1.
Introduction
The study of the infrared radiation (IR) spectral range is of great practical and scientific importance. The set of tasks that will be produced with the development of humanity, will require the constant expansion of the number of used materials, an improvement of their parameters, and also the theoretical justification of the properties that have been covered[1]. AIIIBV semiconductors are, on this day, promising materials for the IR region. Smith and Mailhiot in 1987 proposed the use of type-II superlattices (T2SLs) InAs/GaSb with excellent electro-optical properties, theoretically being comparable with HgCdTe[2]. Recently, the considerable progress towards III–V antimonide-based low dimensional solids development and device design innovations has been presented[3].
In T2SLs the minimum level of the conduction band in InAs is below the maximum of the valence band of GaSb. That band arrangement called the type-II “broken gap” makes it possible to adjust the effective separation between the lowest conduction band C1 and the highest valence band VH1 in the scientifically important 2–10 μm range wavelength by changing the thickness of the InAs and GaSb layers. This tuning of the band gap made the above system technologically interesting for IR lasers and detectors.
2.
Theoretical modeling of detectivity characteristics
The simulated T2SLs InAs/GaSb interband cascade infrared detectors (IB CID) consist of an n-type contact layer grown on the GaSb substrate, active layer, and upper contact layer made of a beryllium doped GaSb. The active layer consists of seven cascades, in which the absorber regions are connected by the AlSb tunnel barrier. The individual absorber region, in turn, consists of 28 periods of 9 mono layers (ML) InAs and 9 ML GaSb (net thickness, d = 153 nm). The total thickness of the absorber is 1.07 μm. The responsivity is low compared to the values for conventional p-i-n junction photodiodes. This is partly explained by a thin individual absorber[4]. The high temperature range was assumed in calculation, i.e. T = 320–380 K.
The detectivity characteristic D* of the IB CID T2SLs InAs/GaSb can be estimated using a theoretical model proposed by Hinkey and Yang[5]. In this paper we assume that the surface recombination rate is zero. For a multistage detector with the identical Ns number of stages, where the ambipolar diffusion length L is constant at each stage, the expression for D* is given by:
$${D^{ m{*}}} = frac{{ m{lambda }}}{{hc}}sqrt {{N_{ m{s}}}} frac{eta }{{sqrt {2{{ m{g}}_{{ m{th}}}}L{ m{tanh}}left( {frac{d}{L}} ight)} }}{ m{exp}}left( { - ,, alpha dleft( {{N_{ m{s}}} - 1} ight)} ight),$$ ![]() | (1) |
where: η, gth, d, c, h, λ, and α are the quantum efficiency one stage, the carrier generation rate per unit volume, the thickness of the absorber in one stage, the speed of light, the Planck constant, the wavelength, and the absorption coefficient, respectively. The increase in the number of stages reduces shot noise and also reduces the overall signal current, due to the attenuation of light at deeper levels. If the detector is illuminated from the side of the carrier collection point, the quantum efficiency η is defined as:
$$eta = left[ {frac{{alpha L}}{{1 - {{left( {alpha L} ight)}^2}}}} ight]left{ {frac{{sinh left( {frac{d}{L}} ight) + alpha L{{ m e}^{ - alpha d}} - alpha Lcosh left( {frac{d}{L}} ight)}}{{cosh left( {frac{d}{L}} ight)}}} ight}.$$ ![]() | (2) |
An absorber is non-intentionally doped, so in high temperature it carrier concentration is comparable to the intrinsic concentration. Therefore, the gth parameter can be described by
m{th}}}} = frac{{2np}}{{left( {n + p}
ight)tau }}$

$${I_{ m{b}}}left( U ight) = 2e{{{n}}_{ m{i}}}L{{ m{tau }}^{ - 1}}mathop sum limits_{i = 1}^{{N_{ m{s}}}} N_{ m{S}}^{ - 1} times frac{{sinh left( {d/L} ight)}}{{cosh left( {d/L} ight)}} times left[ {{ m{exp}}left( {frac{{eU}}{{beta {N_{ m{s}}}{k_{ m{B}}}T}}} ight) - 1} ight],$$ ![]() | (3) |
where e, ni, kB, and β are elementary electric charge, intrinsic carrier concentration, the Boltzmann constant, and diode ideality factor, respectively. Parameter β is estimated experimentally and is equal to 2. The correct fit of the theoretical I–U characteristics to the experimental values allows us to determine the carrier lifetime τ. The correct estimation of τ was defined in our earlier work[6].
The determination for the carrier concentration ni written by:
$${n_{ m{i}}} = 2left( {frac{{2{ m{pi }}{k_{ m{B}}}}}{{{h^2}}}} ight){left( {m_{ m{e}}^{ m{*}}m_{ m{h}}^{ m{*}}} ight)^{0.75}}{T^{1.5}}{ m{exp}}left( { - frac{{{E_{ m{g}}}}}{{2{k_{ m{B}}}T}}} ight),$$ ![]() | (4) |
requires knowledge of the energy gap Eg and the effective electrons and holes masses
m{e}}^{
m{*}}$

m{h}}^{
m{*}}$

The estimation of the electrons
m{e}}^{
m{*}}$

m{h}}^{
m{*}}$

$$m_{{ m{e}},{ m{h}}}^{ m{*}} = {left[ {{{left( {m_{{ m{e}},{ m{h}}||}^{ m{*}}} ight)}^2} m_{{ m{e}},{ m{h}} bot }^{ m{*}}} ight]^{frac{1}{3}}},$$ ![]() | (5) |
where
m{e}}||}^{
m{*}}$

m{e}} bot }^{
m{*}}$

m{h}}||}^{
m{*}}$

m{h}} bot }^{
m{*}}$

The electron band structures and optical properties of T2SLs were modeled using the SimuApsys platform. One of the main difficulties in modeling a narrow-gap superlattice is the determination of the band alignment and the valence band offset (VBO). Schematic of the band alignment diagram along the growth direction for InAs/GaSb heterostructure is shown in Fig. 1. The displacement of the valence band, in turn, is associated with overlapping of bandΔ.

class="figure_img" id="Figure1"/>
Download
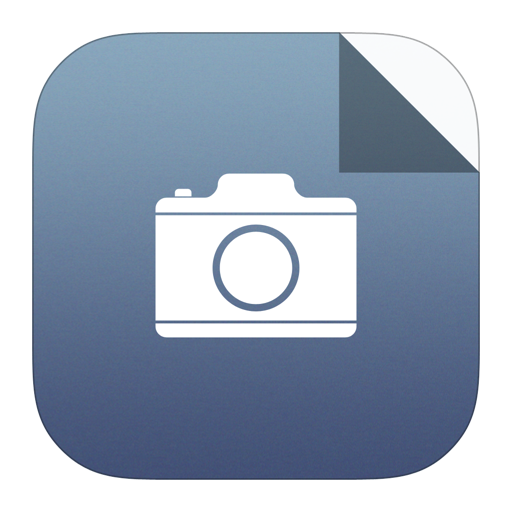
Larger image
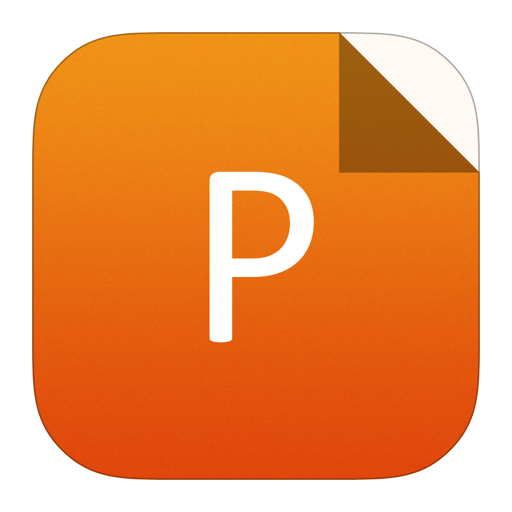
PowerPoint slide
Figure1.
(Color online) Schematic of the band alignment for T2SLs InAs / GaSb, where CBO is conduction band offset.
There are various methods as well as various groups of researchers representing very different experimentally obtained values of the displacement of the valence band at this time. In addition to experimental discrepancy the main reason is the imperfection of the heterojunction formed in practice. Interphase layers are unintentionally formed at the heterojunction, which affect the VBO measurements and other optical properties. In this article, the VBO value was determined by the choice of the overlap band so that the energy bandgap determined from the simulation is consistent with the long-wave optical cut-off.
In order to study dispersion curves (E–k) a 4-band model (k?p 8 × 8 method) for simulation was implemented. The periodic boundary conditions impose periodicity on the wave functions. In our case, the left and right meshes (SimuApsys semantic) were chosen to be half the width of the GaSb layer. The effect of the “non-common atom” (NCA) at the quantum well boundary was also implemented[6]. As shown in Ref. [7], the value of Hxy = 700 meV was used for calculations of the E–k curves. The parameters presented in Table 1 were implemented to simulate the IB CID T2SLs InAs/GaSb active layer[8, 9].
Parameter | Symbol | Material | |
InAs | GaSb | ||
Lattice constant A = a0 + $text{α}$(T – 300) | a (?) | 6.0583 + 2.74 × 10?5 × (T ? 300) | 6.0959 + 4.72 × 10?5 × (T ? 300) |
Band gap Eg = Eg0 ? $text{α}$0T2/(T + β0) | $text{α}$0 (eV/K) | 0.276 × 10?3 | 0.417 × 10?3 |
β0 (K) | 93 | 140 | |
Eg0 (eV) | 0.417 | 0.812 | |
Luttinger parameters | γ1 | 19.481 | 16.291 |
γ2 | 8.428 | 6.305 | |
γ3 | 9.171 | 7.371 | |
Deformation potential | ac (eV) | ?5.08 | ?7.5 |
av (eV) | ?1.00 | ?0.8 | |
b (eV) | ?1.8 | ?2 | |
Elastic constant | C11 (GPa) | 83.29 | 88.42 |
C12 (GPa) | 45.26 | 40.26 | |
C44 (GPa) | 39.59 | 43.22 | |
Spin orbit energy | ΔSO (eV) | 0.39 | 0.76 |
Effective mass | ${textit {m}}_{text{e}}^{*}/{textit {m}}_0$ | 0.028 | 0.035 |
Kane potential | Ep (eV) | 14.427 | 15.726 |
Table1.
Band structure parameters for the InAs and GaSb.
Table options
-->

Download as CSV
Parameter | Symbol | Material | |
InAs | GaSb | ||
Lattice constant A = a0 + $text{α}$(T – 300) | a (?) | 6.0583 + 2.74 × 10?5 × (T ? 300) | 6.0959 + 4.72 × 10?5 × (T ? 300) |
Band gap Eg = Eg0 ? $text{α}$0T2/(T + β0) | $text{α}$0 (eV/K) | 0.276 × 10?3 | 0.417 × 10?3 |
β0 (K) | 93 | 140 | |
Eg0 (eV) | 0.417 | 0.812 | |
Luttinger parameters | γ1 | 19.481 | 16.291 |
γ2 | 8.428 | 6.305 | |
γ3 | 9.171 | 7.371 | |
Deformation potential | ac (eV) | ?5.08 | ?7.5 |
av (eV) | ?1.00 | ?0.8 | |
b (eV) | ?1.8 | ?2 | |
Elastic constant | C11 (GPa) | 83.29 | 88.42 |
C12 (GPa) | 45.26 | 40.26 | |
C44 (GPa) | 39.59 | 43.22 | |
Spin orbit energy | ΔSO (eV) | 0.39 | 0.76 |
Effective mass | ${textit {m}}_{text{e}}^{*}/{textit {m}}_0$ | 0.028 | 0.035 |
Kane potential | Ep (eV) | 14.427 | 15.726 |
3.
Results and discussions
The theoretical calculations were compared with the experimental characteristics IB CID fabricated by The Center for High-Tech Materials (University of New Mexico, Albuquerque). The technological growth procedure for the IB CID T2SLs InAs/GaSb is described in more detail by Gautam et al.[10].
An example of the calculated energy dispersion for directions in the plane of the Brillouin zone and perpendicular (growth) at the investigated temperatures is shown in Fig. 2. The subbands VH1, VH2, and VL1 which are shown in this figure, are responsible for the positions of the heavy and light holes. The horizontal wave vector k|| is normalized to 2/a, where a = 6.100 ? is the lattice constant for GaSb, whereas the perpendicular wave vector k⊥ is normalized to π/L, where L = 5.469 nm is the periodicity of T2SLs.

class="figure_img" id="Figure2"/>
Download
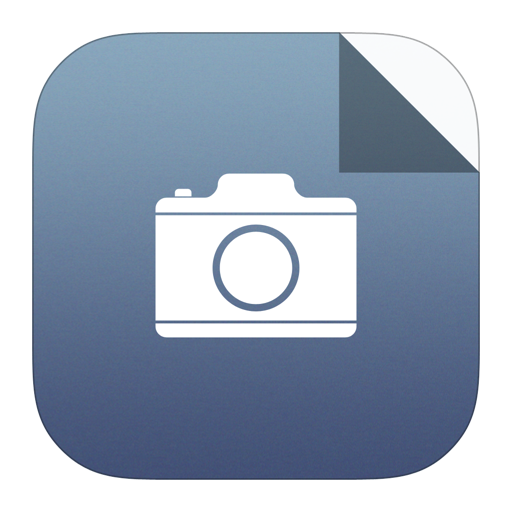
Larger image
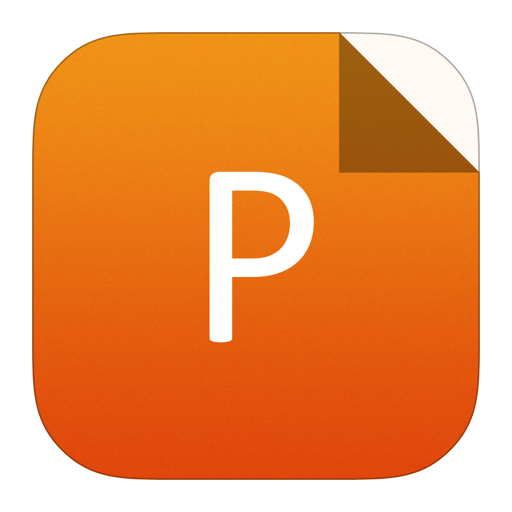
PowerPoint slide
Figure2.
(Color online) The dispersion relation of the simulated T2SLs 9MLs (InAs) / 9MLs (GaSb) for two directions in the Brillouin zone at T = 380 K.
The values of the position of the valence bands, VBO, and the energy gap Eg at temperatures of 320, 340, and 380 K obtained in the vicinity of the Γ-point are given in Table 2.
Temperatures (K) | VH1 (eV) | VH2 (eV) | VL1 (eV) | VL2 (eV) | VH3 (eV) | VH4 (eV) | Eg (eV) | VBO (eV) |
320 | 0.459 | 0.362 | 0.308 | 0.287 | 0.191 | 0.119 | 0.2 | 0.469 |
340 | 0.455 | 0.357 | 0.303 | 0.284 | 0.187 | 0.114 | 0.1935 | 0.463 |
380 | 0.447 | 0.347 | 0.294 | 0.276 | 0.179 | 0.106 | 0.1836 | 0.453 |
Table2.
Calculated band gap parameters of T2SLs InAs/GaSb.
Table options
-->

Download as CSV
Temperatures (K) | VH1 (eV) | VH2 (eV) | VL1 (eV) | VL2 (eV) | VH3 (eV) | VH4 (eV) | Eg (eV) | VBO (eV) |
320 | 0.459 | 0.362 | 0.308 | 0.287 | 0.191 | 0.119 | 0.2 | 0.469 |
340 | 0.455 | 0.357 | 0.303 | 0.284 | 0.187 | 0.114 | 0.1935 | 0.463 |
380 | 0.447 | 0.347 | 0.294 | 0.276 | 0.179 | 0.106 | 0.1836 | 0.453 |
Calculated theoretical energy gaps Eg are comparable to the optical band gaps estimated from the spectral characteristics of long-wave cut-off. The selected values of the displacement of the valence band correspond to the values indicated in the Refs. [11, 12].
The effective masses were calculated from the dispersion curves as the second derivative of energy by the wave vector:
$${m^{ m{*}}} = {hbar ^2}{left( {frac{{{{ m{d}}^2}E}}{{{ m{d}}{k^2}}}} ight)^{ - 1}},$$ ![]() | (6) |
where m* is the effective mass, k is the wave vector, and ? is the reduced Planck constant. For example, at T = 380 K the calculated effective masses of electrons and holes are
m{e}}||}^{
m{*}}$

m{e}} bot }^{
m{*}}$

m{h}}||}^{
m{*}}$

m{h}} bot }^{
m{*}}$


class="figure_img" id="Figure3"/>
Download
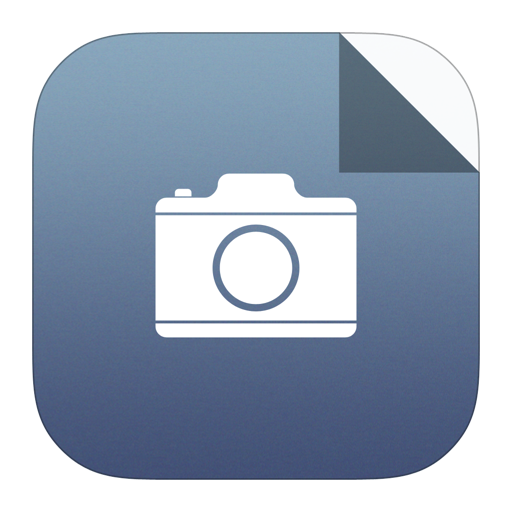
Larger image
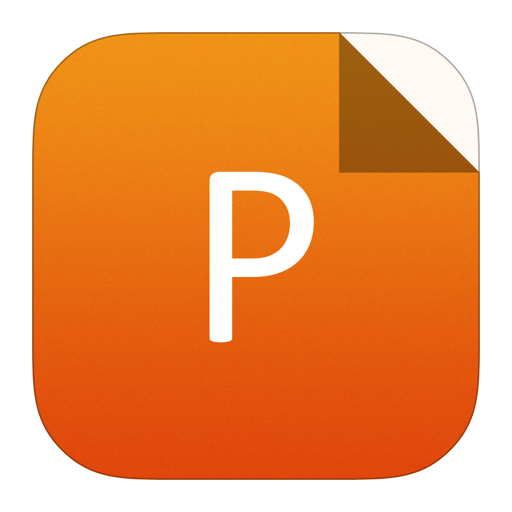
PowerPoint slide
Figure3.
(Color online) Absorption coefficient calculated by SimuApsys.
The one value of the overlap energy equal to Δ = 0.120 eV is chosen for all temperatures. The maximum value of the absorption coefficient α is at 380 K. The α decreases with decreasing photon energy E. The absorption spectrum shows maxima in the regions 0.36, 0.3, and 0.2 eV. These peaks can be explained by the appearance of bands in the superlattice. With decreasing temperature, the value of the absorption coefficient α decreases and the value of distances between bands (including Eg) increases. For further calculation of Johnson noise-limited detectivity D* an average value of absorption coefficient was used.
As noted at the beginning, the comparison theoretically calculated and experimentally measured D* versus wavelength was the main aim of this paper. In order to perform this comparison the first I–U characteristics were analyzed. Fig. 4 shows theoretically calculated and experimentally measured I–U characteristics for temperature range T = 320–380 K. As can be seen the proper fitting of the theoretically calculated and experimentally measured data for the lifetime τ equal ~ 80 ns were reached. At temperature 380 K for reverse bias voltage higher than 0.4 V the measured dark current is higher than the calculated one. Thus, even at high temperatures there could be additional leakage current channels, which lead to higher dark current levels. This increase in the dark current can also be related to the heating of the detector at a high reverse bias voltage.

class="figure_img" id="Figure4"/>
Download
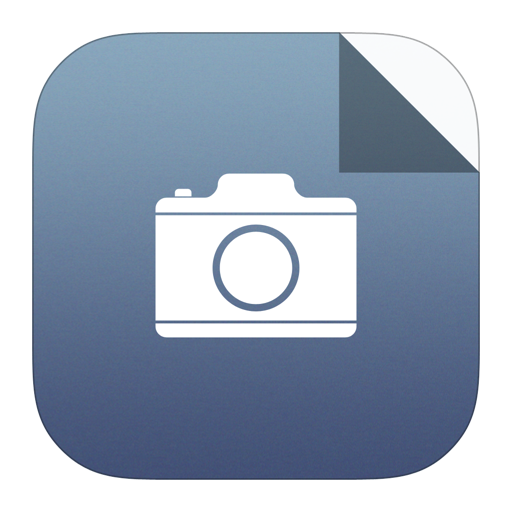
Larger image
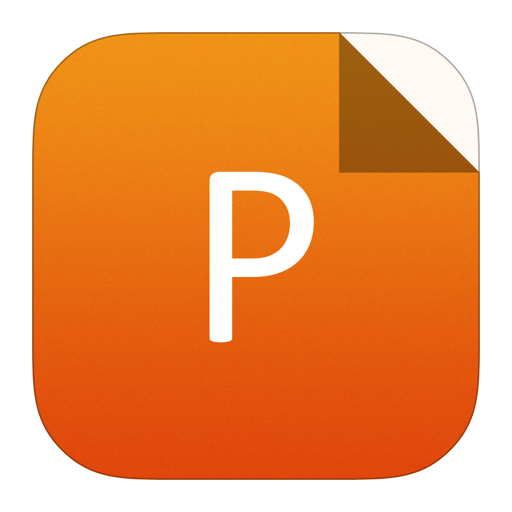
PowerPoint slide
Figure4.
(Color online) Experimental and theoretical I–U characteristics at high temperatures: T = 320, 340, and 380 K.
The theoretically calculated and experimentally measured D* are shown in Fig. 5 for three temperatures T = 320, 340, and 380 K. As can be seen, experimental results do not vary significantly from the calculated theoretical values at 320 K. For higher temperatures, the measured D* is lower than theoretical Johnson noise-limited detectivity. For example at temperature 380 K and at wavelength ≈ 5 μm, the theoretical Johnson noise-limited detectivity is 3 × 108 cmHz1/2W?1 and it is three times higher than the measured one. At higher temperature, the difference between the calculated and measured results is connected with the accuracy of determining the D* measurements. The value of measured Johnson noise-limited detectivity is proportional to quantum efficiency and the square root of dynamic resistance under zero bias. At high temperatures, due to the fact that detectors resistance at zero-bias R0 is relatively low (R0 < 60 Ω), the series resistance ( Rs = 30–40 Ω) could have a significant impact on the accuracy of extracted intrinsic photocurrent. The high series resistance will be connected with the decreasing of the measured quantum efficiency. Since the Johnson noise share is the dominant source at high temperature when the detector operates under zero-bias, the high series resistance increases this noise and decreases measured detectivity.

class="figure_img" id="Figure5"/>
Download
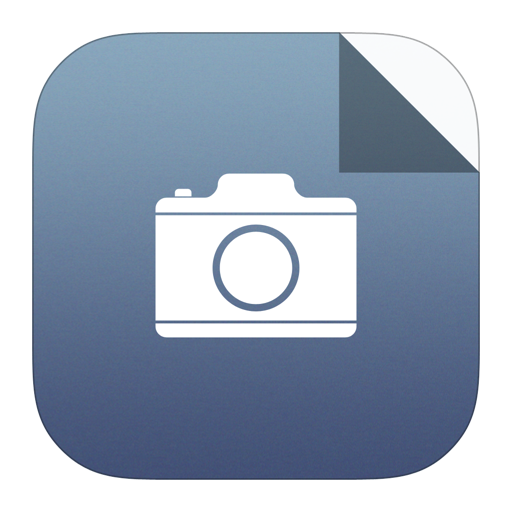
Larger image
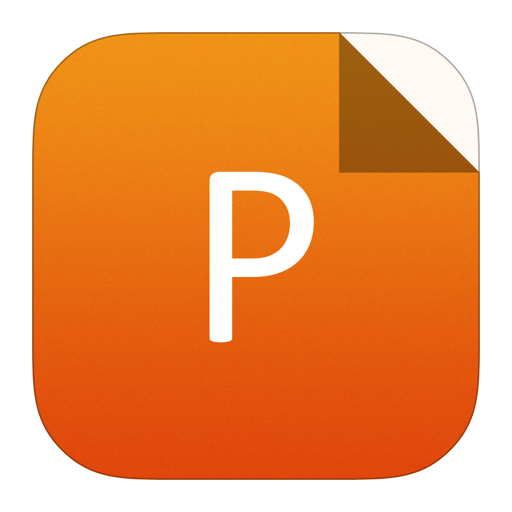
PowerPoint slide
Figure5.
(Color online) Experimental and theoretical Johnson noise limited detectivity.
The performance of the device can further be improved by the doping optimization in the absorber and barrier regions. It is necessary to study the quantum properties of cascades, the physics of their design and material properties for reaching the best performance of the T2SLs HOT detectors. Lei et al. have shown that one way to improve the detectivity value of detectors at high temperatures is to increase the number of stages[13].
4.
Conclusions
The IB CID T2SLs InAs/GaSb in the temperature range of 320–380 K was investigated. The proper fitting of theoretically calculated and experimentally measured spectral characteristics were shown, assuming that the transport in the absorber was determined by the dynamics of almost intrinsic carriers and carrier lifetime. In addition, the overlap value about 120 meV equal for all investigated temperatures was estimated. The possibility to improve the detection parameters of these detectors at higher temperatures, relative to room one, was shown.