1.
Introduction
Nowadays, intense research effort is focused on exploring alternative emerging devices beyond CMOS to perform a binary logical function[1] and special computational operation[2]. One promising device technology type among them is nanomagnetics, such as magnetic quantum cellular automata (MQCA, also called nanomagnet logic, NML)[3–5], domain wall (DW)[6], magnetic tunnel junction (MTJ)[7], and spin transfer torque (STT)[8], etc. The main reason for the interest in these nanomagnetic switches is their nonvolatility and comparably low power consumption in combination with the ability to perform logic and storage in one single element[9].
In nanomagnetics, the NML denotes a relatively smart, but operation-effective structure and mechanism. The basic element of NML is sub-100 nm size single domain magnet (see Fig. 1(a)). Usually, the x–y direction defines the in-plane dimension, while the z axis direction depicts the out-of-plane dimension (thickness). The in-plane magnetizations along the y axis (easy axis) are used to encode binary logic states ‘1’ (up) and ‘0’ (down), respectively; while along the x axis (hard axis) denote ‘null’ logic[4] (see Fig. 1(b)). The logic operation and data transmission in NML are realized by means of dipole-coupled magnetostatic interactions.

class="figure_img" id="Figure1"/>
Download
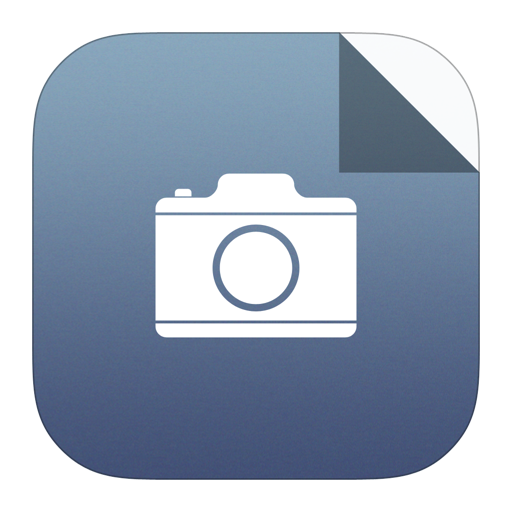
Larger image
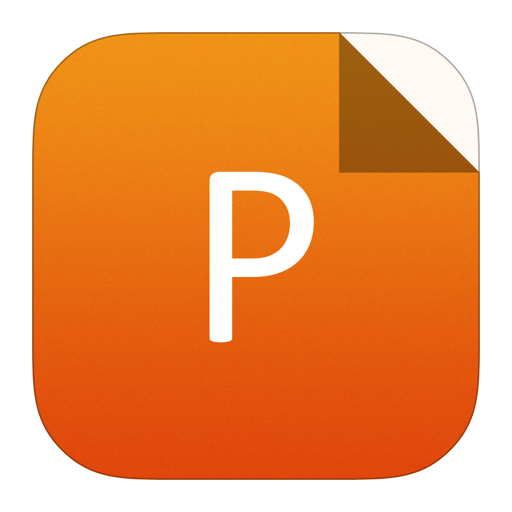
PowerPoint slide
Figure1.
(Color online) The NML device and edge imperfection defect description. (a) Three dimension model of NML device. (b) The definition of NML logic state. (c) Edge imperfection defect modeling and four kinds of defects. (d) The scanning electron microscopy (SEM) image of defective nanomagnet.
To date, there are some candidate fabrication methods to realize nanomagnet-based logic devices[4, 10]. However, although the advanced technologies are used in the NML manufacturing, defects are still likely to occur at such small size[11], one representative fabrication imperfection is the major single missing edge or corner defect[12]. In Refs. [5, 13], the single missing edge defect of magnet in coupled nanomagnet pair had been succinctly explored. However, some basic NML components subjected to a fabrication defect have not been carefully considered until now. We herein employ a triangle to describe the missing corner materials region (see Fig. 1(c)), α = 45°, dv and dh represent the vertical and horizontal-direction missing amplitude, respectively. For one NML device, there are four kinds of possible edge missing defects, namely NLU, NRD, NRU, NLD.
Interconnect wire is a very important component of any NML circuit since it forms a nanomagnet’s data transmission channel. However, unlike the cooper wire making up an interconnect in transistor based CMOS technology, the interconnect in NML technology is consisted of many nanomagnets. Fig. 2 shows an on-chip NML circuit containing several interconnects, where i represents the input of the circuit, O represents the output of the circuit. From Fig. 2, we can see that there are three interconnect wires INT1, INT2 and INT3 in this circuit, which complete logic propagation between four NML gates.

class="figure_img" id="Figure2"/>
Download
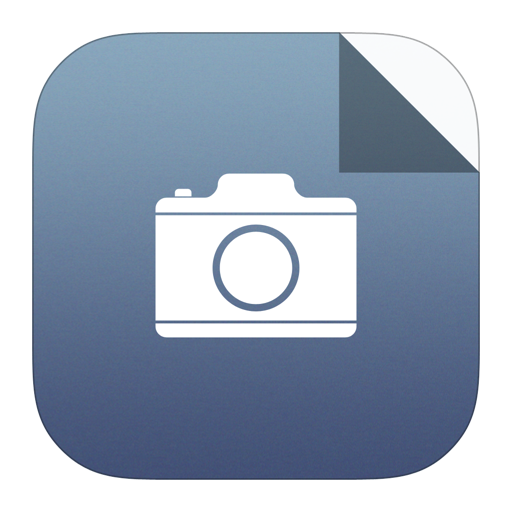
Larger image
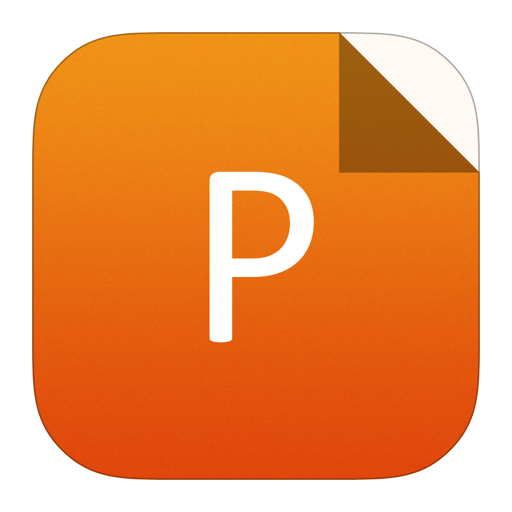
PowerPoint slide
Figure2.
(Color online) An on-chip NML circuit layout containing several interconnect wires.
As stated above, the NML interconnect is constructed by arranging nanomagnets in a series. Consequently, once the magnet defect occurs, whether the interconnect or data channel is reliable becomes a major concern. Motivated by this issue, this paper will evaluate errors for NML wire due to the magnet’s imperfection (missing edge defect) and discuss how different design parameters affect the switching of a typical interconnect.
2.
Preliminary simulations
2.1
Edge missing effect of NML device
In order to complete the logic operation in the NML circuitry, an external magnetic field is usually required to place all the nanomagnets in the interconnect wire into the metastable state (‘null’ logic) for re-evaluation, this external field is called the clocking (Hclock). However, for an isolated nanomagnet with a moderate slanted edge (see Fig. 1(c), the device N), it cannot preserve into a metastable state as the defect-free device does after the removal of the clocking, but demonstrates a preferred magnetization orientation (binary logic state)[12]. Certainly, what state the missing corner defect nanomagnet without a driver device will ultimately relax to (namely pointing down or up) is determined by and relative to the position of the slant and the direction of the clocking field. For example, the nanomagnets NLU and NRD will all point up after the removal of a clocking field toward right, and they would point down after the removal of a clocking field toward left.
2.2
Simulation method
The study throughout the paper will consider NML interconnect wire containing one defective nanomagnet mentioned above, and the missing corner or edge position of the defective nanomagnet is determined by the direction of Hclock and the position of defective nanomagnet. This can be clearly explained by using the typical case appearing in Fig. 3. It is very important to note that the nanomagnet NB in Fig. 3 represents the driver device (generating an equivalent dipole field as the output nanomagnet of the last segment), which can provide an input logic state for the NML wire. For example, with an application of Hclock toward right, suppose that the wire is defect-free, the driver device NB will set the nanomagnet N1 into logic ‘0’ state. However, to evaluate errors of interconnect effectively, the NLU or NRD-type nanomagnet, generating a reverse logic state ‘1’ after the removal of Hclock toward the right, is placed in the position of N1 to test the edge missing effect. Similarly, the NRU or NLD-type nanomagnet is placed in the position of N2 to observe defect-related switching. Namely, if the Hclock toward the right is adopted, the NLU or NRD-type device will be placed in the odd number position of the NML interconnect, while the NRU or NLD-type device will be placed in the even number position of NML wire to evaluate errors. Moreover, DS denotes the nanomagnet spacing in the NML wire, and the block PB is used to eliminate spontaneous switching of the last nanomagnet in the interconnect due to thermal noise[14].

class="figure_img" id="Figure3"/>
Download
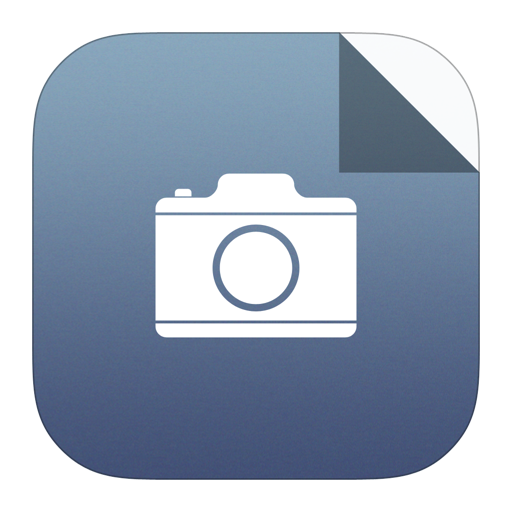
Larger image
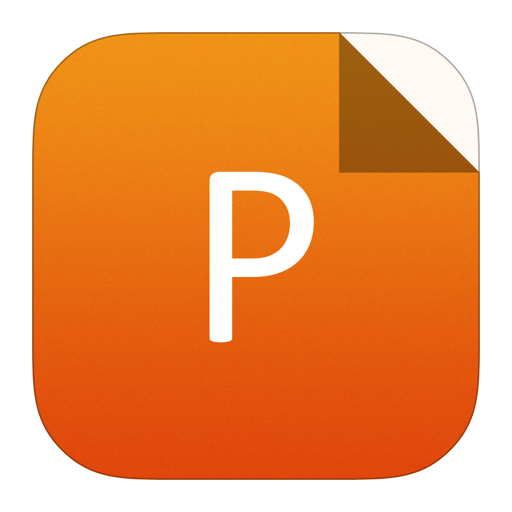
PowerPoint slide
Figure3.
The NML interconnect wire containing one defective nanomagnet. (a) The NLU or NRD-type defective nanomagnet interconnect wire. (b) The NRU or NLD-type defective nanomagnet interconnect wire.
2.3
A case of typical interconnect wire
A typical five-nanomagnet length straight wire NML interconnect[15] including one defective device is considered in the present work. Note that the driver device has a size of 60 × 30 × 30 nm3, while all the interconnect elements have the size of 60 × 100 × 30 nm3. The defective nanomagnet has variable missing amplitudes ranging from 0 nm to 10 nm (with a step of 0.5 nm). Moreover, a thermal stability ratio KuV/kBT (300K) of 10[16] is included. That is to say, we introduce a shape-defined uniaxial anisotropy constant Ku of 25 kJ/m3 to lower spontaneous magnetization. The object oriented micro-magnetic framework (OOMMF)[17] tool is chosen to simulate a defect-related final magnetization state, and the critical edge missing amplitude is recorded. In the simulation, a damping constant of 0.5, an 180 mT clocking field Hclock toward the right and a 2-D periodic boundary condition are used. The preliminary simulation results on the typical five-nanomagnet length interconnect wire in a Permalloy material implementation are shown in Fig. 4.

class="figure_img" id="Figure4"/>
Download
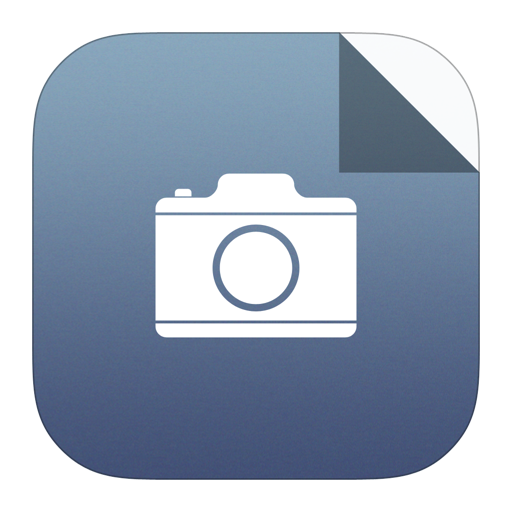
Larger image
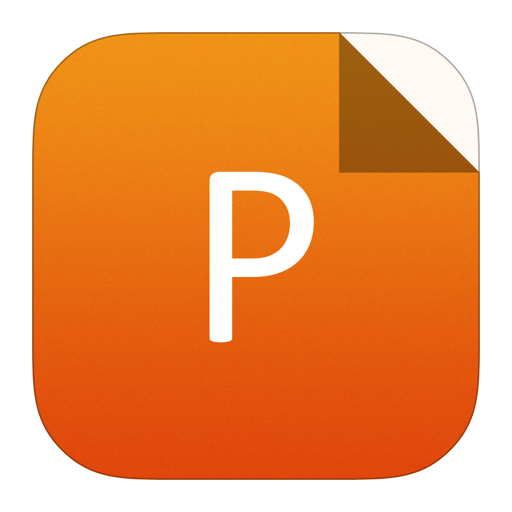
PowerPoint slide
Figure4.
(Color online) Simulation results of interconnect wire shown in Fig. 3. (a) Tolerable edge missing amplitude of different nanomagnet. (b) The initial magnetization with the application of Hclock. (c) The final magnetization after evolvement.
From Fig. 4(a), we can see that when the edge missing amplitude of interconnect element N1 approaches 9 nm (region SA), the interconnect wire can still transmit the logic state correctly. Moreover, the element N2 can also tolerate an edge missing amplitude of 6 nm (region SB). These findings indicate that the NML interconnect wire demonstrates a moderate defect tolerance ability. To clearly display magnetization orientation of defective interconnect wire, Figs. 4(b) and 4(c) denote dynamical magnetization images with 8 nm edge missing amplitude (black circle). Fig. 4(b) shows the initial magnetization with the application of Hclock, while Fig. 4(c) shows the final magnetization direction after evolvement. From this figure, we can see that the logic ‘1’ state does obtain the correct forward transmission (see N5), although a moderate missing corner defect occurs in the interconnect element.
It should be noted that for simplicity, we have not studied the case where dh is not equal to dv. However, we predict that the volume of missing corner zone (determining shape anisotropy energy density) may be an important factor, and this will be considered in our future work. To validate the simulations, we herein still provide an experimental result shown in Fig. 5 that the wire transmits the logic state wrongly for comparison.
Fig. 5(a) depicts the SEM image of interconnect wire in which the first nanomagnet is subjected to 10 nm edge missing imperfection. From the magnetic force microscopy image (MFM) shown in Fig. 5(b), we can see that this defect induces logic state error. It should be noted that we cannot exhaust every defective interconnect wire image with the MFM test. But by experimental comparison, we found out that the edge missing amplitude exceeding the critical defect size does induce the signal propagation error. More experimental results can be found from our previous work, such as Ref. [18].
3.
Discussions
In this section, we will in detail investigate different device parameter effects of five-nanomagnet length interconnect wires subjected to edge imperfections, especially the magnetic material and device spacing effects. The goal of these simulations is to reproduce a random amplitude variation in the defective nanomagnet of NML interconnect and to evaluate its impact on the functional behavior of data transmission.
3.1
Simulation setup
The simulation algorithm is as follows: a 100 times simulation is executed using a random variable AF to represent the possible edge missing amplitude. The random variable AF is characterized by a Gaussian probability distribution function with mean value μ = i and standard deviation σ, where i is the edge missing amplitude. For example, if we will discuss the edge missing amplitude of 3 nm, then i = 3 nm, and the worst-case scenario (σ = 1 nm) is considered in the present work. The percentage of successful output is recorded. Success is judged based on the ability of the simulated interconnect wire to correctly transfer logic state.
3.2
Different magnetic material effects
Three kinds of common magnetic materials, namely Permalloy[19], Co[20] and Fe[21] are adopted here to construct interconnect wire. Their material parameters and the corresponding device size are summarized in Table 1. Note that the nanomagnet thicknesses are different, that is because a defect-free nanomagnet made of the abovementioned three materials at these thicknesses can demonstrate reliable switching.
Parameter | Permalloy | Co | Fe |
MS (A/m) | 8 × 105 | 10 × 105 | 17.5 × 105 |
A (J/m) | 10.5 × 10?12 | 13 × 10?12 | 21 × 10?12 |
Size (nm3) | 60 × 100 × 30 | 60 × 100 × 10 | 60 × 100 × 6 |
Table1.
Three materials parameters and device sizes.
Table options
-->

Download as CSV
Parameter | Permalloy | Co | Fe |
MS (A/m) | 8 × 105 | 10 × 105 | 17.5 × 105 |
A (J/m) | 10.5 × 10?12 | 13 × 10?12 | 21 × 10?12 |
Size (nm3) | 60 × 100 × 30 | 60 × 100 × 10 | 60 × 100 × 6 |
With an application of 180 mT clocking field toward the right, we observe the stable magnetization state of defective interconnect wires (30 nm spacing) for each nanomagnet element by incrementally increasing the corner missing amplitude from 1 to 10 nm, and the success rate for different defects is recorded. The statistical simulation results of three magnetic materials are shown in Fig. 5.

class="figure_img" id="Figure5"/>
Download
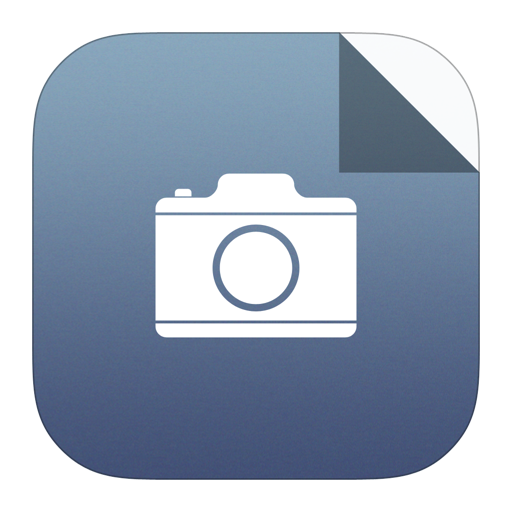
Larger image
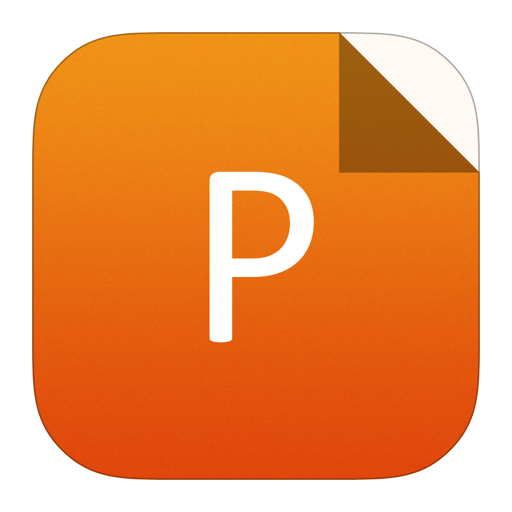
PowerPoint slide
Figure5.
(Color?online)?Experimental results of defective interconnect wire shown in Fig. 3. (a) SEM image of interconnect wire subjected to edge imperfection. (b) The magnetic force microscopy (MFM) image of defective interconnect wire.
From Fig. 6, we can see that the success rate curves of three kinds of different interconnect wires denote a similar trend. The first important phenomenon is that the corner defect amplitude below 5 nm shows a complete success rate of 100% regardless of magnetic materials, which indicates that the defect does not imposed any negative effect on data transmission. This may arise from intense dipole coupling interactions, which overcome reverse flopping caused by the preferred magnetization of the defective nanomagnet element, then the defective device in the interconnect wire outputs expected logic state as the corresponding element does in the defect-free NML interconnect. The second obvious observation is that as the defective interconnect element approaches the input terminal, it shows better missing corner defect tolerance ability, for example the nanomagnet element N1 in the Permalloy interconnect wire, which denotes a success rate of 100% at 8 nm corner missing defect. That is because of the “signal degradation effect” induced magnetization attenuation. Generally speaking, if one clocking zone in the interconnect wire accommodates too many nanomagnets, the average magnetization of the latter nanomagnet would decrease. Therefore, once the fabrication defect happens to the interconnect, then the element close to the input will generate a higher success rate, while the element far away from the input would introduce a lower success rate. Based on these observations, we suggest 9 nm critical amplitude as the manufacturing reference since N1, denoting the best fault tolerance ability, can tolerate edge missing amplitude up to 9 nm.

class="figure_img" id="Figure6"/>
Download
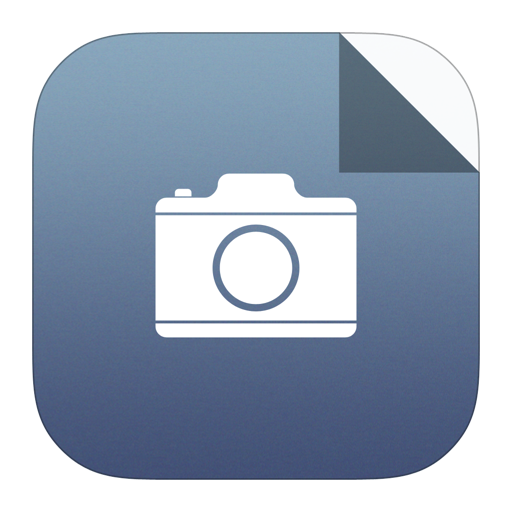
Larger image
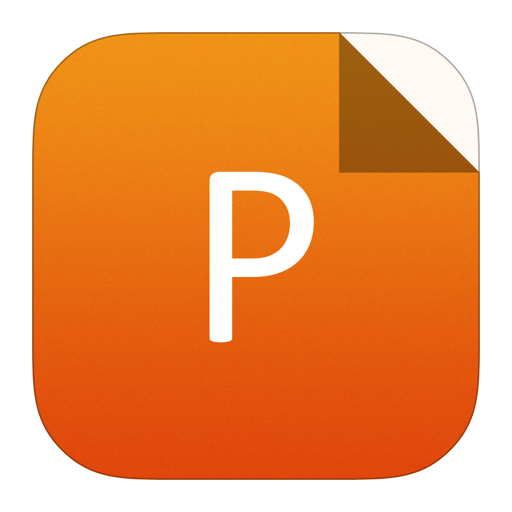
PowerPoint slide
Figure6.
(Color?online)?Number of successes of each nanomagnet element versus the defect amplitude for different materials. (a) Permalloy material. (b) Co material. (c) Fe material.
According to the simulation results, the interconnect wire denotes moderate robustness to the missing corner defect, which provides encouraging information to engineering scientists. The last interesting observation is that the interconnect wire made of iron material shows an outstanding defect tolerance in comparison with the two other magnetic materials. This may arise from its relatively large saturation magnetization and exchange stiffness constant generating enough stability.
3.3
Element spacing effects
In this subsection, the nanomagnet element spacing DS in the defective NML interconnect wire will be considered to evaluate errors. Suppose that the defect amplitude is 7 nm, and the spacing between elements has variable values ranging from 15 to 40 nm (with a step of 5 nm). All the other simulation parameters are the same as those appearing in Section 3.2. However, we herein no longer show a success rate curve of each interconnect element, but denotes the average success rates of three kinds of different interconnects at each spacing. The obtained results for different magnetic materials are shown in Fig. 7.

class="figure_img" id="Figure7"/>
Download
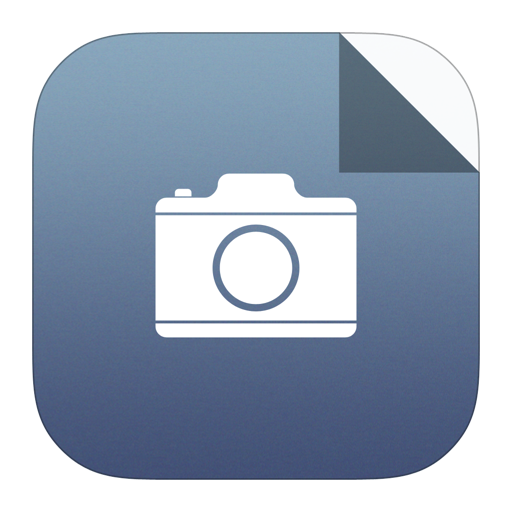
Larger image
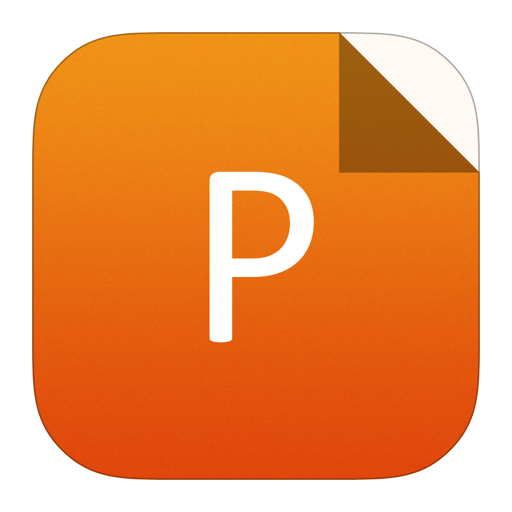
PowerPoint slide
Figure7.
(Color?online)?Nanomagnet spacing effects of defective interconnect wire fabricated with three different materials.
From Fig. 7, we can see that the average success rates (SRA) of three kinds of different NML interconnect wires denote similar trend. In detail, for the spacing less than 25 nm, the average success rate increases with increasing spacing (e.g., SRA = 84% for DS = 25 nm and Fe), while for the spacing over 25 nm, the SRA decreases with increasing spacing, but this decrease seems rather slow for distances less than 35 nm. This observation is completely different from the previous viewpoint that closer spacing shows higher success rate, but demonstrates an optimal spacing for interconnect wire design. At tighter spacing (when the spacing is small), the driver nanomagnet device influences both the nanomagnets N1 and N2 simultaneously, and causes errors between them. On the other hand, as the spacing increases, the coupling energy between N1 and N2 becomes low enough that the parasitic effects become dominant, causing arbitrary switching of the nanomagnets. Therefore, an optimal spacing exists in the defective NML wire. The other interesting phenomenon is that the NML wire made of iron material still shows better defect tolerance than other two magnetic materials. This may also arise from its relatively large saturation magnetization parameter.
4.
Conclusion
Nanomagnet-based logic is an emerging and novel field coupled computing paradigm, while interconnect wire is a very important component of any magnetic logic circuit. This article carries through a systematical study for edge or corner imperfections in the interconnect for the purpose of reliable logic propagation.
We adopt a triangle to model the missing corner defect of nanomagnets in the wire, and investigate the interconnect fabricated with various magnetic materials by micromagnetic simulations under different corner defect amplitudes and device spacings. The results show that as the defect amplitude increases, the success rate of logic propagation in the interconnect wire decreases. However, this phenomenon can be mitigated if the missing edge defect gradually approaches the input terminal. More results show that the interconnect wire fabricated with material iron demonstrates the best defect tolerance ability among the three representative and frequently used NML materials, also logic transmission errors can be mitigated by adjusting the spacing between nanomagnets.