1.
Introduction
In recent years, with the epitaxial technique progress of semiconductor and the continuous improvement of the fabrication technology, the research of high-power vertical cavity surface emitting laser (VCSEL) has become the focus of attention[1–6].
It is reported[7–11] that the difficulty encountered in the first vertical cavity surface emitting laser at room temperature in the twentieth century is how to solve the problem of heat dissipation. Until now, the impact of this problem is still very serious, the excess heat inside the device will seriously hinder the further improvement of the output power and the power conversion efficiency. So it is necessary to consider the thermal characteristics in the design of the device. With the top emitting VCSEL structure as an example, the simulation software of Crosslight is used to simulate the VCSEL with different mesa sizes under the condition of continuous working at room temperature. Lattice heat distribution of different sizes of mesa radius from 4.5 to 12.5 μm are simulated, while the output characteristics are analyzed. Moreover the relationship between mesa size and thermal characteristics are obtained. According to the simulation results, the optimal process parameters (the mesa sizes) are obtained, which provides a strong theoretical basis for the optimization of the actual process.
2.
Structure and fabrication of VCSEL
In this research work, we proposed oxide-confined VCSEL epitaxial structure model operating at wavelength of 980 nm. The VCSEL resonator consists of two distributed Bragg reflector (DBR) mirrors parallel to the surface with an active region consisting of a number of quantum wells for the light generation in between. In the proposed design the active region contains quantum wells (QW) with strained In0.2GaAs/GaAs barrier layers. We employ Al0.98GaAs oxide layer with a thickness of 71.8 nm, the diameter of the bottom DBR (26 pairs of GaAs/AlAs) is assumed to be equal to 0.1535 μm, whereas the diameter of the top DBR (25 pairs of GaAs/Al0.375GaAs) is 0.1497 μm. The proposed VCSEL specific structure is shown in Table 1.
Layer | Material | Group | Repeat | Mole fraction (x) | Thickness (μm) | Dopant | Type | CV level (1018 cm?3) |
15 | GaAs | 0.2121 | Carbon | P | 3.00 | |||
14 | AlxGaAs | 2 | 25 | 0.375 | 0.0790 | Carbon | P | 2.00 |
13 | GaAs | 2 | 25 | 0.0707 | Carbon | P | 2.00 | |
12 | AlxGaAs | 0.980–0.375 | 0.010 | Carbon | P | 2.00 | ||
11 | AlxGaAs | 0.980 | 0.0718 | Carbon | P | 2.00 | ||
10 | AlxGaAs | 0.420 | 0.1073 | Carbon | P | 2.00 | ||
9 | GaAs | 2 | 0.020 | Undoped | U/D | |||
8 | InxGaAs | 2 | 0.200 | 0.008 | Undoped | U/D | ||
7 | GaAs | 0.015 | Undoped | U/D | ||||
6 | InxGaAs | 0.200 | 0.008 | Undoped | U/D | |||
5 | GaAs | 0.020 | Undoped | U/D | ||||
4 | AlxGaAs | 0.420 | 0.1073 | Silicon | N | 2.00 | ||
3 | AlAs | 2 | 26 | 0.0828 | Silicon | N | 2.00 | |
2 | GaAs | 2 | 26 | 0.0707 | Silicon | N | 2.00 | |
1 | AlxGaAs | 0.499 | 20.0000 | Silicon | N | 2.00 | ||
AlGaAs substrate |
Table1.
The structure of 980 nm VCSEL.
Table options
-->

Download as CSV
Layer | Material | Group | Repeat | Mole fraction (x) | Thickness (μm) | Dopant | Type | CV level (1018 cm?3) |
15 | GaAs | 0.2121 | Carbon | P | 3.00 | |||
14 | AlxGaAs | 2 | 25 | 0.375 | 0.0790 | Carbon | P | 2.00 |
13 | GaAs | 2 | 25 | 0.0707 | Carbon | P | 2.00 | |
12 | AlxGaAs | 0.980–0.375 | 0.010 | Carbon | P | 2.00 | ||
11 | AlxGaAs | 0.980 | 0.0718 | Carbon | P | 2.00 | ||
10 | AlxGaAs | 0.420 | 0.1073 | Carbon | P | 2.00 | ||
9 | GaAs | 2 | 0.020 | Undoped | U/D | |||
8 | InxGaAs | 2 | 0.200 | 0.008 | Undoped | U/D | ||
7 | GaAs | 0.015 | Undoped | U/D | ||||
6 | InxGaAs | 0.200 | 0.008 | Undoped | U/D | |||
5 | GaAs | 0.020 | Undoped | U/D | ||||
4 | AlxGaAs | 0.420 | 0.1073 | Silicon | N | 2.00 | ||
3 | AlAs | 2 | 26 | 0.0828 | Silicon | N | 2.00 | |
2 | GaAs | 2 | 26 | 0.0707 | Silicon | N | 2.00 | |
1 | AlxGaAs | 0.499 | 20.0000 | Silicon | N | 2.00 | ||
AlGaAs substrate |
The traditional oxide-confined VCSEL structure is used in the paper, as shown in Fig. 1. The epitaxial growth was carried out by metal organic chemical vapor deposition (MOCVD). After etching the mesa of laser, the oxide layer of the high aluminum component is exposed to form a confined zone of high resistance by the method of wet oxidation. Finally, P-type and N-type electrodes are fabricated.
The size of the oxide aperture determines the size of the current path. According to the report by Rohan Bajaj et al.[12–14], the size of the oxide aperture should be about 2–5μm for stable single mode output. In this paper, VCSEL with a light-hole radius of 1 μm is fabricated, and the oxide layer of high aluminum component can be exposed to air by fabrication of a high mesa. The size of the mesa is closely related to the thermal characteristics of the device, the following is the selection of different values of the mesa radius (the distance from the center to the edge of the mesa), in order to simulate the output characteristics of the device. The mesa radius has been marked out in the Fig. 1, and it is the same as here below, no longer explained.

class="figure_img" id="Figure1"/>
Download
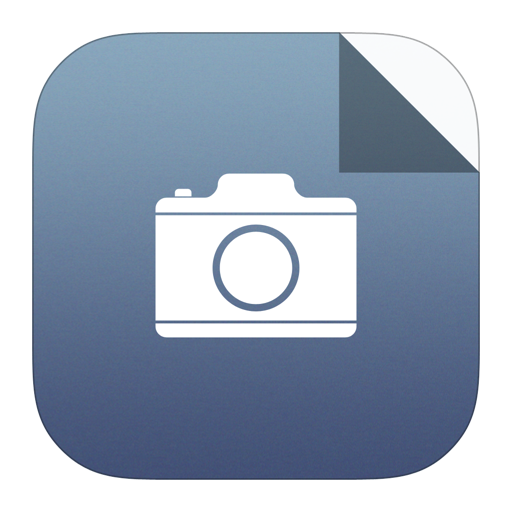
Larger image
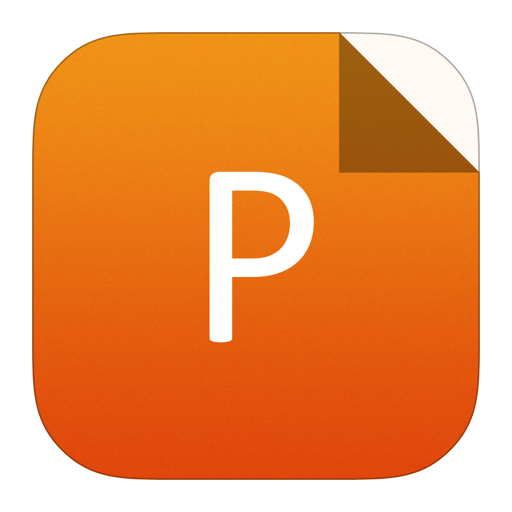
PowerPoint slide
Figure1.
(Color online) The structure of traditional oxide-confined VCSEL.
3.
Theoretical analysis
3.1
The model of heat source
There are many models for the heat source distribution of semiconductor laser. In this paper, the model of heat source is improved by the analysis, and the internal heat source of the semiconductor laser is divided into the following parts[15]:
(1) A lot of carrier density and photon density can be generated in the active region. Moreover, there will be a lot of non-radiative recombination, radiation absorption and spontaneous absorption, so it will generate a lot of heat, and internal heat flux density in the active region is represented by the following formula:
$$begin{split}&{Q_{ m active}} = frac{{{V_{ m j}}}}{{d{}_{ m active}}} times qquadqquadqquad &left[{{j_{ m th}}left( {1-{eta _{ m sp}}{f_{ m sp}}} ight) + (j-{j_{ m th}})(1-{eta _{ m i}}) + (j - {j_{ m th}}){eta _{ m i}}(1 - {eta _{ m ex}})} ight], end{split}$$ ![]() | (1) |
where dactive is the thickness of the active region, Vj is the voltage drop of PN junction, j is the current injection density, jth is the threshold current density, ηsp is the internal quantum efficiency of spontaneous emission, fsp is the escape factor of spontaneous radiation photon from the active region, and ηex is the extraction efficiency.
Therefore we obtain the following expression of efficiency for a general laser diode:
$${eta _{{ m{ex}}}} = 1/(1 + F_{ m r}),$$ ![]() | (2) |
where, using definition of fraction Fr,
$${F_{ m{r}}} = { m{ }}int {frac{{{alpha _{ m{i}}}{P_{{ m{tot}}}}(z) m{d}z}}{{{P_{{ m{tot}}}}({z_{ m{1}}})(1 - {R_{ m{1}}})/(1 + {R_{ m{1}}}) + {P_{{ m{tot}}}}({z_{ m{2}}})(1 - {R_{ m{2}}})/(1 + {R_{ m{2}}})}},} $$ ![]() | (3) |
where αi is the internal material loss, Ptot is the linear power density, and R1 and R2 are the facet power reflectivities.
The above derivation ignores any grating/coupling effects. However, it can be shown that if the coupling coefficient is real, the formula applies to any type of lasers including FP, DFB, DBR and VCSEL with variations in all quantities. This expression leads to some useful conclusions. Suppose we fix the photon density at the facets Ptot(z1) and Ptot(z2) and allow the intensity to vary inside the cavity. If the intensity inside is low (such as in FP laser), the integral in the numerator will be small and thus lead to a higher efficiency. On the other hand, if the power is high in the mid-section and small near the facets, such as in 1/4 wavelength shifted DFB laser and VCSEL, the laser will be a less efficient laser.
(2) The heat sources of other layers are in the form of Joule heat, and heat flux density is represented by the following formula:
$${Q_{ m i}} = {j^2}{ ho _{ m i}},$$ ![]() | (4) |
where ρi is the resistivity of the each layer.
(3) Heat flux density in the alloy layer is represented by contact resistance:
$${Q_{ m c}} = frac{{{j^2}{ ho _{ m c}}}}{{{d_{ m c}}}},$$ ![]() | (5) |
where ρc is the specific contact resistivity of GaAs and metal layer, and dc is the thickness of metal layer.
3.2
Calculation and analysis
After the laser reaches a stable working state, the differential equation of heat conduction is observed:
$$frac{partial }{{partial {{x}}}}left( {{k_{{x}}}frac{{partial T}}{{partial {{x}}}}} ight) + frac{partial }{{partial {{y}}}}left( {{k_{{y}}}frac{{partial T}}{{partial {{y}}}}} ight) + frac{partial }{{partial {{z}}}}left( {{k_{{z}}}frac{{partial T}}{{partial {{z}}}}} ight) + Q = 0,$$ ![]() | (6) |
wherekx, ky,kz are x, y, zthermal conductivities, Q is the internal heat flux density. According to the symmetry, in order to reduce the amount of calculation, the right half of mesa is calculated. The boundary conditions in the calculation are as follows:
(1) Ignore the heat-exchange between the device and the surrounding air.
(2) Consider the heat-exchange between the heat sink and the air in natural convection.
4.
Result analysis
4.1
Comparison of lattice temperature with different mesa sizes
In Fig. 2, Crosslight simulation software is used to simulate the three-dimensional lattice temperature with different mesa sizes, and the temperature increases significantly in the active region. The x-axis in the figure represents the horizontal r of the VCSEL mesa (the origin represents the center of the mesa), the y-axis represents the light direction z (the origin represents the substrate position), and the longitudinal axis represents the lattice temperature in Fig. 3. With injection of 2.6 mA continuous current, the temperature rise with different mesa sizes is obtained by comparison. Therefore, when the size of mesa radius is 4.5 μm, the temperature reaches to about 600 K in the active region, the temperature rise is too large, and when the size of mesa radius is 5.5 μm, the temperature drops to about 440 K in the active region. It means that the mesa size is too small, which causes the heat up quickly.

class="figure_img" id="Figure3"/>
Download
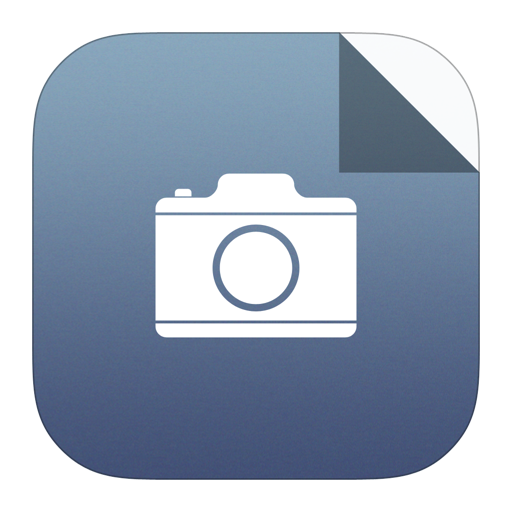
Larger image
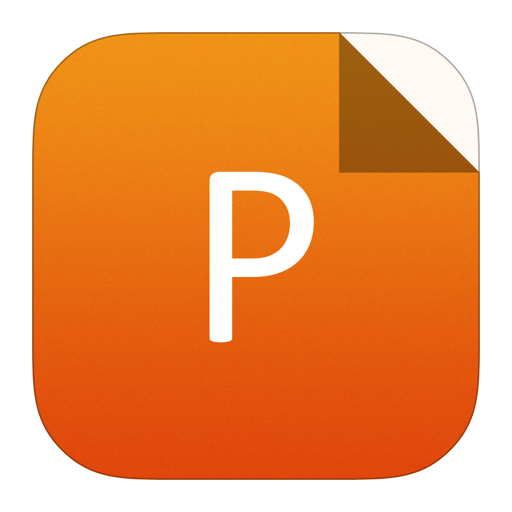
PowerPoint slide
Figure3.
(Color online) The parameter description of traditional oxide-confined VCSEL.

class="figure_img" id="Figure2"/>
Download
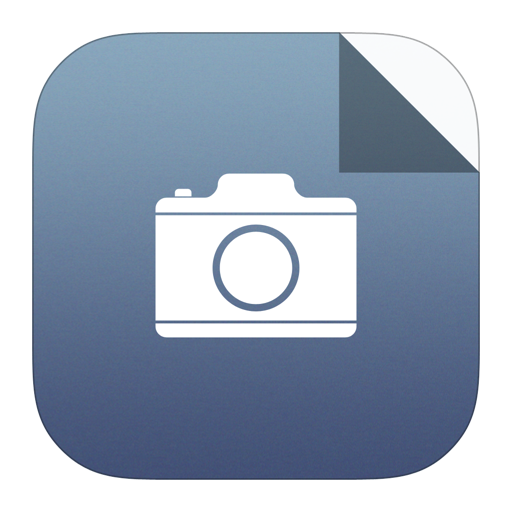
Larger image
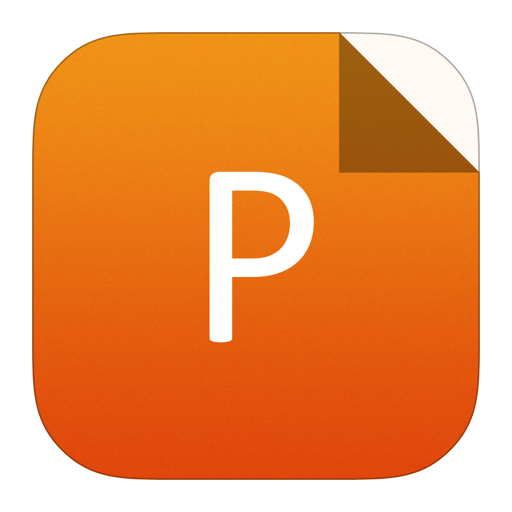
PowerPoint slide
Figure2.
(Color online) Lattice temperature distribution with different mesa radius. (a) 4.5 μm. (b) 5.5 μm. (c) 6.5 μm. (d) 8.5 μm.
Therefore, we only consider the sizes of mesa radius above 5.5 μm to compare. When the continuous current of 2.8 mA is injected, the highest temperature in the active region with a mesa radius of 6.5 μm is about 390 K and the highest temperature in the active region with a mesa radius of 8.5 μm is also about 380 K; the latter is slightly lower than the former, and differences are not much. It shows that the lattice temperature variation of the device is no longer obvious after the size of mesa increases to 8.5 μm, and reaches a steady state.
4.2
Influence of different mesa sizes on characteristics of power and voltage
VCSEL with different mesa sizes of nine groups are simulated by the simulation software. The curves of output power and voltage with current are obtained, the results are shown in Figs. 4 and 5.

class="figure_img" id="Figure4"/>
Download
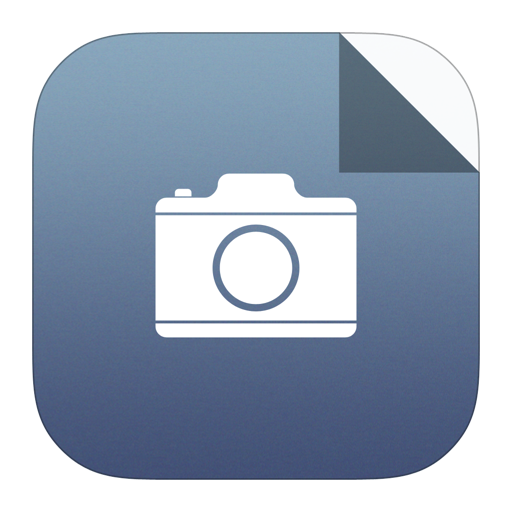
Larger image
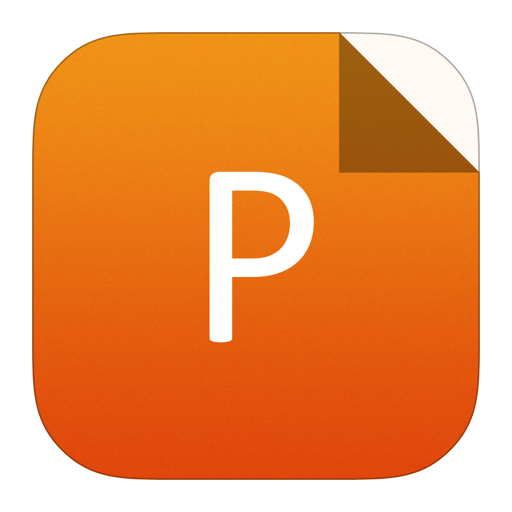
PowerPoint slide
Figure4.
(Color online) The power with the variation of current in different sizes of mesa.

class="figure_img" id="Figure5"/>
Download
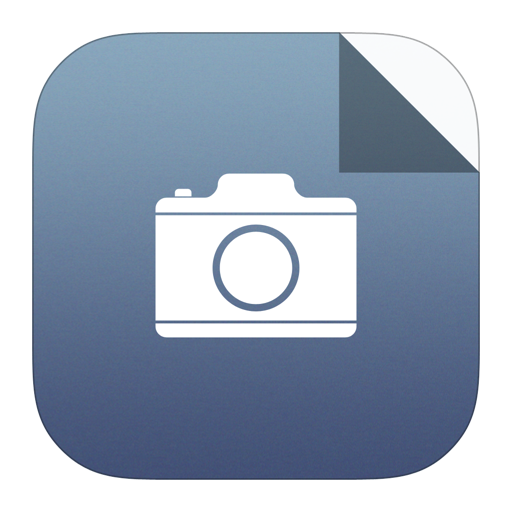
Larger image
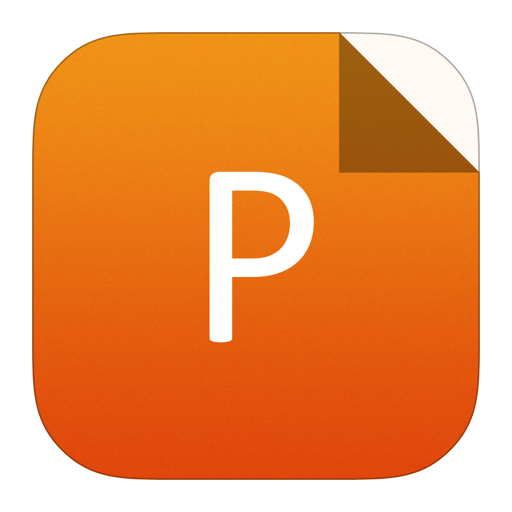
PowerPoint slide
Figure5.
(Color online) The I–V characteristic curve with different sizes of mesa.
We show the output power of 980 nm VCSEL with the variation of current (P–I) in Fig. 4. It can be clearly seen from the figure that the threshold and the saturation current increase with the increase of the mesa size, and the peak optical power also increases. The maximum output power is almost constant after the mesa is increased to 9.5 μm, which means that the size of the mesa is too large to increase the output power and power conversion efficiency of the device.
The voltage of 980 nm VCSEL with the variation of current (P–I) is shown in Fig. 5. With injection of 2.6 mA continuous current, the voltages of different mesa sizes are simulated at room temperature. As can be seen in the figure, the voltage increases with the increase of current. The voltage, where the size of mesa radius is 4.5 μm, is 3.815 V, and the voltage, where the size of mesa radius is 5.5 μm, is 3.505 V; the drop of voltage is more obvious and the difference reaches 0.346 V. When the size of mesa radius is 9.5 nm or more, the voltage is less than 3 V, the variation of voltage is less than 0.1 V. I–V characteristic curve tends to be smooth, indicating that the power conversion efficiency is increasing, however too large a size of the mesa has little effect on the output characteristics of the device.
Therefore, according to the simulation results, considering the factors of optical power and voltage, optimize the size of mesa radius between 9.5 to 11.5 μm, the maximum output power and the appropriate voltage can be obtained and the maximum power conversion efficiency is achieved.
5.
Conclusion
In this paper, the simulation software of Crosslight is used to simulate 980 nm oxide-confined VCSEL, in which the sizes of mesa radius are from 4.5 to 12.5 μm, in order to improve the output power and power conversion efficiency of devices. When the size of mesa radius is 4.5 μm, the voltage is up to 3.851 V, the peak power is only 0.203 mW, the lattice temperature is as high as 600 K, and the power conversion efficiency is too low. With the increased size of mesa, the peak power increases and the voltage decreases. When the size of mesa radius is 9.5 μm, the peak power can reach 0.38 mW, the voltage is down to 3 V, the power conversion efficiency is greatly increased, and the device is not easily damaged. When the mesa radius increased to 12.5 μm, the peak power does not become larger, although the voltage has decreased, and the size of the mesa is too large to improve the power conversion efficiency. Therefore, for 980 nm oxide-confined VCSEL of the oxide aperture of 2 μm, the sizes of mesa radius are between 9.5 to 12.5 μm, which can not only obtain the maximum output power, but also improve heat dissipation of the device, and reduce the influence of internal heating on the device, thus showing good performance.