1.
Introduction
Zinc sulphide (ZnS) is a semiconductor material with a large direct band gap between 3.4–3.70 eV depending upon composition[1, 2]. ZnS thin films have attracted the interest of many researchers during the last few years, due to their potential applications in the industrial domain, especially optoelectronic devices. In comparison with CdS, zinc sulphide (ZnS) is the most promising compound that plays an important role in the area of optoelectronic and photovoltaic applications, because of many advantages including: it has a transparent semiconductor, a wide gap, is non toxic, environmentally safe and exists on earth abundantly[3]. These advantages can be utilised in several applications such as: thin film solar cells, light emitting diodes and electroluminescent devices[4], antireflection coatings and optical filters[5]. There are many techniques such as pulsed-laser deposition (PLD), sputtering[6], chemical vapour deposition (CVD)[7], molecular beam epitaxial (MBE)[8], atomic layer epitaxial (ALE)[9], thermal evaporation[10], as well as spray pyrolysis[11], sol–gel[12], electro-deposition[13] and chemical bath deposition (CBD)[14] have been used to fabrication ZnS thin films. However, compared to the other deposition method, ultrasonic spray is widely used for the synthesis of nano-materials and high quality thin films and known as the most suitable method for preparing thin layers of ZnS, due to it beingeconomical, simple, feasible, flexible, cost effective, convenient for large-area deposition at low temperatures and it allows easy deposition in the atmospheric condition. Further, there are several factors that mainly affect the basic properties of the deposited thin films by this technique such as the deposition time, solution flow rate, substrate temperature, distance nozzle-substrate etc.
In this work, we investigate the effect of solution flow rate on the structural, optical and electrical properties of ZnS thin films prepared by ultrasonic spray technique at 450 °C. Obtained results prove the beneficial effect of this varied parameter. The prepared thin films can be used as an alternative to cadmium sulphide (CdS) and as a buffer layer in CIGS solar cells.
2.
Experimental details
The deposition system is a special setup prepared in the laboratory as shown in Fig. 1. The nozzle is fed by the solution content in the syringe pump, which is controlled by a push syringe device (to control the infusion flow rate solution) and the incoming liquid is atomized by ultrasonic wave (40 kHz) derived from a generator (Sonics vibra-cell). The obtained stream is formed by very small and uniform droplets with diameter around 40 μm. ZnS thin films were deposited on glass substrates with dimensions of 25 × 15 mm2 at 450 °C using the ultrasonic spray technique. Prior to the deposition, the glass substrates were treated in standard cleaning as follows: rinsed in acetone and ethanol for 10 min to remove grease, then washed in distilled water to remove the impurities and residuals from substrate surfaces, finally they were dried with compressed air. The starting solution was prepared by dissolving zinc chloride (ZnCl2) and thiourea (SC(NH2)2) with 0.1 M concentration in 30 mL of methanol CH3OH and it was stirred thoroughly using a magnetic stirrer at room temperature for 2 h to obtain a complete homogeneity and transparent solution. The solution was sprayed over the heated substrate held at constant temperature. The deposition parameters of ZnS thin films were all kept constant such as: the substrate temperature was 450 °C, the deposition time was fixed at 6 min, and the distance nozzle–substrate was fixed at 50 mm. The solution flow rate was varied from 10 to 50 mL/h with a step of 10 mL/h.

class="figure_img" id="Figure1"/>
Download
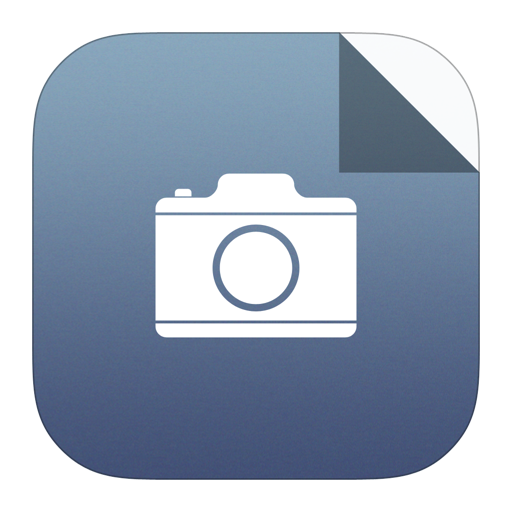
Larger image
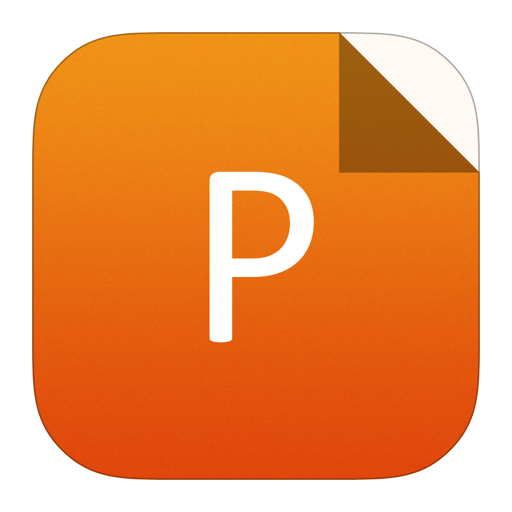
PowerPoint slide
Figure1.
(Color online) Experimental set up schematic of the used spray deposition system. 1- Spray nozzle. 2- Droplets stream. 3- Substrate. 4- Substrate holder. 5- Syringe (container of solution). 6- Thermocouple K.
The deposition parameters are summarized in Table 1. Commonly, when the solution droplets reach the heated substrate surface the following chemical reaction occurs:
Deposition parameter | Corresponding value |
Solution quantity | 30 mL |
Substrate–nozzle distance | 50 mm |
Substrate temperature | 450 °C |
Molarity of solution | 0.1 M |
Deposition time | 6 min |
Solution flow rate | 10, 20, 30, 40, 50 mL/h |
Table1.
Deposition parameters used to prepare the ZnS thin film by spray ultrasonic.
Table options
-->

Download as CSV
Deposition parameter | Corresponding value |
Solution quantity | 30 mL |
Substrate–nozzle distance | 50 mm |
Substrate temperature | 450 °C |
Molarity of solution | 0.1 M |
Deposition time | 6 min |
Solution flow rate | 10, 20, 30, 40, 50 mL/h |
$$begin{split}& { m {ZnCl}_2} +{ m {CS}}{left( { m {NH}_2} ight)_2} + { m {CH}}_{ m {3}}{ m OH} + frac{3}{2}{{ m O}_2} & qquad qquad to{ m ZnS} + { m {2NH}}_4 { m Cl}left( { m {gas}} ight) + { m {2CO}}_2left( { m {gas}} ight). end{split}$$ ![]() |
According to this reaction, a ZnS thin film should be formed onto the glass substrate surface and the 2NH4Cl, CO2 leaves the system as gas.
The structural, optical and electrical characterization of all the films were treated by many techniques. The crystalline structure of the film was characterized using an X-ray diffracto-meter (D8 ADVANCED BRUKER) with Cu-Kα radiation (λ = 1.5418 ?) within the 2θ range of 10°–70°. The surface morphology of the obtained thin films was studied using a VEGAS3 TESCAN scanning electron microscope. The optical transmittance was measured at room temperature using an UV–Vis spectrophotometer (PerkinElmer LAMBDA1050). The electrical resistivity was measured at room temperature via the four-probe method, using the JANDEL RM 3000 device.
3.
Results and discussion
3.1
Structural properties
Zinc sulphide exists in sphalerite, cubic (zinc blende) and hexagonal (wurtzite) forms. The cubic form is stable at room temperature, while the less dense hexagonal form is stable at above 1020 °C at atmospheric pressure[15]. The XRD pattern of all ZnS films prepared at different solution flow rates at the same substrate temperature (450 °C) are shown in Fig. 2. Grown films at various solution flow rates show only one preferential orientation along the (111) direction at 2θ = 28°, 75° indicating a cubic structure (JCPDS card N°: 55-0566). The (111) planes are the closest-packed ones for the cubic sphalerite structure. No other peaks related to hexagonal phases or the oxidation phases (ZnO) are seen in the XRD spectra, confirming the stoichiometric of ZnS thin films. From the XRD analysis we found that the (111) peak has a high intensity, this is due to two reasons: first, the (111) plane has the lowest surface energy[16], ascribed to the highest atomic density achieved along the (111) direction; second, that the (111) plane was parallel to the substrate surface[17]. The preferential orientation (111) was reported by several authors[18–20]. As also observed in Fig. 2, that the intensity of the (111) peak increases gradually as the solution flow rate goes up to 40 mL/h, then decreases again when the solution flow rate increases. The latter indicates an improved crystallinity of the obtained films, which can be ascribed to the increased thickness of ZnS films for a solution flow rate lower than 50 mL/h and the crystallinity decreases for 50 mL/h. Moreover, we notice that there is a small difference in the position of the ZnS (111) diffraction peak (variation in the value of the angle diffraction 2θ) that is probably caused by the manifestation of the stress during the films growth.

class="figure_img" id="Figure2"/>
Download
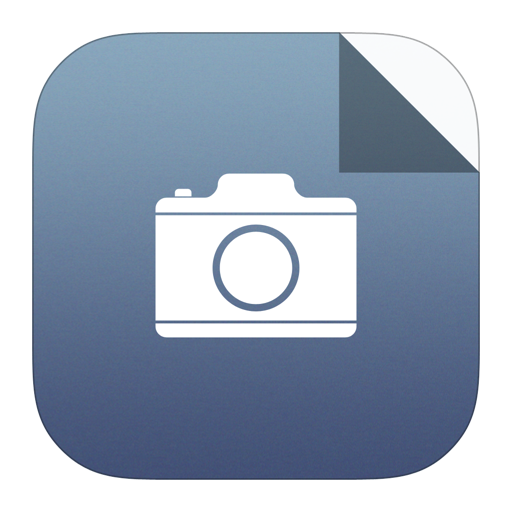
Larger image
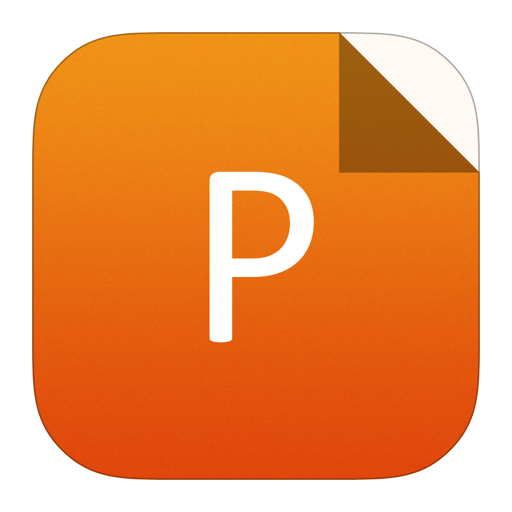
PowerPoint slide
Figure2.
(Color online) XDR pattern of ZnS films deposited at different solution flow rate.
The lattice constant (a) for the cubic phase of ZnS is determined by the expression given by Eq. (1)[21]:
$$a = d{left( {{h^2} + {k^2} + {l^2}} ight)^{frac{1}{2}}},$$ ![]() | (1) |
where h, k and l denote the lattice planes. The inter-planar spacing d is evaluated using the standard Bragg relation (Eq. (2)):
$$d = frac{{n lambda }}{{2sin theta }},$$ ![]() | (2) |
where θ is the diffraction angle and λ is the X-ray wavelength (1.5418 ?).
In Table 2 we present a summary of the calculation lattice constant (a) from the XRD pattern of the ZnS thin films at different solution flow rates.
Solution flow rate (mL/h) | hkl planes | 2θ (degree) | Calculated parameters | Reference parameter (JCPDS card No 05-0566) | |
a (?) | d (?) | ||||
10 | (111) | 28.74 | 5.379 | 3.1055 | a = 5.406 ?, d = 3.123 ? |
20 | (111) | 28.68 | 5.383 | 3.108 | |
30 | (111) | 28.74 | 5.386 | 3.1095 | |
40 | (111) | 28.72 | 5.39 | 3.112 | |
50 | (111) | 28.80 | 5.369 | 3.1 |
Table2.
Calculated lattice constant (a) from XRD pattern at different solution flow rates.
Table options
-->

Download as CSV
Solution flow rate (mL/h) | hkl planes | 2θ (degree) | Calculated parameters | Reference parameter (JCPDS card No 05-0566) | |
a (?) | d (?) | ||||
10 | (111) | 28.74 | 5.379 | 3.1055 | a = 5.406 ?, d = 3.123 ? |
20 | (111) | 28.68 | 5.383 | 3.108 | |
30 | (111) | 28.74 | 5.386 | 3.1095 | |
40 | (111) | 28.72 | 5.39 | 3.112 | |
50 | (111) | 28.80 | 5.369 | 3.1 |
The resulting changes in the lattice parameter value are related to the intrinsic stress developed in the ZnS crystal lattice.
The crystallite size of ZnS was estimated by using the well-known Debye-Scherer equation[22]:
$$D = frac{{0.94lambda }}{{beta cos theta }},$$ ![]() | (3) |
where λ is the used radiation wavelength (λ = 1.5418 ?), θ is the diffraction angle of the concerned diffraction peak, and β is the full width at half maximum (FWHM) of the diffraction peak corresponding to a particular crystal plane.
Using crystallite size values and the formula of Williamson and Smallman[23] (Eq. (4)) we can determine the dislocation density (δ) that represented the length of dislocation lines per unit volume:
$$delta = frac{1}{{{D^2}}}.$$ ![]() | (4) |
The micro strains (ε) of films were estimated using the equations[24]:
$$varepsilon = frac{{beta cos theta }}{4}.$$ ![]() | (5) |
The calculated values of crystallite size, dislocation and strain are presented in Table 3.
Solution flow rate (mL/h) | (hkl) | 2θ (degree) | FWHM (degree) | D (nm) | δ (1014 lines/m2) | ε (10?4) |
10 | (111) | 28.74 | 0.216 | 38 | 6.93 | 9.425 |
20 | (111) | 28.68 | 0.144 | 57 | 3.08 | 6.28 |
30 | (111) | 28.74 | 0.108 | 76 | 1.73 | 4.71 |
40 | (111) | 28.72 | 0.1 | 82 | 1.48 | 4.36 |
50 | (111) | 28.80 | 0.2 | 41 | 5.94 | 8.73 |
Table3.
Structural parameters of chemically deposited ZnS thin films at different solution flow rates.
Table options
-->

Download as CSV
Solution flow rate (mL/h) | (hkl) | 2θ (degree) | FWHM (degree) | D (nm) | δ (1014 lines/m2) | ε (10?4) |
10 | (111) | 28.74 | 0.216 | 38 | 6.93 | 9.425 |
20 | (111) | 28.68 | 0.144 | 57 | 3.08 | 6.28 |
30 | (111) | 28.74 | 0.108 | 76 | 1.73 | 4.71 |
40 | (111) | 28.72 | 0.1 | 82 | 1.48 | 4.36 |
50 | (111) | 28.80 | 0.2 | 41 | 5.94 | 8.73 |
Table 3 shows obviously the dependence of the crystallite size with the variation of the solution flow rate during the deposition. The crystallite size was found to increase with increasing the flow rate between 10 and 40 mL/h. The individual final sizes of the nanocrystallites depend on the number of nucleation points. The solute atoms of the liquid spread along the substrate surface and begin to bind to one another at the nucleation points and form clusters. The size of the crystals increases because the nucleation density points augment due to the increased solute atoms arriving at the substrate surface, until it touches the neighbouring nanocrystallites, which means with increasing the solution flow rate the nuclei size growth is faster than the growth rate of the films, hence the nanocrystallites sizes become larger. Imen Bouhaf et al.[25] have found that the average crystallite size between 7.26 to 19.36 nm with variation of flow rates and the similar increasing of grain size between 19 to 67 nm has been obtained by Bouhdjer et al.[26]. Above the value of 40 mL/h the grain size is reduced with increasing of the solution flow rate. This indicates the deterioration of the film crystallinity because the orientation (111) was decreased and this can be due to an increase of defects density in the film network, enabling the rise of internal strain in the formed crystallites. Valenzuela and Russer reported that the FWHM of an XRD peak is reliant on the crystallite size and the lattice strain caused by the defect and/or dislocations[27].
The dislocation density varied between 1.48 × 1014 and 6.93 × 1014 lines/m2, due to the edge of a crystal or a grain boundary, which mean the grain boundary is a dislocation trap.
3.2
Surface morphology
The surface morphology of ZnS thin films prepared at different solution flow rates is shown in Fig. 3.

class="figure_img" id="Figure3"/>
Download
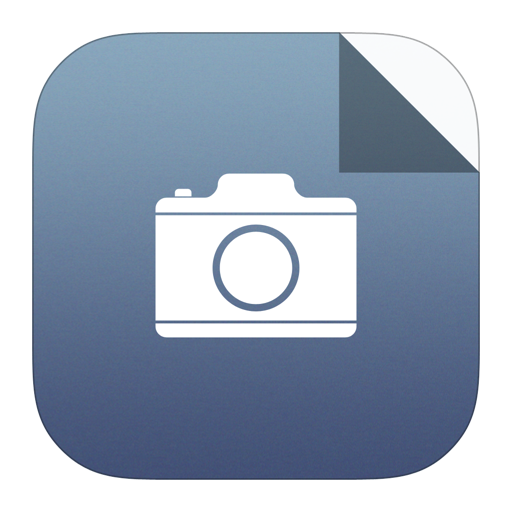
Larger image
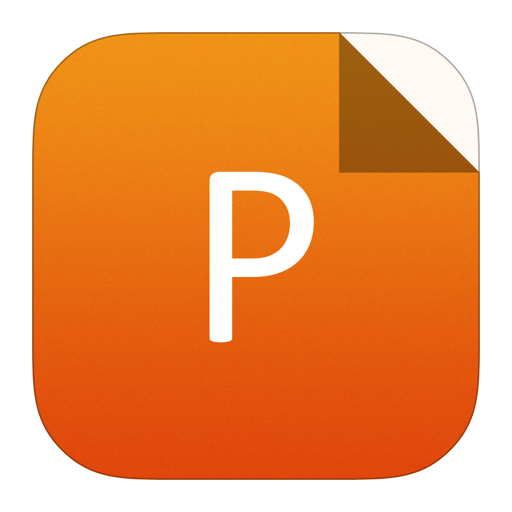
PowerPoint slide
Figure3.
Scanning electron microscopy of ZnS thin films deposited at various solution flow rates: (a) 10 mL/h, (b) 20 mL/h, (c) 30 mL/h, (d) 40 mL/h, and (e) 50 mL/h.
Evidently from Fig. 3 we conclude that the prepared thin films have the same morphology and good adherence to the substrate surfaces, the thin films display a homogenous, uniform and dense without crack and pin holes surface. As also seen in Fig. 3, the surface is covered with grains having a disk-like form with different size in all scanned areas of the samples and along the surface become larger with increasing the solution flow rate, which agree with XRD results. Moreover, larger grain sizes raise the film surface roughness causing an enhancement in the optical transmittance, and this is what makes these films suitable for potential applications in optoelectronic devices.
3.3
Optical properties
The film thickness of ZnS films deposited at various solution flow rates was determined using the cross sectional SEM images shown in Fig. 4.

class="figure_img" id="Figure4"/>
Download
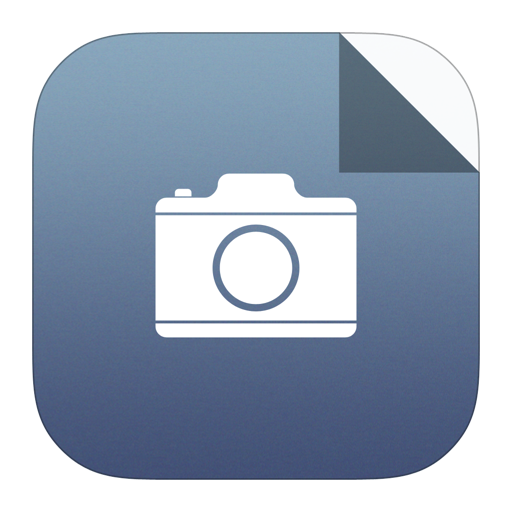
Larger image
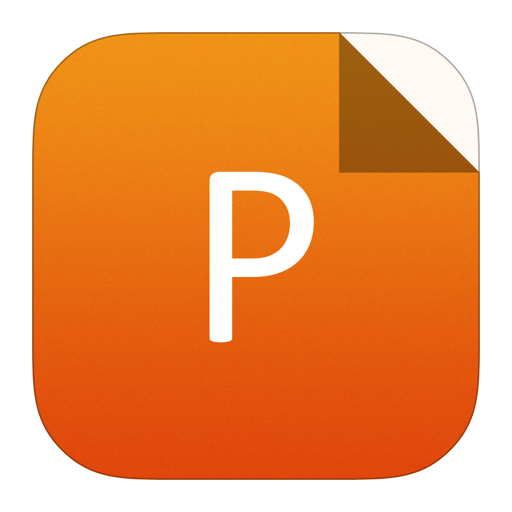
PowerPoint slide
Figure4.
(Color online) Direct thickness measurement of the film of ZnS from the cross sectional SEM images.
Fig. 5 shows the variation of the film thickness as a function of the solution flow rate. It is shown that the increased solution flow rate results in an apparent rise of the film thickness from 390 nm (10 mL/h) to 1040 nm (50 mL/h). Obtained thickness values are in good agreement with earlier reported works[28]. The highest growth rate is obtained for the film deposited with 50 mL/h, which is equal to 173 nm/min. Otherwise, the smallest growth rate equal to 65 nm/min corresponded to 10 mL/h of the solution flow rate. The increase in the deposition rate with varying the solution flow rate is suggested to be due to the increase in the kinetics of reactions during the growth of the films, which are activated thermally by means of the controlled substrate surface temperature, this allows the incoming species on the surface substrate to have enough time to find a favorable site and to form a more organized material. The resulting film thickness variation can be attributed to the increase of the amount of flow rate droplets reaching the surface substrate, hence bringing a large quantity of ingredient ions and solute atoms from the atomizer to the surface of the substrate with the increased solution flow rate. A similar increase in the film thickness has been reported by Gangopadhyay et al.[29] for ZnS thin films prepared by chemical bath deposition.

class="figure_img" id="Figure5"/>
Download
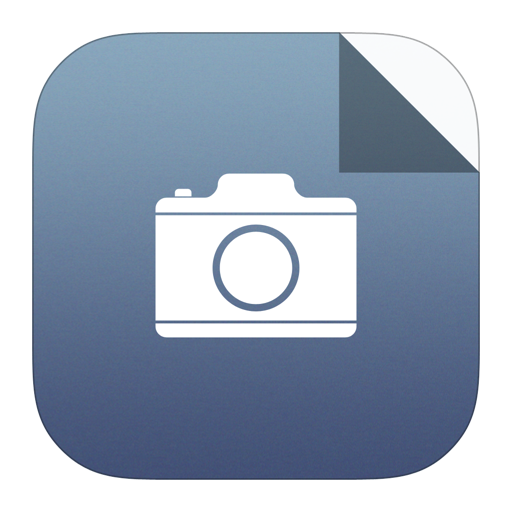
Larger image
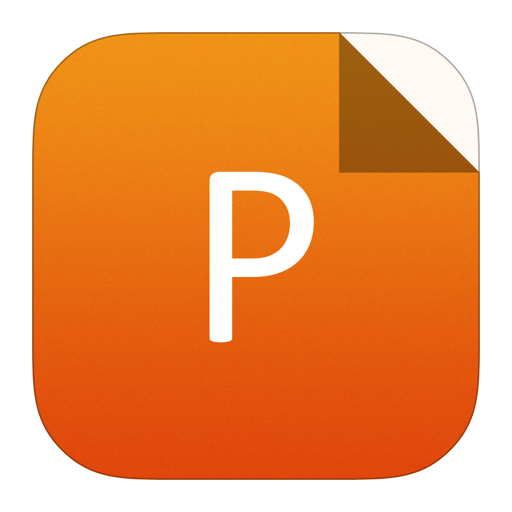
PowerPoint slide
Figure5.
(Color online) Film thickness and deposition rate as a function of solution flow rate.
The optical transmittance of the deposited ZnS films at different solution flow rate has been measured at room temperature by an UV/VIS spectrophotometer, as shown in Fig. 6, in the wavelength region of 300–1100 nm.

class="figure_img" id="Figure6"/>
Download
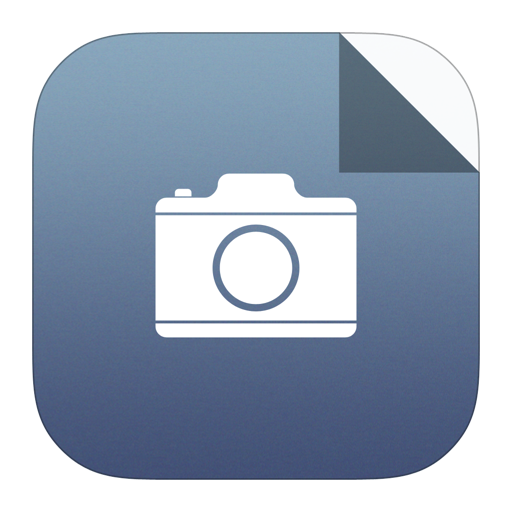
Larger image
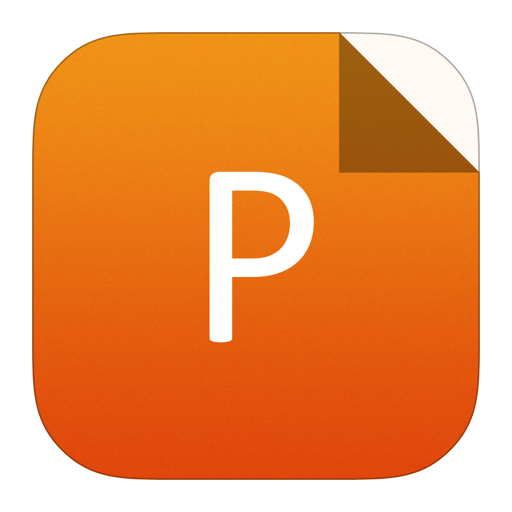
PowerPoint slide
Figure6.
(Color online) Transmission spectra of ZnS films deposited at different solution flow rates.
The spectral transmittance curves indicate a high transmission for an average transmittance of 65%–77% in the visible range, which is what makes it very appropriate to be used as a dielectric filter in the area of optics. These values were reported by several authors[30, 31]. According to Fig. 6, we conclude that the transparency of the prepared films decreased slightly after increasing the solution flow rate, this can be attributed to the increase in films thickness. The same observation was obtained by Benkhetta[32]. Furthermore, we notice the absence of interference fringes that may be due to the multiple reflections on the levels of the interfaces film/substrate and to film/air. So the absence of these fringes in our films indicates a roughness of free surface of ZnS films, which causes scattering of incident light on the material[33]. As we can see an abrupt fall of the transmission for the wavelengths less than 380 nm, which corresponds to strong absorption in ZnS following the transition between the valence band (VB) and the conduction band (CB). This last phenomenon is a very important characteristic for a semiconductor, which corresponds to the optical band gap energy (Eg).
The optical band gap of a semiconductor material can be obtained after drawing the curve of a tuk relationship[34]:
$$alpha h upsilon = K{left( {h upsilon - {E_{ m g}}} ight)^n},$$ ![]() | (6) |
where Eg is the optical band gap, K is a constant and hυ is the photon energy. The index n characterizes the transition between the valence and the conduction bands. For a direct band gap semiconductor, n is 1/2. The band gap energy (Eg) is determined by extrapolating the straight line portion of the spectrum to αhυ = 0. Fig. 7 shows the band gap energy of the ZnS films as a function of the solution flow rate.

class="figure_img" id="Figure7"/>
Download
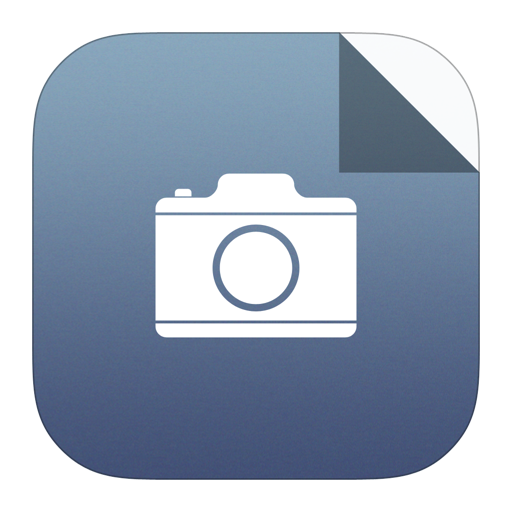
Larger image
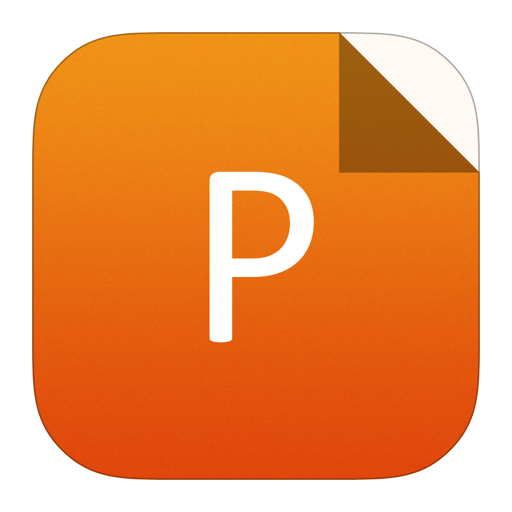
PowerPoint slide
Figure7.
(Color online) Band gap energy of the ZnS films as a function of solution flow rate.
It can be observed that the band gap slightly increases from 3.5 to 3.63 eV between 10 and 40 mL/h solution flow rates. This increase in the band gap energy (Eg) values may be attributed to the Moss-Burstein effect caused by the increase in the film thickness. According to Brustein and Moss[35] this difference is due to an increase in the charge carriers in the band of conduction. The slight increase of gap optic is a consequence of the convergence of the changing grains size values. Between the 40 and 50 mL/h flow rates the band gap energy increase quickly from 3.63 to 3.92 eV, due to the quantum size effects owing to the decrease in particle size[36] as shown in Table 3; as a result there is an increase of the grain boundary, which indicates the increasing of defects density and impurities in the ZnS thin film network. These values of the band gap energies are in good agreement with the reported values in other reports in the literature[37?39]. The widening band gap energy makes these films one of the most important candidates for use as a light emitting diode (LED) and the window layer in photovoltaic solar cells, this is due to decreases in the window absorption losses and that will cause an increase in the short circuit current[40].
3.4
Electrical properties
The resistivity of our ZnS thin films was calculated according to Eq. (7)[41]:
$$ ho = {R_{ m s}}d,$$ ![]() | (7) |
where ρ is the resistivity, RS is the square resistance (
m s}} = frac{pi }{
m {ln2}}frac{U}{I}$


class="figure_img" id="Figure8"/>
Download
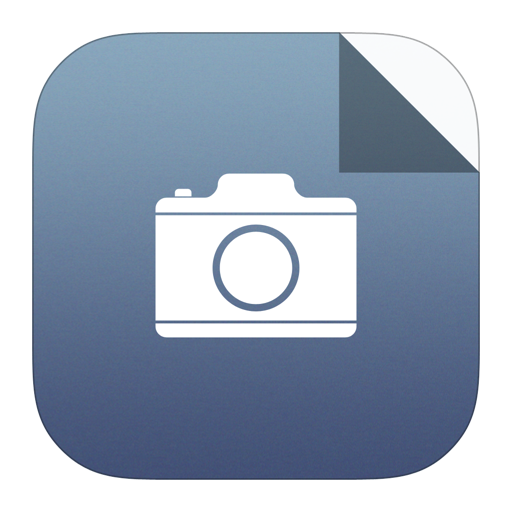
Larger image
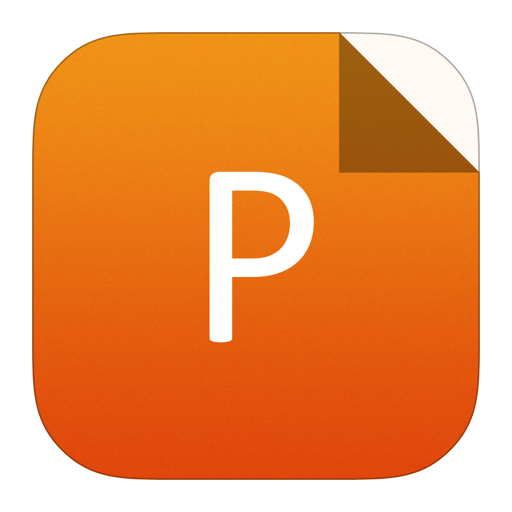
PowerPoint slide
Figure8.
Variation in electrical resistivity of the ZnS thin films at different solution flow rates.
As it is observed in Fig. 8, the resistivity was increased from 3.51 × 105 to 11 × 105 Ω·cm with increasing of the solution flow rate. These variations are less than those reported before[42, 43] and are in good agreement with the previous obtained results[28]. Some physical variations such as crystal structure, carrier concentration, mobility and grain size are controlled by the films resistivity. This increase in resistivity is due to the decreases of disorder in the films network, this decrease in disorder yields to the enhancement of the film homogeneity, while on the other hand the diminution of Zn atoms in the interstitial site, causing the reduction of free carriers concentration and subsequently the film resistivity rises. The increasing of the resistivity can be explained also by the effect of films thickness, the electrical resistivity in semiconductors is proportional to the film thickness, as seen in Eq. (7).
4.
Conclusion
In this work, the effects of solution flow rate on the structural, optical and electrical properties of ZnS thin films deposited by ultrasonic spray were investigated. The XRD measurements reveal that the crystallinity of the films can be improved using a solution flow rate up to 40 mL/h with strongly (111) preferred orientation. The average grain size is around 58.82 nm. The resistivity was found in the range of 3.51 × 105–11 × 105 Ω·cm. The film thickness increases from 390 to 1040 nm with increasing solution flow rate. Obtained results promise a potential application of ultrasonic spray deposited ZnS thin films for the solar cells.
These produced films could be useful for applications as transparent windows in solar cells or other optoelectronic devices. For example, a stoichiometric ZnS in CIGS solar cell as a buffer layer strongly increases the CIGS cell efficiency by the alteration in the electronic structure at the ZnS/CIGS interface, moreover, the ZnS has a wider energy band gap, which transmits even higher energy photons and increases the light absorption in the absorber layer.
Finally, the solution flow rate is an important and sensitive factor for controlling the quality of the ZnS thin films deposited by ultrasonic spray technique.