全文HTML
--> --> -->STED技术通过纯光学方法实现对荧光团亮态和暗态的调控[16], 利用受激辐射效应来压缩发光点的点扩展函数(point spread function, PSF), 从而绕过光学衍射极限限制. 通常情况下, 激发光斑为高斯型, 如果在激发光斑上套叠一个用于产生受激辐射的环形损耗光, 在重叠区域处于激发态的荧光分子将以受激辐射的形式迅速回到基态, 只剩下环形光斑中心区域的分子以自发辐射的形式发射荧光, 从而达到压缩点扩展函数的目的. 为了能有效迫使荧光团进入暗态, 激发光要先于损耗光(STED光)约200 ps到达样品, 且受激辐射过程必须先于自发辐射. 自发辐射通常在样品被激发后几个纳秒内(荧光团的激发态寿命)发生, 因此要在这个时间段内完成受激辐射过程. 由于时间范围很短, 以及荧光分子的受激辐射横截面很小, 要想完全耗尽重叠区域的荧光, 需要非常高的STED光强度. 另外, STED系统的空间分辨率与STED光的强度成正比, STED光强度越大, 系统的空间分辨率也就越高. 因此要达到高的空间分辨率, 一般情况下会导致样品的光漂白[17]和光毒性[18], 这对活细胞成像非常不利, 阻碍了STED技术的应用和发展.
针对这些问题, STED技术的发明人Hell教授[18]于2013年提出了利用时间门控探测技术, 在较低的能量下实现了分辨率的提升. 我们课题组于2018年提出利用相图分析技术分离出具有长荧光寿命的光子, 来提高系统的空间分辨率, 或者在保持空间分辨率不变的情况下降低STED光的功率[8]. 2017年北京大学Liu等[19]和华南师范大学Zhan等[20]同时提出利用上转换纳米粒子实现STED超分辨成像, 大大提高了抗光漂白特性. 同时也有许多其他量子点被用于STED超分辨成像[21,22], 在保持高空间分辨率的同时具有很好的光稳定性, 并能承受高的激光功率和长的曝光时间. 自适应光学像差校正技术也是降低STED光强度和提高图像质量的有效方法之一[4-5,23]. 其他克服这些问题的方法还包括2013年浙江大学Kuang等[24]提出的一种类似于STED的超分辨成像方法, 即荧光发射差分(fluorescence emission difference, FED)显微, 可实现低光功率超分辨成像. 虽然人们在STED光学成像系统、方法和探针上做了很多改进, 也取得了很大进展. 但是系统和方法的改进毕竟有限, 量子点探针的靶向性和毒性依然是需要解决的难题. 虽然有商用的ATTO系列STED有机探针可以使用, 但这些探针只能用于制备固定样品, 染色过程很繁琐, 很难用于活细胞成像[25,26]. 因此, 非常有必要发展新型的具有较高的抗光漂白特性、低的光毒性、较好的生物靶向性和低的饱和擦除光功率, 可用于活细胞长时间成像的STED探针.
线粒体通常被称为细胞的“动力工厂”, 是真核细胞最重要的细胞器之一. 线粒体最重要的作用是产生细胞中腺嘌呤核苷三磷酸(adenosine triphosphate, ATP), 为生物体提供能量, 是细胞进行有氧呼吸的主要场所. 线粒体具有典型的结构特征[27], 一般呈短棒状或圆球状, 在某些生物种类和生理状态下, 还可呈环状、线状、哑铃状、分杈状、扁盘状或其他形状. 成型蛋白介导线粒体以不同方式与周围的细胞骨架接触或在线粒体的两层膜间形成不同的连接, 可能是线粒体在不同细胞中呈现出不同形态的原因. 除了合成ATP为细胞提供能量等主要功能外, 线粒体还承担了许多其他生理功能[28], 比如调节膜电位并控制细胞程序性死亡. 线粒体膜通透性的增加会诱导凋亡因子等分子释放进入细胞质基质, 破坏细胞结构. 线粒体在生物体中起着如此重要的作用, 生物学家们采用了多种方法来研究线粒体的精细结构和复杂的生物功能.
线粒体是对各种损伤最为敏感的细胞器之一. 在细胞损伤时最常见的病理改变可概括为线粒体数量、大小和结构的改变, 可以通过观察线粒体的外形变化来判断细胞的健康状态. 多年来, 荧光显微镜是研究细胞动力学的有力工具, 已被用于对单个线粒体中特定蛋白的分析[29]. 但是线粒体的内膜尺寸小于光学衍射极限, 传统的荧光显微镜无法观察线粒体内膜的微结构以及一些相关的动态变化过程. 超分辨成像技术的出现, 为线粒体精细结构和功能的研究提供了非常好的工具[30].
本文找到了一种新型的可用于线粒体STED成像的有机探针. 该探针对线粒体的靶向性好, 具有较好的抗光漂白特性和较低的擦除光饱和强度, 可用于活细胞长时间STED超分辨成像. 在实验中, 对线粒体成像的最高分辨率可达62 nm. 该探针与STED超分辨成像结合, 为活细胞线粒体精细结构和功能研究提供了新的手段.
2.1.STED显微镜的原理
1873年德国物理学家Ernst Abbe提出衍射极限的概念. 他指出, 由于光的衍射效应, 光学显微镜的远场分辨率不可能高于半波长, 衍射极限公式为
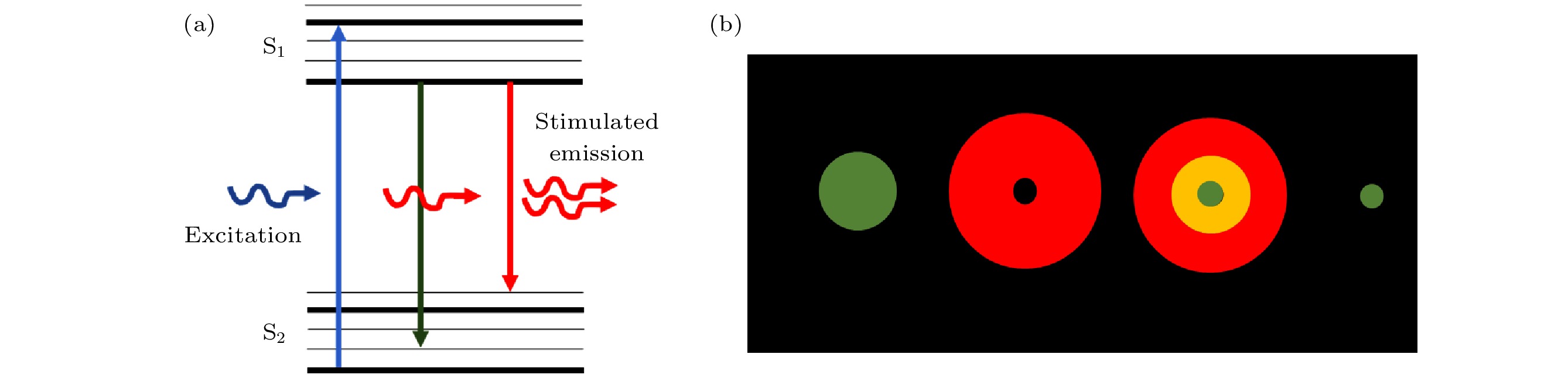
Figure1. Schematic diagram of STED: (a) Diagram of energy level for stimulated emission depletion; (b) schematic diagram of STED light spot.
而且Hell[31]还通过总结大量的实验结果, 推导出了STED显微镜的横向空间分辨率公式:




















2
2.2.探针的机理分析
探针的化学结构和光谱表征结果如图2所示. 从图2可见, 探针(命名为探针1)的激发峰值在578 nm附近, 但是当探针1和人血清蛋白(human serum albumin, HSA) 1∶1混合后(命名探针2)吸收峰会蓝移到561 nm附近(图2(b)所示). 图2(c)为两种探针的发射谱图, 可以看到两个探针的发射峰值都在683 nm附近, 探针2的发射谱完全覆盖探针1的发射谱, 且其信号强度约为探针1的10倍. 由于两个探针的发射峰值都在683 nm附近, 而探针2的吸收峰发生了蓝移, 因此探针的斯托克斯红移由原来的105 nm扩大到122 nm(如图2(d)所示). 通过加入HSA使激发蓝移, 增大了激发和发射峰之间的红移, 斯托克斯红移较大的染料可以避免STED光束对样品的二次激发, 在STED成像中具有很大的优势.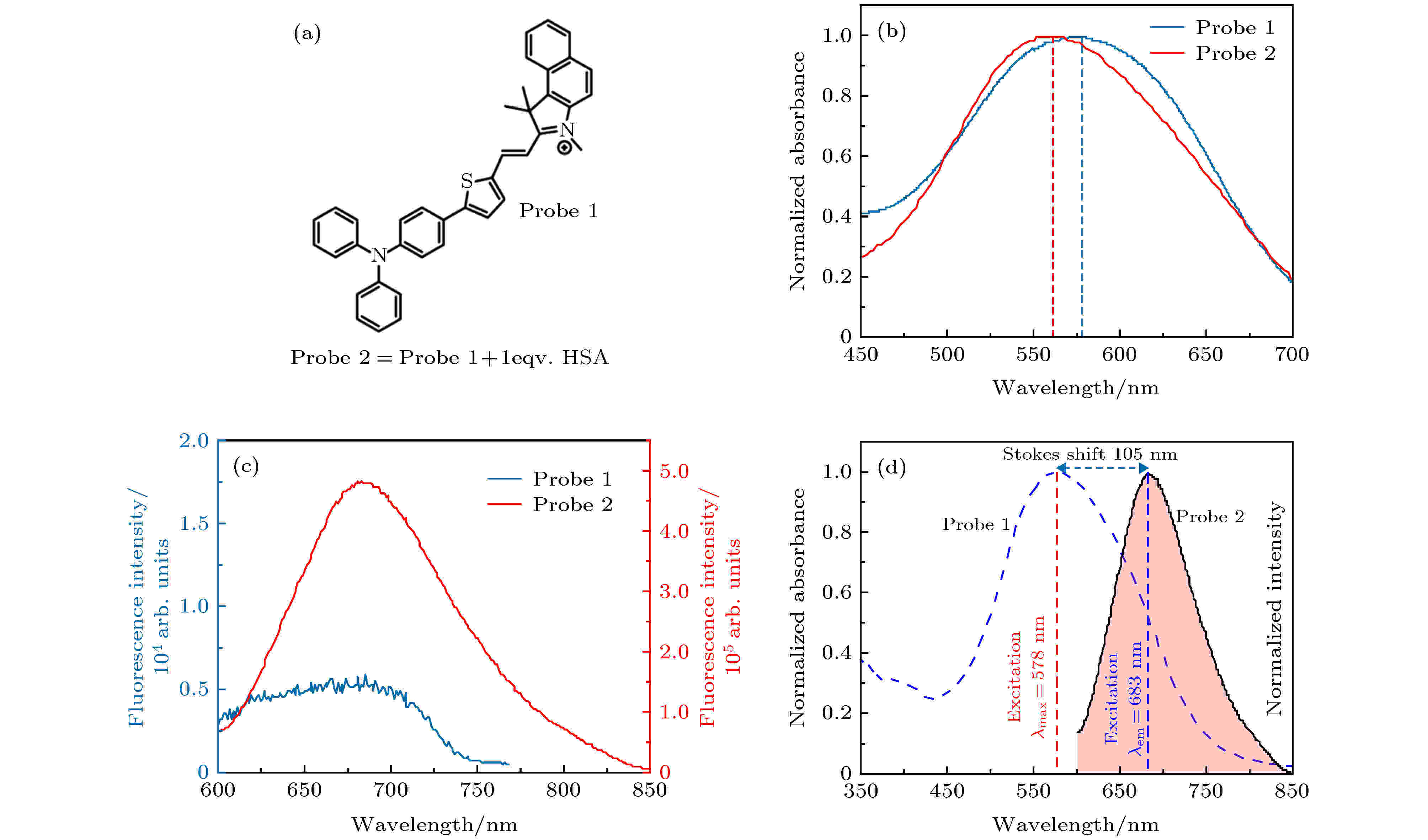
Figure2. Chemical structure and spectra characterization of target probe: (a) Chemical structure of probe 1; (b) excitation spectra of probe1 (blue)and 2 (red); (c) emission spectra of probe1 (blue) and 2 (red); (d) excitation spectrum of probe 1 (blue) and emission spectrum of probe 2 (red).
HSA是血液中最丰富的巯基化合物蛋白质, 具有多种重要的生物学功能, 例如促进药物、脂肪酸和代谢物的运输, 同时在维持血液的渗透压中具有重要作用, 因此将HAS与荧光染料结合, 有着重要的研究意义[32-35]. 已有报道表明小分子荧光探针与HSA结合, 可以形成HSA-dye纳米小颗粒[33], 而且发现这些探针有助于提高信噪比, 主要是由于荧光探针与HSA结合在生理条件和活细胞中会表现出对细胞器更高的选择性和灵敏度, 从而表现出荧光信号的增强, 同时具有较高的热稳定性和生物相容性[34-35], 该结论为探针2的高性能表现在原理上提供了有力支撑.
3.1.共聚焦成像
本文制备的新型探针用于标记活细胞, 所使用的细胞均为HeLa细胞. 染色方法为: 将状态较好的HeLa细胞在培养箱(37 ℃, 5%CO2)中接种在30 mm的共聚焦皿中, 探针1与探针2分别与活细胞在培养箱中共孵育15 min, 孵育好后在室温下用缓冲剂PBS冲洗3遍, 继而使用商用共聚焦显微镜(A1 R MP+, Nikon, Japan)记录图像, 结果如图3所示. 相对于探针1的共聚焦图像, 加了HSA的混合液探针2的共聚焦图像的荧光信号更强, 且定位更准确, 背景更加干净.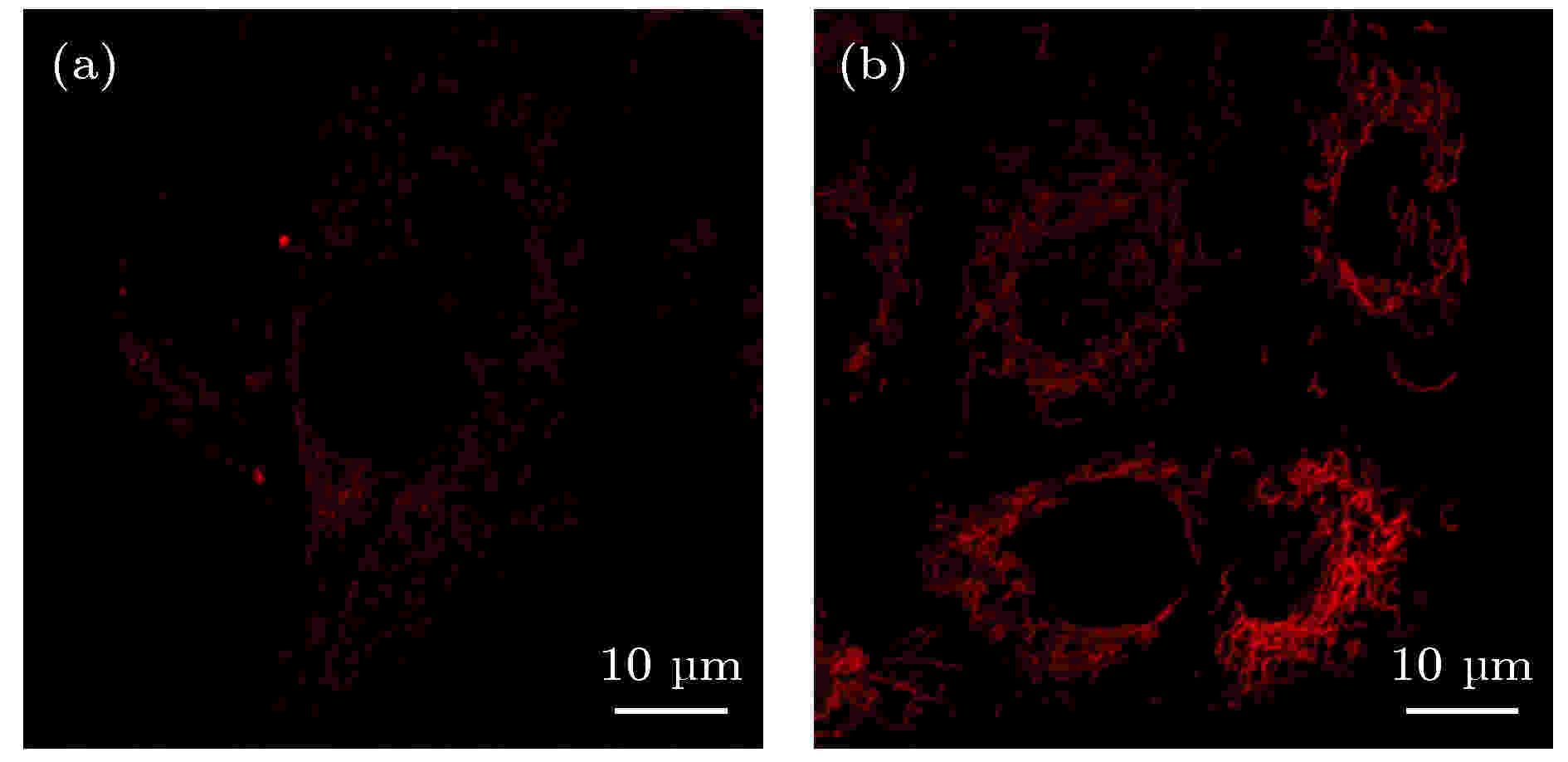
Figure3. Confocal images of HeLa cells labeled with probe 1 and probe 2. Scale bar is 10 μm: (a) Confocal image of HeLa cells labeled with probe 1; (b) confocal image of HeLa cells labeled with probe2.
从图3可以看出, 探针的两种形式都可以对线粒体进行标记, 但是相比较而言, 探针2的定位效果更好, 图像的信噪比更高, 这与图2(c)的光谱结果一致, 因此选用探针2对线粒体进行成像. 在此基础上, 对标记的条件进行了多项优化, 例如孵育时间和探针浓度等. 经过多次反复测试发现, 当孵育时间为15 min时, 已有充足的探针分子进入细胞, 且具有良好的染色效果. 此外多次浓度测试结果表明, 在1 ml培养基中, 使用1 μl染料(染料原始浓度为0.2 mM)荧光图像效果最好, 即探针的最佳浓度为0.2 μM.
为了研究探针2的定位效果, 使用商用的线粒体探针Mito Tracker Green FM(M7514, Thermo Fisher; 激发波长490 nm, 发射波长516 nm)和探针2进行共定位实验. 把探针2和Mito Tracker Green FM同时放置在已接种好HeLa细胞的共聚焦皿内, 置于细胞培养箱孵育15 min后, 在室温条件下用PBS进行洗涤, 可以减少背景干扰. 然后在商用的共聚焦显微镜下进行观察, 分别用488 nm的光激发Mito Tracker Green FM和561 nm的光激发探针2, 结果如图4所示. 从图4(a)可以看到, 虽然商用的探针Mito Tracker Green FM能够有效地标记线粒体, 但是线粒体周围还有较强的背景信号, 图像的信噪比较差. 图4(b)为探针2标记线粒体的图像, 从图像上可以看到探针2不仅能有效地标记上线粒体而且线粒体周围几乎没有背景信号, 这说明图像的信噪比要好于商用染料. 为了进一步研究探针2的定位效果, 将探针2与商用探针进行共定位对比, 图4(c)所示为两种染料共定位重叠后的图(绿色和红色叠加后呈黄色), 黄色区域越多说明探针2的定位效果越接近商用染料, 此处用皮尔逊相关系数





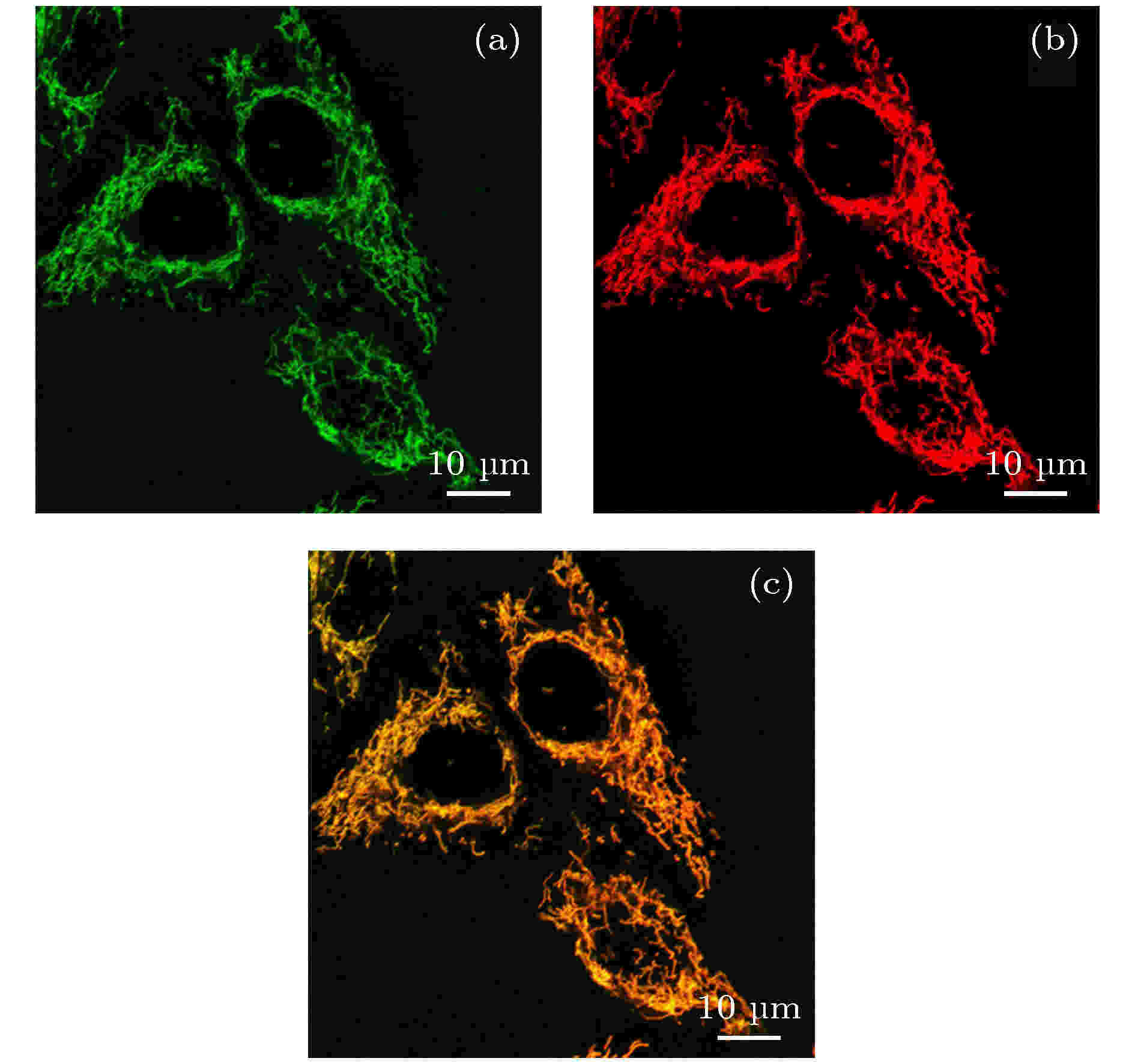
Figure4. Co-localization images of Hela cells treated with Mito Tracker Green FM and Probe 2. Scale bar is 10 μm: (a) Image of Mito Tracker Green FM; (b) image of probe 2; (c) overlay of image (a) and (b).
2
3.2.STED成像
首先需要确定探针2的损耗光(STED光)波长(本文使用的STED系统中, STED光可选择的波长有592, 660和775 nm). 根据STED的原理, STED光的波长应尽量避开激发谱[36], 避免STED光激发荧光. 参考图2的结果, 探针2的激发峰在561 nm处, 发射峰在683 nm附近. 592 nm与激发峰太近, 因此, STED光可选的波长为660和775 nm. 分别用1 μW 561 nm的激发光, 20 mW 660 nm的STED光和20 mW 775 nm的STED光激发样品, 结果如图5所示, 从图5(a)可以看到, 当用561 nm波长的激光激发样品时, 荧光信号较强; 图5(b)是用660 nm的光照射样品得到的结果, 荧光信号也比较强, 用其作为STED光会对样品二次激发, 严重影响超分辨成像的质量, 因此660 nm的光不宜作为探针2的损耗光; 由图5(c)可以看出, 用775 nm的光照射样品, 几乎没有发射荧光, 也就是说, 775 nm的光不会造成二次激发, 可以作为探针2的损耗光.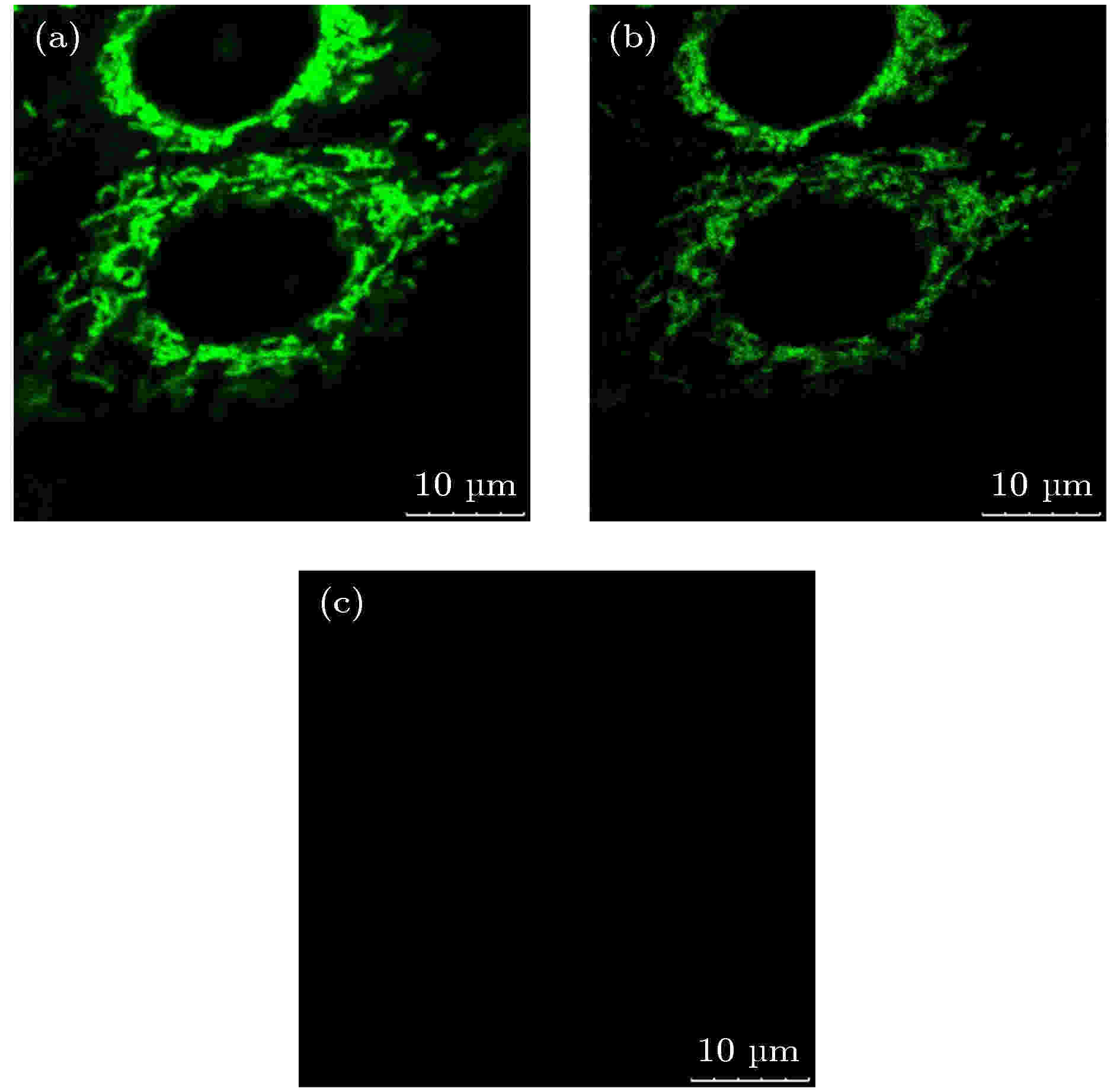
Figure5. Cell images illuminated by light of different wavelengths: (a) Illuminated by light of 561 nm; (b) illuminated by light of 660 nm; (c) illuminated by light of 775 nm.
接下来, 对探针2进行STED成像研究. 本实验所有的STED超分辨实验均在商用的STED显微镜(Leica SP8, Leica, Germany)上完成, 该系统使用了80 MHz且脉冲频率可调的超连续谱激光作为激发光. 根据探针的激发和发射谱(如图2所示), 以及图5的结果, 探针2的激发波长为561 nm, 选用波长为775 nm的脉冲光作为STED光. 实验中选用100 × /NA 1.4的STED专用物镜(HCX PL APO CS2 100× 1.40 oil, Leica Microsystems)进行成像, 探测器的光谱接收范围为650—750 nm.
将HeLa细胞与探针2孵育后进行STED成像, 用功率为1 μW的561 nm激光激发样品, 用不同功率的775 nm的STED光作为损耗光, 成像结果如图6所示. 结果表明, 当损耗光功率为19.75 mW时, 图像的分辨率可以达到90 nm(如图6(b)和图6(e)所示). 与共聚焦图像(图6(a)和图6(d)所示)相比, 原本不能区分的线粒体内膜可以区分开来, 并且可以看到线粒体内部脊的细微结构. 进一步将损耗光功率增加到39.5 mW, 可以更清楚地观察到线粒体内部脊的结构(图6(c)和图6(f)所示), 最高分辨率可达62 nm.
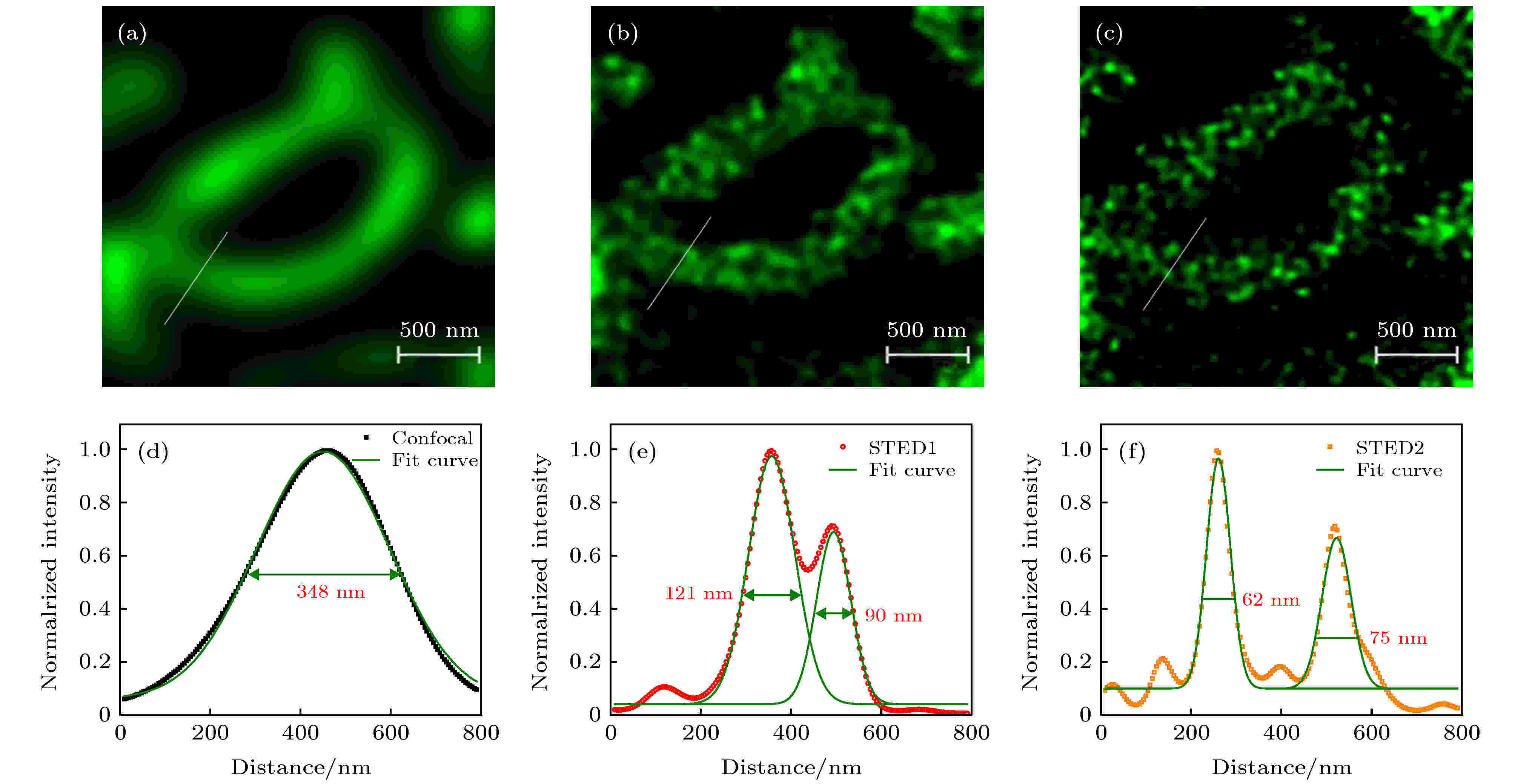
Figure6. Confocal and STED images of HeLa cells labeled with probe 2. Scale bar is 500 nm: (a) Confocal image; (b) STED image of mitochondria obtained with 19.75 mW STED light; (c) STED image of mitochondria obtained with 39.5 mW STED light; (d)?(f) normalized signal intensity profiles along the lines in (a)?(c) respectively as well as the spatial resolutions.
在进一步的实验中, 评估了探针在STED成像中的性能. 测量了探针2在不同功率下的发射强度, 研究了发射损耗效率, 如图7(a)所示, 探针的发射强度随着损耗光强度的增强而迅速降低. 当功率为10 mW时, 探针的受激辐射损耗比接近85%, 而后荧光强度减弱速度明显变得缓慢; 当功率为30 mW时, 达到95%的损耗效率, 高效的发射损耗比对提高探针的STED纳米成像的横向分辨率非常有利, 至此功率的进一步增加并没有进一步提高损耗比. 为了研究探针2在775 nm STED光下的饱和受激辐射功率, 测量了不同功率下775 nm STED光获得的横向分辨率, 结果如图7(b)所示. 根据(2)式可以拟合计算得出, 探针2在775 nm STED光下的饱和功率为

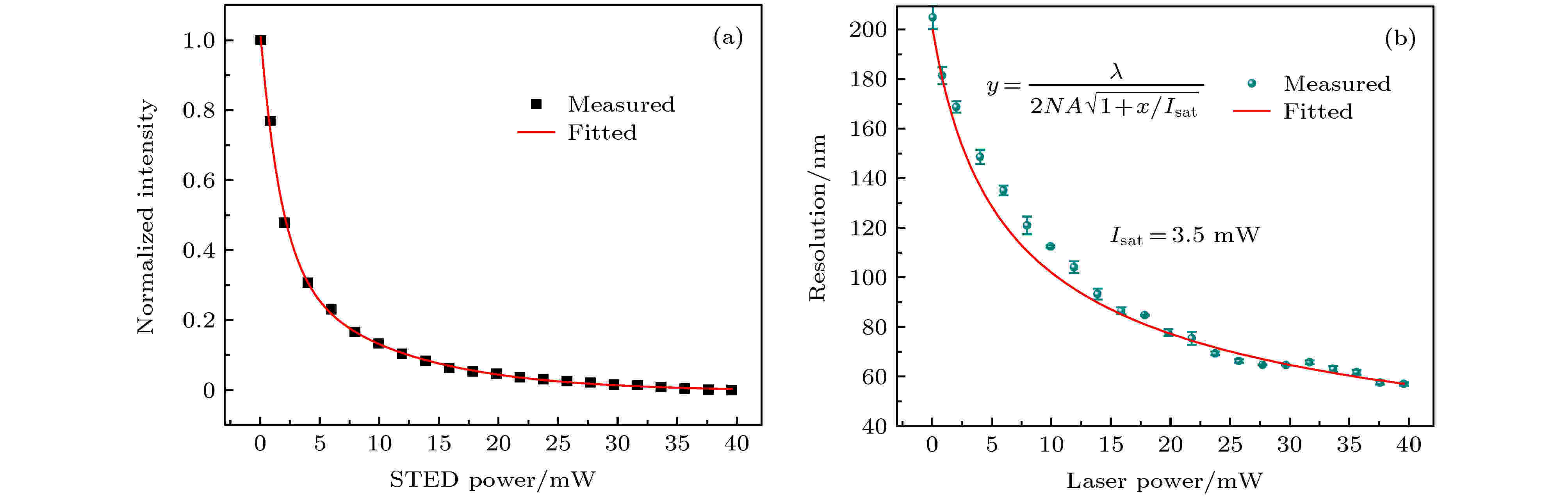
Figure7. Effect of STED power on imaging performance: (a) Stimulated emission depletion efficiency of Probe 2; (b) resolution of STED images obtained at increased depletion power.
2
3.3.抗光漂白测试
STED成像对荧光探针的选择比共聚焦等其他成像技术有更高的要求, 抗光漂白特性是最重要的参考因素之一. 使用功率为19.75 mW的STED光对染色处理好的样品进行多次扫描, 测试探针的抗光漂白特性, 结果如图8所示. 图8中的内插图给出了在第一次扫描后的荧光图像, 此时的荧光信号较强. 经过180次扫描后, 依然可以清楚地看到完整的细胞形态. 经过长达360次扫描后, 虽然细胞结构开始变得模糊, 但仍然可以看到细胞的荧光信号, 其信号强度相对第一次和180次扫描后有明显减弱. 结果表明, 所制备的新型探针具有良好的抗光漂白特性, 适用于长时间STED成像.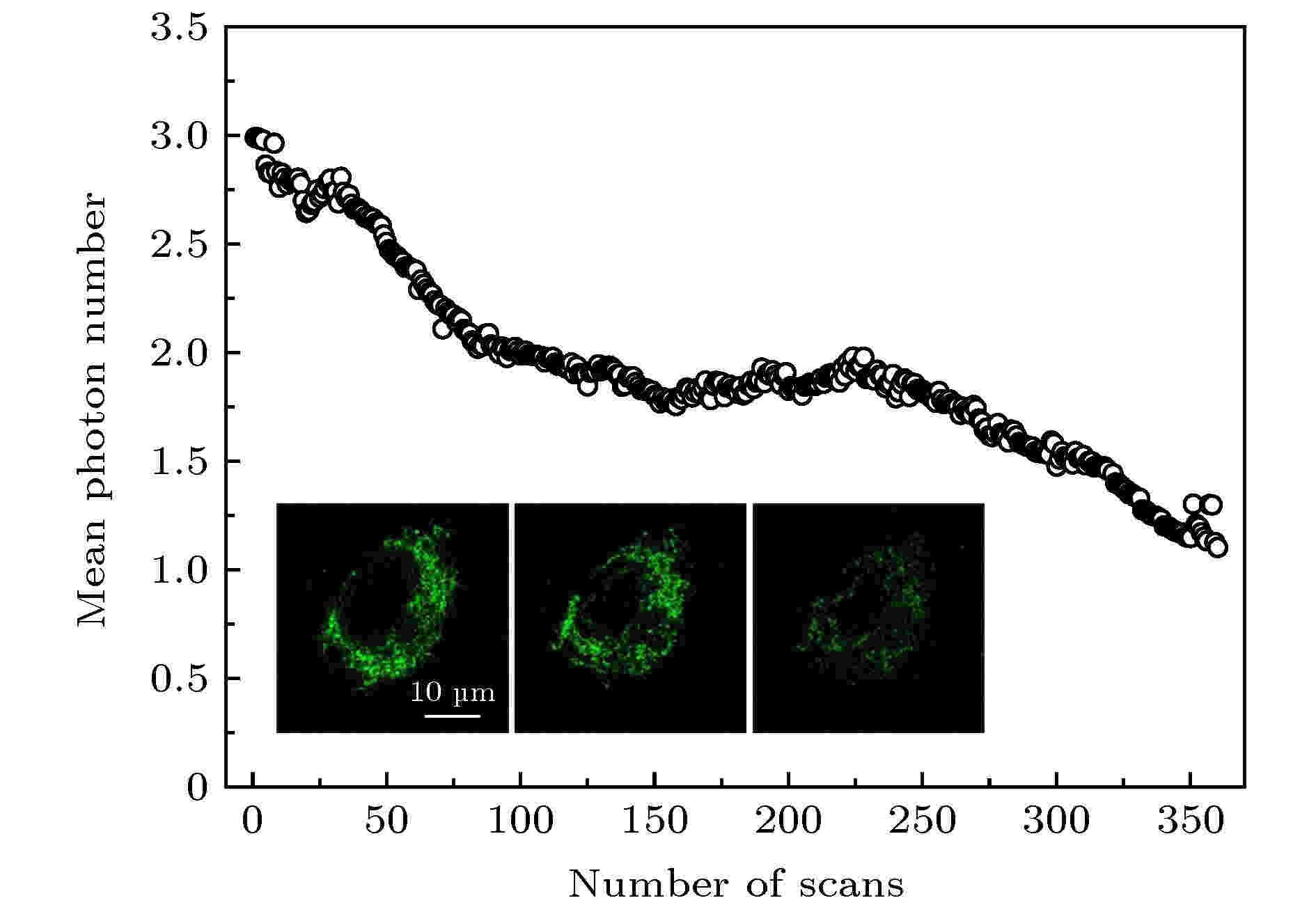
Figure8. Results of bleaching test. Inset pictures are the images of single cell obtained after the first scan, 180 th scan, and 360 th scan. Scale bar is 10 μm.