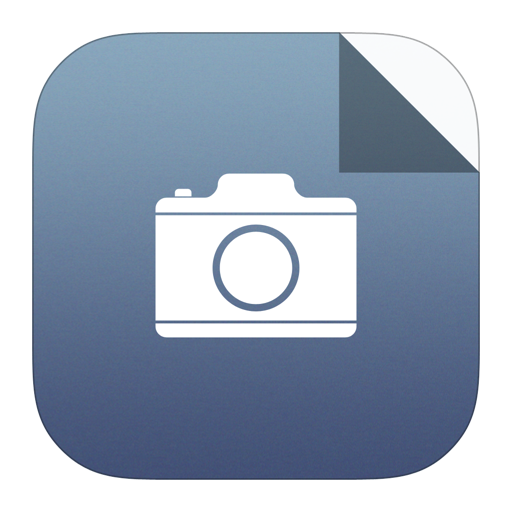
Larger image
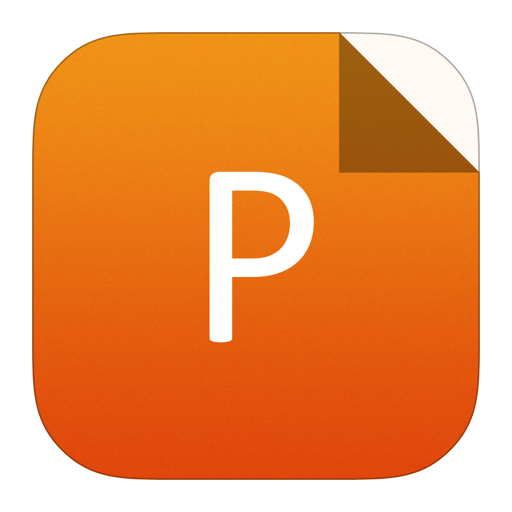
PowerPoint slide
Figure4.
The curve of frequency discrimination of harmonic frequency stabilization based on F–P [11].
Liu and Liang used a microcontroller MSP430, F–P confocal interferometer, current control, and temperature control system to build a harmonic frequency stabilization based on the F–P system[12]. The system could achieve laser light modulation through adding an audio scanning voltage to the interferometer, and the frequency offset can be obtained from the phase-locked amplifier. Through controlling the working temperature and driving current of lasers with the servo system, frequency stabilization can be achieved. This method is not only simple in structure, but also no band limit, with an automatic controlling frequency stability of 10?8.
3.2
PDH frequency stabilization
The curves of frequency discrimination of PDH frequency stabilization are the curves of absorption or dispersion which are obtained by using a spectral technique of phase modulation[13]. There are two typical methods as follows.
3.2.1
Edge frequency locked frequency stabilization based on F–P
The typical structure of the edge frequency locked frequency stabilization system based on F–P is as shown in Fig. 5. Firstly, laser frequency is modulated to produce two opposite amplitude sidebands which are distributed on both sides of the laser center frequency[14]. When the frequency of laser deviates from the reference frequency, the amplitude and phase of the two sidebands will change after the reflection of the F–P etalon. The beat signal is not zero, and the frequency of signals exported from the detector is equal to the modulation frequency. Then, the signal is locked in the resonance frequency of the F–P cavity through a servo system to achieve frequency stabilization. This system has a strong anti-interference ability, high sensitivity and high signal-to-noise ratio. Meanwhile, the adjustment range is very large which is hard to lose lock[15].

class="figure_img" id="Figure5"/>
Download
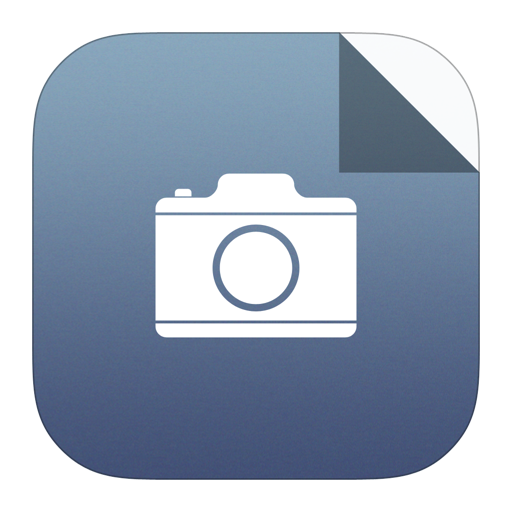
Larger image
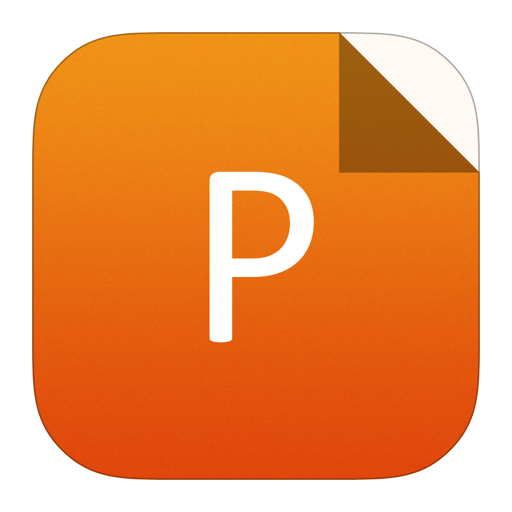
PowerPoint slide
Figure5.
(Color?online)?Edge frequency locked frequency stabilization based on F–P system structure. PBS: polarization-beam-splitter cube.
Webster and Oxborrow used a side frequency locking system to stabilize the seed light of an injection locked laser. The maximum stability has reached 10?15[16]. After frequency stabilization, the laser line-width is 0.46 Hz. This system is an ideal frequency stabilization system and a more widely used frequency stabilization method based on F–P. In addition, the effect of temperature, sound and vibration can be reduced by hanging the F–P etalon in a vacuum.
3.2.2
Modulation transfer spectroscopy stabilization
The typical system structure is as shown in Fig. 6. There are two beams of light, one is not modulated and the other is modulated. They pass the nonlinear medium reversely through a four wave mixing process. Then, a new sideband is generated from the original unmodulated light beam. The unmodulated beam and the newly generated edge band are beat on the fast photodetector. The absorption and dispersion signals of the nonlinear medium and their combinations can be obtained by the phase sensitive detection[17]. Using the transfer technique of optical heterodyne modulation, the hyperfine spectral structure with high signal to noise ratio (SNR) and removed Doppler background can be obtained. It is also used to achieve high precision of laser frequency locking[18]. Bi and Liu used spectroscopy stabilization of modulation transfer to obtain a hyperfine spectrum in iodine molecular transition, which has an ultrahigh signal to noise ratio structure[19]. Its frequency stability is better than 10?12. However, the structure is complex and cost is high, thus it is not suitable for widespread application[19].

class="figure_img" id="Figure6"/>
Download
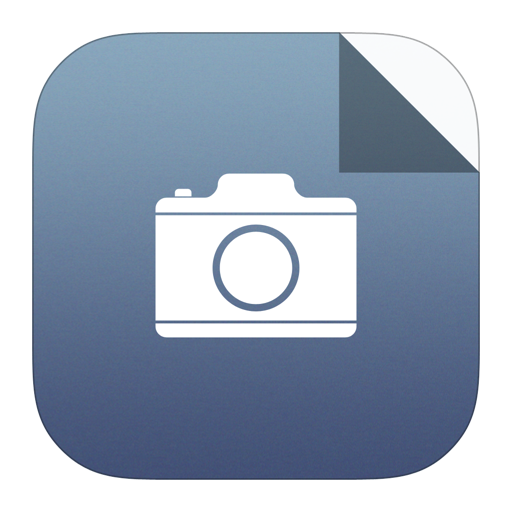
Larger image
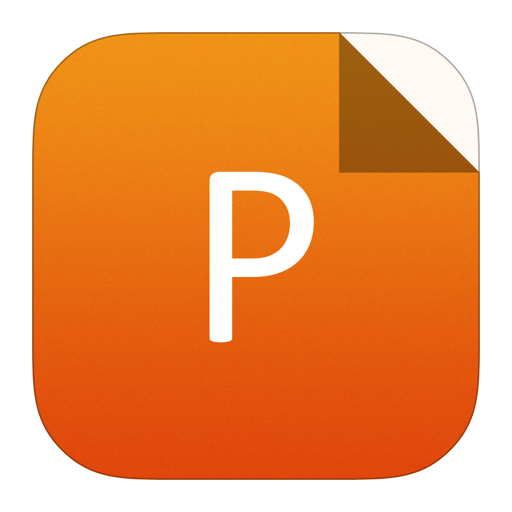
PowerPoint slide
Figure6.
(Color?online)?Modulation transfer spectroscopy stabilization system structure. AOM: acousto-optic modulator.
3.3
Curve subtraction frequency stabilization
The curve subtraction frequency stabilization is based on the principle of curve subtraction[20]. Two misplaced curves are obtained by different kinds of methods. Then the curve of frequency discrimination is obtained by subtracting the two curves through the subtraction circuit. There are three kinds of representative methods:
3.3.1
Modulation-free frequency stabilization based on a confocal F–P cavity
The typical structure of this method is shown in Fig. 7, two beams with a small included angle light and the same frequency are injected into a confocal F–P cavity, which has different resonance frequency for these two beams[21]. When the laser frequency is linear scanned, there is a relative frequency shift between the center of two transmission peaks. Therefore, the curve of cavity transmission can be obtained. The projection signals detected by the two same types of photodetectors are subtracted by the subtractor, and the curve which is similar to the dispersion curve is obtained as the frequency discrimination signal of the laser.

class="figure_img" id="Figure7"/>
Download
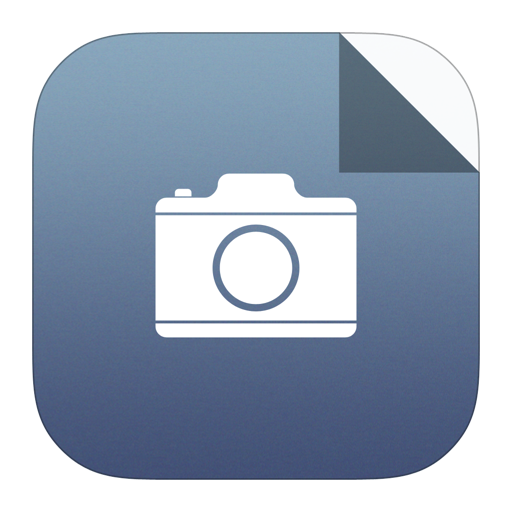
Larger image
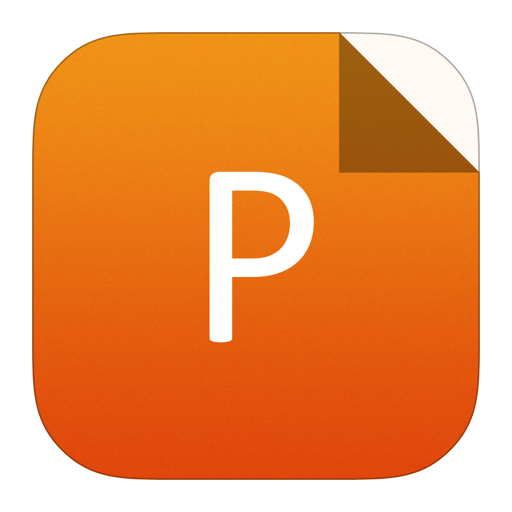
PowerPoint slide
Figure7.
(Color?online)?Modulation-free frequency stabilization based on a confocal F–P cavity system structure. ECDL: external-cavity diode laser; HP: half-wave plate; CFP: confocal Fabry–Perot cavity; P–I: proportion and integration amplifier.
Yang and Wang achieved a grating feedback semiconductor laser whose wavelength is 852 nm with no modulating of frequency locking based on the F–P cavity[22]. After locking, the frequency fluctuation decreased from 10 MHz to 340 kHz. Its stability is 10?12.
This method can be implemented easily. The laser output of no modulation disturbance can be obtained with no amplifier. However, the curve of frequency discrimination is directly obtained from the transmission curve of the cavity. Thus, the choice of cavity is quite strict[23].
3.3.2
Dichroic atomic vapor laser lock (DAVLL)
The typical system structure of the method is shown in Fig. 8. The absorption chamber is placed in a magnetic field. The absorption line is split into left-handed and right-handed polarized light when it propagates along the longitudinal direction of the magnetic field. According to the difference of the intensity of the two circularly polarized lights, the direction of laser oscillation frequency and the deviation from the center frequency can be determined. So that a control signal can be formed to adjust the resonator and stabilize it at the center frequency of the spectral line[24–26].

class="figure_img" id="Figure8"/>
Download
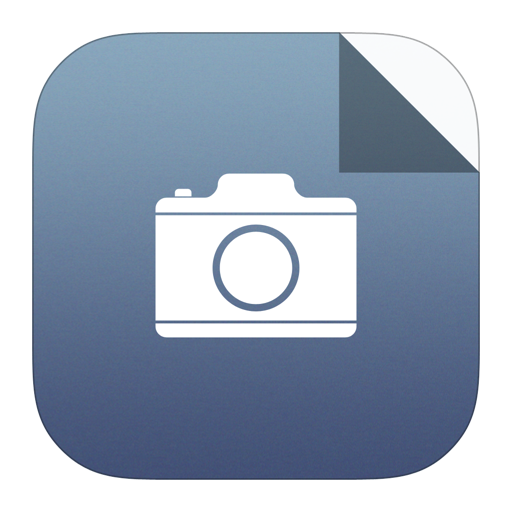
Larger image
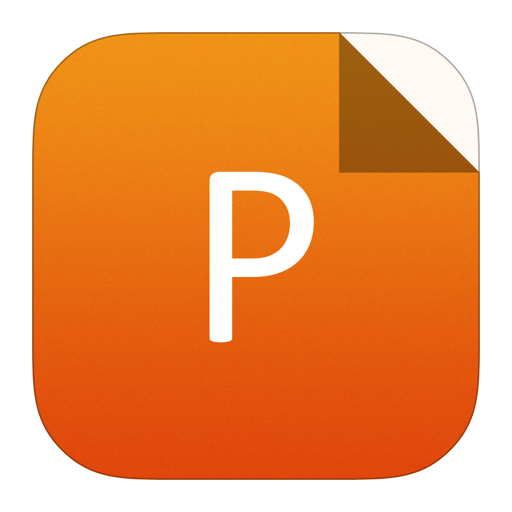
PowerPoint slide
Figure8.
(Color?online)?Dichroic atomic vapor laser lock (DAVLL) system structure.
A semiconductor laser of DFB 894.6 nm and Cs atomic gas chamber are used to build a DAVLL experimental device by Liu and Zhuo[27]; the experimental schematic diagram is shown in Fig. 9. The DAVLL spectra under different magnetic field conditions were also measured. It is confirmed that the intensity of magnetic field only affects the curve slope of the zero point of the DAVLL spectrum, but it does not affect the frequency on zero point. According to the frequency locking principle, the temperature control circuit and current control circuit of the semiconductor laser are made. Its frequency stability is 10?8.

class="figure_img" id="Figure9"/>
Download
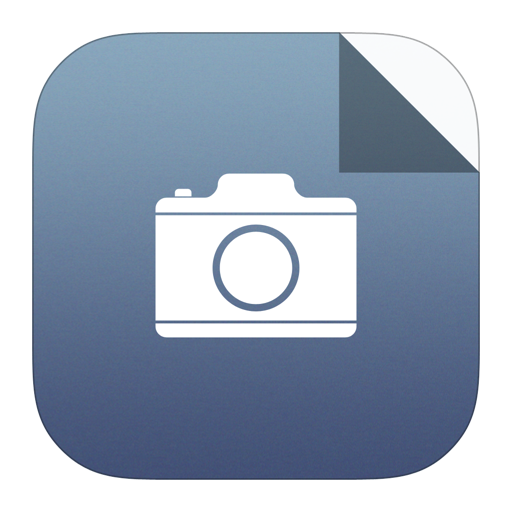
Larger image
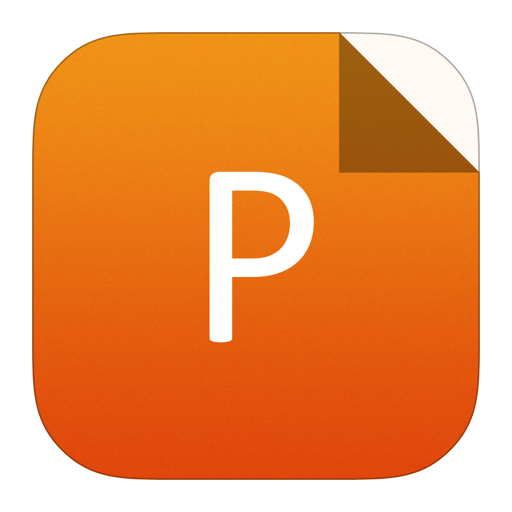
PowerPoint slide
Figure9.
(Color?online)?Experimental schematic diagram of DAVLL.
The DAVLL frequency stabilization system belongs to the modulation free frequency stabilization, which does not cause additional interference to the frequency of lasers. The system scheme is simple and feasible. Without the lock-in amplifier, the output of laser with no modulating disturbance can be obtained. Meanwhile, the continuous tunable range is wider than that of the saturated absorption method. However, DAVLL needs a specific absorption line. The application scope of DAVLL is limited. It does not eliminate Doppler broadening. Researchers need to control the temperature and shield the magnetic field for stabilizing the system. The stability is not ideal, which is about 10?9.
3.3.3
Doppler-free dichroic lock (DFDL)
The typical structure of this method is shown in Fig. 10. It is a frequency stabilization by combining the Zeeman effect and saturated absorption.

class="figure_img" id="Figure10"/>
Download
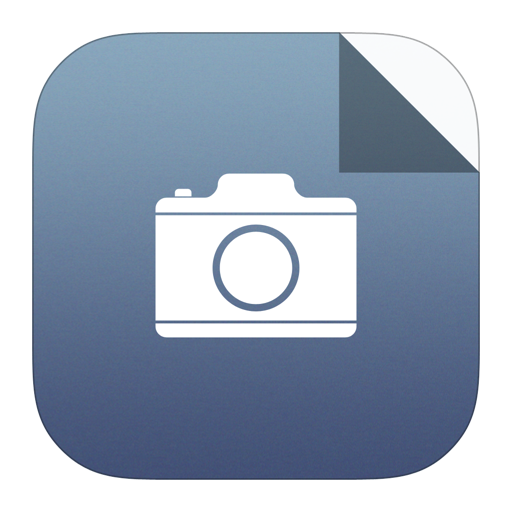
Larger image
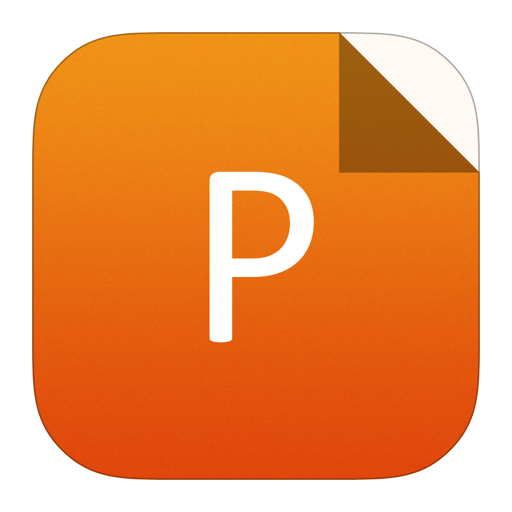
PowerPoint slide
Figure10.
(Color?online)?Doppler-free dichroic lock (DFDL) system structure.
On the basis of the DAVLL optical path, SUN Li added a group of 1/4 slides, a polarization-beam-splitter cube (PBS), a pair of mirrors, and a splitting prism BS to form the system of DFDL frequency stabilization. After testing, the stability of frequency is 10?10.
The tuning range of this method is smaller than DAVLL, but it eliminates the influence of Doppler broadening on the spectrum. It stabilizes semiconductor lasers in any saturated absorption peak of the atomic hyperfine spectrum, and there is a relatively wide range of signal[28].
Compared with the frequency stabilization based on saturated absorption, a Doppler-free dichroic lock system is not sensitive to the noise of light intensity and it is hard to lose the lock. Its light path is simple and feasible. The output frequency of this method has no modulation disturbance, but it still needs a specific absorption line to match the frequency. Therefore, its application is also very limited. Though its stability is higher than DAVLL, it is more sensitive to the intensity of magnetic field, because the slope of the difference signal is greatly influenced by the intensity of the magnetic field[29].
4.
Conclusion and discussion
Three typical methods of frequency stabilization are overviewed in this paper. All of the three methods have been developed maturely and have been the basis of the common frequency stabilization technology at present. The main idea is to use a certain frequency as the reference standard for frequency stabilization.
Table 1 summarizes the relevant information of the three methods which is mentioned above. From the point of modulation, the direct frequency modulation of lasers would introduce additional frequency noise which affects the frequency stabilization accuracy. Therefore, the method of external modulation or no modulation is used to obtain the output light source without frequency disturbance, which is the development trend of the laser frequency stabilization. This kind of method is simple and easy to build. If the precision of frequency stabilization is firstly considered, the atomic spectrum line as a reference frequency has a good stability, which is more common in practical applications. This method could be used in laboratories except spacelab, because the atomic spectrum line is unstable in space radiation. Thus, the frequency stabilization of space lasers should use stable methods in space, such as the methods based on F–P cavity. From a comprehensive point of view, the methods based on saturated absorption and F–P standard are currently the most popular method of frequency stabilization. However, saturated absorption has good long-term stability and poor short-term stability. So, the methods based on saturated absorption or F–P cavity are not used separately[30]. Researchers generally combine them with other methods, such as PID electronic control for optimizing the servo system. Such measures could be very useful to improve the stability of frequency stabilization precision. They could also improve the laser performance of anti-jamming. These comprehensive methods have good prospects for development[31–33].
The methods of frequency stabilization | Advantages and disadvantages |
Harmonic frequency stabilization | |
Molecular absorption harmonic frequency stabilization Harmonic frequency stabilization based on F–P cavity | Complex system structure, the frequency stability is 10?9[10] Loud noise, weak signal, wide frequency stabilization controlling, simple structure, the frequency stability is 10?8[12] |
PDH frequency stabilization | |
Edge frequency locked frequency stabilization based on F–P Modulation transfer spectroscopy stabilization | Strong anti-interference ability, frequency stability 10?15[16] Complex system structure, high cost, high precision of frequency locking, the frequency stability is 10?12[19] |
Curve subtraction frequency stabilization | |
Modulation-free frequency stabilization based on a confocal F–P cavity | Rely on cavity material, simple structure, frequency stabilization without modulation dither, frequency stability 10?12[22] |
Dichroic atomic vapor laser lock(DAVLL) Doppler-free dichroic lock(DFDL) | Low precision of frequency lock, sensitive system, easy to lock, the frequency stability is 10?8[27] Very sensitive to the intensity of magnetic field, restricted application, simple light path, the frequency stability is 10?10[28] |
Table1.
Advantages and disadvantages of three methods.
Table options
-->

Download as CSV
The methods of frequency stabilization | Advantages and disadvantages |
Harmonic frequency stabilization | |
Molecular absorption harmonic frequency stabilization Harmonic frequency stabilization based on F–P cavity | Complex system structure, the frequency stability is 10?9[10] Loud noise, weak signal, wide frequency stabilization controlling, simple structure, the frequency stability is 10?8[12] |
PDH frequency stabilization | |
Edge frequency locked frequency stabilization based on F–P Modulation transfer spectroscopy stabilization | Strong anti-interference ability, frequency stability 10?15[16] Complex system structure, high cost, high precision of frequency locking, the frequency stability is 10?12[19] |
Curve subtraction frequency stabilization | |
Modulation-free frequency stabilization based on a confocal F–P cavity | Rely on cavity material, simple structure, frequency stabilization without modulation dither, frequency stability 10?12[22] |
Dichroic atomic vapor laser lock(DAVLL) Doppler-free dichroic lock(DFDL) | Low precision of frequency lock, sensitive system, easy to lock, the frequency stability is 10?8[27] Very sensitive to the intensity of magnetic field, restricted application, simple light path, the frequency stability is 10?10[28] |