全文HTML
--> --> -->齿鲸均能发出声信号进行回声定位[1,2,14-21]. 在齿鲸的发声机制研究中, 科学家们曾提出猜想: 喉咙驱动振动产生哨叫声(whistles)、鼻道系统以及相关气道系统之间协同作用产生脉冲声(pulses)[22]. 文献[23, 24]提出鼻道系统系声源所在处, 鼻道以及相应的气囊结构在发声过程起着重要作用. Cranford等[25]利用计算机断层扫描(computed tomography, CT)研究齿鲸生物, 发现齿鲸头部前额有四个脂肪组织体嵌入在鼻道通气孔壁上, 并推测这些脂肪组织体以及相连接的唇状物是发声声源. 这些脂肪组织体被称作背滑囊, 与唇状物形成声唇. 声唇的韧带起支撑作用, 并且可以调整声唇形状[14]. 文献[25-27]基于对齿鲸回声定位的脉冲频率、强度以及脉冲间隔的分析, 提出齿鲸发声机制应当是气流通过某个发声部位产生声波. Cranford 等[25,26]利用CT扫描和核磁共振(magnetic resonance imaging, MRI)技术研究了齿鲸亚目19个种类的40多个样本, 细致描述了发声系统的各个结构. 基于所研究的齿鲸发声系统的结构相似性, Cranford等提出了气动致声模型, 并将该发声机制推广到所有齿鲸生物.
齿鲸声发射过程与前额系统中的气质结构(鼻道气腔、气囊等)、软组织(额隆、结缔组织、肌肉组织等)以及骨质结构(上颌骨、头骨等)的作用是密不可分的. 这些声学结构的形态、尺寸以及物理参数(声速、密度)的变化均能调控声传播与声波波束[28-31]. 此外, 齿鲸还能通过变换声信号的频率、调节额隆和气囊的尺寸、形状等方式调控声波波束[32]. 2015年, Wisniewska等[33]研究发现鼠海豚在探测目标的过程中能改变前额组织的形状来调控波束. 实验测量虽能有效揭示了齿鲸声波波束在头部外围空间的指向性, 但难以深入头部内部探究声传播规律. 数值模型则能直观地显示声波在齿鲸头部内部的传播过程, 并探究各个声学结构在声波传播过程中以及波束的形成中的调控作用. Aroyan等[28]在1992年建立的真海豚(delphinus delphis)模型研究表明上颌骨和鼻道、气囊组成的气质结构对声波的反射作用明显, 使声波能量往前集中, 而额隆软组织的作用相对较弱. Cranford等[29]的模型研究表明海豚的头骨、鼻道和相应的气道系统以及结缔组织等软组织在头部声传播中均发挥一定作用. 本文从生物解剖学、回声定位声信号与数值模型角度出发介绍齿鲸生物的三维声学结构、生物组织的梯度材料特性、生物声呐声信号特性、声发射特性与调控机理研究, 从多方面探讨齿鲸生物声发射过程. 研究齿鲸声发射物理机理与其生物组织多相介质的声学传输效应, 对研究齿鲸声发射物理机理、设计新型声功能材料等具有积极意义.
2.1.生物声呐声发射系统结构组成
齿鲸声发射系统由物理特性各异的声学结构组合形成. 齿鲸生物声呐系统位于头部, 发声系统的各个结构位于前额. 齿鲸生物之间虽大小尺寸各异, 但发射系统结构组成相似[25]. 东亚江豚(narrow-ridged finless porpoise, Neophocaena sunameri)、小抹香鲸(pygmy sperm whale, Kogia breviceps)、以及中华白海豚(Indo-Pacific humpback dolphin, Sousa chinensis)的头部三维重建(图1)表明不同齿鲸生物的头部形态各异. 东亚江豚与小抹香鲸无明显的喙部吻突, 而中华白海豚的喙部吻突明显. 结构的差异性相应地会影响声波波束.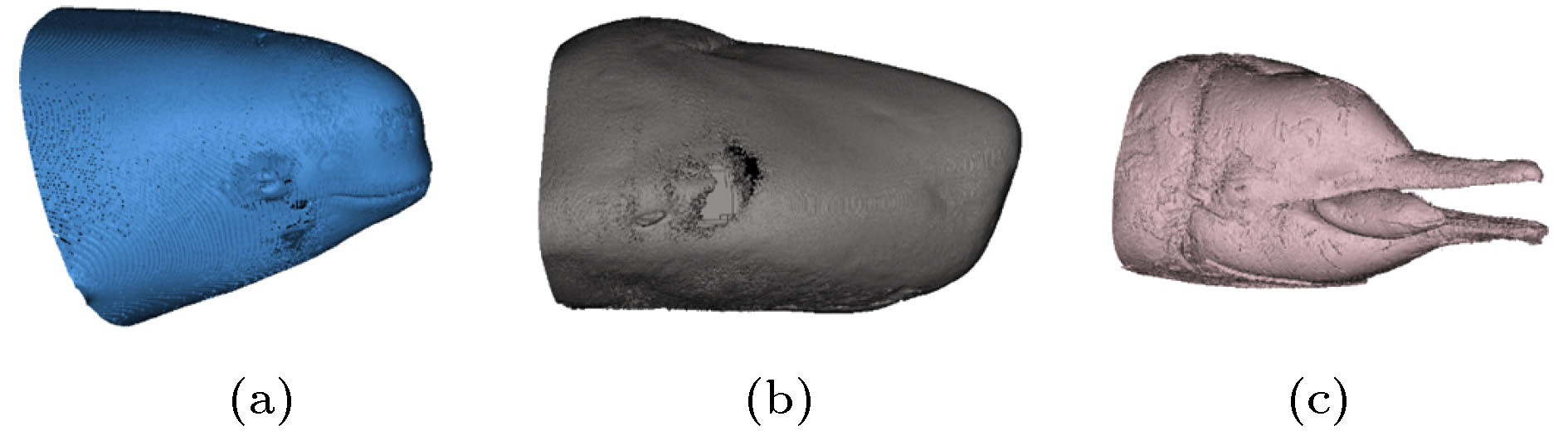
Figure1. (a) Three-dimensional reconstruction of a finless porpoise head; (b) three-dimensional reconstruction of a pygmy sperm whale head; (c) three-dimensional reconstruction of an Indo-Pacific humpback dolphin.
三种齿鲸声发射系统的结构(图2)表明骨质结构的密度高, 能较完整地进行重建, 而软组织与气道系统的重建与样本状态息息相关. 脂肪组织、结缔组织和肌肉组织的声速、密度呈现梯度分布, 形成由内到外的层化结构. 东亚江豚和中华白海豚的声源结构尺寸较小, 以中轴线为参考, 位置大致呈现左右对称, 前、后各有一块由脂肪块组成的复合结构. 小抹香鲸的额隆前侧呈现出方形、钝形. 相对于东亚江豚与中华白海豚, 小抹香鲸的鼻道结构呈现左、右不对称[34-37]. 东亚江豚的左、右声源相对于额隆末端呈对称分布, 声源未与额隆后侧衔接, 而中华白海豚的右侧声源与额隆末端是衔接的. 齿鲸生物虽遵循类似的气动致声原理, 但具体的声源位置、结构与尺寸存在一定的差别.
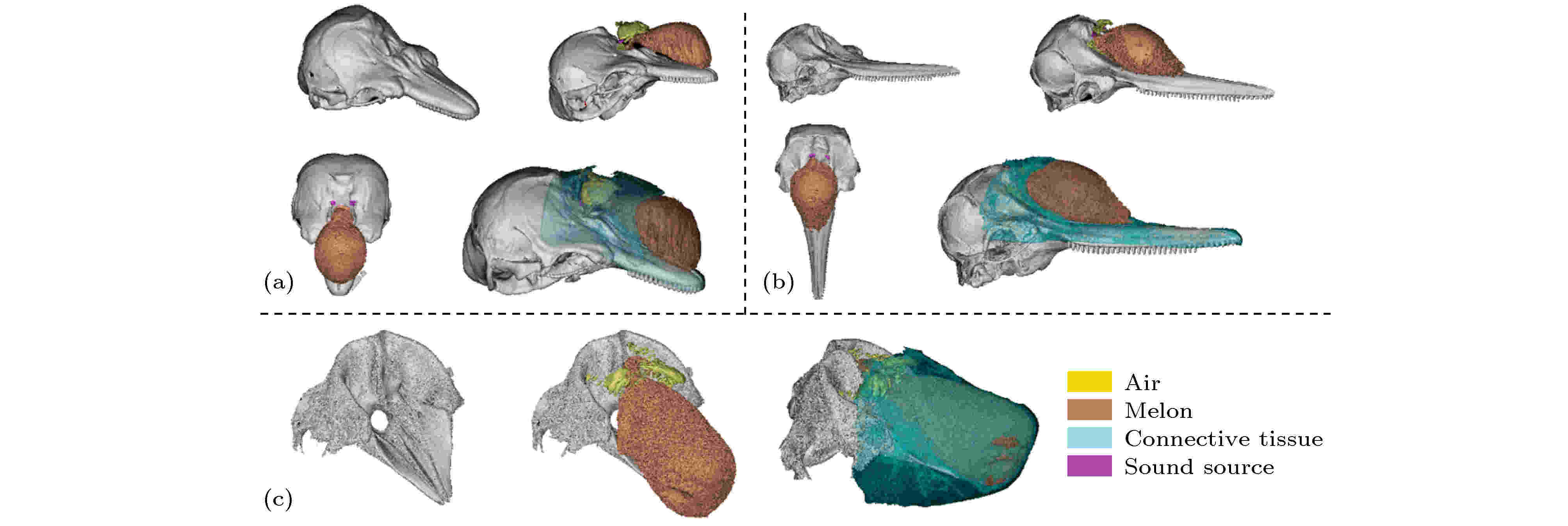
Figure2. (a) Three-dimensional acoustic structure reconstructions of a finless porpoise; (b) three-dimensional acoustic structure reconstructions of a pygmy sperm whale; (c) three-dimensional acoustic structure reconstructions of an Indo-Pacific humpback dolphin. Upper jaw, sound source, air components (including the air sacs and nasal passage) are represented in different colors.
2
2.2.声发射系统的声速分布重建
CT扫描重建的齿鲸头部声发射系统的三维结构能为声场建模提供必要的几何结构信息[37], 但获取其声学特性如声速仍需进行相应测量. 声速和密度是声传播模型所需的基础物理参数. 通过物理测量方法获得各组织的声速和密度, 与CT扫描得到的组织的亨氏单位(Hounsfield unit, HU)值进行回归分析, 可以确定软组织的声速与HU值、密度与HU值之间的函数关系, 从而重建整个声发射系统的声速与密度分布[34,36,38]. 对东亚江豚、小抹香鲸以及中华白海豚组织的回归分析表明, 这些齿鲸头部组织的声速c和HU值均呈现显著的线性关系: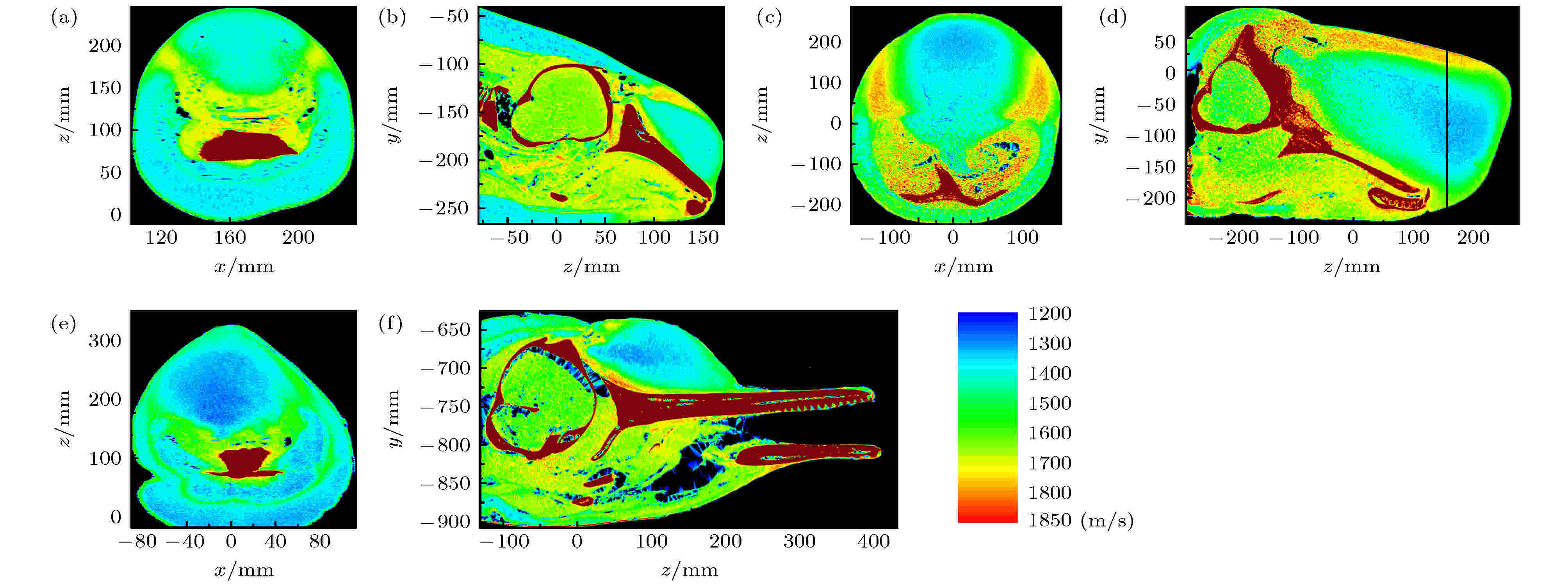
Figure3. (a) Sound speed reconstructions of finless porpoise for sound emission system in horizontal section; (b) sound speed reconstructions of finless porpoise for sound emission system in vertical section[34]; (c) sound speed reconstructions of pygmy sperm whale for sound emission system in horizontal section; (d) sound speed reconstructions of pygmy sperm whale for sound emission system in vertical section[36]; (e) sound speed reconstructions of the Indo-Pacific humpback dolphin for sound emission system in horizontal section; (f) sound speed reconstructions of the Indo-Pacific humpback dolphin for sound emission system in vertical section[38].
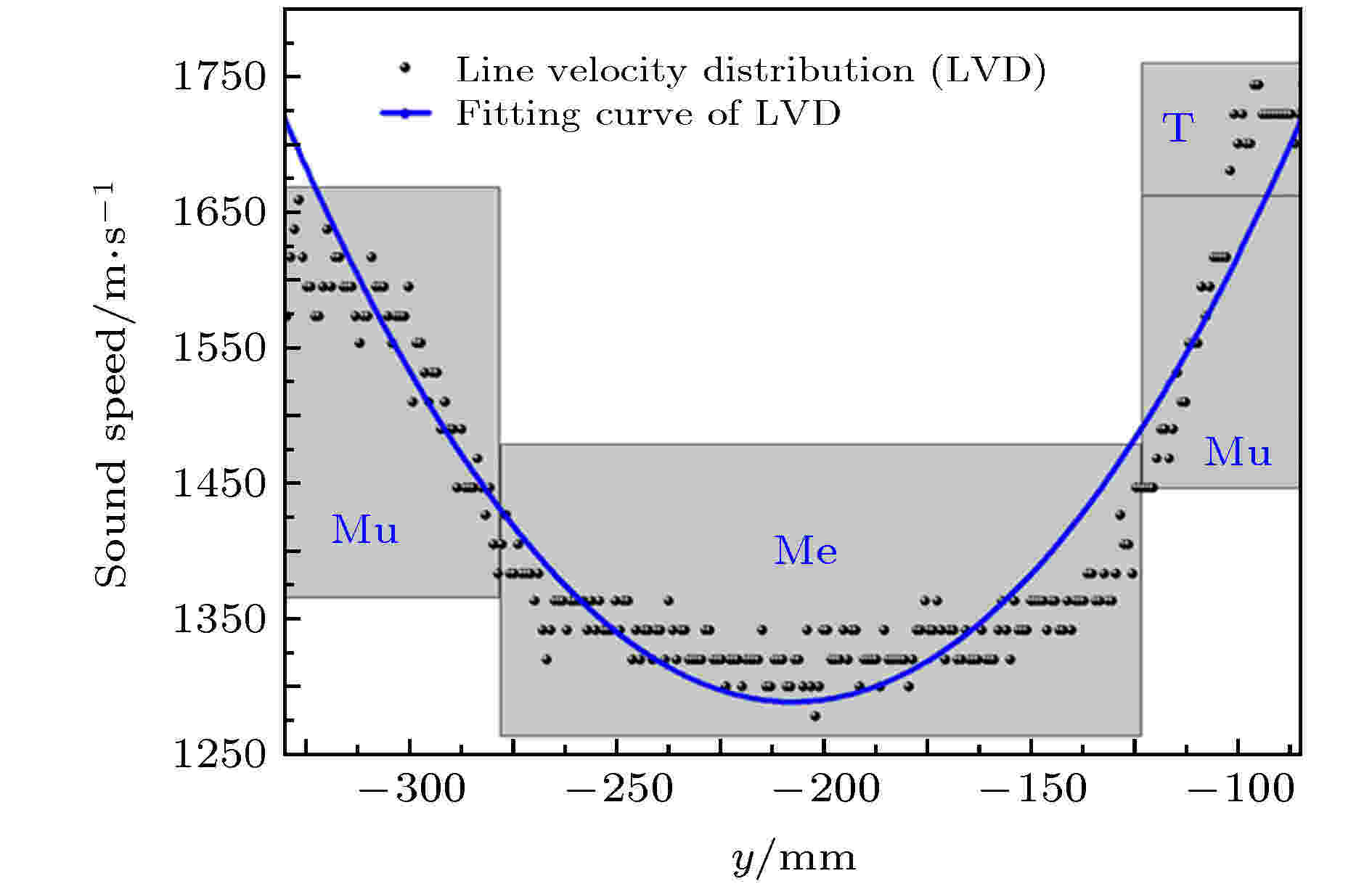
Figure4. Sound velocity distribution along the y axis corresponds to the line in the right part of Fig. 3(d). Mu, muscle; Me, melon; T, theca[36].
齿鲸声呐发射系统的声速分布重建结果表明齿鲸前额的组织结构分布相似. 中心核是一层低声速的脂肪性质的额隆组织. 外围为声速和密度较高的肌肉组织和结缔组织. 齿鲸前额软组织与骨质结构、气质结构形成了天然的声学梯度材料.
国内****对齿鲸生物声学的研究主要集中于声信号, 涉及种类包括分布于长江中下游流域的淡水性豚类和海洋性豚类, 包括白鱀豚(Lipotes vexillifer)、江豚(Neophocaena phocaenoides)、中华白海豚(Sousa chinensis)等[15,39-47]. 研究加深了对齿鲸回声定位过程中的一些现象的了解, 如江豚回声定位过程中对增益的控制[47]、声信号脉冲结构的分析[15]等. 图5比较了在厦门海域测量得到的中华白海豚宽带回声定位脉冲与东亚江豚窄带高频脉冲的时频特性. 中华白海豚回声定位脉冲的峰值频率与中心频率小于东亚江豚, 但其回声定位脉冲的–3 dB带宽以及–10 dB带宽均大于东亚江豚. 东亚江豚回声定位脉冲信号的结果与相关文献中的结果类似, 体现高频且窄带特点. 这两种齿鲸生物发出的不同能量的回声定位脉冲具有相似的频谱特性.
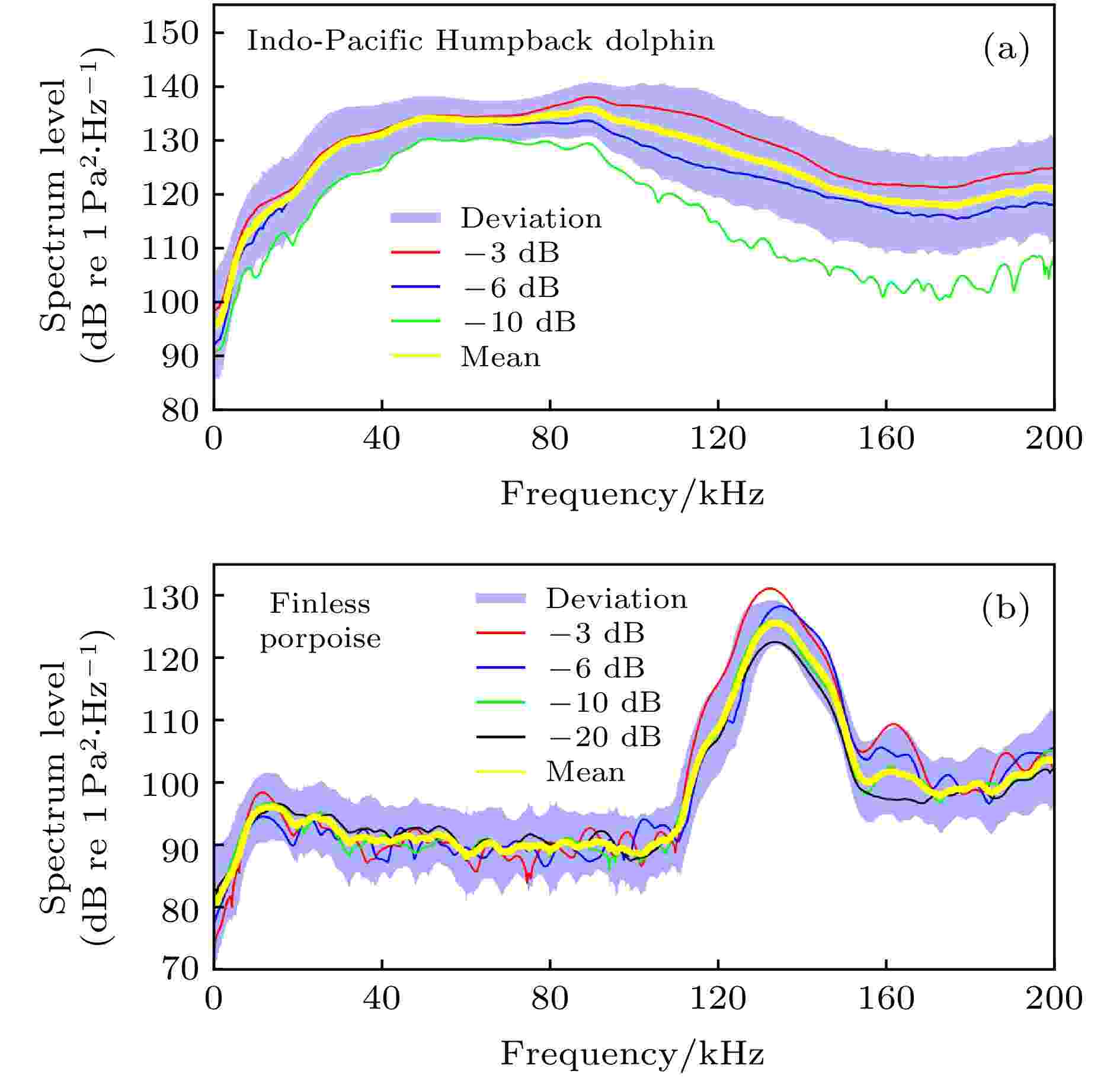
Figure5. (a) Mean spectrum of the clicks from –3 dB, –6 dB, and –10 dB groups for the Indo-Pacific humpback dolphin; (b) the mean spectrum of the clicks from –3 dB, –6 dB, –10 dB and –20 dB groups for the finless porpoise.
除了回声定位信号, 齿鲸另外一种具有代表性的声信号就是通信信号(whistles)[48-51]. 相比于回声定位脉冲, 通信声信号的时长变化范围大, 短的为毫秒量级, 长的可达秒级. 宽吻海豚还能发出用来鉴定个体的特征通信信号(signature whistles)[14]. 通信声信号可结合机器学习、卷积神经网络等人工智能算法进行识别与分类[52-54]. 许多研究以通信信号频谱曲线形状为参考, 根据频谱曲线随着时间变化的趋势, 将齿鲸通信声信号分为六类[55-59]: 固定频率类型信号(constant frequency)、上扫频类型信号(upsweep)、下扫频类型信号(downsweep)、凹型信号(concave or valley)、凸型信号(convex or hill)、正弦型信号(sinusoidal or multiple), 如图6所示. 齿鲸发出不同种类的通信信号可能与其行为有关. 分析厦门五缘湾圈养的宽吻海豚的通信声信号发现, 自由游动与训练两种状态下产生的通信声信号类别以及相关比例存在显著差异(如图7所示)[58,59]. 宽吻海豚在自由游动状态下的通信声信号主要为正弦型, 占总数66.4%. 其次是占22.47%的凸型信号和占9.03%的上扫频类型, 而其他类型的占比较小[59]. 宽吻海豚在训练状态下发出通信声信号绝大多数为上扫频类型, 占总数98.75%. 这与野外自由游动的齿鲸产生的通信信号与声学行为之间存在的联系是类似的[60-62].
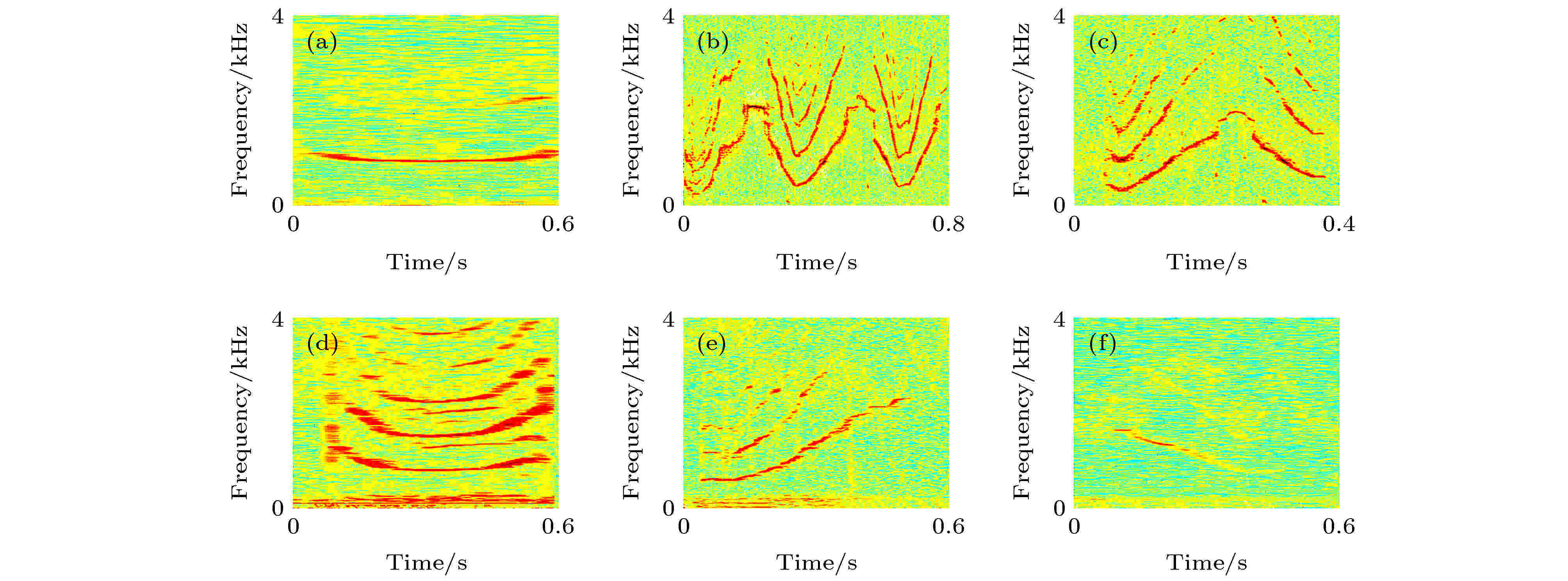
Figure6. (a) Spectrogram of constant frequency whistles of the bottlenose dolphins; (b) the spectrogram sinusoidal whistles of the bottlenose dolphins; (c) the spectrogram of convex or hill whistles of the bottlenose dolphins; (d) the spectrogram of concave or valley whistles of the bottlenose dolphins; (e) the spectrogram of upsweep whistles of the bottlenose dolphins; (f) the spectrogram of down sweep frequency whistles of the bottlenose dolphins[58,59].
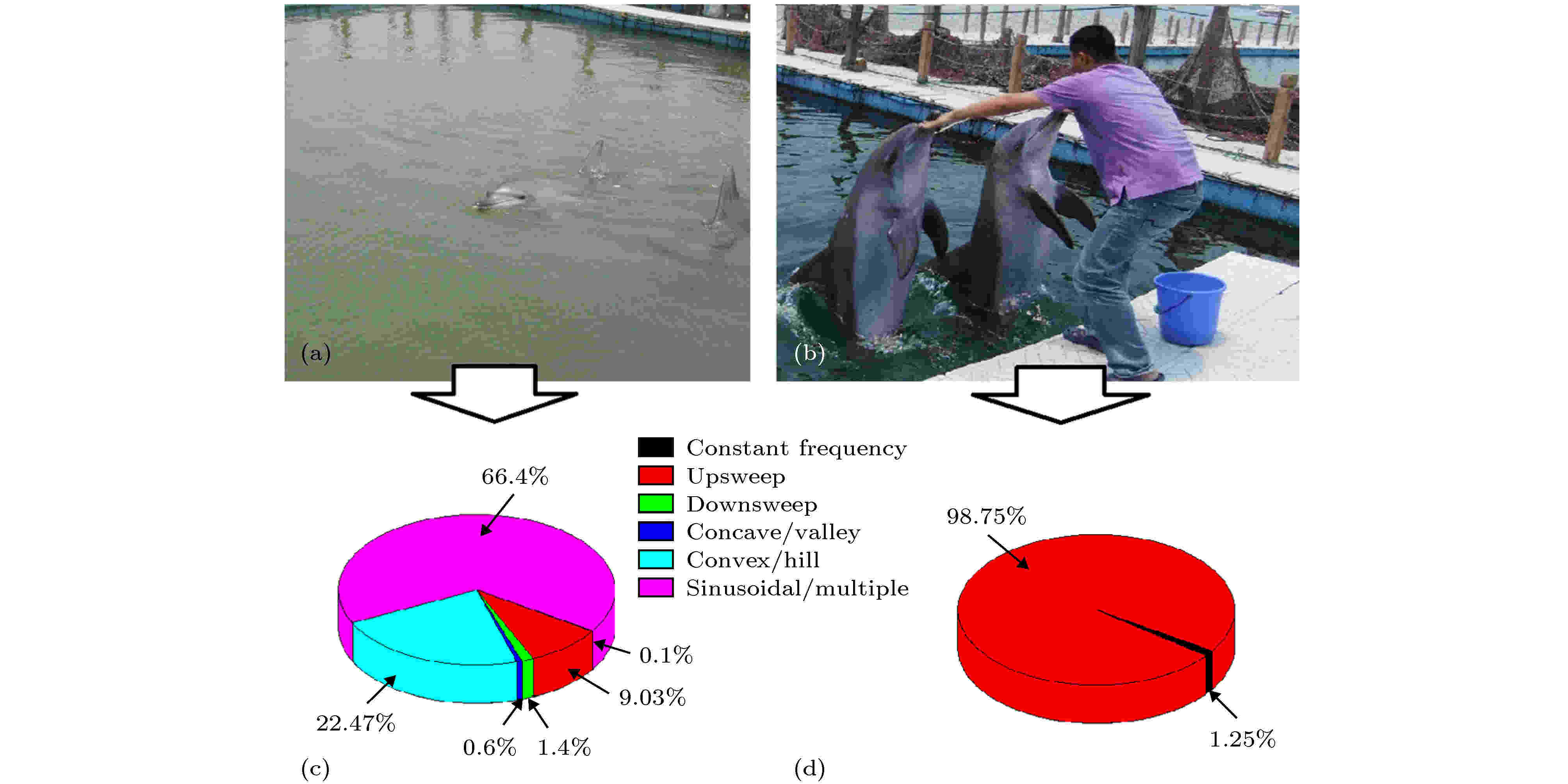
Figure7. (a) Two captive free swimming bottlenose dolphins in Xiamen; (b) bottlenose dolphins under training; (c) pie chart of the classified whistles of two bottlenose dolphins under free swimming; (d) pie chart of the classified whistles of two bottlenose dolphins under training conditions[58,59].
2
4.1.骨质结构的声反射和界面波波导作用
齿鲸的骨质结构由颅骨和上颌骨组成, 二者在结构上是相连的. 骨质结构的压力波声速、剪切波声速和密度分别为3380 m/s, 2200 m/s与2035 kg/m3[71-73]. 数值模拟研究结果表明, 骨质结构在齿鲸头部声传播过程中起着重要的反射作用[28-29,31,74-77]. 图8比较了鼠海豚包含头骨结构的模型I和移除头骨的模型II的声波波束特性[31]. 头骨骨质结构能有效地限制旁瓣. 头骨结构的声特性阻抗大于软组织、空气和水, 将声波往前反射的同时使声波往喙部以上传播, 从而形成向前传播的指向性波束, 体现了骨质结构对声波的反射作用, 这与传统上对上颌骨作用的认识相符合.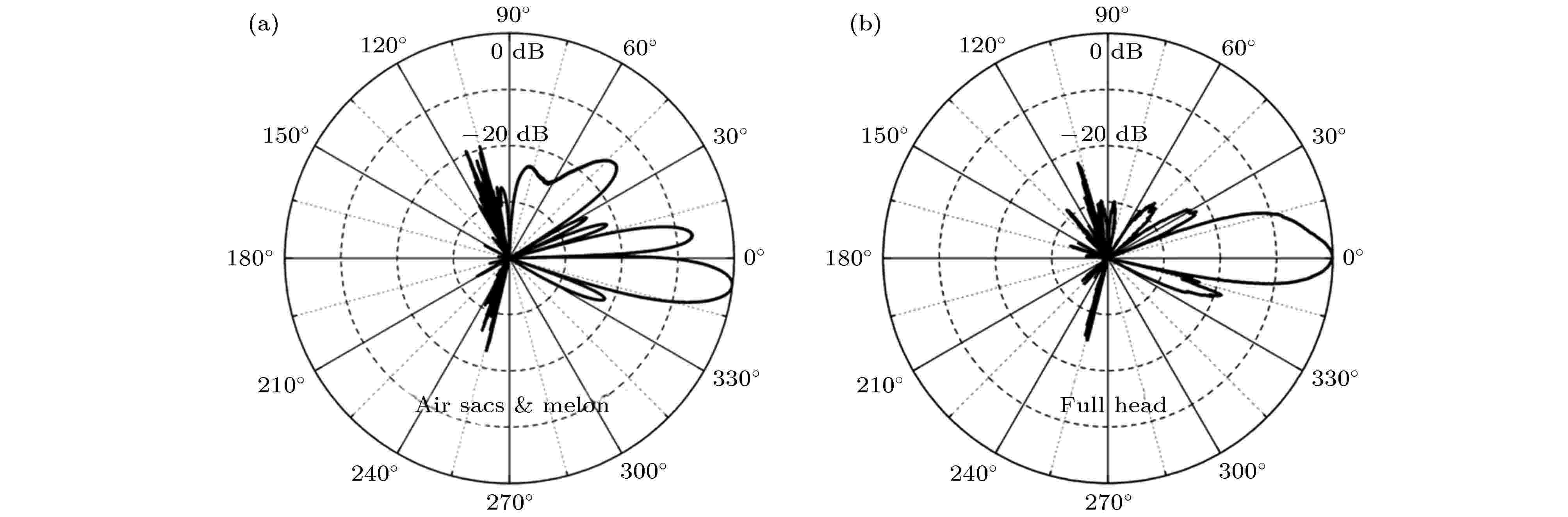
Figure8. (a) Beam directivity of a sound pulse with a centroid frequency of 130 kHz for No-Skull model of harbor porpoise; (b) the beam directivity of a sound pulse with a centroid frequency of 130 kHz for a complete model of harbor porpoise[31].
此外, 齿鲸的上颌骨还能激发剪切波, 产生固体位移振动[74]. 图9描述了白鱀豚声呐系统的声波传播过程, 声波除了沿着软组织往前传播, 还在上颌骨中激发出了系列声波向前传播[74]. 图10给出的位移分布表明, 上颌骨中的位移往前呈现出上升趋势, 在局部振动会达到最高峰, 接着逐渐减弱, 在到达上颌骨前端时, 又出现了一个局部区域峰值. 声传播过程表明软组织与上颌骨的界面会有沿着界面传播的声波, 界面波在离开上颌骨后与来源于软组织的声波叠加继续往水中传播. 骨质结构不仅能够反射声波, 还会激发沿着上颌骨-软组织界面传播的界面波, 完善对骨质结构声功能的认识.
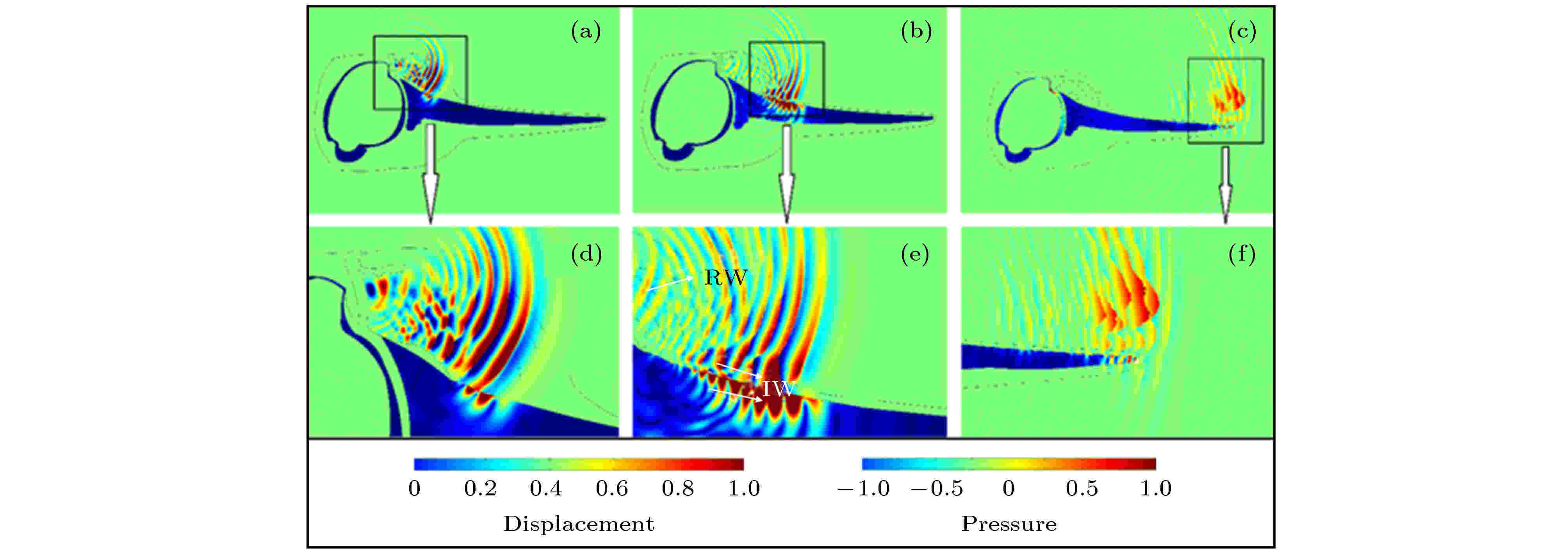
Figure9. (a) Propagation plot of a short-duration impulse source for Baiji in vertical section at time 1; (b) propagation plot of a short-duration impulse source for Baiji in vertical section at time 2; (c) propagation plot of a short-duration impulse source for Baiji in vertical section at time 3; (d) enlarged details of (a); (e) enlarged details of (b); (f) enlarged details of (c)[74].
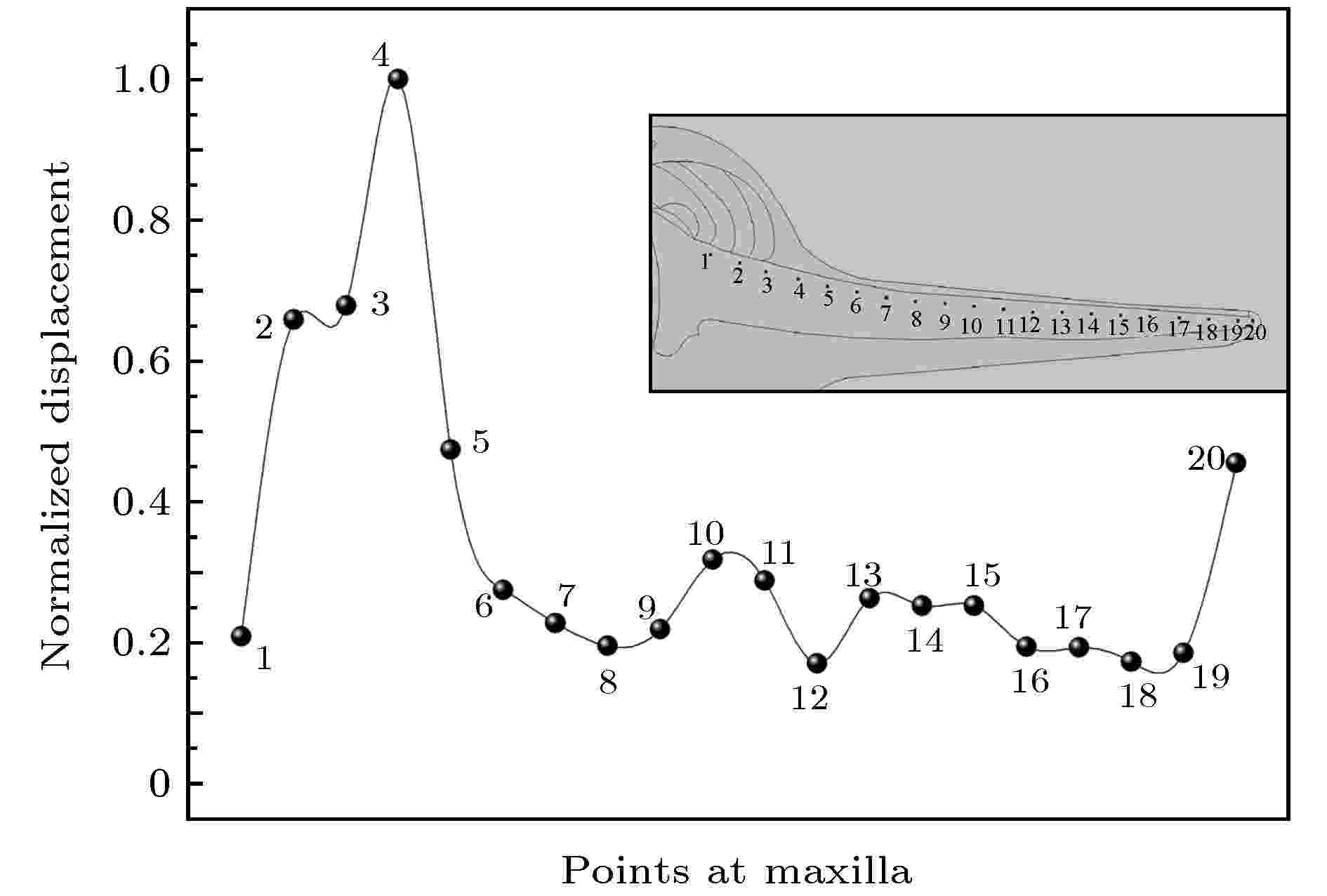
Figure10. Solid displacements of the 20 maxilla points of baiji[74].
2
4.2.气质结构在声传播与波束形成中的作用
齿鲸生物声呐系统的气质结构包含鼻道以及气囊系统. 气囊与鼻道是相连接在一起的气体通道系统. 气质系统内的气体循环与齿鲸的呼吸和发声相关. 以研究最为广泛的宽吻海豚为例, 其气质系统向外开口位于喷孔, 并通过鼻道向下相连至咽喉位置. 大部分齿鲸生物的气囊系统由喷气孔往鼻道方向包含三对气囊. 前庭囊位于额隆后侧的上方, 是最靠近喷气孔的一对气囊. 前颌囊紧贴于上颌骨后侧. 鼻额囊位于前庭囊和前颌囊之间. 齿鲸的气囊系统以体轴为中轴线, 在结构上呈左右对称, 但尺寸并不完全对称. 近年来的研究表明气囊和鼻道对声波传播与波束形成起着重要作用[28-31,35,75-77]. 以小抹香鲸为例(图11)[35], 头部模型在失去鼻道与气囊结构时, 部分声波向着后方、上方以及下方传播, 声波波束的旁瓣增多且能量泄漏变强. 鼻道的反射作用主要源于气质结构与前额软组织的声阻抗存在显著的差异, 在声学上起着绝对软边界的作用. 气质结构还可能通过结构共振对声波传播与波束控制产生影响.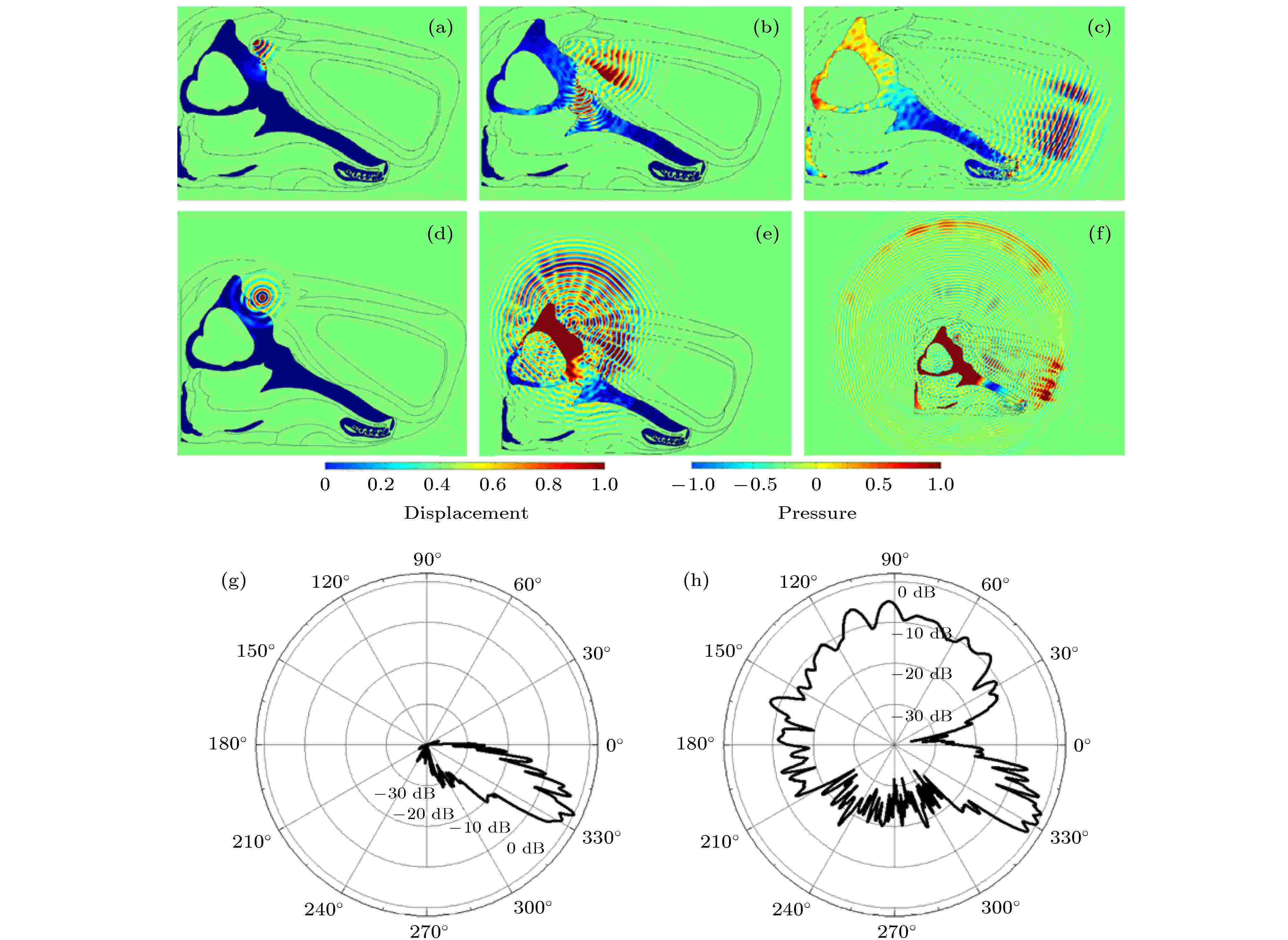
Figure11. (a) Propagation plot of the transient sound waves at time 1 under a full model case of pygmy sperm whale; (b) propagation plot of the transient sound waves at time 2 under a full model case of pygmy sperm whale; (c) propagation plot of the transient sound waves at time 3 under a full model case of pygmy sperm whale; (d) propagation plot of the transient sound waves at time 1 under a model case without air components; (e) propagation plot of the transient sound waves at time 2 under a model case without air components; (f) propagation plot of the transient sound waves at time 3 under a model case without air components; (g) the beam directivity of a sound pulse with a peak frequency of 125 kHz for the full model case of the pygmy sperm whale; (h) the beam directivity of a sound pulse with a peak frequency of 125 kHz for the model case of pygmy sperm whale without air components[35].
2
4.3.软组织在声波传播与波束形成中的作用
齿鲸的软组织位于鼻道前方, 上颌骨的上方, 占据着前额的大部分空间. 齿鲸前额软组织的声速和密度由内而外呈梯度分布, 存在一个低声速、低密度的内核[78]. 早期研究推测脂肪性质的额隆起着聚焦声波的功能. 然而, 近年来数值模型研究通过揭示声波在齿鲸头部内部传播的具体物理过程[30,31], 发现额隆的聚焦作用相对有限, 而更主要的是承担声波导、抑制旁瓣以及对声波辐射到水中进行阻抗匹配的作用. 图12描述了鼠海豚头部内部的声传播, 声波束的指向性在声波传播到额隆以前已经形成[31]. 当声波在前额软组织内部继续往前传播时, 低声速软组织对声波的传播起一定的波导作用, 改变了主瓣方向. 鼠海豚的额隆虽未对其远场的声波束主瓣产生明显的影响, 但是对于旁瓣还是有一定的抑制作用.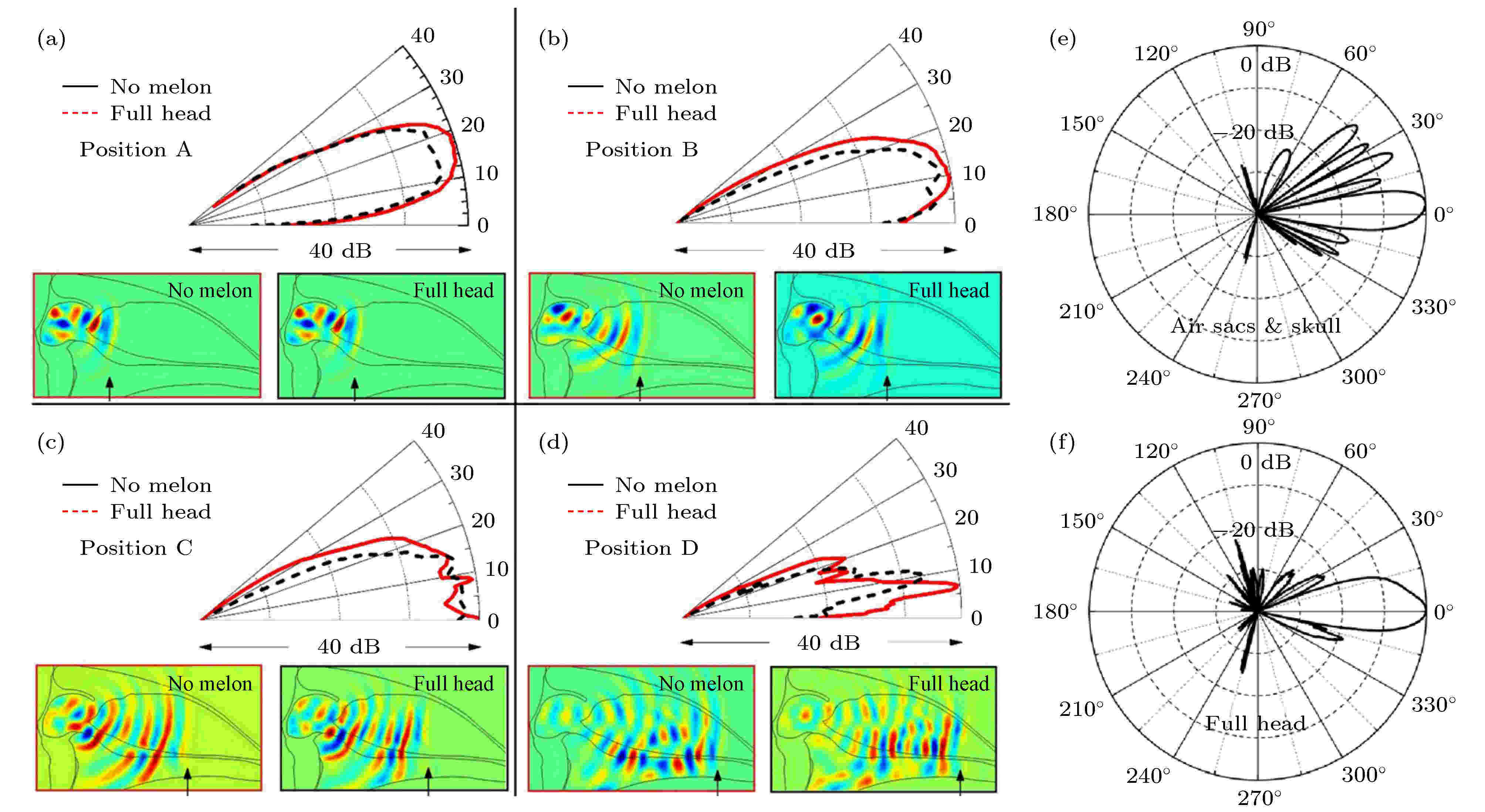
Figure12. (a) Acoustic field of no-melon and full head cases at time 1; (b) acoustic field of no-melon and full head cases at time 2; (c) acoustic field of no-melon and full head cases at time 3; (d) acoustic field of no-melon and full head cases at time 4; (e) the beam directivity of a sound pulse with a centroid frequency of 130 kHz for no-melon case of harbor porpoise; (f) the beam directivity of a sound pulse with a centroid frequency of 130 kHz for the full head case[31].
2
4.4.齿鲸头部声学结构联合作用对指向性声波束的调控效应
图13描述了东亚江豚气囊和软组织的联合作用对声波波束控制的综合调控效应[34]. 改变前庭囊方向从0°依次变为5°, 10°, 15°与20°, 并与此同时压缩软组织使前额的相对面积逐步从1减小0.96, 0.92, 0.87与0.83. 结果表明随着气囊朝向和压缩程度的增加, 声波的传播方向虽未受显著影响, 但声波波束呈现出规律展宽现象. 声波波束的–3 dB宽度随着压缩程度以及前庭囊的弯曲加深呈现上升趋势, 这与Wisniewska等[33]在实验中观测到鼠海豚在近距离探测中通过头部变形来展宽声波束, 提高声学视野的现象是一致的. 实验与仿真表明齿鲸也许能通过变形柔性声学结构来调控指向性声波束, 这为指导人工材料的设计提供了参考[79,80].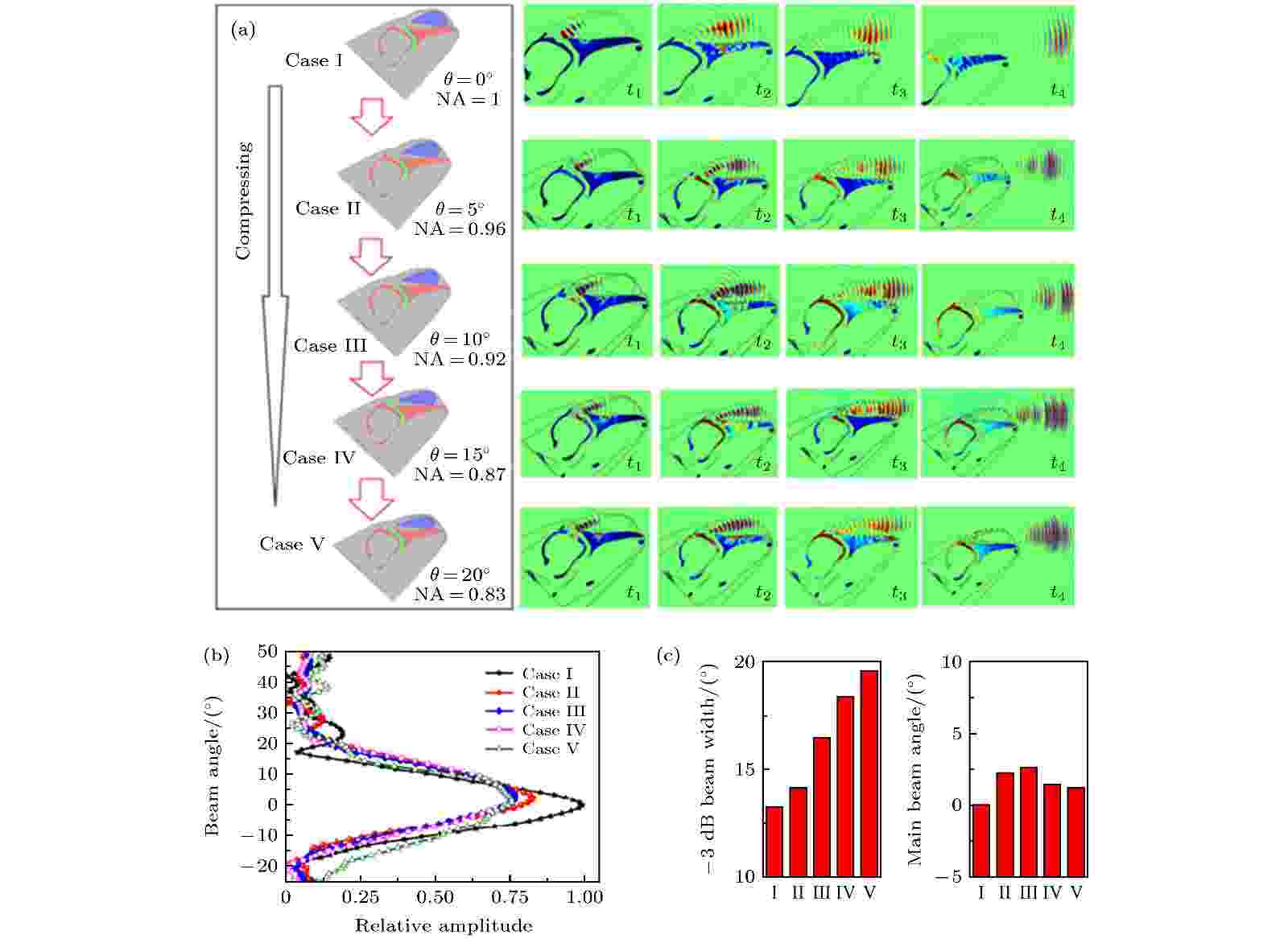
Figure13. (a) Compressing effect of models I, II, III, IV, and V on acoustic field of the sound pulse with a peak frequency of 125 kHz inside the head, where θ represents the orientation angle of the vestibular sac and NA represents the normalized area of the forehead tissues with respect to those of the original model I; (b) beam directivities of the five cases; (c) sound beams’ –3 dB beam widths and main beam angle distribution of the five cases[34].
骨质结构、气质结构和软组织形成一个多相系统, 联合调控齿鲸生物声呐系统的声传播以及波束形成. 上颌骨的声阻抗较大, 通过能反射声波与形成界面波波导双重作用调控声波. 气质结构的气囊系统与鼻道结构的声阻抗较小, 主要通过形成软边界反射声波影响声波传播. 而软组织的声阻抗虽然接近水, 却形成一个声速、密度呈梯度状的整体指引声波往低声速、低密度区域引导. 声波能在这三种声学结构形成的天然的多相介质调控下形成高指向性的声波束. 声源位于鼻道系统上, 发出的声波首先被位于后侧的鼻道与上侧的气囊反射向前传播. 位于前额下侧的上颌骨则能保障声波始终处在水平面上方而阻止声波往头部下方泄漏. 位于气质系统前侧, 上颌骨上侧的软组织结构将被反射的声波聚集往前传播至水中. 这种不同声阻抗特性结构按照特定的空间几何分布形成了高效的声调控系统有效调控声波传播与波束形成.
感谢中国科学院深水生生物研究所王丁研究员、王克雄研究员, 中国科学院深海科学与工程研究所李松海研究员, 自然资源部第三海洋研究所王先艳副研究员与杨燕明研究员, 以及厦门大学环境与生态学院李炎教授的支持与帮助.