1.
Introduction
As an important III–V wide band-gap (~6.20 eV) semiconductor, aluminum nitride (AlN) displays many outstanding properties, such as high breakdown field, high thermal conductivity, high thermal stability and small electron affinity which make it a promising candidate for the fabrication of surface acoustic wave devices[1, 2], light-emitting devices[3], field emission devices[4] and electronic packages[5]. This particularly attractive material has attracted great research enthusiasm for fabricating AlN nanostructures and investigating their properties. Recently, one-dimensional (1-D) AlN nanostructures, such as nanorings, nanotubes and nanowires have been successfully fabricated by chemical vapor deposi- tion[6–8], DC-arc discharge plasma method[9, 10], the direct nitridation method[11–13], plasma process[14] and catalyst-assisted growth[15–17]. However, AlN nanostructures from those methods are synthesized at low temperature, which will inevitability induce defects, such as screw dislocations, stacking faults or mismatch between single-crystal components. Moreover, most of the methods have some disadvantages of low productivity and severe impurities from their employed precursors or incomplete conversions of the starting materials. So far, AlN nanowires have been successfully synthesized by PVT method[18–20], whereas complex nanostructures such as nanorings, nanosprings and nanohelices have not been fabricated by physical vapor transport (PVT) method. Now, it remains a challenge to synthesize new 1-D AlN nanostructures via high-temperature and catalyst-free process. In this letter, we performed a detailed investigation on the synthesis of AlN nanostructures via an ultrahigh-temperature, catalyst-free, PVT process, and focus on the effect of growth duration and temperature on AlN morphology.
2.
Results and discussion
AlN nanostructures were synthesized by PVT method in an RF-heated furnace. The schematic diagram of typical PVT sublimation configuration is shown in Fig. 1(a). To obtain ultrapure raw material, AlN polycrystalline powder (Alfa Aesar, 99.999%) was sintered at 2000 °C for 10 h to reduce the impurity content in the samples. Glow discharge mass spectrometry (GDMS) revealed that the content of Mg, Fe and Ga is less than 20 ppm. The sintered AlN powder decomposes into AlN vapor at a high-temperature region in (5.0–7.0) × 104 Pa nitrogen atmosphere (99.999%) and the decomposition products were transported to a low-temperature region where they formed AlN nanostructure crystals on TaC substrate surface as shown in Fig. 1(b). A typical low-magnification SEM image of the products deposited on the substrate that were grown at 1800 °C for 40 min is shown in Fig. 1(c). The nanowires have a typical length of several tens of microns. The EDX spectrum revealed that the element composition of N and Al was 46% and 53%, respectively, and no trace of other elements was observed.

class="figure_img" id="Figure1"/>
Download
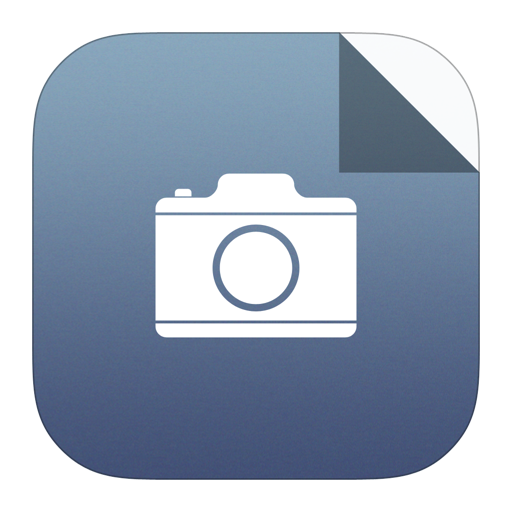
Larger image
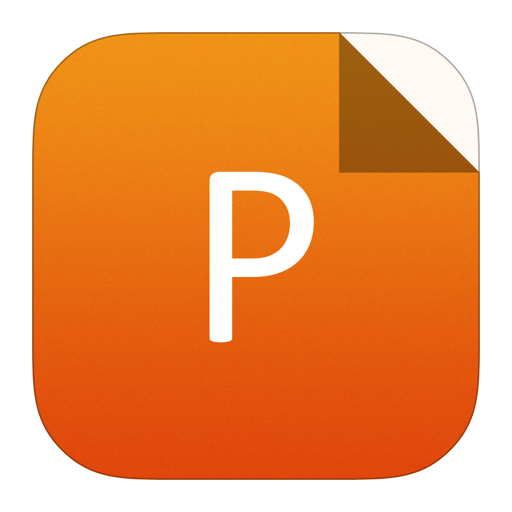
PowerPoint slide
Figure1.
(Color online) (a) Schematic diagram of growth typical PVT sublimation configuration. (b) Photograph of the products. (c) SEM image with EDX spectrum displayed in the inset.
As shown in Fig. 2(a), the XRD pattern indicates that the as-prepared product consists of wurtzite AlN. The lattice parameter of the wurtzite AlN cell calculated from the XRD data is a = b = 0.3110 nm, c = 0.4977 nm, which is in good agreement with the standard value for 2H-AlN (a = b = 0.3111 nm, c = 0.4978 nm, JCPDS-PDF no. 76-0565). No characteristic peak associated with other crystalline forms was found in the XRD pattern. XPS spectra are also measured to examine the components and chemical states of the as-grown AlN nanowires, as shown in Fig. 2(b). The peaks at 73.72 and 396.43 eV correspond to Al 2p and N 1s states, respectively[21]. Figs. 2(c) and 2(d) show bright-field transmission electron microscope (TEM) images and high-resolution TEM (HRTEM) images of an individual AlN nanowire, respectively. The corresponding fringe spacing of 0.25 nm can be assigned to d100 values of hexagonal AlN based on the known International Centre for Diffraction Data (ICDD) data as mentioned above. The selected area electron diffraction (SAED) pattern was shown in the inset of Fig. 2(d). Obviously, on the basis of the typical Debye?Scherrer diffraction dots, single-crystal nature was verified in as-obtained AlN nanowire. These results further confirm that the as-prepared AlN helices possess good crystallinity.

class="figure_img" id="Figure2"/>
Download
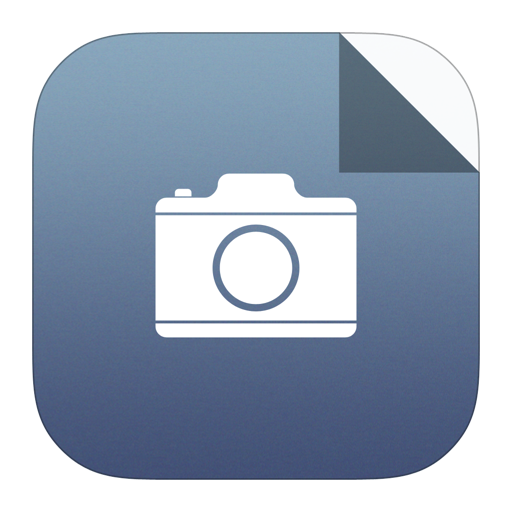
Larger image
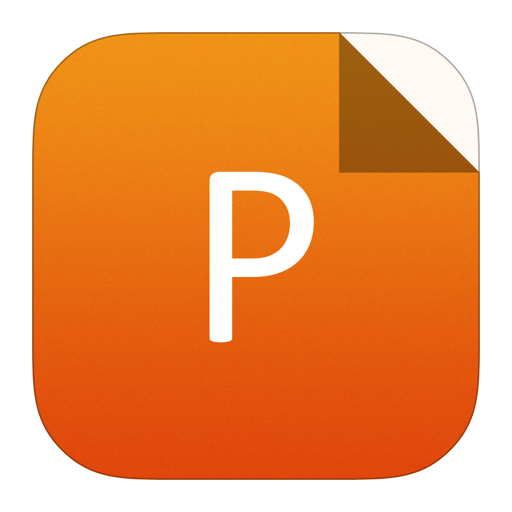
PowerPoint slide
Figure2.
(Color online) (a) XRD spectra of as-grown product. (b) XPS spectra of the AlN nanowires, upper shows Al 2p spectrum and N 1s spectrum is displayed below. (c) TEM image of a single AlN nanowire. (d) HRTEM image for the dot as denoted by the red box in (c). The inset in (d) shows the SAED pattern.
Fig. 3 shows SEM images of AlN nanostructure with increasing growth duration from 20 to 180 min at 1800 °C and the morphology of individual AlN nanostructure is also shown in the inset of each image. For 20 min growth duration, the AlN nanowires were about 100–200 nm in diameter which tend to form AlN nanorings as shown in Fig. 3(a). As the growth duration was increased to 40 min, most of the nanowires exhibit nanosprings structure in Fig. 3(b). In Fig. 3(c), when the growth duration was continually increased to 80 min, the nanowires were irregular in shape, it was observed that partial surface was smooth, whereas others had jagged surface. When the growth duration was more than 180 min, most of the straight AlN nanowires showed a zigzag surface as shown in Fig. 3(d).

class="figure_img" id="Figure3"/>
Download
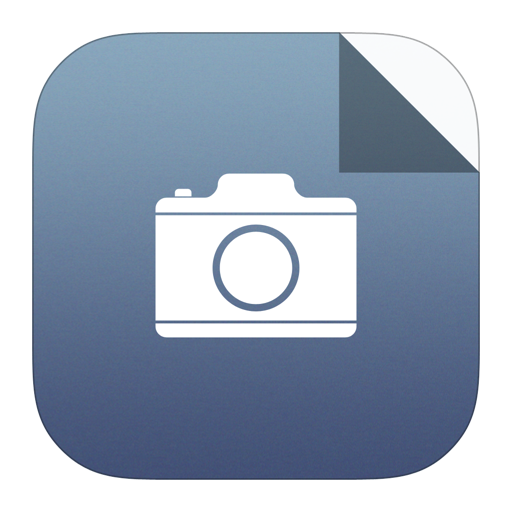
Larger image
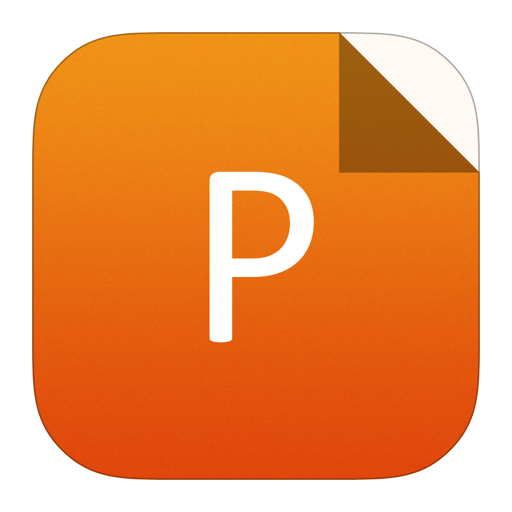
PowerPoint slide
Figure3.
SEM images of AlN nanostructure with different growth duration at 1800 °C. (a) 20 min. (b) 40 min. (c) 80 min. (d) 180 min. The details morphology of individual AlN nanostructure corresponding to (a)–(d) are shown in the inset each image, respectively.
Fig. 4 shows SEM images of the as-prepared AlN nanostructure at different growth temperatures in 40 min growth duration. As shown in Fig. 4(a), when the growth temperature was at 1750 °C, most of the AlN nanowires show irregular structure, and chain-like structure AlN nanowires with a periodically and symmetrically jagged morphology also observed. At 1800 °C, there is no significant change in the surface morphology of AlN, similar to Fig. 3(a). As the temperature increases to 1850 °C, the nanostructures incline to show a six-fold symmetric structure with diameter modulated along its axis. Further increasing the temperature to 1900 °C leads to a rod-like structure with a zigzag surface morphology, and the average diameter of rods was about 10 μm.

class="figure_img" id="Figure4"/>
Download
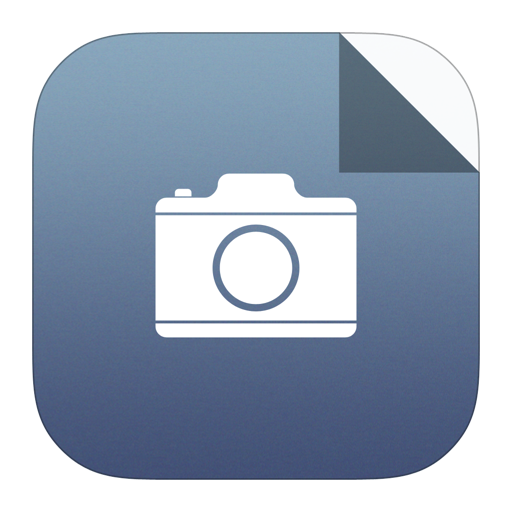
Larger image
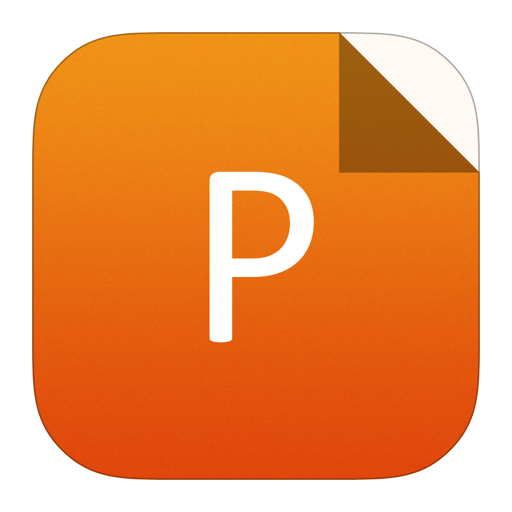
PowerPoint slide
Figure4.
SEM images of AlN nanostructure with different growth temperature in 40 min growth duration. (a) 1750 °C. (b) 1800 °C. (c) 1850 °C. (d) 1900 °C. The details morphology of individual AlN nanostructure corresponding to (a)–(d) are shown in the inset each image, respectively.
To further examine the effect of the heating temperature and growth time on the morphologies of the products, a series of SEM images of individual AlN nanostructure was shown in Fig. 5(a). Interestingly, the morphology of AlN nanowires altered greatly with increasing growth duration. The morphology evolution can be explained by electrostatic polar charge model[15, 22–25] as shown in Figs. 5(b) and 5(c). It has been reported that a dipole moment existing between the two surfaces along the c-axis will induce electrostatic energy[22, 23]. For ZnO nanowires, the electrostatic energy decreases after forming a nanohelix, and a small diameter is strongly in favor of forming helices[23]. Duan et al. reported that the electrostatic polar charge model is employed in the explanation of the AlN nanoring formation[15]. In fact, the growth of other nanostructures also can be understood on the basis of the electrostatic polar charge model. Due to the increase of the diameter of the AlN nanowires with increasing the growth duration in PVT processes, the nanowire structure tends to transform to nanoring, nanospring, nanohelix and other nanostructures to decrease the total energy. This phenomenon is attributed to a consequence of minimizing the total energy caused by spontaneous polarization and elasticity.
On the other hand, the morphology of AlN nanowires is also dependent on growth temperature. With increasing the growth temperature, the morphology of AlN nanowires is transformed from chain-like structure to nanosprings, and then to six-fold symmetric structure, at last rod-like structure with a zigzag surface morphology is obtained. The evolution of morphology dependent on growth temperature can be explained by the crystalline lattice structure of AlN[26–28] as shown in Fig. 5(d). The nature of the β1 bond is more covalent while that of the β2 is more ionic and β2 bond energy is relatively smaller than that of the β1 bond. At a lower growth temperature, the growth rate of β2 is faster than β1, so extra-long nanowire was observed. At a higher temperature (~2000 °C), the increasing energy of Al/N atoms makes the growth rate of β1 bond and β2 bond comparable. It has been reported that the growth rate for AlN crystal at 2200 °C is estimated to be roughly 1 : 3 : 10 for m-plane {1010}, r-plane {1013}, and c-plane {0001} facets[29]. As result, the six-fold symmetric structure and rod-like structure with a zigzag surface morphology was fabricated. In addition, SEM images marked by the red box in Fig. 5(a) present the morphological features of the produce obtained at 1900 °C for 80 min and 1850 °C for 180 min, respectively. Evidently, the regular six-sided prism with hexagonal pyramidal cap is found which is similar to previous report[18]. AlN growth at 2000 °C for 180 min is shown in Fig. 5(a) marked by the blue box,and indicated that bulk AlN crystal was obtained at such growth condition[30, 31]. Based on the aforementioned analysis this demonstrates that the desired nanostructures can be synthesized via a rational and well-controlled synthesis strategy in PVT process. In fact, both electrostatic polar charge model and the crystalline lattice structure of AlN play an important role in AlN crystal growth.

class="figure_img" id="Figure5"/>
Download
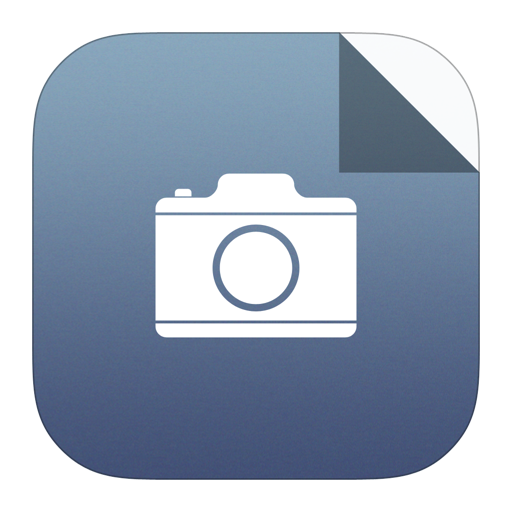
Larger image
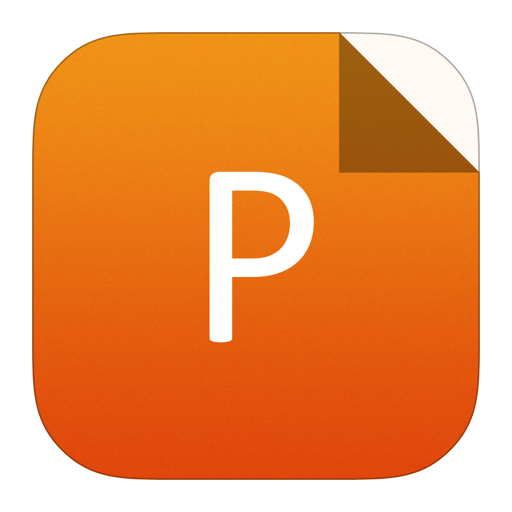
PowerPoint slide
Figure5.
(Color online) (a) The morphological dependence of AlN nanostructures on the heating temperature and growth time. (b) A schematic model of a nanowire as viewed parallel to its flat surfaces. (c) The bottom-up and top-down views of the nanohelical model. (d) Atomic structure of wurtzite AlN.
3.
Conclusion
In summary, diverse morphologies of AlN nanostructures are obtained via PVT method with ultrahigh-temperature and catalyst-free process. At 1800 °C, the nanostructure is varied from nanoring, nanospring to nanowire with jagged and zigzag surface with increasing the growth duration. For 40 min growth duration, the morphology of AlN nanowires changes significantly with increasing the growth temperature, which is varied from chain-like structure to nanospring, and then to six-fold symmetric structure, and finally to a rod-like structure. The morphology evolution is attributed to electrostatic polar charge model and the crystalline lattice structure of AlN.