全文HTML
--> --> -->次临界能源堆能源供应主要依靠裂变包层中贫化铀裂变释放裂变能来实现, 裂变反应率的准确性在设计能量放大指标中异常重要. 英国的Weale等[10]用直径29.2 mm的天然铀棒搭建了一个高1066 mm, 直径990 mm的准圆柱装置, 用圆柱形裂变电离室测量了238U(n, f)及235U(n, f)裂变反应率分布. 日本东京大学的Akiyama等[11]用铀块堆砌了等效半径为457.2 mm的准球形装置, 利用柱形裂变室测量了14 MeV中子源条件下装置内侧238U(n, f)及235U(n, f)反应率, 不确定度为5.5%—6.0%. Afanas’ev等[12]在聚变堆包层模拟装置上开展14 MeV中子学积分实验, 用固体径迹探测器测量238U(n, f)及235U(n, f)反应率, 相对不确定度在8%. 国内朱通华等[13]在贫化铀聚乙烯交替球壳上用长杆平板型贫化铀裂变室测量了裂变反应率径向分布, 不确定度为3.4%.
Weale实验与Akiyama实验所用的装置均采用现有材料堆砌而成, 基准性不足. 另外, 上述实验测量无论采用裂变室[14]还是固体径迹探测器[15,16], 均在被测位置引入了其他材料, 破坏了测量对象的原始状态, 使得测量点的物理参数发生改变, 要对测量值进行修正才能得到所需的物理量. 为了解决以上探测手段对测量的影响, 本文采用与宏观贫化铀球壳丰度完全相同的贫化铀片状样品作为活化探测器[17,18], 活化探测器可以看作被测模型的一部分, 最大限度降低了对被测对象的影响. 利用中国工程物理研究院现有系列贫化铀球壳, 构建五种不同厚度的实验模型, 选择与D+粒子(D+粒子指氘气电离后形成的带正电的原子核, 经引出、加速、然后轰击氚靶产生中子)水平呈45°角的测量孔道, 采用活化法基于14 MeV D-T中子开展实验研究. 选择裂变碎片中的143Ce[19]作为测量对象, 通过测量其发射的γ射线, 实现143Ce核子数的测量, 再根据裂变产额数据和中子产额监测数据, 获得裂变反应率沿45°方向分布规律, 为裂变放能与球壳厚度关系研究提供数据支持.
2.1.测量原理
绝对裂变反应率是指一个源中子诱发一个核材料原子核产生裂变的概率[20]. 通过测量装置内被测位置的核裂变计数, 可得到该处的裂变反应率. 裂变率计算公式为


本实验选取裂变产额比较准确的裂变碎片143Ce作为测量对象, 核裂变数



贫化铀主要成分为238U和235U, 将中子能量从0 keV到15 MeV分成8个能段(238U只计算后7个能段), 143Ce总裂变产额







143Ce的半衰期为33 h, 能量为293 keV的γ射线的分支比是42%. 通过测量这条γ射线可以得到143Ce核子数. 143Ce核子数









由(1), (2)和(4)式可得裂变反应率f, 如(5)式所示:
(5)式中各参数的意义同上.
2
2.2.贫化铀装置
贫化铀装置[21]共分五种模型, 内半径Rin均为13.1 cm, 外径及组合厚度见表1. 贫化铀的密度为(18.8 ± 0.1) g/cm3, 其中238U和235U同位素丰度分别为99.58%和0.416%[22]. 在装置的水平径向留有放置D+离子束流漂移管的靶室孔道, 水平方向与D+粒子漂移方向呈45°和90°方向留有两条测量孔道, 竖直方向有一条测量(吊装)孔道, 靶室孔道及测量孔道直径均为44 mm. 本次实验在45°孔道开展实验.模型编号 | 外半径Rout/cm | 厚度L/cm |
1 | 18.10 | 5.00 |
2 | 19.40 | 6.30 |
3 | 23.35 | 10.25 |
4 | 25.40 | 12.30 |
5 | 28.45 | 15.35 |
表1五种贫化铀球壳的外径及厚度
Table1.Radius and thickness of depleted uranium shells.
实验测量时需在测量孔道内放置一个贫化铀套筒, 与套筒匹配有不同厚度(5, 10, 20和30 mm)的圆柱形贫化铀塞块, 用于填充活化探测器之外的空间, 以尽可能保证贫化铀球壳的完整性, 最大限度避免空腔效应及其他材料对贫化铀球壳中子场的扰动. 贫化铀装置及其蒙特卡罗模型分别见图1(a)和图1(b), 贫化铀装置置于一铁支架上, 其中心与中子源中心重合(偏差 < 3 mm). 活化探测器与塞块交替放置在套筒内, 它们的材料成分均与贫化铀装置一样的, 5片活化探测器在装置中的分布情况见图1(b)中“1, 2, 3, 4, 5”. 为降低实验大厅散射中子本底影响, 实验装置距离实验大厅周围墙壁、地面及屋顶的距离均在3.5 m以上.
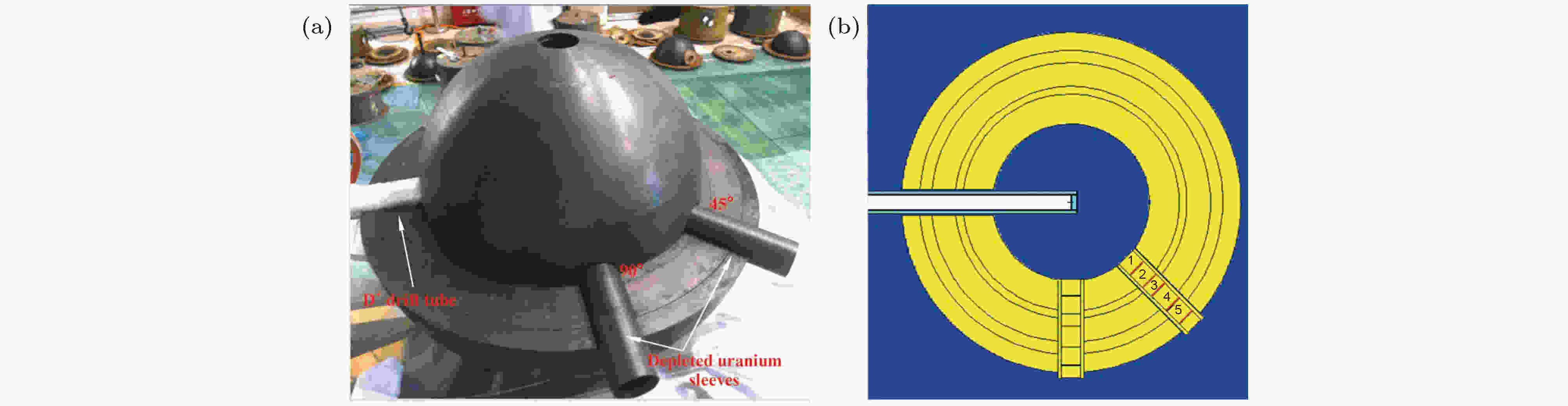
Figure1. (a) Physical map of depleted uranium device; (b) distribution of five activation detectors in Monte Carlo Model 5 (1, 2, 3, 4, 5 in the direction of 45°).
2
2.3.活化探测器
实验时, 每种模型均布放5片活化探测器, 活化探测器位置pi见(距中子源距离)表2. 测量孔道与D+粒子入射方向呈45°, 测量孔道内放置贫化铀套筒, 活化探测器置于贫化铀套筒内, 活化探测器之间用贫化铀塞块填充. 套筒内径Φ3.2 cm, 外径Φ4.2 cm, 活化探测器直径Φ2.4 cm, 标称厚度0.2 mm, 塞块直径Φ3.15 cm.模型编号 | L/cm | ||||
p1 | p2 | p3 | p4 | p5 | |
1 | 13.60 | 14.62 | 15.64 | 16.16 | 17.18 |
2 | 14.60 | 15.62 | 16.64 | 17.66 | 18.68 |
3 | 14.60 | 16.62 | 18.64 | 20.66 | 21.68 |
4 | 15.60 | 18.62 | 20.64 | 22.66 | 24.68 |
5 | 15.60 | 18.62 | 20.64 | 24.16 | 27.18 |
表2五种模型中活化探测器的布放位置
Table2.Position of activation detector in various models
2
2.4.中子源及产额监测
中子源由中国工程物理研究院核物理与化学研究所的中子发生器提供, 中子源靶室置于装置中心. 直管式铝制靶室外径26 mm, 靶管外为铝制水套, 内外直径分别为37 mm和39 mm, 靶管与水套之间为循环冷却水. TiT靶活性区直径为12 mm, TiT靶为厚靶(D+粒子全部阻止在T-Ti层内), 平均入射D+粒子能量为135 keV, 对应最大中子能量为14.9 MeV.绝对中子产额通过伴随α粒子法监测, 探测器置于漂移管中与D+束流方向夹角178.2°, 束流强度为300 μA时, 中子产额约为3 × 1010—4 × 1010 s–1. 中子产额监测采用分时记录系统, 该记录系统时间步长为10 s, 能够精确反映实验期间中子产额波动, 实验期间中子产额波动修正由(4)式中的“辐照过程修正因子”k完成.
2
2.5.γ射线探测系统
测量γ射线的探测系统为ORTEC公司的TRANS-SPEC-DX100电制冷HPGe探测器和GammaVision谱分析软件. Ge晶体直径67.0 mm, 长度51.7 mm, 死层厚度0.7 mm. HPGe探测器置于屏蔽体内, 工作高压为–4500 V, 对60Co的1.33 MeV γ射线能量分辨率为1.87 keV, 相对探测效率40%.利用到60Co, 133Ba, 152Eu等γ点源对HPGe探测器效率刻度, 得到探测器表面中心位置点源的探测效率曲线. 面源的探测效率采用积分的方法获得. 在探测器表面径向每隔3 mm测量一个点源探测效率, 拟合出径向的探测效率变化曲线, 在半径为12 mm的圆面内积分得到面源探测效率. 经标定, 位于探测器端面直径为24 mm的面源发射的293 keV γ射线的探测效率为7.44%. 293 keV的γ射线在贫化铀片中的自吸收因子由理论模拟得到(已由实验验证), 每片贫化铀片的自吸收因子根据实际厚度进行计算, 实验所用25片贫化铀片自吸收因子介于85%—92%之间.
3.1.实验结果及分析
辐照实验完成后, 为降低短半衰期的γ本底, 将活化探测器冷却2 h, 然后在HPGe探测器表面对活化探测器进行了实验测量, 得到裂变碎片143Ce发射的293.3 keV γ射线能谱, 如图2所示. 与γ射线测量相关的自吸收修正因子、探测效率、辐照过程修正因子等实验前都进行了测量及标定.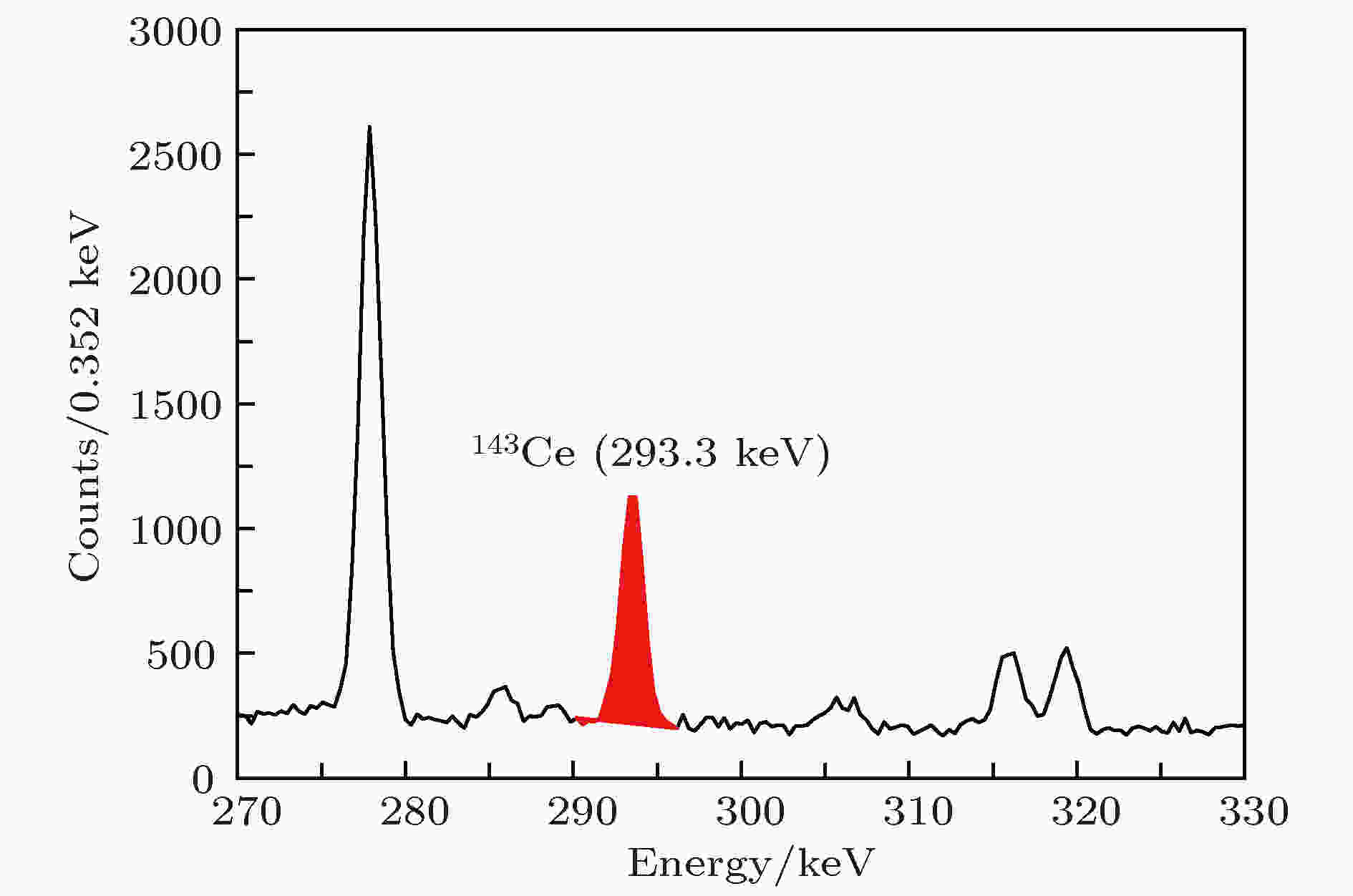
Figure2. γ spectrum of depleted uranium activation detector, detected by using HPGe detector.
为了获得143Ce的总裂变产额, 分能区理论模拟了不同位置238U和235U的裂变反应率, 得到了






将293.3 keV γ射线总计数、自吸收修正因子、射线分支比、探测效率、辐照过程修正因子、

Model No. | p1/% | p2/% | p3/% | p4/% | p5/% |
1 | 4.29 | 4.32 | 4.33 | 4.34 | 4.34 |
2 | 4.33 | 4.35 | 4.37 | 4.38 | 4.37 |
3 | 4.36 | 4.41 | 4.45 | 4.45 | 4.46 |
4 | 4.40 | 4.47 | 4.49 | 4.50 | 4.49 |
5 | 4.41 | 4.48 | 4.51 | 4.55 | 4.55 |
表3YCe-143值
Table3.Values of YCe-143.
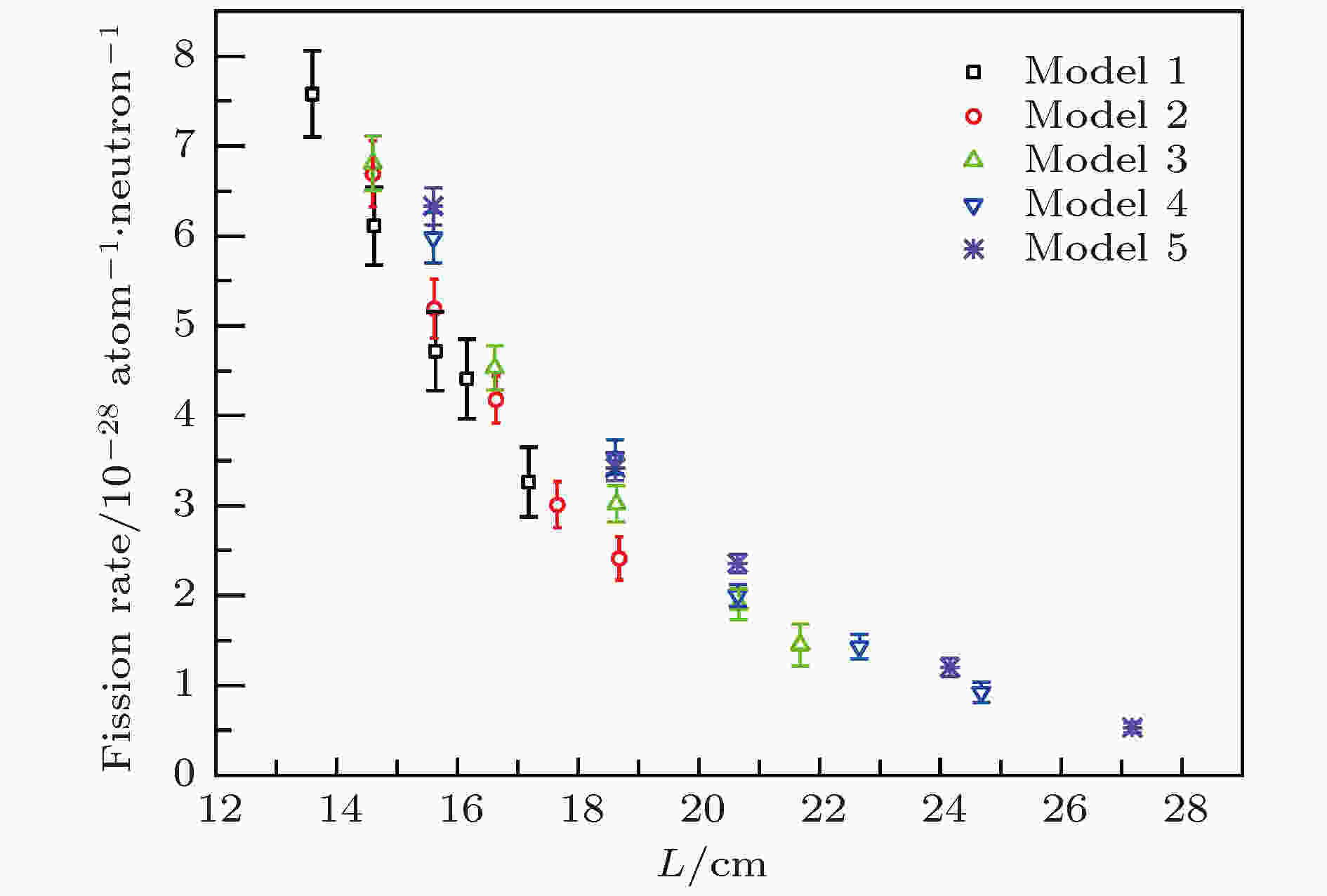
Figure3. Fission reaction rate distribution for five models.
从图3可以看出: 1)每种模型, 随着距中子源距离L的增加裂变反应率逐渐变小, 厚模型变化幅度比薄模型变化幅度更大, 主要原因是距中子源越远中子通量密度越小, 裂变反应率自然降低; 2)相同测量位置, 模型越厚, 裂变反应率越大, 原因是外层贫化铀球壳的屏蔽与反射作用使得测点处中子通量密度增大, 为此用蒙特卡罗模拟计算了各模型测量位置处的中子通量密度, 结果见图4.
实验结果的不确定度主要来自中子产额、裂变产额和γ射线测量三个方面. 实验前对α探测器和靶片在靶管中的几何位置进行了准确测量, 对准直光栏孔径采用显微镜进行了测量, 各向异性修正因子通过查表得到, 保证了中子产额的不确定度小于2.5%[24]. 裂变产额不确定度小于5%. γ射线测量的不确定度主要来自HPGE探测器探测效率、γ射线自吸收因子[25]及γ谱解谱. 探测效率用系列标准源进行了标定, 不确定度小于2%[26], 自吸收因子进行了理论模拟及实验验证, 不确定度小于0.5%, 解谱不确定度为2%—10% (不同位置处活化探测器不确定度不同). 五种模型各位置裂变反应率的总不确定度见表4.
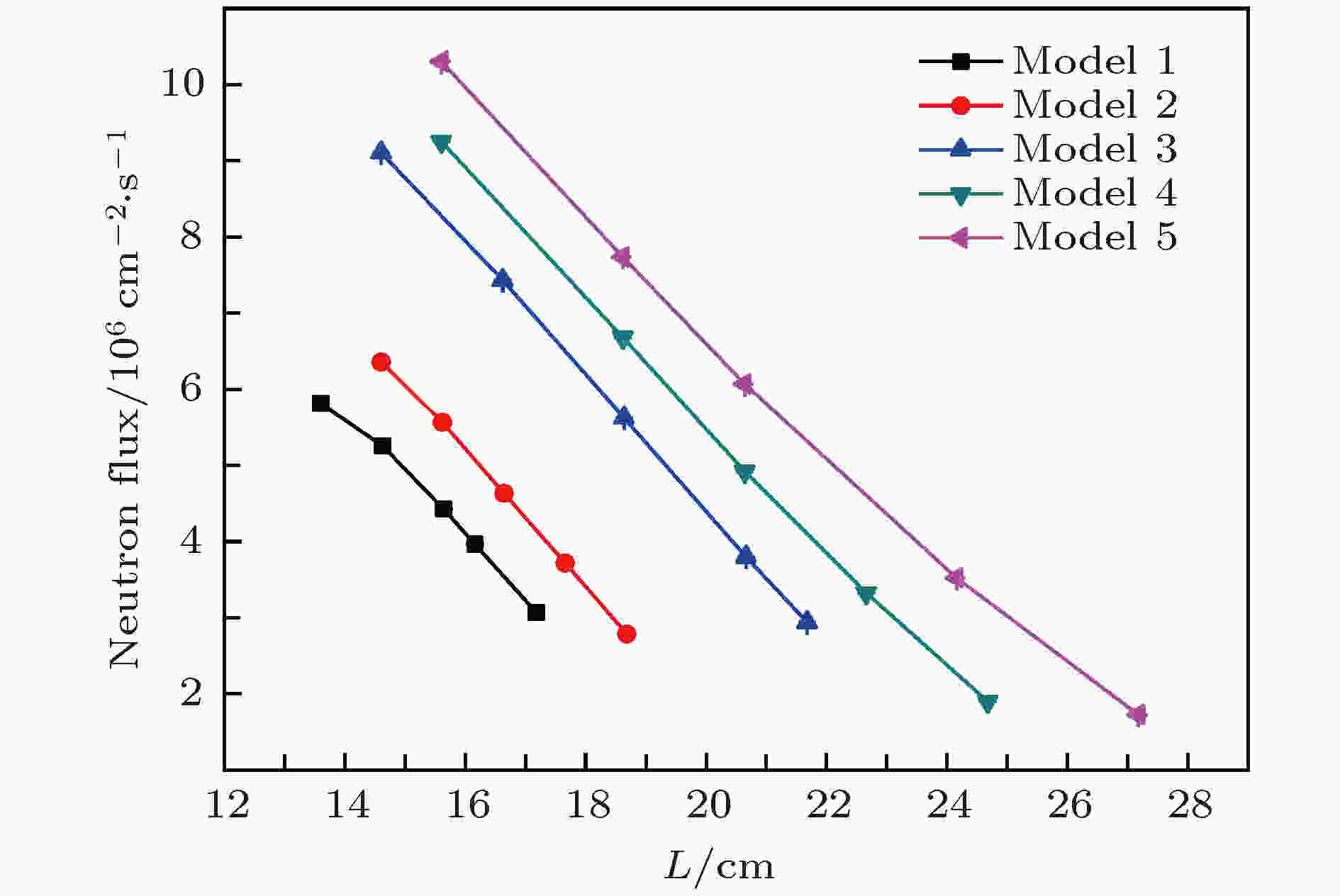
Figure4. Neutron flux density at various measuring positions of five models (Monte Carlo simulation).
Position | Model 1 | Model 2 | Model 3 | Model 4 | Model 5 |
p1/% | 6.5 | 6.5 | 6.5 | 7.4 | 6.1 |
p2/% | 6.2 | 6.3 | 5.7 | 7.2 | 7.0 |
p3/% | 6.5 | 5.8 | 6.7 | 8.6 | 10.0 |
p4/% | 6.5 | 6.3 | 6.5 | 9.5 | 9.5 |
p5/% | 6.5 | 6.1 | 7.0 | 10.9 | 10.9 |
表4裂变反应率总不确定度
Table4.Synthesize uncertainty of fission reaction rate.
2
3.2.蒙特卡罗模拟与实验比较
利用MCNP5程序和ENDF/VI.8数据库对五种模型进行理论模拟, 用F4栅元卡配合计数乘子卡F4得到了不同测量位置的裂变反应率, 不确定度小于3.4%. 为更清晰地分析计算值与实验值的差异, 用计算值与实验值的比值(C/E)来进行表征, 结果见图5. 可以看到, 五种实验模型C/E值介于0.9至1.1之间, 表明计算与实验在10%以内符合, 且对于大部分测量位置其比值都落在测量标准不确定度范围之内.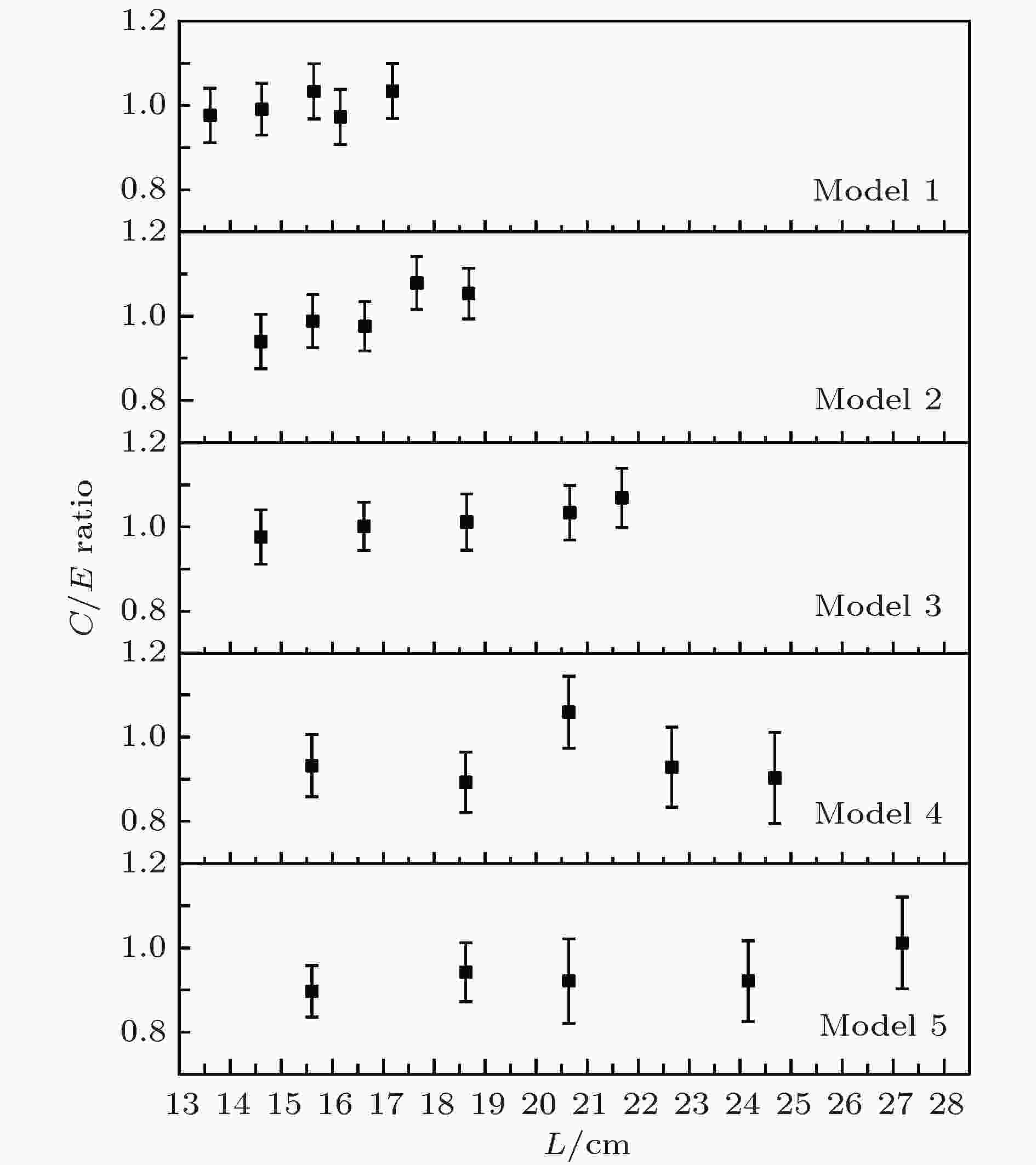
Figure5. C/E ratio of fission reaction rate for various measuring position in depleted uranium assembly.
本方法克服了裂变室及固体径迹探测器的不足, 最大限度降低了探测器对测量对象的影响, 使得测量结果更加真实反映宏观模型内部裂变放能特性; 细分了测量位置的中子能谱, 采用最新的143Ce裂变产额数据, 提高了总裂变产额
