1.
Introduction
In recent years, ZnO has attracted a tremendous research interest due to its fantastic physical and chemical properties. ZnO is a II–VI group semiconductor material with a direct energy band gap of 3.37 eV, and a large exciton binding energy (60 mev) at room temperature[1]. It is a desirable material for many important applications in electronic and optical devices, such as solar cells, gas sensors and energy storage[2]. The ZnO thin films can be fabricated by sputtering, electrodeposition and sol–gel, etc. Among these methods, the sol–gel process is a simple and low-cost process compared to other technology[3]. In this study, the ZnO films were produced by sol–gel technology.
Graphene, an atomic-layer-thick and two-dimensional conjugated honeycomb lattice structure, has remarkable electronic and mechanic properties. Besides, as a 0 eV bandgap semiconductor, graphene has high carrier mobility, outstanding thermal/chemical stability and transmittance. The excellent properties of graphene make it suitable for optoelectronic devices such as organic solar cells, organic light-emitting diodes and ultrafast photodetectors[4]. Graphene films have been produced by many methods such as chemical vapor deposition (CVD) on metal substrates, spin coating and spray deposition, etc. Although CVD is the most successful method of producing a high-quality graphene film in these processes, it is difficult to transfer the film to an insulating material[4]. In contrast, the spin coating is the primary technique at present to fabricate the graphene oxide (GO) films that then reduced to reduced graphene oxide (RGO) films due to the ease of fabrication and economic feasibility. Supinda Watcharotone et al. first reported the transparent and electrically conductive materials with RGO films made by spin coating method[16].
RGO-ZnO composite is particularly interesting, because the RGO may provide functionality in addition to ZnO, such as photoelectric and capacitive properties[5]. Recently, several researchers have studied the composite materials of RGO-ZnO. Jijun Ding et al. have studied the PL properties of GO-ZnO nanorods and RGO-ZnO nanorods prepared by spin coating[5]. Lei Huanget al. have prepared RGO-ZnO nanorods-CVD graphene and RGO-ZnO nanorods-RGO. The electrochemical properties of synthetic 3D materials were studied. Although many studies have been reported on RGO-ZnO nanorods the RGO-ZnO films have rarely reported[6]. In this study, ZnO thin films coated with RGO thin films were prepared. Electrical and structural properties of RGO-ZnO films were researched. To study the practical application of RGO-ZnO films, we have fabricated an electrode based on the RGO-ZnO films for super capacitor. The electrochemical properties of the electrode were researched.
2.
Experimental section
2.1
Preparation of GO suspension
The oxidized graphene (GO) was prepared from natural graphite by modified Hummers method. The water in the product was removed through a drying process. Dry graphene oxide (60 mg) was added to ethanol solution (150 mL) to produce a stable suspension (0.4 mg/mL) of individual graphene oxide sheets. The GO powders in the ethanol were first treated through stirring for 48 h at room temperature. After the first step, the obtained mixtures were placed on the ultrasound (100 W) for 3 h. The suspensions were then sealed in the bottle.
2.2
Preparation of ZnO films and ZnO films coated with RGO (RGO-ZnO)
The ZnO films were synthesized by sol–gel method. The precursor of sol–gel was Zn(CH3COO)2·2H2O, and ethanol and ethanolamine were solvents and stabilizers, respectively. Zn(CH3COO)2·2H2O was dissolved in the mixed solution of ethanol and ethanolamine, which was then stirred in the water bath pot for 2 h at 60 °C. After standing for 48 h, the obtained transparent and uniform solution was then coated on the substrates by sol–gel dip-coating method. The wet films prepared on the substrates were dried at 300 °C for 10 min. After repeating the coating procedures five times the films were subjected to annealing at temperatures of 300 °C in argon atmosphere for 1 h. This pure ZnO film was labeled sample A.
The RGO-ZnO films were prepared by two steps. The first step was the growth of the ZnO film coated with graphene oxide (GO-ZnO). The second step was to reduce GO-ZnO to RGO-ZnO film. Schematic illustration for the preparation of RGO-ZnO films is shown in Fig. 1. The suspension of GO was spin coated on the ZnO films without annealing treatment at 600 rpm for 1 min, ZnO film was prepared by the same steps as sample A. The wet GO-ZnO films were dried at 130 °C for 1 min. The resulting composite film was then exposed to an atmosphere that has been saturated with vapor of hydrazine monohydrate (80%) for 18 h at 40 °C. After chemically reduced by the hydrazine vapor, the film was dried at 80 °C for 1 h. Finally, the films were heated at 600 °C at an argon atmosphere for 30 min to form consolidated RGO-ZnO films. The thickness of RGO was decided by the number of layers in the spin coating process. Herein we have prepared two RGO-ZnO film samples on quartz: samples B and C (the number of layers for samples B and C were 4 and 6, respectively). As a demonstration for the practical application of RGO-ZnO films, the ZnO and RGO-ZnO films were prepared on the Si substrates, which act as current collector, for supercapacitor application.

class="figure_img" id="Figure1"/>
Download
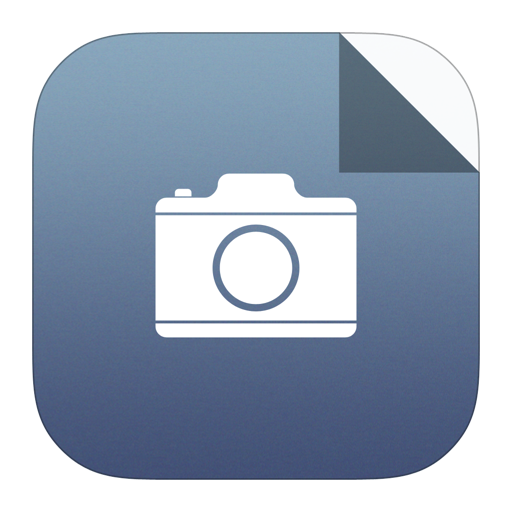
Larger image
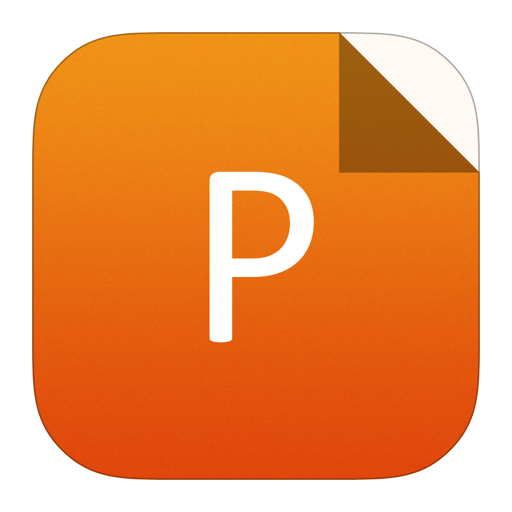
PowerPoint slide
Figure1.
(Color online) Schematic illustrations for the preparation of RGO-ZnO films.
2.3
Characterization
The crystal structure of films was characterized by X-ray diffraction (XRD) with cu Kα radiation (λ = 1.54056 ?). The microstructure and morphology of the RGO-ZnO films was observed by scanning electron microscopy (SEM, QuanTA-200 F). The optical transmittance of the RGO-ZnO films was performed with a UV/vis spectrophotometer (Hitachiu-4100). The resistivity of RGO-ZnO films was measured by a Hall measurement system (Hall 8800). Raman spectrum was collected through a Raman microscope with laser excitation wavelength of 514 nm. X-ray photoelectron spectroscopy (XPS) utilized Themo escalab 250xi with Al Kα (hv = 1486.6 eV) as source. All electrochemical measurement was carried out in 1M KOH solution as electrolyte. Cyclic voltammetry (CV) was analyzed by CHI660D electrochemical working station.
3.
Results and discussion
3.1
Characterizations of GO and RGO
Raman spectroscopy is usually used to research the compositional characteristics of graphene. The Raman spectra of GO-ZnO film (Sample C before reduction) and RGO-ZnO film (Sample C) are shown in Fig. 2. The spectrum of Raman for GO-ZnO film and RGO-ZnO film display two bonds: D band and G band. The D and G bands mainly indicate the surface defects and graphitic composition of the materials, respectively. GO-ZnO film displays D peak at 1339 cm?1 and G peak at 1587 cm?1. After reduction, the D peak and G peak shifted to 1345 and 1576 cm?1. The D peak and G peak show a measurable red shift and blue shift, respectively. ID/IG (intensity ration of D and G line) was 0.92 for GO-ZnO film and 1.21 for RGO-ZnO film. The change of D, G peak position and ID/IG indicated a reduction of GO film[7].

class="figure_img" id="Figure2"/>
Download
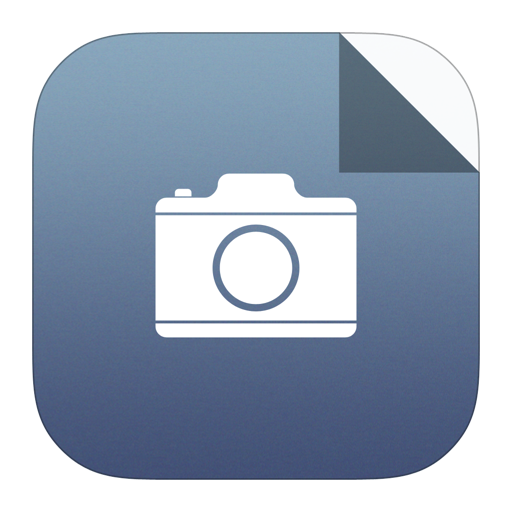
Larger image
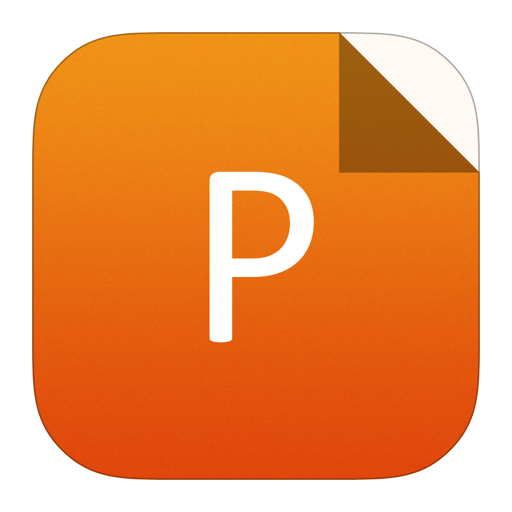
PowerPoint slide
Figure2.
(Color online) Raman spectra of ZnO, GO-ZnO and RGO-ZnO.
To further confirm this conclusion, XPS of both GO-ZnO and RGO-ZnO were collected which was shown in Fig. 3. In the XPS, the energy had been calibrated by using C peak in the surface of the sample. The surface contamination C1s (284.6 eV) was as the standard for calibration. C/O atomic ratio commonly represented the oxygen moieties present in the carbon skeleton of GO and RGO. The relative contents of carbon, oxygen and other functional groups existing in GO-ZnO film and RGO-ZnO film were determined through XPS[8]. The XPS spectra of GO-ZnO film and RGO-ZnO film indicated C1s peaks with three main components which corresponds to the carbon atoms in different functional groups: C–C (≈284.7 eV), C–O (≈286.6 eV), C=O (≈287.1 eV)[9]. After hydrazine-vapor plus annealing at 600 °C treatment of GO-ZnO film, the intensity of the peak related to the C–O bonds and C=O bonds groups decreased to a much lower value compared to the C–C bonds. It is indicating that a considerable degree of oxygen functional groups of GO-ZnO film was reduced by hydrazine-vapor plus annealing treatment[10]. By comparing the XPS results of GO-ZnO and RGO-ZnO, we find that the GO has been reduced to RGO.

class="figure_img" id="Figure3"/>
Download
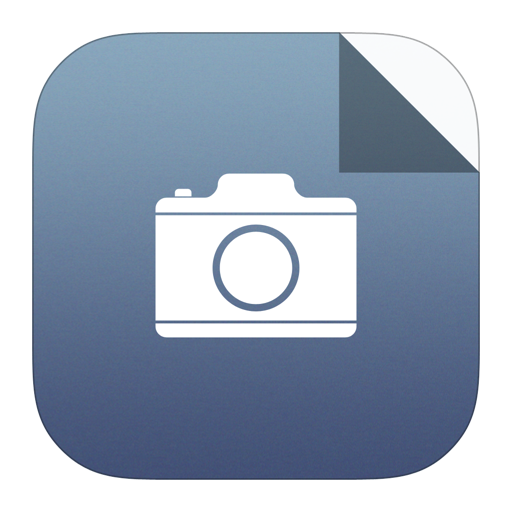
Larger image
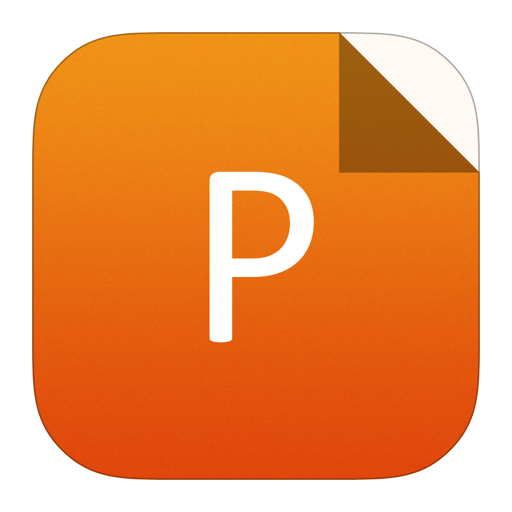
PowerPoint slide
Figure3.
(Color online) XPS pattern of graphene. (a) C1S region of GO-ZnO. (b) C1S region of RGO-ZnO.
3.2
Characterizations of RGO-ZnO films
Fig. 4(a) shows the XRD patterns of ZnO films and RGO-ZnO films prepared on quartz substrates. ZnO (002) diffraction peaks as well as several other peaks are observed in the spectrum of samples, which reveal that all the films have polycrystalline wurtzite hexagonal structure and preferred orientation along the c-axis perpendicular to the substrate[11]. However, the diffraction peak corresponding to a graphite did not appear in RGO-ZnO films. This similar result has already been reported by Jijun Ding et al.[14]; they found that GO and RGO diffraction peaks will also disappear in the composite materials. It is due to the destroyed regular stacks and limited amounts of RGO in the RGO-ZnO films.

class="figure_img" id="Figure4"/>
Download
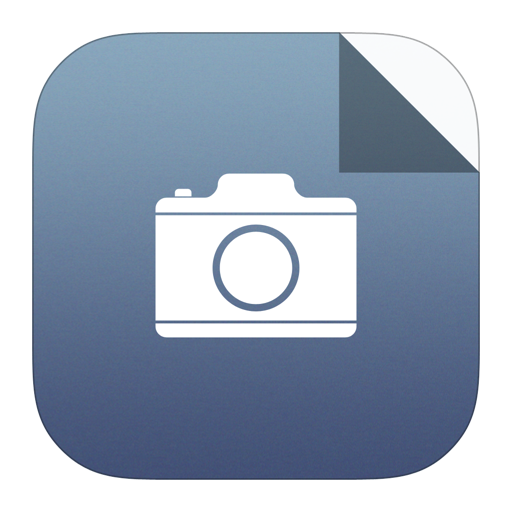
Larger image
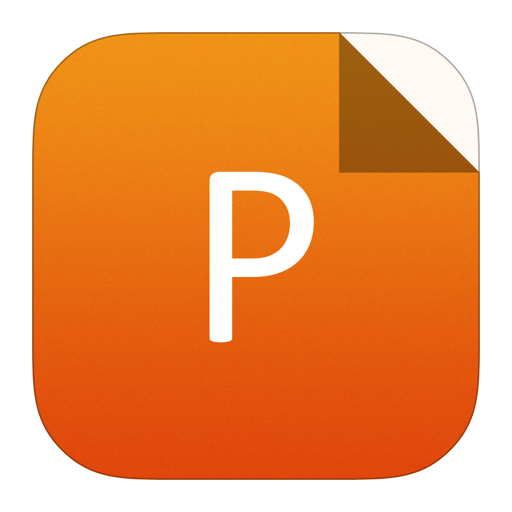
PowerPoint slide
Figure4.
(Color online) (a) XRD patterns of samples. (b) FWHM, residual stress and estimated crystallite size from XRD peaks.
The position of (002) peak is respectively located at 34.44°, 34.36°, and 34.36° for Sample A, B, C. It can be observed that the diffraction angle of RGO-ZnO (002) diffraction peaks shifted to smaller angle compared with the XRD spectrum of ZnO. The (002) diffraction intensity of ZnO film was decreased by coated RGO films. Full width at half-width (FWHM) and the grain size of the two samples are shown in Fig. 4(b). The average gain (D) size was calculated accord to the Scherrer formula:
$$ D = frac{{Klambda }}{{beta { m cos}theta }},$$ ![]() | (1) |
where K is the X-ray wavelengh, θ is the maximum of the Bragg diffraction peak, and β is the full width at half maximum (FWHM) of the XRD peak. The crystallite size of the RGO-ZnO films was smaller than pure ZnO films, which indicate that the quality of ZnO films was influenced by coating RGO films.
In order to study the structural properties of RGO-ZnO films more thoroughly, the lattice parameters of the ZnO films coated with different thickness RGO films were calculated through the following equations[11].
$$2{{{d}}_{{{hkl}}}}sin theta = nlambda, $$ ![]() | (2) |
$$frac{1}{{{{{d}}_{{{hkl}}}}^2}} = frac{4}{3}left( {frac{{{h^2} + hk + {k^2}}}{{{a^2}}}} ight) + frac{{{l^2}}}{{{c^2}}},$$ ![]() | (3) |
where θ and λ are the diffraction angle and the wavelength; hkl is the Milker indices, dhkl is the interplanar spacing for indices hkl; a, c are lattice parameters. The values of lattice constants are shown in Table 1. The lattice constant c of RGO-ZnO films is slightly larger than lattice constant of pure ZnO, which may be due to the residual stress in the films. The residual stress of films can be calculated by the biaxial model[11]
Sample | hkl | The position of (002) peak (°) | Lattice c (nm) | dhkl (nm) |
A | 002 | 34.44 | 0.5202 | 0.2601 |
B | 002 | 34.36 | 0.5214 | 0.2607 |
C | 002 | 34.36 | 0.5214 | 0.2607 |
Table1.
Various parameters of different samples.
Table options
-->

Download as CSV
Sample | hkl | The position of (002) peak (°) | Lattice c (nm) | dhkl (nm) |
A | 002 | 34.44 | 0.5202 | 0.2601 |
B | 002 | 34.36 | 0.5214 | 0.2607 |
C | 002 | 34.36 | 0.5214 | 0.2607 |
$$delta = { m{-}}233 frac{{C-{C_0}}}{{{C_0}}} left( {{ m{ GPa}}} ight),$$ ![]() | (4) |
where C0 is the lattice constant of bulk ZnO films. Fig. 4(b) indicates that the residual stress in samples A, B and C are 0.17, 0.35, and 0.35 GPa, respectively.
Fig. 5 shows the SEM images of (a) as prepared ZnO films, RGO-ZnO films (b) and (c), cross-sectional images of RGO-ZnO (d). In Fig. 5(a) for sample A, it was clearly seen that ZnO films were compact and smooth. Figs. 5(b) and 5(c) show that the large-scale RGO films have been successfully prepared on the ZnO films. Compared with ZnO films, the RGO-ZnO films were rough. The average grain size of RGO-ZnO was smaller than the pure ZnO films. All the results imply that the crystal quality of RGO-ZnO was worse than ZnO films, which agree with the XRD spectrum. The cross-sectional SEM image of sample C is shown in Fig. 5 (d), which confirmed that the ZnO films were enveloped by thin transparent RGO sheets.

class="figure_img" id="Figure5"/>
Download
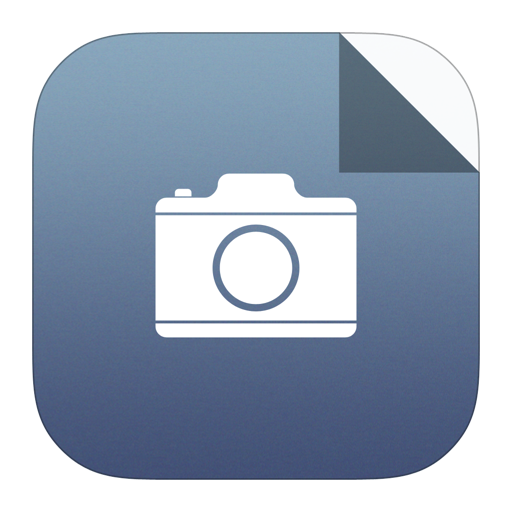
Larger image
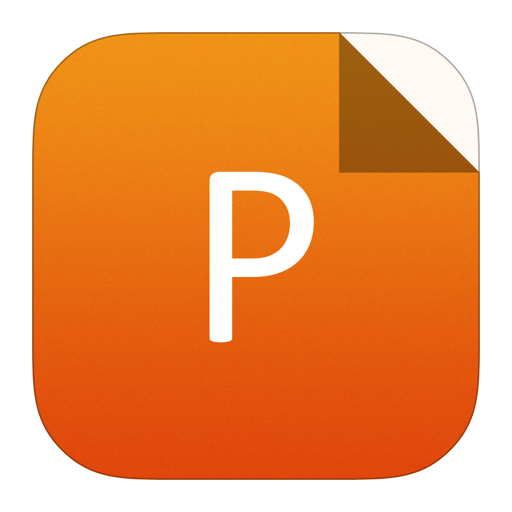
PowerPoint slide
Figure5.
SEM images of films. (a) Sample A, RGO-ZnO films. (b, c) Samples B, C. (d) Cross-sectional images of RGO-ZnO (Sample C).
Fig. 6 shows PL of spectra of ZnO and RGO-ZnO (Sample C). The PL spectrum of ZnO films exhibits broad visible emission at 490 nm an and shape emission line around 390 nm. The emission peak around 390 nm of ZnO originated from near-band-edge emission, which can be related to free or donor bound excitonic emissions. However the deep-level emission that occurs in the visible wavelength region is mostly related to structural lattice defects such as oxygen vacancies and zinc interstitial[12]. The intensity of emission peak at 390 nm significantly increased when ZnO films were coated with RGO, which is contrary to many previous reports[5]. The increase of intensity of emission peak may be due to the collective effect of the high temperature treatment and RGO. In this study, the GO films were reduced to RGO films by high temperature treatment. These acquired thin transparent RGO sheets enveloped on the ZnO films have promoted exciton recombination in the ZnO. It is interesting to note that the visible emission at 490–550 nm from RGO-ZnO was not only blue shifted as compared to that of pure ZnO but also is significantly quenched. The quenching behavior is due to the charge transfer between ZnO and RGO[5]. From the investigation above, the RGO sheets play an important role in the associated radiative recombination process. The PL spectrum is a reliable tool to monitor the interaction of ZnO with RGO.

class="figure_img" id="Figure6"/>
Download
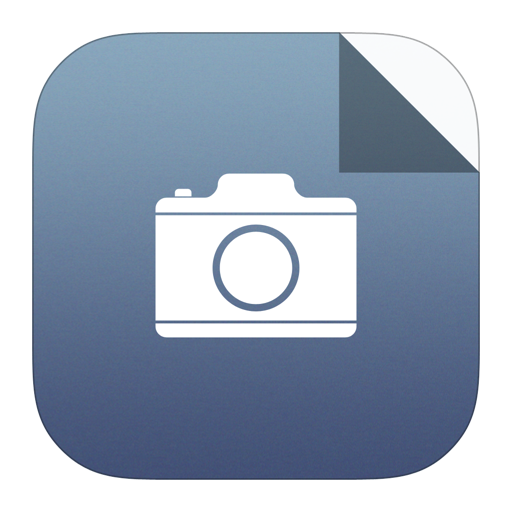
Larger image
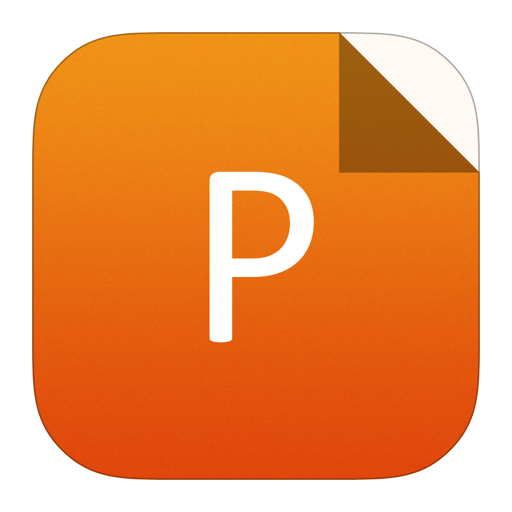
PowerPoint slide
Figure6.
(Color online) PL spectra of as-prepared RGO-ZnO (Sample C) and ZnO at room temperature.
3.3
Electrical properties of RGO-ZnO
Graphene, which has remarkable electronic and mechanic properties, acts as conducting channel after coating on the ZnO films. The electrical properties of ZnO and RGO-ZnO are shown in Fig. 7. Regarding the conductivity, the resistivity of RGO-ZnO films (Samples B, C) was measured to be 0.14 and 0.56 Ω·cm respectively, which is less than ZnO films (16 Ω·cm). The resulting electrical values show that GO films partly reduced and then improved the electrical conductivity of films. The main reason for this phenomenon: First, the RGO surface and its interface with ZnO are used as anchors for charge carriers. Second, graphene shows bipolar nature, so it can transfer electrons and holes almost immediately, which can increase the carrier concentration[12].

class="figure_img" id="Figure7"/>
Download
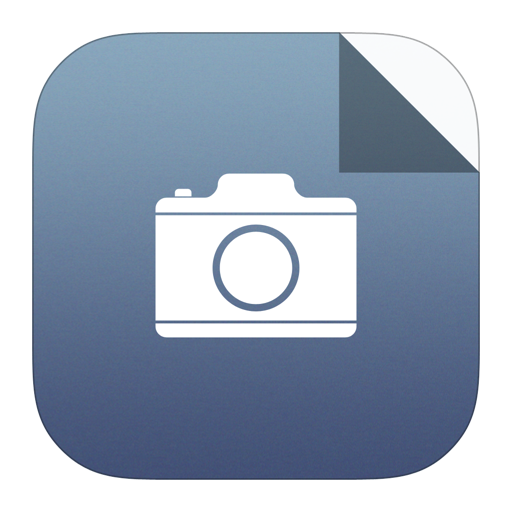
Larger image
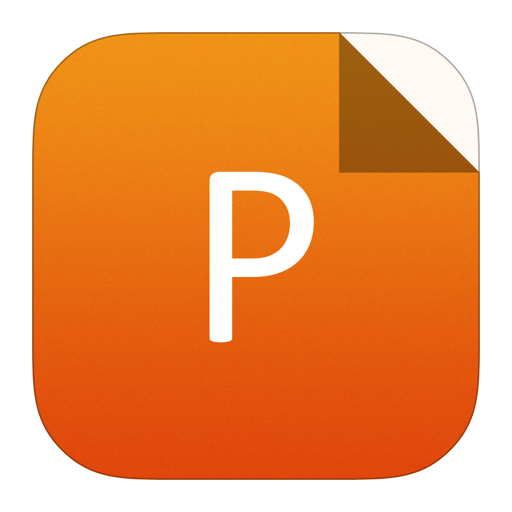
PowerPoint slide
Figure7.
(Color online) The resistivity of ZnO and RGO-ZnO films.
3.4
Electrochemical measurements of ZnO and RGO-ZnO
Herein we fabricated an electrode, using RGO-ZnO films fabricated on Si substrate as active materials for super capacitor application. RGO-ZnO film structure was successively built on the silicon substrate, as is shown in the inset of Fig. 8(b). The SEM of RGO-ZnO/Si is illustrated in the Fig. 8(d). To demonstrate that the RGO-ZnO composite materials couples possess the properties of super capacitor, the electrochemical properties of the electrode were tested. Fig. 8(a) shows the C–V curves for ZnO and RGO-ZnO at scan rate of 0.02 V/s. The shapes of C–V loop for ZnO and RGO-ZnO composites electrode are partially rectangle shape, indicating capacitive behavior of capacitor[13]. As Fig. 8(a) shows, it can be seen that the current level and area of C–V curves are higher for RGO-ZnO than pure ZnO, which clearly indicates better capacitive performance of electrode.

class="figure_img" id="Figure8"/>
Download
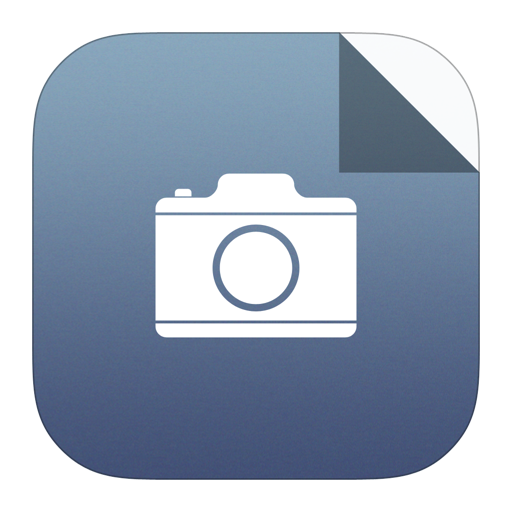
Larger image
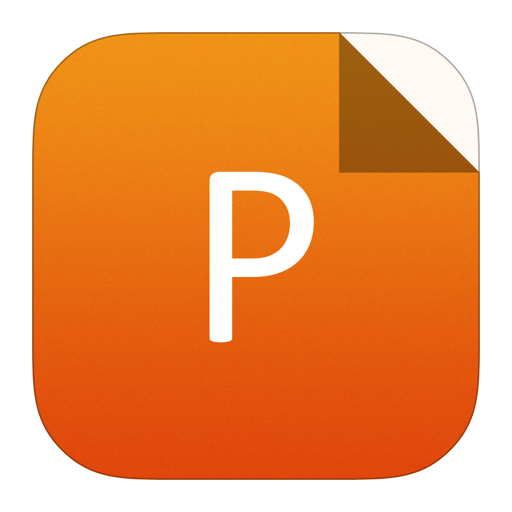
PowerPoint slide
Figure8.
(Color online) (a), (b) CV curves of ZnO and RGO-ZnO electrode. (c) Impedance spectra of ZnO and RGO-ZnO. (d) SEM of as-prepared RGO-ZnO/Si.
C–V of the as-prepared RGO-ZnO electrode at different scan rates (5, 20, 50, 100 mV/s) in the potential range of –1.0 to 0 V are shown in Fig. 8(b). It can be seen from the C–V curves that the current increases as the scanning rate increases. As seen from the figure, it shows deviation from an ideal rectangular shape with some redox peaks due to Faradaic reaction of materials. Electrochemical impedance spectroscopy (EIS) is an informative technique to evaluate the electrochemical characteristics of the electrode/electrolyte interface[2]. Fig. 8(c) shows the impedance spectra of ZnO and RGO-ZnO in the frequency range from 100 kHz to 0.01 Hz. In the low-frequency region, the impedance pattern of RGO-ZnO increases sharply and tends to become vertical which is due to the capacitive properties of the electrode. A straight line at an angle of 45° to the x axis can be seen that results from the permeation process of the electrolyte ions in the electrode material of RGO-ZnO. The Warburg impedance associated with the diffusion resistance of the electrochemical system can be observed in the RGO-ZnO electrode[14]. As a contrast, the ESR of the electrode without RGO performs badly, which shows worse capacitive properties. These results demonstrate that the RGO-ZnO electrode could be used for supercapacitor applications.
4.
Conclusion
ZnO films coated with reduced graphene oxide (RGO-ZnO) were prepared by a simple chemical approach. The graphene oxide (GO) films deposited on ZnO films by spin coating were reduced to RGO films by two steps (exposed to hydrazine vapor for 12 h and annealed at 600 °C). The PL properties of ZnO and RGO-ZnO were compared. Results demonstrated that RGO-ZnO hybrid films exhibit broad visible emission at 490 nm and shape emission line around 390 nm. The RGO surface and its interface with ZnO are used as anchors for charge carriers. The resistivity of RGO-ZnO films (Sample B, C) were measured to be 0.14 and 0.56 Ω·cm respectively, which is lesser than ZnO films. We fabricated an electrode, using RGO-ZnO films deposited on Si substrate as active materials for super capacitor application. The shape of C–V loop for RGO-ZnO composites electrode are partially rectangle shape, indicating capacitive behavior of capacitor. Electrochemical impedance spectroscopy (EIS) was used to evaluate the electrochemical characteristics of the electrode/electrolyte interface. The results of EIS andC–V curves illustrate that the RGO-ZnO electrode could be used for supercapacitor applications.