1.
Introduction
Owing to the high electron mobility, high breakdown electric field, high density of the two-dimensional electron gas (2DEG), and suitability for commercial Si-based processes, AlGaN/GaN high electron mobility transistors (HEMTs) on silicon substrate are promising candidates for high frequency power switching applications[1, 2]. However, AlGaN/GaN HEMTs also suffer some challenges in application, such as normal-off devices, current collapse, higher work voltage and lower leakage current[3–5], etc. The possibility to achieve a low leakage current with AlGaN back-barrier due to improved 2DEG confinement and improved breakdown voltage has been demonstrated[6, 7]. Through studying the growth condition of GaN HEMTs with different AlGaN back-barrier thicknesses, the back-barrier with optimal design can significantly reduce the GaN channel electron spillover and improve electron mobility[8]. With the increasing of the Al composition of the AlGaN back-barrier, the decrease of 2DEG density and the enhancement of mobility were found, the RF characteristics also were improved[9, 10]. GaN HEMTs with AlGaN back-barrier have shown the ability to suppress leakage current and enhance breakdown voltage, while suffering from low saturation current[6, 10–12]. Besides, comparing with the GaN HEMTs on Si without AlGaN back-barrier, the defect density in the GaN channel of that with the AlGaN back-barrier will decrease due to lower lattice mismatch. Many efforts have also been made in the device with the GaN channel below 200 nm for dynamic on-resistance and DC characteristics[6, 10, 13]. Nevertheless, due to the drain-induced barrier lowering (DIBL) effect in a thin GaN channel, poor breakdown voltage still is observed in the GaN HEMTs.
In this work, we investigated the influence of GaN channel and AlGaN back-barrier thickness on the breakdown characteristics of AlGaN/GaN MIS-HEMTs. Besides, to exclude the effect of gate current leakage on the breakdown characteristics of the devices, SiNx dielectric deposited by low-pressure chemical vapor deposition (LPCVD) was adopted as the gate dielectric due to its high quality and low leakage[4].
2.
Device fabrication
Three AlGaN/GaN MIS-HEMTs wafers, named sample A, sample B and sample C were grown on 4-inch Si substrate by metal-organic chemical vapor deposition (MOCVD) with 120 nm AlN nucleation layer, 600 nm stepped AlGaN buffer layer, Al0.25Ga0.75N back-barrier, GaN channel and 20 nm of Al0.24Ga0.76N barrier layer. Different GaN-channel/AlGaN-back-barrier thicknesses of 800 nm/1500 nm, 1000 nm/ 1000 nm and 2000 nm/500 nm with similar total thickness were grown for sample A, B and C, respectively, to investigate their influence on the device performance and eliminate the influence of the total epitaxial thickness. Fig. 1 shows the schematic structure diagrams of three samples.

class="figure_img" id="Figure1"/>
Download
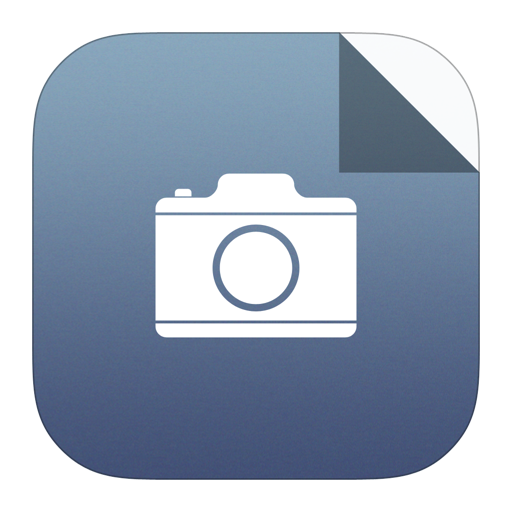
Larger image
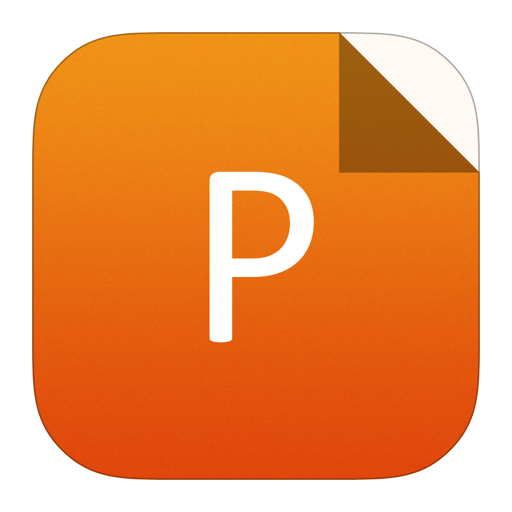
PowerPoint slide
Figure1.
(Color online) Schematic diagrams of AlGaN/GaN HEMT structures for three samples.
The fabrication process started with insulator deposition. A 20-nm-thick LPCVD-SiNx insulator was deposited at 780 °C as the gate dielectric and passivation layer. Device isolation was achieved by multi-energy fluorine ion implantation. After opening the source and drain windows by the neutral loop discharge (NLD) etching, O2 plasma treating for 5 min at 300 W and HCl : H2O = 1 : 10 dipping for 3 min were carried out in order to eliminate the etching damage and remove the native oxide layer. Ohmic contacts were formed by an alloyed Ti/Al/Ni/Au (20 nm/130 nm/50 nm/50 nm) metal stack and annealed at 850 °C for 30 s in N2 ambient. Ni/Au (50 nm/150 nm) contacts were evaporated by an e-beam evaporator for the gate metal. The source–drain and source–gate spacing of all devices were 23 and 5μm, respectively. The gate length and width were 2 and 100 μm, respectively. All DC properties of the devices were measured by the Agilent B1505A and Cascade 150.
3.
Results and discussion
The transfer and output curves of three devices are shown in Fig. 2. Transfer characteristics were measured with VDS at 10 V and output curves were attained with the VGS stepped between ?12 and 0 V with the step of 2 V. The off-state current IOFF for three samples are as low as 6.11 × 10?10 A/mm with an ION/IOFF of ~109. The threshold voltage, VTH, defined by the leakage current @ 1 μA/mm of transfer curves[14], are ?9.2, ?9.8, and ?10.2 V for sample A, sample B, and sample C, respectively. The decrease of threshold voltage is due to the increase of GaN channel thickness and the consequent increase of electron density. The increase of GaN channel thickness also leads to the decrease of the transconductance, with 77.2, 76.9, and 74.3 mS/mm for samples A, B, and C, respectively. The saturation current densities of three devices are 530.0, 528.5, and 527.8 mA/mm, and the on-state resistances are 11.9, 10.7 and 10.3 Ω·mm for samples A, B and C, respectively. More details of all samples are shown in Table 1.
Parameter | Ron (Ω·mm) | Saturation current (mA/mm) | Subthreshold swing (mV/dec) | Maximum Gm (mS/mm) |
Sample A | 11.9 | 530.0 | 75.2 | 77.2 |
Sample B | 10.7 | 528.5 | 80.3 | 76.9 |
Sample C | 10.3 | 527.8 | 95.3 | 74.3 |
Table1.
Summary of the main DC characterization results.
Table options
-->

Download as CSV
Parameter | Ron (Ω·mm) | Saturation current (mA/mm) | Subthreshold swing (mV/dec) | Maximum Gm (mS/mm) |
Sample A | 11.9 | 530.0 | 75.2 | 77.2 |
Sample B | 10.7 | 528.5 | 80.3 | 76.9 |
Sample C | 10.3 | 527.8 | 95.3 | 74.3 |

class="figure_img" id="Figure2"/>
Download
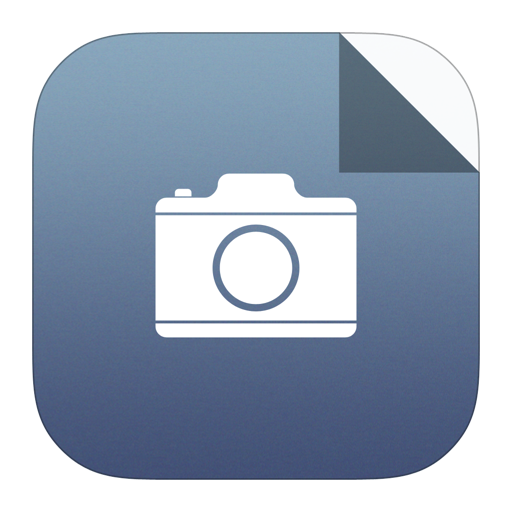
Larger image
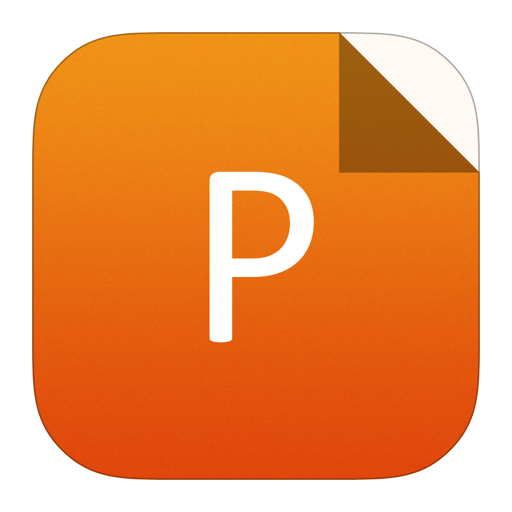
PowerPoint slide
Figure2.
(Color online) The (a) linear and (b) semi-log scale of transfer curves with VDS at 10 V and (c) output curves with VGS varying between ?12 and 0 V with a step of 2 V of three samples.
Fig. 3 shows the capacitance–voltage (C–V) curves and the extracted 2DEG concentrations of the three samples with a structure shown in the inset of Fig. 3(a). The sheet 2DEG concentrations are 0.83 × 1013, 0.87 × 1013 and 0.93 × 1013 cm?2, for samples A, B, and C, respectively. The large polarization-induced electric field in the AlGaN back-barrier will raise the conduction band edge of the GaN channel[6], as shown in Fig. 3(c). The higher potential of GaN channel is expected to decrease the sheet 2DEG concentrations. The VTH of the three samples from the C–V curves and the transfer curves show the same trend but different values. This is due to the response of deep-level bulk traps in the GaN channel and AlGaN barriers at different scan speeds[15].

class="figure_img" id="Figure3"/>
Download
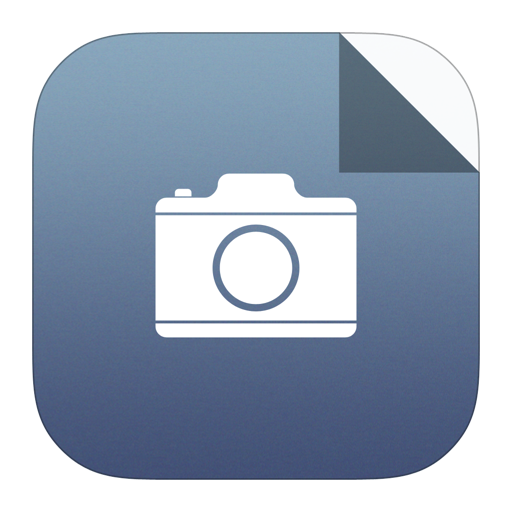
Larger image
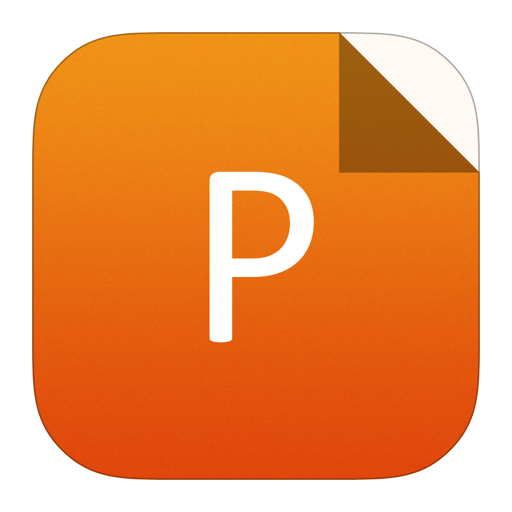
PowerPoint slide
Figure3.
(Color online) (a) C–V characteristics of AlGaN/GaN MIS-HEMTs for three samples (the inset is the structure of C–V measuring). (b) 2DEG sheet concentrations of three samples. (c) Schematic band diagram of AlGaN/GaN heterostructures for three samples.
The breakdown characteristics of three devices were measured. The off-state gate voltage for three samples was set at VGS = ?15 V, as shown in Fig. 4. It can be displayed clearly that the breakdown voltage increased with the increase of AlGaN back-barrier thickness. At low voltages (VDS < 400 V), the off-state leakage of three samples shows a similar trend or the off-state leakage current of three samples shows a slight dependency on the channel thickness at lower bias. This also indicated that the leakages of the three samples are not affected by the conduction band raising effect by the AlGaN back-barrier resulting in preventing electrons penetrating [16] since all the samples have thick GaN channel layers. However, compared with samples B and C, the leakage of sample A shows a completely different trend at high voltages. It could be inferred that the off-state leakage at high voltages will mainly be affected by the thickness of the GaN-channel/AlGaN-back-barrier, and a too large and thick GaN channel will introduce a continued increase of off-state leakage and consequently a low breakdown voltage. With the bias increasing, the negative bias between the gate and the channel will be higher, and the electrons would be pushed in AlGaN, the leakage current path is mainly due to the GaN channel layer at lower bias for all samples (the red solid line), as shown in Figs. 5(a) and 5(b). The back-barrier acts as the second leakage path especially for the ones with a thinner channel, due to the weakened confinement of the back-barrier at higher bias. This will result in the very slow increase in the region of sample A between 300 and 800 V partly due to acceptor traps introduced by the unintentionally doped Carbon in the AlGaN[17]. Then the off-state leakage current of sample A comes to a further increase with the further increase of bias beyond 800 V, and it is suggesting that the acceptor traps in the AlGaN back-barrier are completely filled with electrons[18].

class="figure_img" id="Figure4"/>
Download
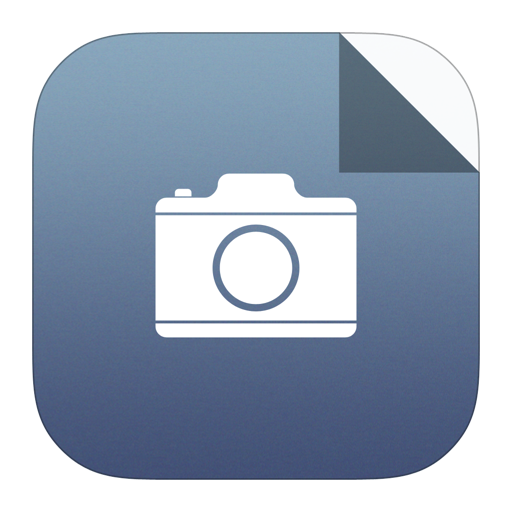
Larger image
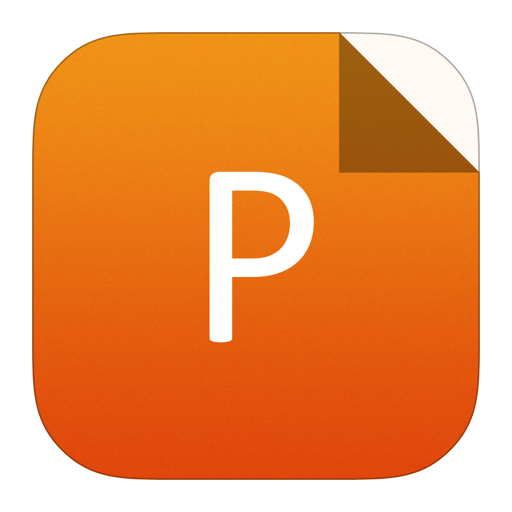
PowerPoint slide
Figure4.
(Color online) Breakdown characteristic of samples A, B and C at VGS = ?15 V.

class="figure_img" id="Figure5"/>
Download
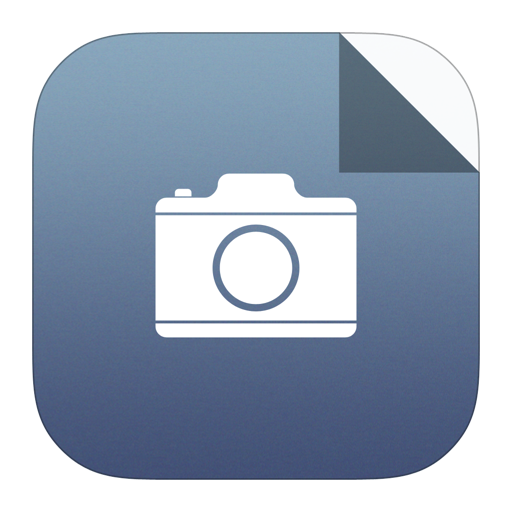
Larger image
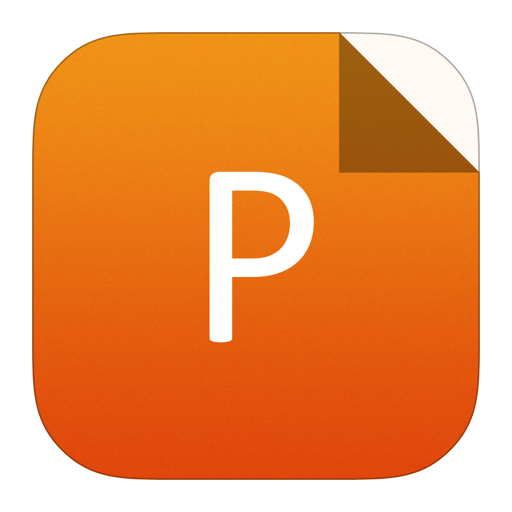
PowerPoint slide
Figure5.
(Color online) Schematic diagram of leakage current for samples with (a) thick GaN channel and (b) thin GaN channel.
It should also be noticed that the leakage current of sample B is lower than that of samples A and C. To clarify this problem, the logarithmic ID–VDS curves were plotted, as shown in Fig. 6. The inflexions (sudden change in the slope of the curve) were found at 133 and 216 V for sample A (or C) and sample B, respectively. This inflexion represents the traps-filled-limit voltage of traps based on the space charge limited current conduction[18], which means that acceptor traps were completely filled with the electron. In this theory, the density of acceptor traps in sample B is much higher than that of samples A and C. This explanation could be identified by the C–V characteristic at low frequency in the inset of Fig. 6. When the VG at the positive bias is above 0.98 V, the curve of sample B has an evident surge increase due to the trap effects in the material[19]. The full width at half-maximum (FWHM) values of the (002) diffraction peak for samples A, B, and C are 780, 1294 and 562 arc sec respectively. The significantly broadened (002) FWHM of sample B suggests that the high density of dislocations for sample B.

class="figure_img" id="Figure6"/>
Download
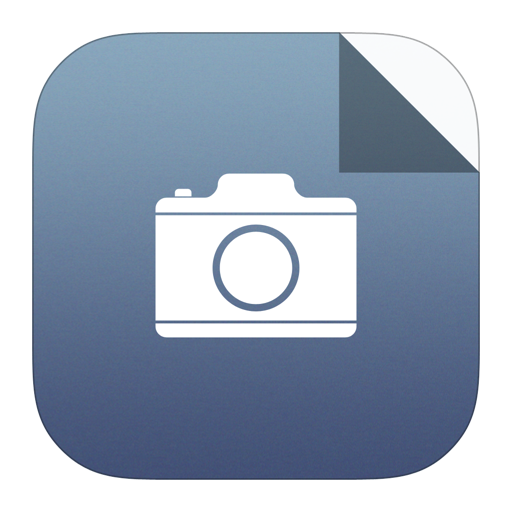
Larger image
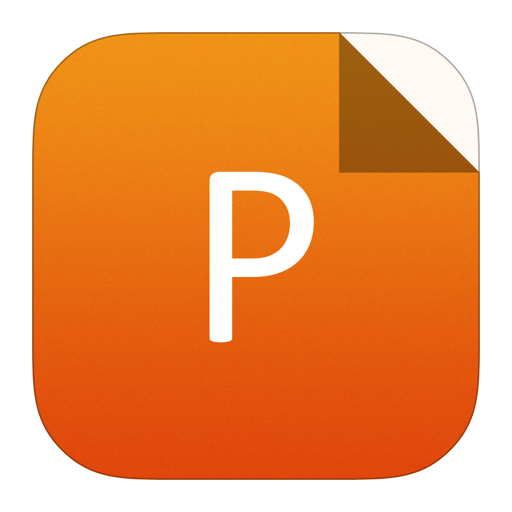
PowerPoint slide
Figure6.
(Color online) log IDS–log VDS characteristics from 0 V to high voltage for three samples. The inset is the low frequency C–V characteristics at 1 kHz.
4. Conclusions
We discussed the influence of the thickness of GaN-channel/AlGaN-back-barrier on the breakdown characteristics of AlGaN/GaN MIS-HEMTs. Although the 2DEG density increased with the increase of the GaN channel thickness, this also led to the continued increase of off-state leakage current thicker than 800 nm in this work. On the other hand, the breakdown voltage of AlGaN/GaN MIS-HEMTs would be improved remarkably by appropriately reducing the thickness of GaN channel and improving the thickness of AlGaN back-barrier.
Acknowledgments
We thank the Suzhou nanofabrication facility of SINANO, CAS, for the fabrication, characterization and testing of the AlGaN/GaN MIS-HEMT. We also thank the Tang Optoelectronics Equipment Co. Ltd, for the growth of the AlGaN/GaN HEMTs.