全文HTML
--> --> -->法布里-珀罗谐振腔(Fabry-Pérot, FP)谐振腔有着悠久的历史, 1899由Pérot和Fabry提出并设计[9]. 在光学领域中, FP谐振腔是指由两片平行反射面(反射镜)构成的光学腔. 只有满足FP谐振腔的共振条件, 光波才能穿过该谐振腔[10]. FP谐振腔具有控制、测量光波的能力, 广泛应用于通讯、激光、光谱学、传感器等领域[11-14]. 随着加工制造技术的提高, 多种可调谐FP谐振腔被设计制造[15,16]出来. 最近, 一种集成微流腔的FP生物传感器被提出. 通过调节FP谐振腔两面反射镜间的有效介质, FP谐振腔可以揭示其对相移改变或吸收率改变的敏感度[13,14]. 因此, FP谐振腔是一种设计无标签生物传感器的优秀方法. FP谐振腔传感器结构简单, 制备成本低, 可以有效地替代其他用于检测溶液中生物成分的光学生物传感器.
二维范德瓦尔斯(2D van der Waals, 2D vdW)材料吸引了大量的研究. 2D vdW材料是由层状晶体构成, 层与层之间通过范德瓦尔斯力约束, 具有很多独特的光学性质. 自从石墨烯于2004年被成功制备[17]以来, 越来越多的2D材料加入了2D vdW材料这个大家庭. 石墨烯具有典型的对称晶体结构, 表现出各向同性的物理性质. 另一类2D vdW材料的晶体结构呈现不同程度的不对称性, 如黑磷(black phosphorus, BP)[18-23]、碲化镓 (gallium telluride, GaTe)[24-26]、六角氮化硼(hexagonal boron nitride, hBN)[27,28]. 由于晶体结构的不对称性, 这类2D vdW材料不仅能如各向同性的石墨烯一样具有高开关比、高电子移动性等2D材料的性质, 还可以表现出电学、光学、热学等方面的各向异性. 这种各向异性丰富了物理学应用, 并为设计可调谐装置提供了新的维度. 其中, 光学2D vdW材料是设计实现新型光学极性设备的优良选择[29-32]. α型三氧化钼(α-phase Molybdenum trioxide, α-MoO3)是其中的佼佼者, 作为一种新兴天然2D vdW材料, 具有非对称晶体结构, 表现出独特的各向异性光学性质. 相较其他双折射率材料, 比如BP和CaCO3, α-MoO3实现了低光学耗散和高折射率比的良好平衡. 最近, Zheng等[33]在近红外波段证实了通过照射极性材料—α-MoO3可以产生一种混合准粒子, 即声子极化子(phonon polaritons, PhPs). 这一研究引起了广泛关注, α-MoO3的PhPs得到了进一步深入的研究, 多种新型纳米光学设备得以发展, 比如宽谱吸收器、高品质因数极化子谐振器、亚波长光学调谐和聚焦设备[33-36]. 与人工超材料相比, 天然2D vdW通常具有更低的光学损耗, 也不需要复杂的光刻技术来实现功能.
比色生物传感器是一种以颜色变化为检测分析基础, 可以通过裸眼或分光光度计进行观察、检测, 进而对待测目标物进行定性或定量检测的光学生物传感器. 比色生物传感器有诸多优势, 比如裸眼可见、操作成本低、设计制备成本低、实时检测、不需要配备昂贵的精密分析仪器等. 另一方面, 由于人眼中视锥细胞的数量不同, 人眼对不同波长的可见光敏感度不同, 进而对不同颜色的分辨能力不同. 一般而言, 人眼对绿光(580 nm)的敏感度最强. 故在比色生物传感器中实现工作波长的调谐显得尤为重要, 通过调节工作波长可以使比色生物传感器尽可能工作在人眼的颜色敏感区. 可调谐光学设备通常集成相变材料实现调谐功能, 为使相变材料相变, 需额外附加结构进行加热等操作[37,38]. 本文在可见光波段设计基于α-MoO3的FP谐振腔比色生物传感器, 颜色可随着NaCl溶液折射率的变化而变化. 通过改变器件的方向 (或入射光的偏振角)即可实现调谐, 从而更好地匹配人眼对光的敏感区间. 另一方面, 改变FP谐振腔比色生物传感器中微流腔的层厚可以拓展该传感器的工作区间至整个可见光波段. 我们设计的比色传感器能实现最高600 nm/RIU的检测灵敏度, 品质因数达到23.1, 最高可以分辨NaCl溶液约9‰的浓度变化. 我们设计的生物传感器结构简单易于集成, 在可见光波段实现了较高的可调检测灵敏度, 通过人类视觉系统的比色能力实现方便快捷的生物检测功能, 为以后设计可调谐比色传感器提供了一种新的思路.
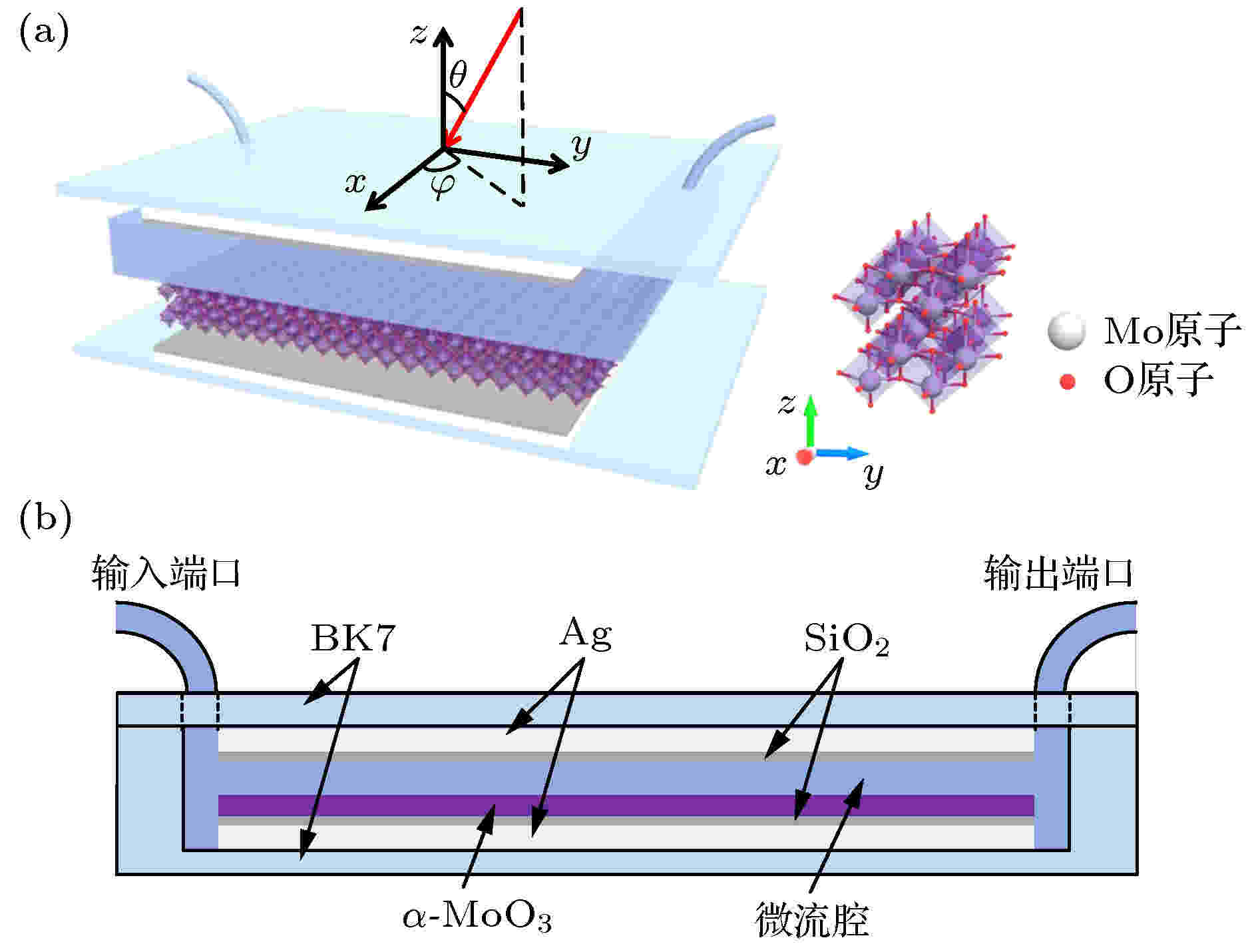
Figure1. Schematic of FP cavity biosensor based on α-MoO3: (a) 3D view; (b) cross-sectional view. The inset is the illustration of orthorhombic α-MoO3 with layered structure held by van der Waals’ forces.
传输矩阵法(transfer-matrix method, TMM)是电磁学和光学中常用的数学方法, 可以计算任意平面波(不同传播方向、入射角、极性)通过多层平板结构的透射谱、反射谱等光谱特征[44,45]. 利用TMM可以在确定初始界面的输入电场的条件下通过一系列矩阵操作得出输出电场. 多层结构可以看作是一个系统矩阵, 表示为单层矩阵的乘积. 最后通过转换系统矩阵即可得出输出光谱的透射系数和反射系数. 本文设计的FP谐振腔的反射镜面由BK7/Ag/SiO2构成. 首先利用TMM计算了三种结构(单层BK7, BK7/Ag和BK7/Ag/SiO2)的透射光谱, 如图2所示. 由于BK7基底在可见光波段具有较大的带隙且几乎没有吸收, 入射光通过单层BK7基底时的透射率可达0.95左右. 当Ag层薄膜(30 nm)覆盖在BK7基底上时, 透射率显著下降. 这是因为入射光的光子会与金属的原子发生交互作用产生一系列反射、透射等, Ag层薄膜也会对可见光波段的光子有一定的吸收作用. 由于Ag层薄膜在空气中易氧化, 故在Ag层薄膜上再镀膜一层SiO2薄膜(3 nm)防氧化. SiO2在可见光波段一般可以认为是透明材料且SiO2薄膜极薄, SiO2/Ag薄膜的透射率只相对下降少许. 入射光在到达Ag层薄膜表面时会诱发Ag中部分电子振荡, 由于光子和自由电子的相互作用产生了金属原子通过弱键合作用构造出的负电子云. 这些电子随着入射光波长改变而振荡从而增强了反射. 一些具有不同能量的光子不会与松散键合的电子发生相互作用, 从而成为了透射光和能量耗散(吸收). 透射和反射光谱取决于整体有效折射率, 而SiO2由于极薄故在有效折射率中贡献很小. 在BK7基底和SiO2中, 电子被牢牢地键合, 只能在其正常位置附近振荡. 这一运动影响了入射光的传播, 降低了入射光的波速, 还会引发小的能量耗散. 整体而言, 整体有效折射率的改变是由光子与Ag层薄膜自由电子、SiO2的束缚电子、液态生物样本的相互作用决定的, 这一相互作用与入射光的波长高度相关, 进一步影响折射率. 在基于α-MoO3的FP腔生物传感器中, 选取窄波长范围进行传感监测, 从而使得入射光波长对折射率传感测量的影响较小, 可以忽略.
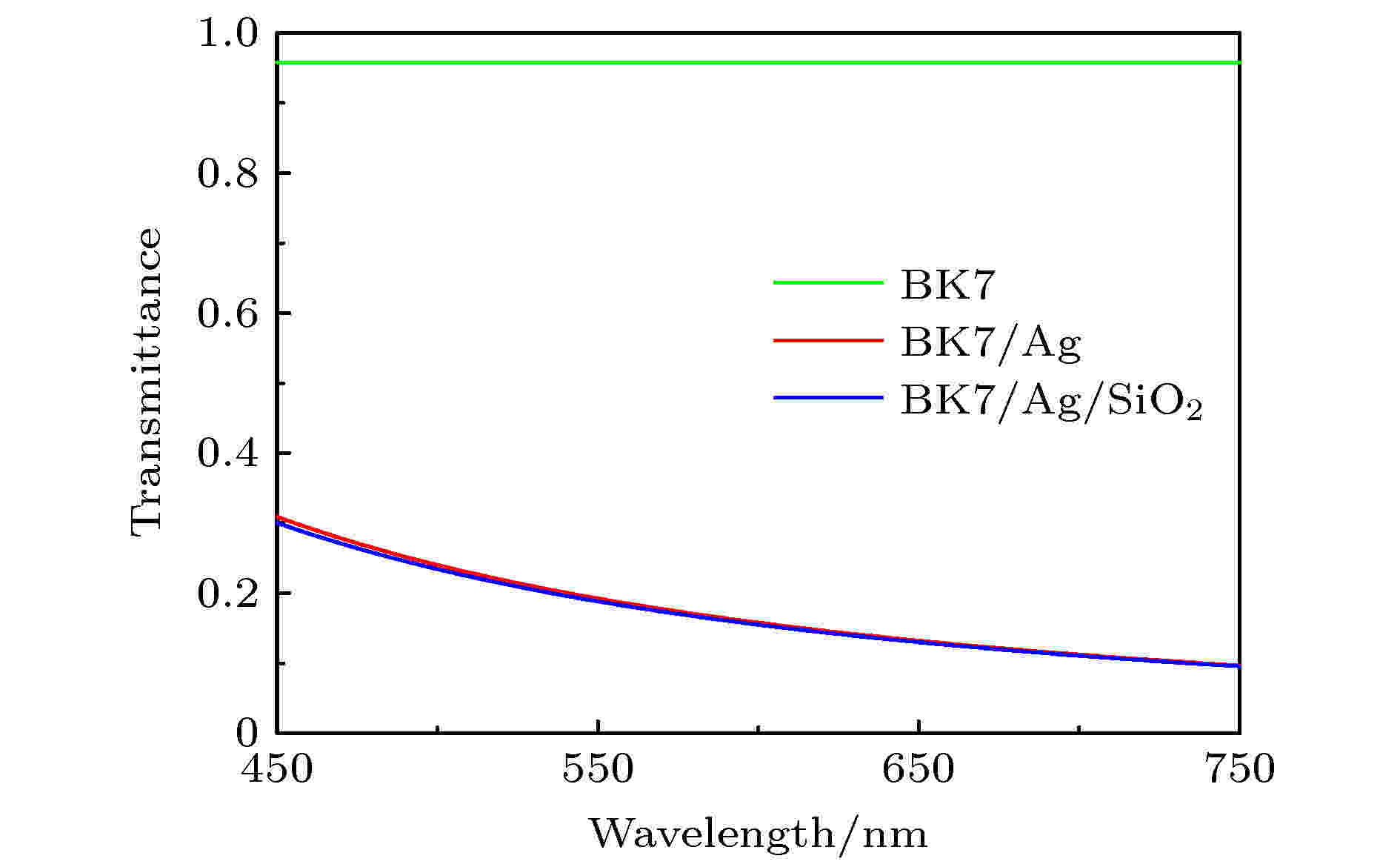
Figure2. Transmittance spectrum of three different structure, including single layer BK7, BK7/Ag and BK7/Ag/SiO2.
运用TMM计算不同浓度NaCl溶液通过基于α-MoO3的FP谐振腔生物传感器的透射率. TMM中特征矩阵考虑到了FP谐振腔各层厚度、折射率在不同入射光波长下的影响. NaCl溶液不同浓度下的折射率来源于前人的工作[46]. 选取入射角θ = 75°. 由于消光系数和色散效应在可见光波段对折射率的影响较小, 可以忽略, 故在计算中设消光系数为0, 忽略色散效应. SiO2的厚度只有3 nm, 故SiO2在可见光波段的吸收效应也可以忽略. 在FP谐振腔比色传感器设计中, Ag层太厚会阻止入射光穿过FP谐振腔使透射率太低, Ag层太薄则难以形成良好的谐振, 即透射谱共振峰品质因数低. 本文设计的基于α-MoO3的FP谐振腔比色传感器中Ag层薄膜的厚度选为30 nm, 兼顾了透射率和品质因数. SiO2层薄膜的厚度为3 nm, 可以保证Ag层不被氧化同时不会过多影响FP谐振腔. α-MoO3层太厚时生物样本溶液折射率改变对整体FP谐振腔内有效折射率的影响会降低, 即生物传感器的灵敏度会降低, 若α-MoO3层太薄, 则生物传感器的调谐性能下降. 本文选取α-MoO3层的厚度为100 nm, 兼顾了调谐性和灵敏度. 表1总结了仿真计算中所有的各层材料的参数.
材料 | 折射率 | 厚度 | |
基底 | BK7 | 1.515 | Inf. |
1 | Ag | drude模型[47] | 30 nm |
2 | SiO2 | 1.458 | 3 nm |
3 | 纯水 | 1.333 | |
5% NaCl溶液 | 1.3418 | ||
10% NaCl溶液 | 1.3505 | ||
15% NaCl溶液 | 1.3594 | ||
20% NaCl溶液 | 1.3684 | ||
25% NaCl溶液 | 1.3778 | ||
4 | α-MoO3 | drude模型[48] | 100 nm |
5 | SiO2 | 1.458 | 3 nm |
6 | Ag | drude模型[47] | 30 nm |
基底 | BK7 | 1.515 | Inf. |
表1TMM计算FP谐振腔透射光谱所用的参数
Table1.Parameter for simulation of transmittance spectrum of proposed FP cavity biosensor using TMM.
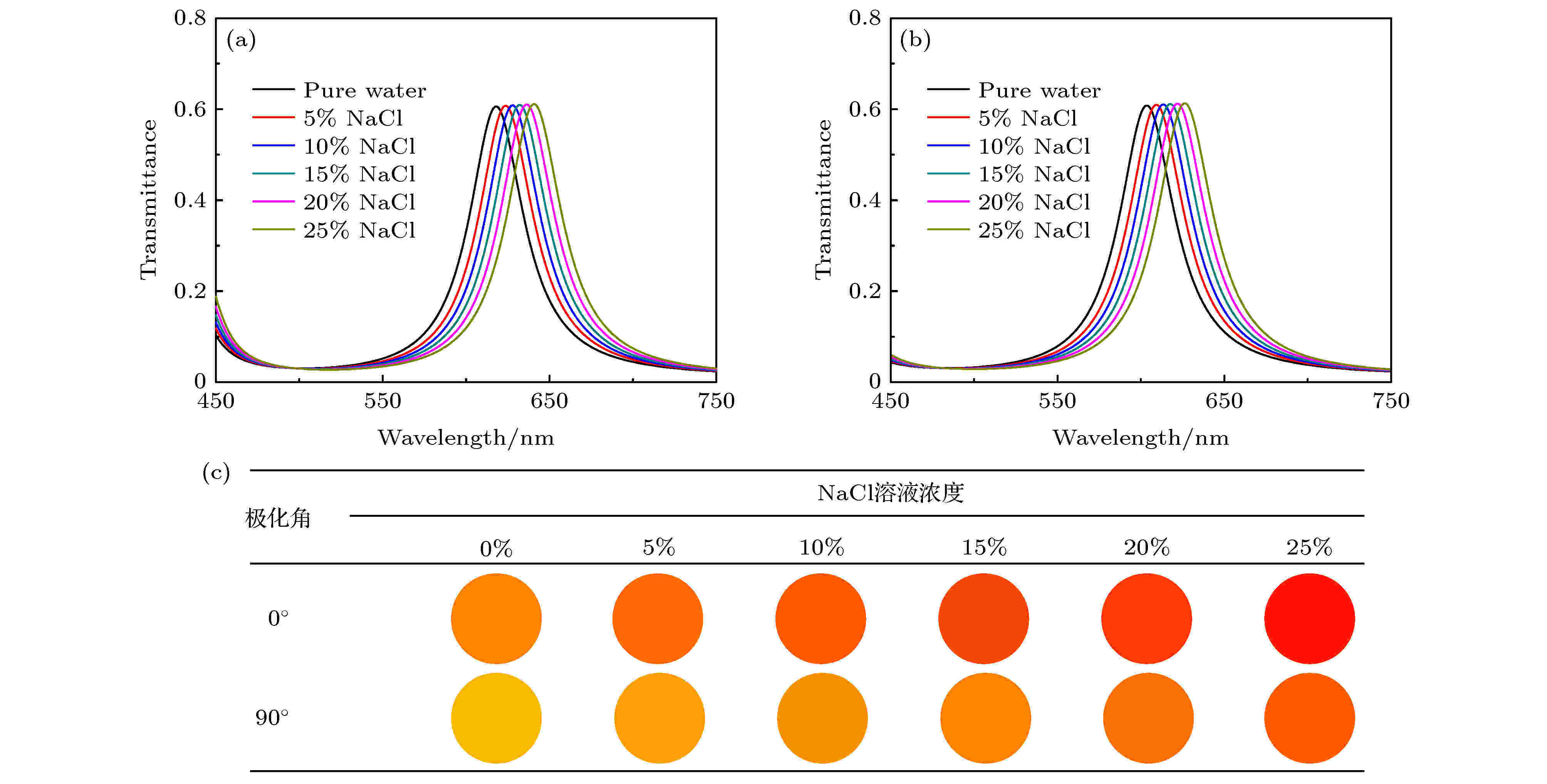
Figure3. Transmittance spectrum of the FP cavity biosensor on (a) x polarization (φ = 0°) and (b) y polarization (φ = 90°) while the micofluidic chamber (300 nm) was filled with NaCl solution in different concentration; (c) colormap for NaCl solution in different concentration filled in micofluidic chamber (300 nm) at x polarization (φ = 0°) and y polarization (φ = 90°).
对于生物传感器, 灵敏度(sensitivity, S)是一个重要的指标, 通常定义为可测量物理量变化(透射峰波长, nm)/折射率变化(refractive index unit, RIU):
如图4所示, x偏振光下和y偏振光下透射峰波长随折射率(NaCl溶液浓度)近似线性变化. 图中线性拟合斜率即为灵敏度, 在x偏振光下和y偏振光下的灵敏度约为507 nm/RIU. 由Ag层反射镜构成的FP谐振腔实现了良好的共振效果, 在x偏振光下的FoM为15.8, 在y偏振光下的FoM为15.3. 最小可觉差(just-noticeable difference, JND)代表人或其他动物某一特定器官受到刺激时所能察觉到的最小改变. 颜色的改变本质是光的波长发生变化, 在可见光范围内从长波长到短波长可简单分为红、橙、黄、绿、青、蓝和紫. 在合适的观测条件下, 裸眼的分辨能力最强为0.4 nm (光波长580 nm处), 在光波长490—620 nm范围内分辨能力约为1 nm, 随着光波长红移至650 nm处, 裸眼的分辨能力降低至3.6 nm左右[49]. JND存在个体差异, 也会因环境、心理生理状态等发生改变. 考虑到人眼对颜色的分辨能力及该FP生物传感器的工作范围, 运用该FP生物传感器使用者可分辨约39‰的NaCl溶液的浓度变化. 由方程(2)可知, 透射峰波长与FP腔内有效折射率的一次成反比, 故当入射光的极性改变时, α-MoO3的折射率随之改变, 从而改变了FP谐振腔内有效折射率, 实现了不同入射极性下透射峰波长的位移. 而灵敏度S则与FP谐振腔内有效折射率的二次方成反比. 由方程(2)可推出不同NaCl溶液浓度引发的共振峰频率变化

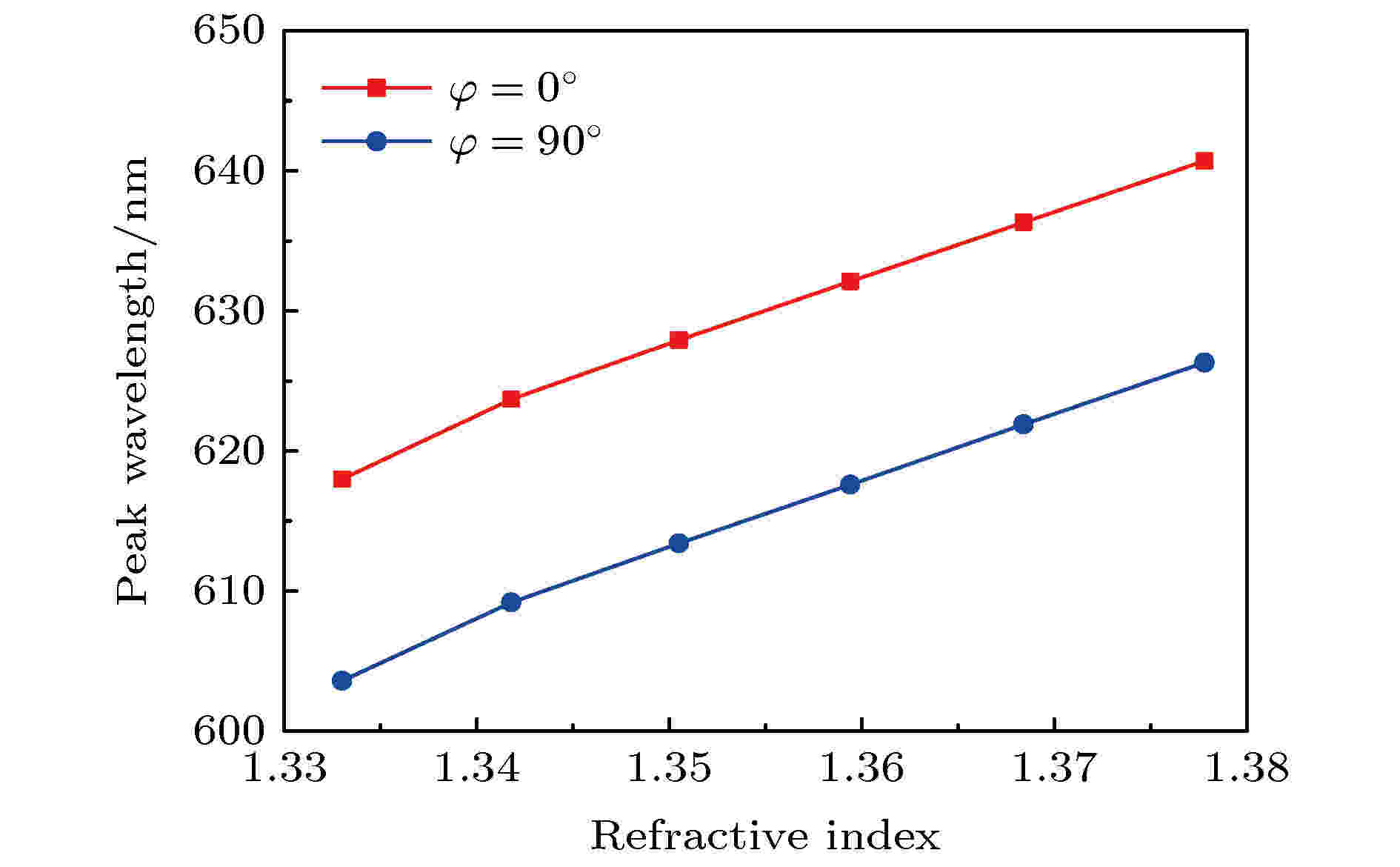
Figure4. The peak wavelength of transmittance spertrum of FP cavity biosensor based on α-MoO3 on x polarization and y polarization as a function of refractive index, while the thickness of microfluic chamber is 300 nm.
为使基于α-MoO3的FP谐振腔生物传感器的工作范围转移, 提高FP谐振腔中微流腔的厚度至550 nm. 图5(a)和图5(b)分别为x偏振光和y偏振光时不同NaCl溶液浓度通过微流腔时FP谐振腔生物传感器的透射光谱图, 该FP谐振腔生物传感器的主要工作波长范围为550—600 nm. 由于FP谐振腔内厚度增加, 透射率略微降低, 当两束相邻的光束相位延迟为π的整数倍时通过FP谐振腔生物传感器的透射率达到最大值0.58左右. 从图5中可以看出, 生物样本空间厚度改变后的FP谐振腔生物传感器也可以完成生物传感的功能且工作波段蓝移了约50 nm. 从图5(c)中可以看出, 同样检测0%浓度(纯水)到25%浓度的NaCl溶液, x偏振光下透射率的峰值波长从樱草色(λ = 560.6 nm)红移至鹅黄色(λ = 587.3 nm), y偏振光下透射率的峰值波长从柳绿色(λ = 550.9 nm)红移至近纯黄色(λ = 577.9 nm). 该生物传感器也可以实现调谐功能, 在y偏振光下透射谱相对x偏振光下发生蓝移, y偏振光下的透射峰值波长蓝移了10 nm左右. 此外, 基于α-MoO3的FP谐振腔生物传感器在生物样本空间的厚度为550 nm时的透射谱的强度和品质因数也能保持稳定, 在x偏振光下的FoM为23.1, 在y偏振光下的FoM为22.2.
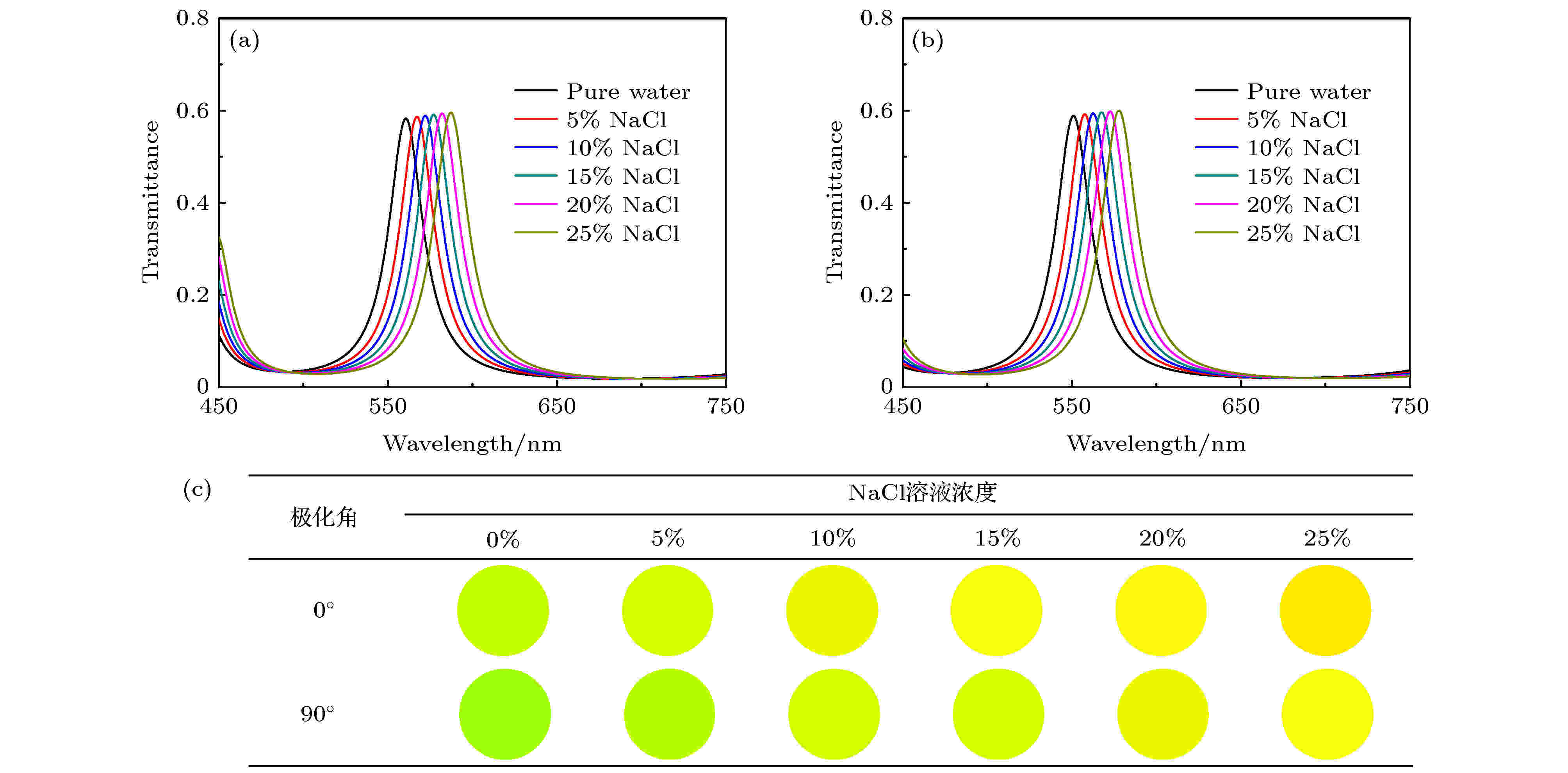
Figure5. Transmittance spectrum of the FP cavity biosensor on (a) x polarization (φ = 0°) and (b) y polarization (φ = 90°) while the micofluidic chamber (550 nm) was filled with NaCl solution in different concentration; (c) colormap for NaCl solution in different concentration filled in micofluidic chamber (550 nm) at x polarization (φ = 0°) and y polarization (φ = 90°).
如图6所示, x偏振光下和y偏振光下透射峰波长随折射率(NaCl溶液浓度)近似线性变化. 图中线性拟合的斜率即为灵敏度, 在x偏振光下和y偏振光下的灵敏度约为600 nm/RIU, 运用该FP生物传感器, 使用者可分辨约9‰的NaCl溶液的浓度变化. 由于生物样本空间的厚度提高, FP谐振腔中待测生物样本对有效折射率的影响增大, 根据方程(2)可知FP谐振腔生物传感器对待测生物样本的灵敏度会随之提高. 另一方面, FP谐振腔中α-MoO3的相对占比下降, 从而调谐能力下降. 在设计中, 应该有效控制各层厚度, 从而实现灵敏度和调谐能力的平衡.
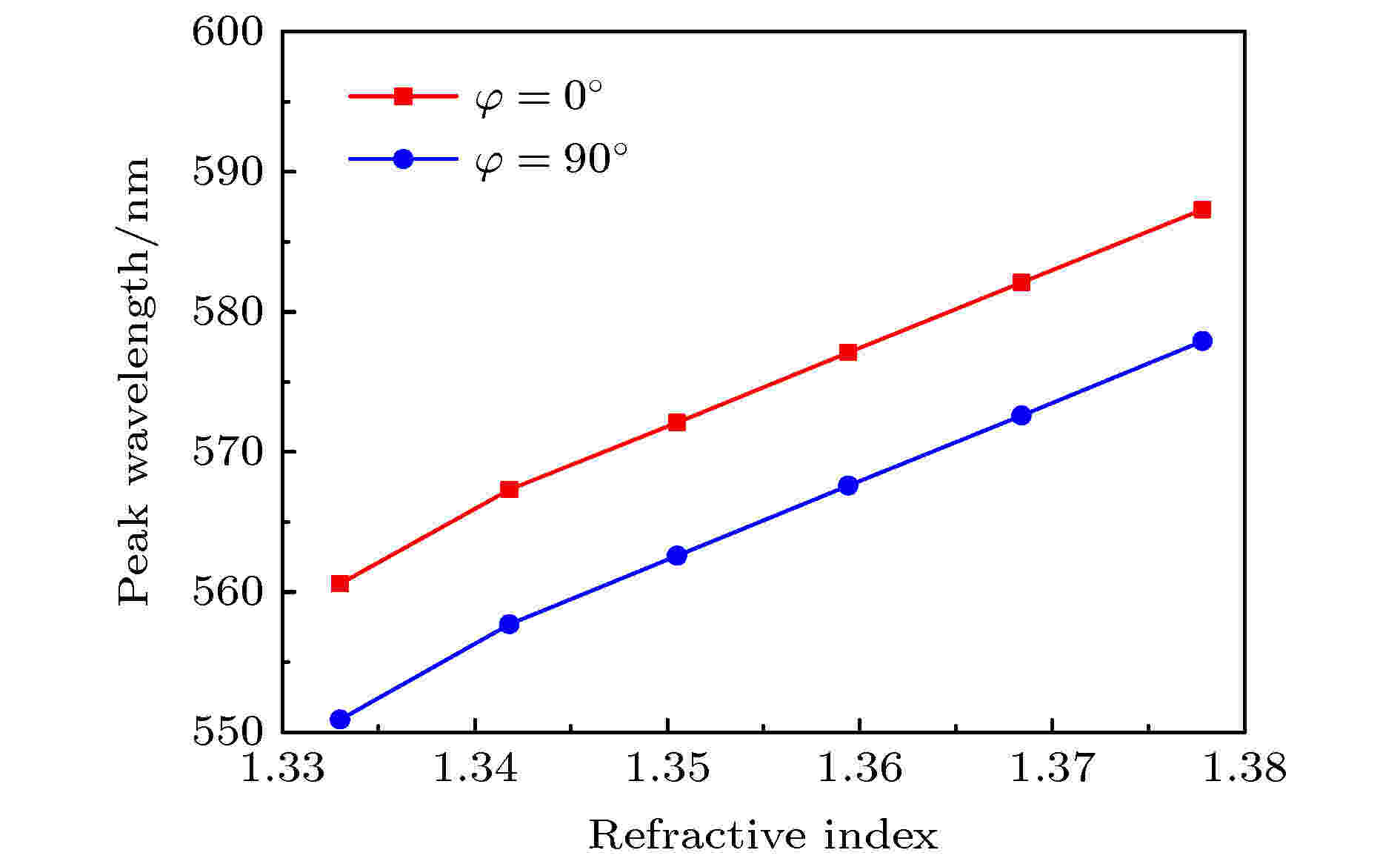
Figure6. The peak wavelength of transmittance spertrum of FP cavity biosensor based on α-MoO3 on x polarization and y polarization as a function of refractive index, while the thickness of microfluic chamber is 550 nm.