全文HTML
--> --> -->氮化镓(GaN)基III—V族异质结材料(典型如AlGaN/GaN)作为第三代宽禁带半导体的重要代表之一, 具有明显的自身材料优势. 首先, GaN具有大的禁带宽度, 室温下为3.4 eV, 是硅的3倍, 砷化铟的9倍, 锑化铟的20倍, 因此其具有更高的临界击穿电场、更优的高温工作稳定性, 能够应用在300 ℃以上的极端环境中[10—14]. 其次, GaN的理论本征载流子浓度很低, 因此制作的霍尔传感器噪声小、灵敏度较高. 另外, GaN基III—V族异质结界面处势阱中, 高密度的自发极化和压电极化面电荷产生强的电场, 诱导电子汇聚在势阱中并限制其在二维空间运动, 从而形成无需故意掺杂就具有的2-DEG[15,16], 因此具有明显高出体材料的电子迁移率(约2000 cm2/(V·s)). 利用这种GaN基异质结材料制作的霍尔传感器, 其工作敏感度较高, 非常适合应用在高温工作环境中[17,18]. 最后, GaN基霍尔传感器的研发还可借助于现有庞大的GaN基LED行业, 从而提高产品生产规模, 降低成本, 因此具有广阔的市场应用前景.
目前, 国际上已经有少量基于AlGaN/GaN异质结材料的霍尔传感器的研究报道, 其霍尔电极分布主要是十字形或者方形结构的水平型, 一般具有四端电极, 这种类型的传感器只能探测垂直于器件表面的磁场[13,19,20]. 而用于探测平行于器件表面磁场的垂直型霍尔传感器至今并未见相关研制成果报道. 垂直型霍尔传感器的设计和制作具有重要意义, 它的突破使得在外延片上进行芯片制作环节就能实现具有三维磁场探测功能的高端霍尔传感器[19,21—24], 因此能明显减小芯片体积、缩减成本. 三维霍尔传感器具有更广的应用范围、更优的使用便捷性和准确性, 缩小芯片体积后还能应用于某些对空间要求苛刻的特殊测试环境. 目前限制GaN基材料垂直型霍尔传感器的主要技术瓶颈之一是2-DEG沟道虽然具有高的电子迁移率, 但是在异质结界面处垂直于沟道方向具有很高的纵向电场, 电子被限制在界面的沟道中, 因霍尔效应产生的洛伦兹力驱离载流子偏移原输运轨道的能力较弱, 从而导致检测到的霍尔电压较小, 传感器探测敏感度降低.
为了解决以上问题, 本文提出具有五端电极分布、采用2-DEG沟道势垒层选区浅刻蚀形成局部凹槽结构的AlGaN/GaN异质结垂直型霍尔传感器. 本文首先从理论上论证采用该方案实现垂直型霍尔传感器的优势, 同时, 为了获得更加准确可靠的器件仿真结果, 在仿真前对材料参数和物理模型进行了校准. 仿真中对器件的几何参数进行了系统地优化, 并探讨了不同结构参数对垂直型器件性能的影响, 重点研究势垒层刻蚀深度对器件霍尔电压的影响. 仿真结果表明本文设计的器件具有较大的磁场探测灵敏度和优良的工作温度稳定性, 对磁场传感器技术的应用发展具有理论指导意义.
2.1.霍尔传感器的关键评价指标
传统的半导体水平型霍尔传感器一般有四个电极, 其中两个电极是电流输入端, 另外两个电极是电压输出端. 通过在半导体两个输入端电极通入恒定电流, 在垂直于半导体平面方向施加外磁场, 则半导体内的载流子将受到洛伦兹力的作用, 因此向垂直于电流和磁场方向的半导体两侧聚集, 稳定时在两侧电极形成电势差, 即霍尔电压(VH). 五电极垂直型霍尔传感器首先由Popovic[24]提出, 可以采用恒压或恒流方式激励, 采用恒流激励测量电压信号时, 其霍尔电压可表达为[24—26]评价霍尔传感器的关键指标之一是磁场灵敏度, 常用电流相关敏感度SI标记, 表示单位电流、单位磁场强度下获得的霍尔电压大小, 其具体表达式为[24,26]
2
2.2.GaN基异质结垂直型霍尔传感器结构设计
本文利用局部刻蚀减薄AlGaN势垒层, 通过减小异质结界面处极化电荷诱导产生的纵向电场, 从而有效提高测量得到的霍尔电压, 该垂直型霍尔传感器的剖面和俯视结构如图1所示. 传感器结构采用Si衬底和AlN应力缓冲层, 其上外延生长非故意掺杂的GaN材料和Al0.25Ga0.75N势垒层. C0, C1和C2为传感器主电极; 长度均为l1; S1和S2为感测电极, 长度均为l2; C0与C1(或C2)间距为L1, 器件结构关于中心主电极C0呈对称分布. S1和S2经浅刻蚀减薄后, 保留其势垒层厚度为d, 感测电极S1(或S2)与中心主电极C0边缘的距离为L2, 传感器的有效宽度记作w. 测量过程磁场方向平行于传感器表面电极, 如图1所示.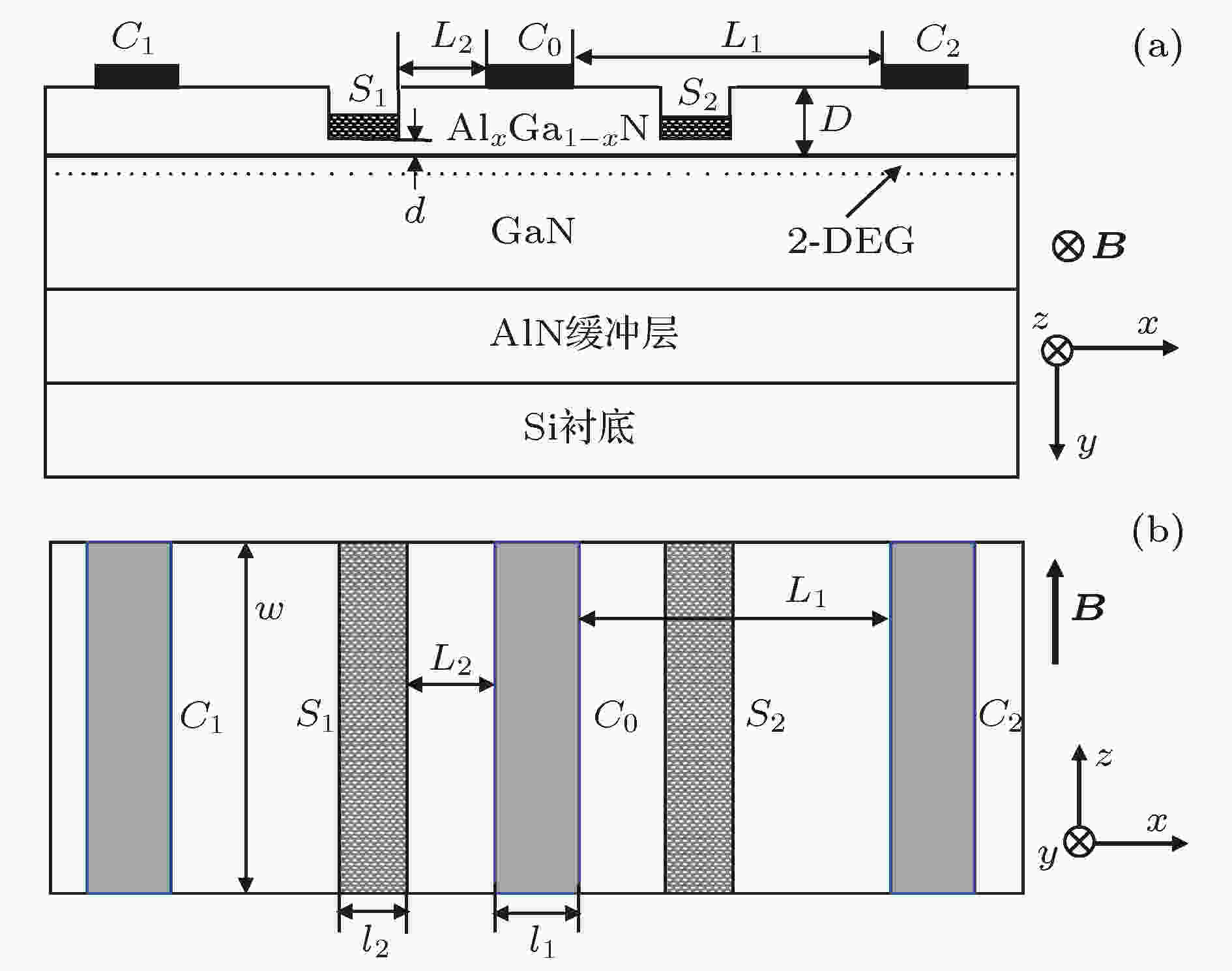
Figure1. Schematic diagram of GaN-based vertical Hall sensor: (a) Sectional and (b) top views.
垂直型霍尔传感器工作原理如下: C1和C2电极作为公共端接地, C0作为激励源的电流输入端, 测量S1和S2之间的电势差即为霍尔电压; 无平行器件表面的外加磁场时, 由于器件结构的对称性, 感测电极处的电势相等, 霍尔电压为零; 如图1所示, 当存在平行器件电极方向的磁场B时, 主电极C0→C1和C0→C2的电流方向与磁场方向相互垂直, 载流子在运动过程中会受到垂直于电流和磁场方向(即y方向)的洛伦兹力, 由于C0→C1和C0→C2的电流方向相反, 两者受到的洛伦兹力方向也相反, 从而发生载流子在y方向(纵向载流子分离, 这是垂直型霍尔传感器的重要特征)运动偏移, 形成明显的电势差, 即霍尔电压VH.
对于常规GaN基异质结结构(S1和S2下方势垒层未进行刻蚀), 2-DEG沟道界面处极化正电荷诱导产生的电场力能有效抵消外加磁场形成的洛伦兹力, 因此沟道中载流子发生纵向偏移的能力明显减弱, 因此感测得到的霍尔电压过小, 传感器的灵敏度较低. 本文通过减薄感测电极下方的势垒层, 缩短势垒层表面极化负电荷与2-DEG沟道距离, 利用该极化负电荷诱导产生的相反方向电场力来部分抵消异质结界面处过高的纵向电场力. 由于该方案保留部分势垒层厚度, 保证了光滑平整、完好无损的沟道界面, 2-DEG电子迁移率大小并未受明显影响, 而载流子纵向运动偏移的能力得到增强, 因此传感器感测霍尔电压明显增大.

从真实器件应用出发, 本文仿真过程重点关注温度对磁场传感器特性的影响, 因此仿真过程引入温度相关迁移率模型并加以优化. 本文采用了Farahmand等[30]通过拟合蒙特卡罗计算结果得出的用于GaN材料温度相关的低场电子迁移率模型, 该解析模型较为精确地考虑了合金组分、温度特性、合金无序效应等因素, 其表达式如下:
本文仿真中材料和器件结构参数取值如下: AlxGa1–xN势垒层厚度为25 nm, Al组分x = 0.25, GaN外延层厚度为6 μm, AlN缓冲层厚度为25 nm; C0, C1和C2主电极长度l1 = 1.5 μm, S1和S2感测电极长度l2 = 1 μm, C0与C1 (或C2)间距L1 = 8.5 μm, 感测电极S1 (或S2)与中心主电极C0的距离L2 = 2 μm; 器件台面宽度w = 10 μm; GaN材料的背景载流子浓度为5 × 1014 cm–3; 结构参数优化过程中, AlxGa1–xN势垒层厚度d的取值范围为1 nm ≤ d ≤ 25 nm. AlxGa1–xN势垒层剩余厚度d的大小将显著影响2-DEG沟道中电子浓度和迁移率大小, 为了使仿真贴近实际器件工艺, 本文首先在仿真中对物理参数进行校准. Anderson等[31]报道了AlGaN势垒层浅刻蚀的HEMT器件的电学特性, 给出了刻蚀深度对器件参数变化(特别是电子迁移率)的具体影响, 本文通过对比仿真得到的转移特性曲线与文献实验数据来校准仿真中所用物理参数. 如图2所示, 两者阈值电压和电流大小数据匹配较好, 说明本文仿真结果具有较好的准确性和可靠性, 校正后的主要器件物理参数列于表1.
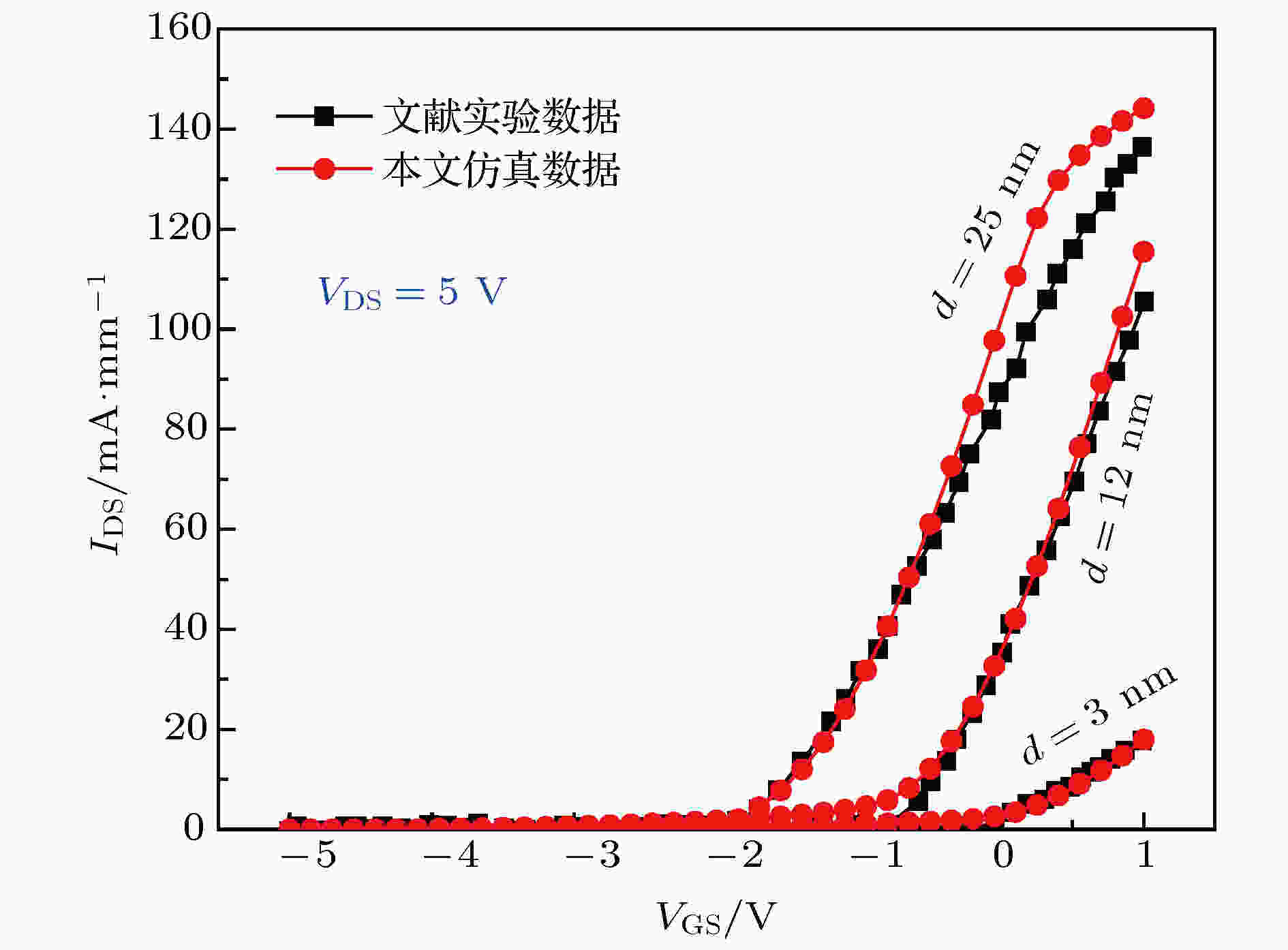
Figure2. Comparisons of simulated IDS-VGS characteristics of the Hall sensor with the experimental data.
物理参数 | 单位 | GaN | AlN |
禁带宽度 Eg | eV | 3.4 | 6.2 |
电子亲和能χ | V | 3.4 | 1.9 |
相对介电常数$\epsilon $ | — | 9.4 | 8.8 |
迁移率 μ | cm2/(V·s) | 1310 | 300 |
电子饱和速率 vsat | cm/s | 1.8 × 107 | 1.3 × 107 |
电子发射截面 σ0n | cm2 | 1.0 × 10–15 | 1.0 × 10–15 |
导带状态密度 Nc | cm–3 | 2.7 × 1018 | 4.1 × 1018 |
价带状态密度 Nv | cm–3 | 2.5 × 1019 | 2.8 × 1020 |
热导率 κ | W/(cm·K) | 1.3 | 2.9 |
表1仿真中所用的典型器件物理参数
Table1.Summary of physical parameters adopted in the simulations.
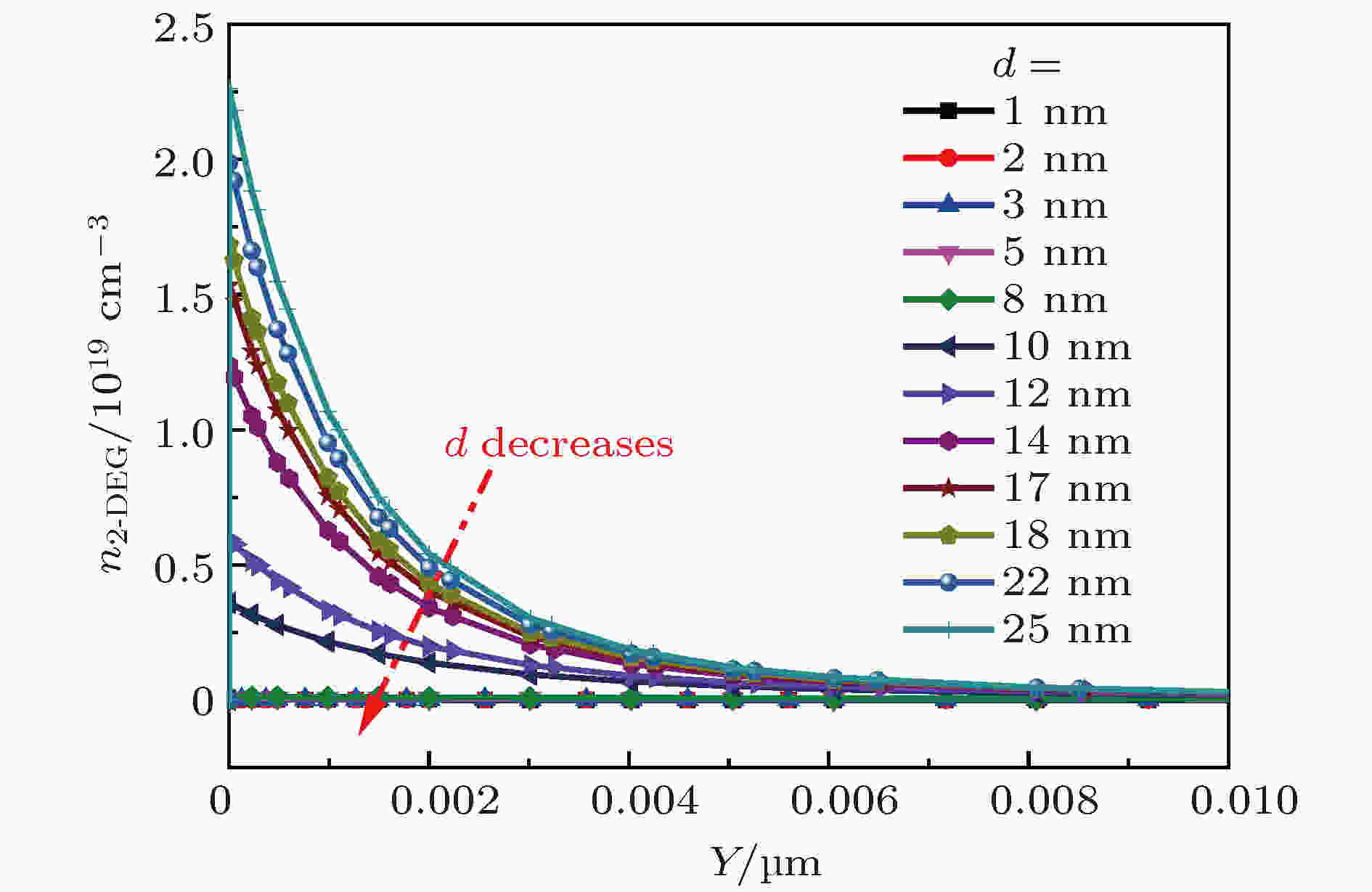
Figure3. Profiles of 2-DEG concentration vs. AlGaN barrier thickness.
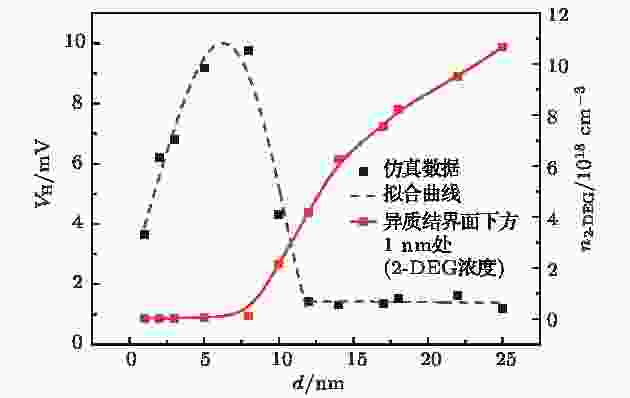
Figure4. Hall voltage (or 2-DEG concentration) vs. AlGaN barrier thickness.
图5展示了感测电极下方势垒层剩余厚度d = 7 nm时, 在无外加磁场和外加磁场B = 1 T情况下, 传感器电流密度空间分布对比情况. 从图5可以看出, 当器件表面平行方向不存在磁场时, 两侧的感测电极下方电流密度呈空间对称分布, 因此感测电极S1和S2之间电势差为零, 如图5(a)所示; 而当垂直纸面向里方向存在磁场时, 感测电极S1下方的电子在磁场中受到洛伦兹力作用发生向下偏移, 而S2下方的电子运动向上偏移, 两边感测电极处纵向束缚电场的减弱间接加速了电子在纵向的偏移过程, 稳定后感测电极S1和S2之间将产生明显的电势差, 如图5(b)所示, 图中虚线清晰地标明两侧电流空间分布差值情况.
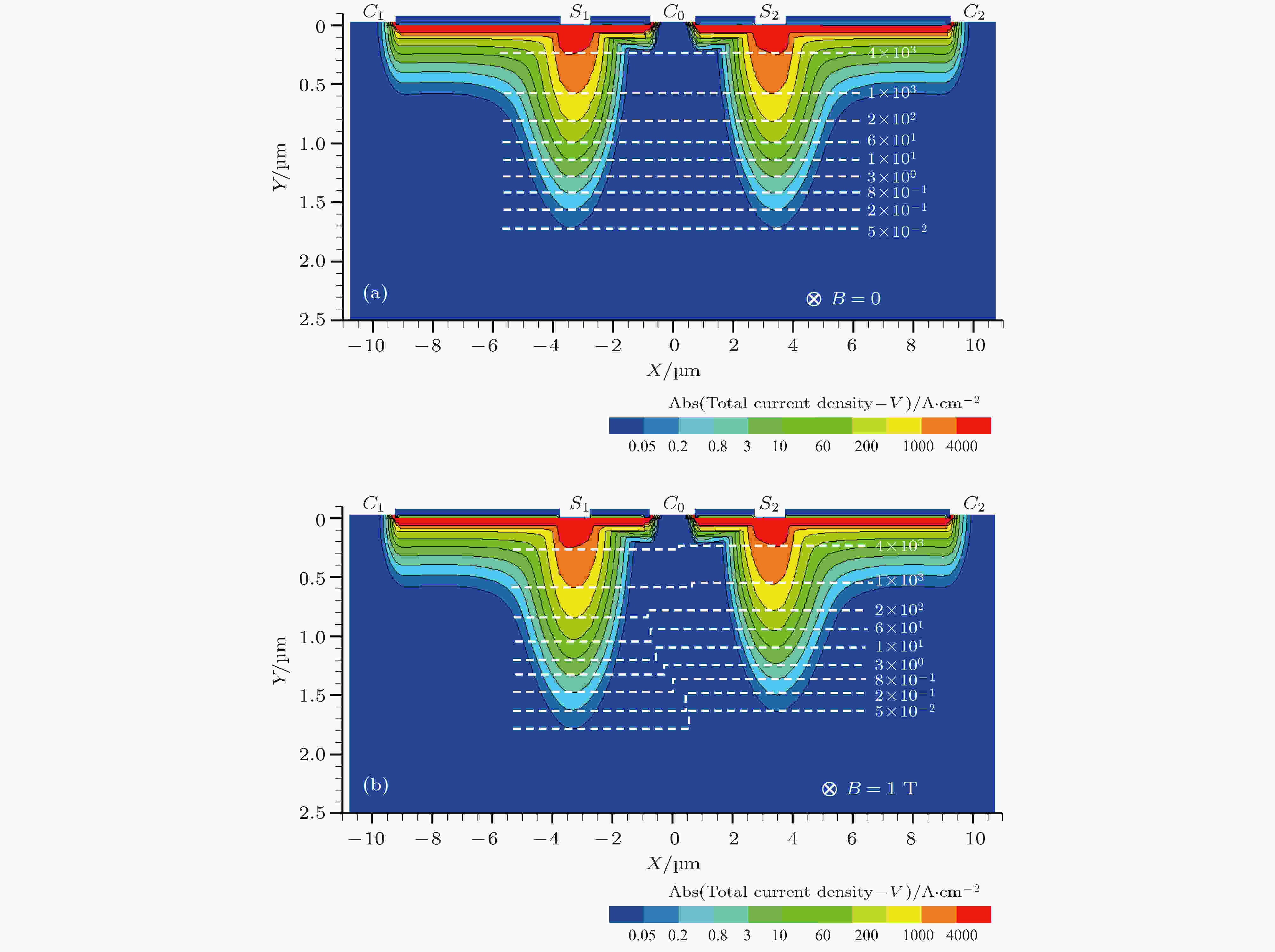
Figure5. Comparisons of current density distribution in vertical Hall sensor with d = 7 nm under the conditions of (a) B = 0 and (b) B = 1 T.
霍尔传感器中的结构尺寸, 特别是L2/L1比值、感测电极长度l2和器件台面宽度w等参数对传感器灵敏度影响较大, 本文在仿真过程重点对这些参数进行优化, 仿真中采用的激励电流为0.5 mA, 外加磁场强度B = 0.5 T.
图6展示了霍尔传感器电流相关敏感度SI与L2/L1比值的关系, 图中数据点为经过对数十个不同尺寸器件仿真模拟并经过计算得到. 仿真中设置感测电极的长度l2 = 1 μm, 器件的宽度w = 10 μm. 图6中虚线是对仿真所得数据点分布进行线性拟合得出的, 其数学关系式已列在图中. 从仿真结果可以看出, 电流相关敏感度随着L2/L1比值的增加而增加. 其原因是: 随着L2/L1比值增加, 感测电极相对两侧主电极的位置发生改变, 当感测电极靠近器件两侧时, 器件的几何因子G增加[21,26], 由前面表达式(2)可知电流相关敏感度也随之增加; 另外, 随着L2/L1比值增加, 靠近两侧主电极处的载流子受洛伦兹力的影响增强, 感测电极之间的电势差逐渐增加, 因此霍尔电压增加, 器件的电流相关敏感度也随之增加. 因此在实际器件设计制作过程中, 为了增大传感器的灵敏度, 可以适当减小主电极C0与C1(或C2)之间的间距L1, 或者适当增加C0与S1 (或S2)之间的间距L2.
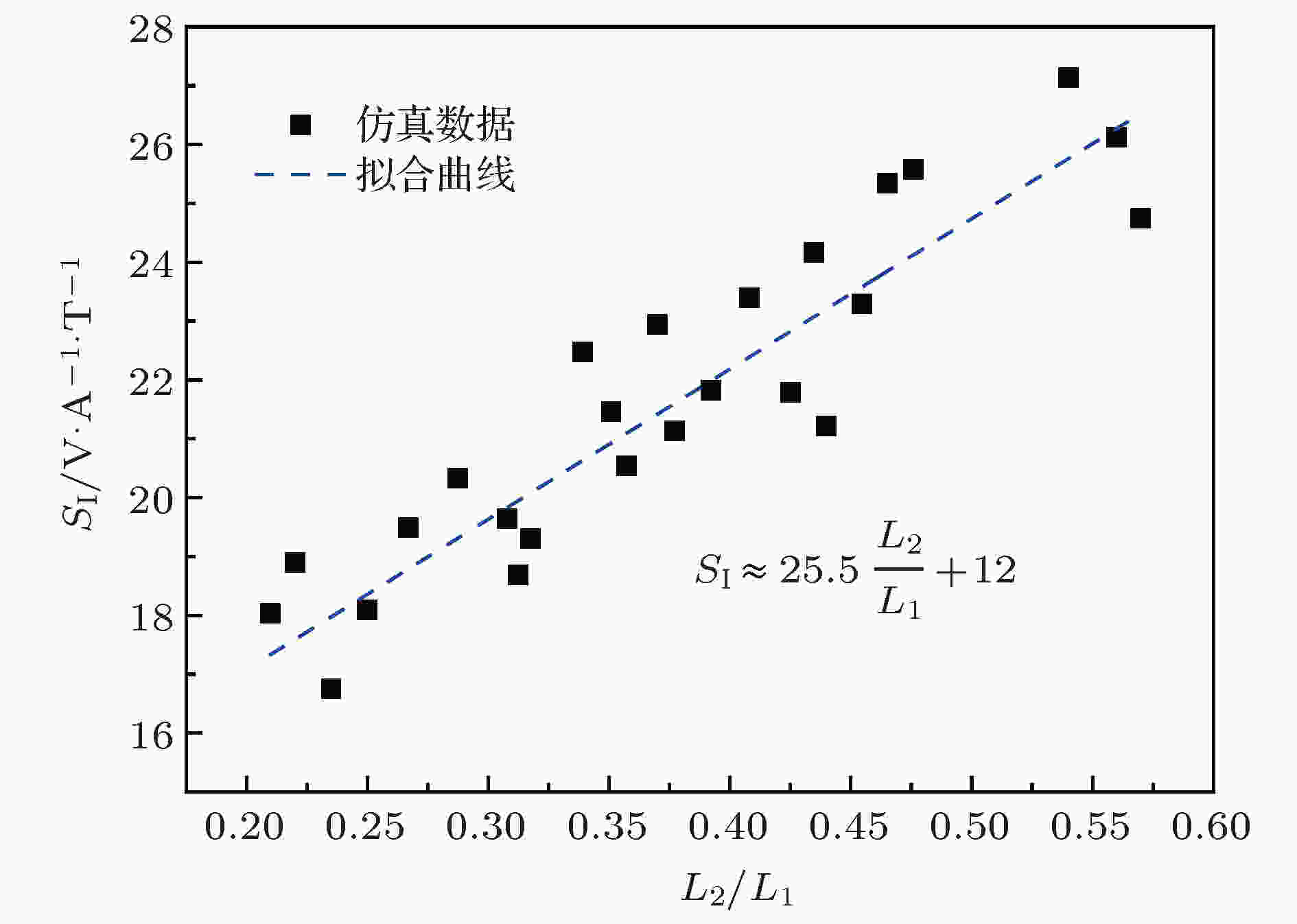
Figure6. Current-related sensitivity as a function of the ratio of L2/L1.
图7展示了电流敏感度以及输入电阻仿真结果, 与感测电极长度的关系, 其中感测电极l2的长度变化范围为0.50—2 μm, 而主电极间距L1 = 4.05 μm, 感测电极与主电极间距L2 = 2 μm以及器件宽度w = 10 μm. 由仿真结果可知, 器件的敏感度随着感测电极长度的增加有所增加, 当感测电极长度为1.25 μm时, 器件的峰值电流相关敏感度为22.8 V/(A·T). 随着感测电极长度继续增加, 器件的输入电阻呈线性增加, 而敏感度逐渐下降. 其原因是: 器件的电流相关敏感度的大小与器件导电沟道中的载流子浓度成反比, 随着感测电极长度的增加, 其下方势垒层经过刻蚀后, 异质结界面处的2-DEG浓度平均值有所降低[31], 因此电流敏感度逐渐增加; 而当感测电极的长度过大时, 两侧有效电势差部分抵消, 因此霍尔电压开始下降[25]. 综合考虑, 本文选取其最优值为l2 = 1.25 μm.
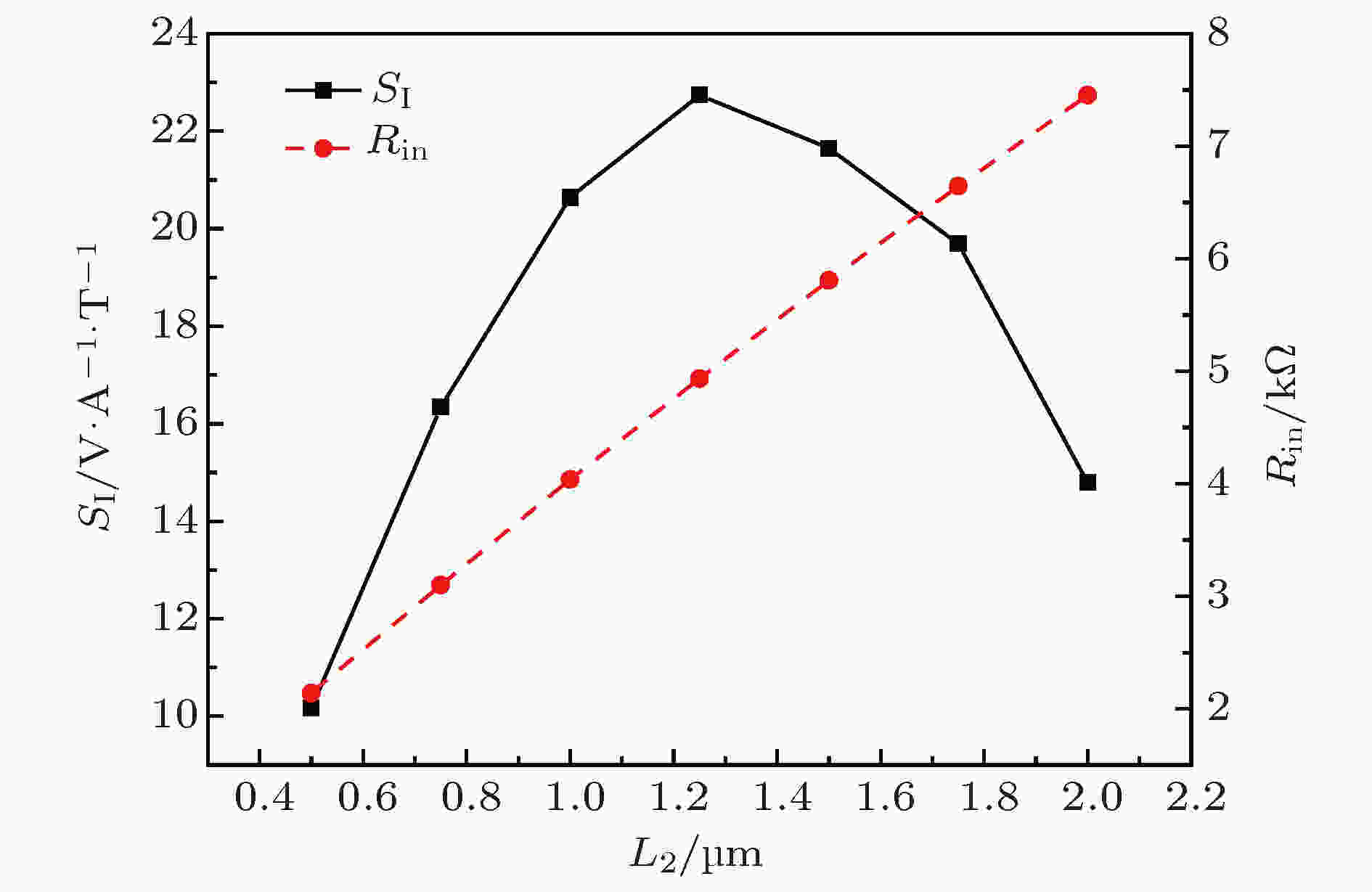
Figure7. Current-related sensitivity and input resistance as a function of the l2.
接下来进一步优化霍尔传感器台面宽度w, 仿真中w值分别设置为2, 4, 6, 8, 10 μm. 器件的其他参数如下: 主电极间距L1 = 4.05 μm, 感测电极与主电极间距L2 = 2 μm, 感测电极长度l2 = 1.25 μm. 仿真结果如图8所示, 随着器件台面宽度的缩减, 电流敏感度和输入电阻逐渐上升, 其变化规律符合(2)式. 器件的霍尔电压VH及电流相关敏感度SI与器件的宽度w成反比关系, 即w越大, 器件的霍尔电压及电流相关敏感度均降低. 因此理论上器件宽度w应当减小[21], 但是电流敏感度的提高将以牺牲器件输入电阻为代价. 此外, 考虑实验室中利用常规光刻技术制作传感器, 线宽越小曝光和剥离过程难度越大, 成品率更低, 因此在实际器件设计中应当折衷考虑, 同时结合电路匹配情况进行选择.
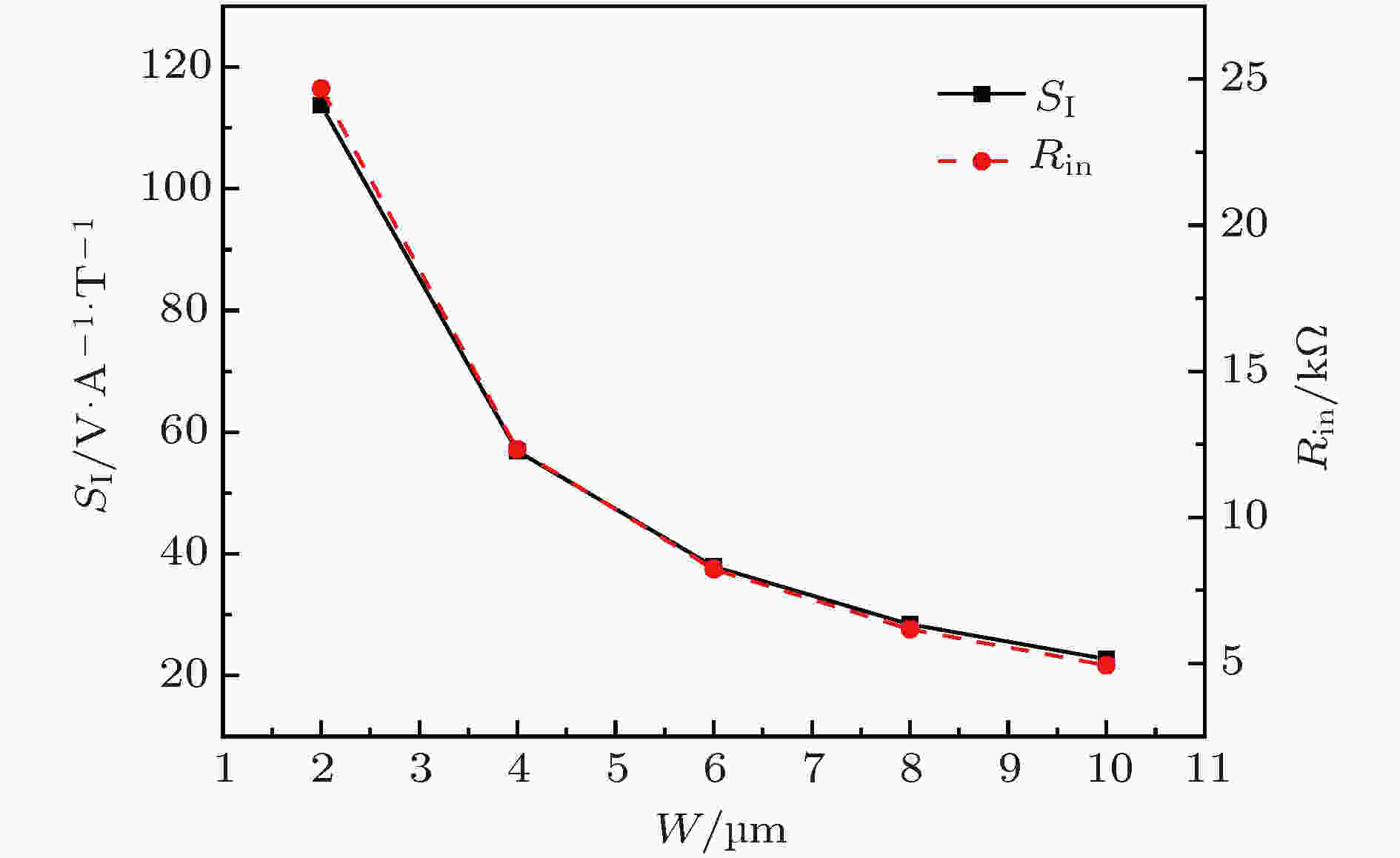
Figure8. Current-related sensitivity and input resistance as a function of the w.
图9展示了不同工作温度下, GaN材料垂直型霍尔传感器的霍尔电压随外加磁场强度的变化关系. 仿真中, 选取主电极间距L1 = 4.05 μm、感测电极的长度l2 = 1.25 μm、感测电极与中心主电极的距离L2 = 2 μm以及器件的宽度w = 3 μm. 传感器工作中激励电流选取为0.3 mA, 外加磁场强度变化范围为–0.5 T ≤ B ≤ 0.5 T, 温度变化范围为300—525 K. 从图9可以看出, 无论正反磁场条件下或者不同环境温度下, 输出霍尔电压与磁场大小均呈现良好的线性关系. 进一步利用(2)和(3)式可以计算得到电流相关敏感度和温漂系数. 图10展示了传感器敏感度随工作温度的变化关系, 其中虚线由线性回归拟合得到, 图中列出了利用该线性关系计算得到的温漂系数. 从仿真结果可知, 室温下(T = 300 K)器件的电流敏感度为75.7 V/(A·T), 在300—375 K温度范围内器件的温度漂移系数为637.4 ppm/K, 在375—525 K温度范围内器件的温度漂移系数为–613.0 ppm/K, 图10电流敏感度变化趋势反映真实传感器工作过程中的物理规律[32]. 其原因是, 材料迁移率主要受电离杂质散射和晶格散射这两种机制的影响. 当传感器处于相对较低工作温度时, 载流子迁移率主要受电离杂质散射影响, 随着温度上升, 该散射影响减弱, 因此电流敏感度增加; 而当传感器处于高温工作情况时, 载流子迁移率主要受晶格振动散射影响, 以声学散射为主且晶格散射随着温度的升高而增强, 因此电流敏感度开始下降. 但本文宽带隙GaN材料霍尔传感器相对其他窄带隙材料, 晶格散射对其敏感度影响明显更小.
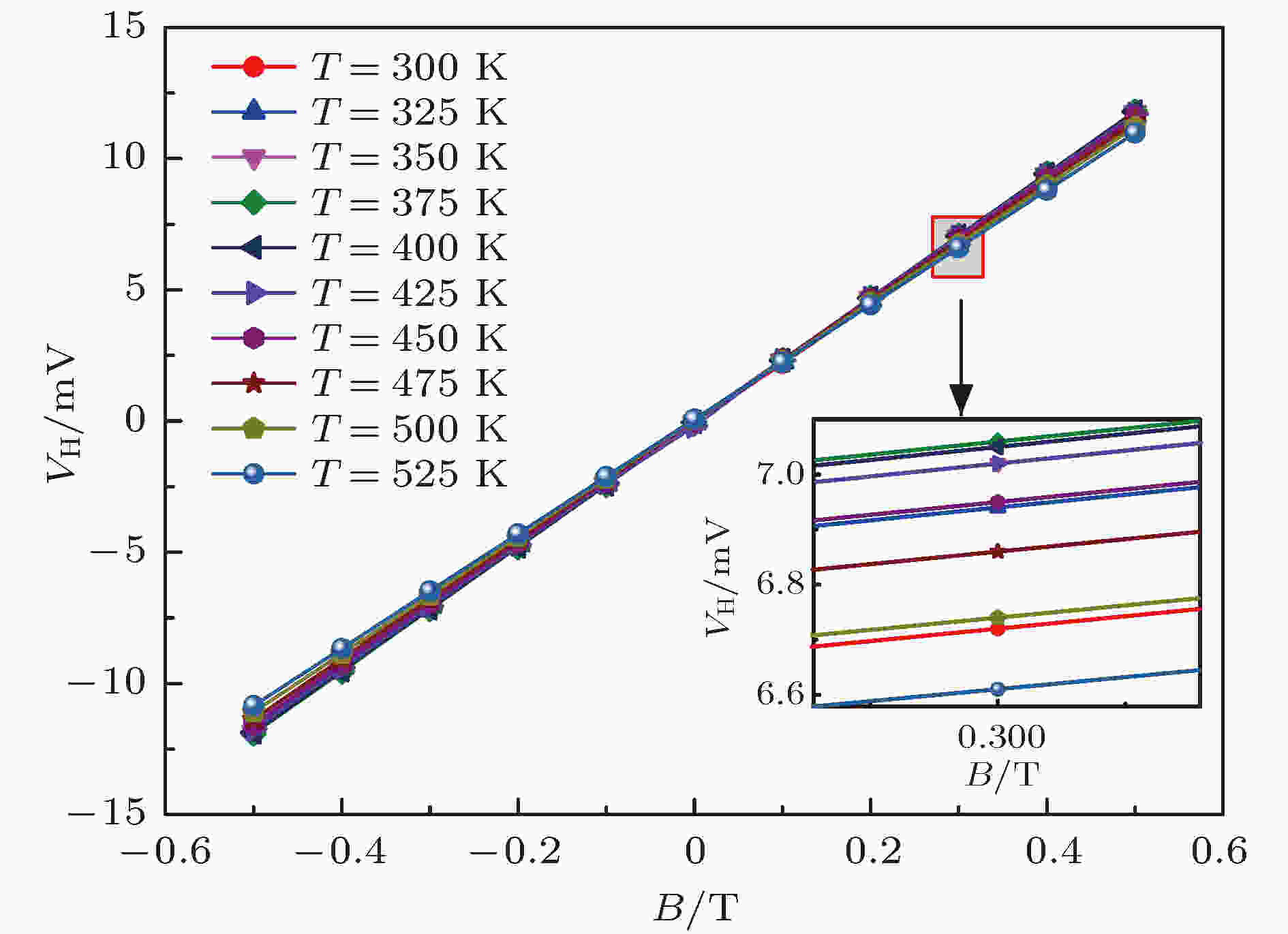
Figure9. Temperature dependence of output Hall voltage as a function of magnetic induction.
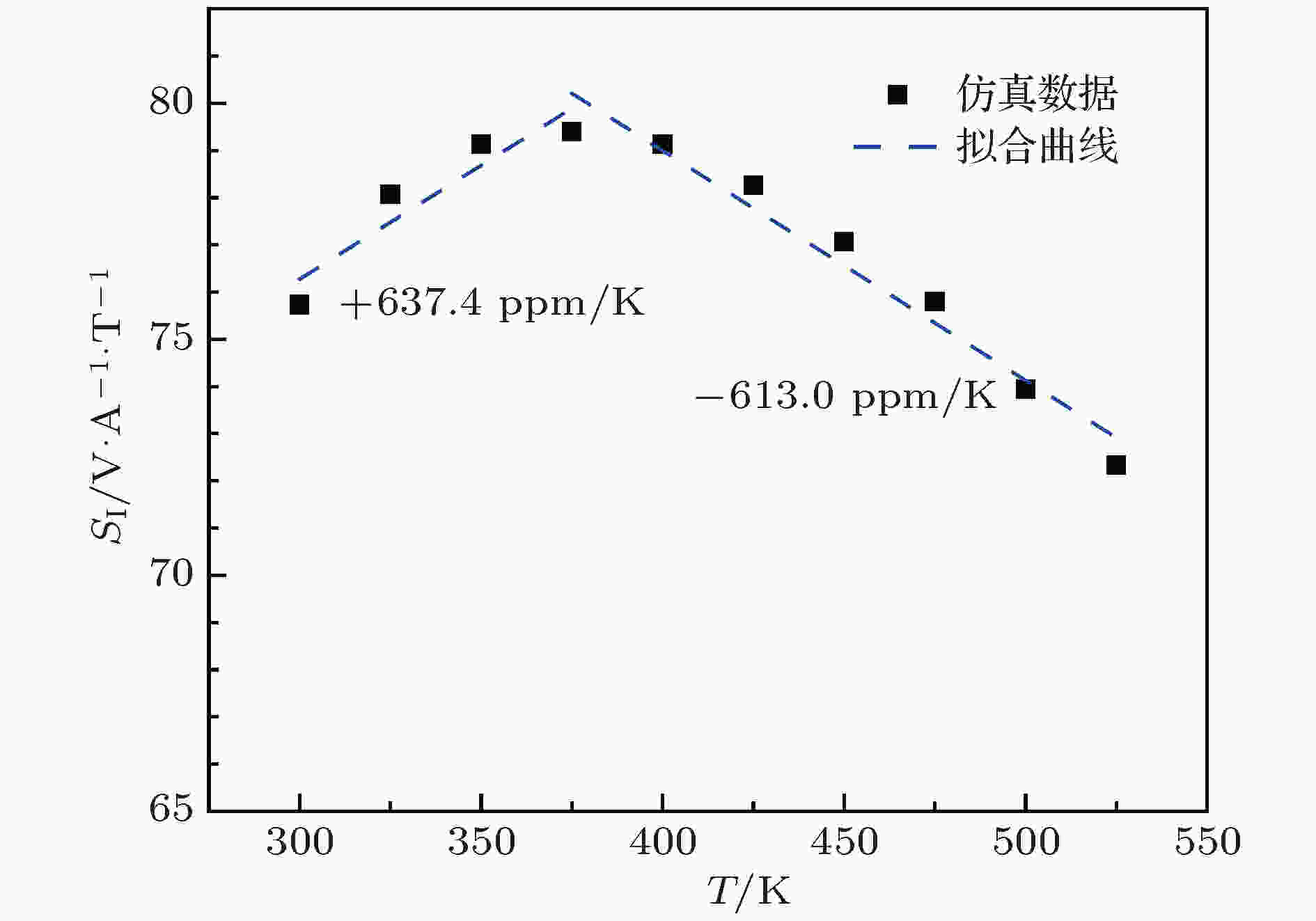
Figure10. Current-related sensitivity as a function of temperature.
本文工作与目前具有代表性的、能代表目前霍尔传感器发展水平的器件类型进行对比, 表2列出了不同材料和结构类型的半导体霍尔传感器关键性能指标对比结果[7,19,33,34]. 从表2可以看出, 传统Si基窄禁带半导体霍尔传感器受制于载流子迁移率较低的影响, 其电流相关灵敏度一般较小. 而由于材料禁带宽度小, 其器件温漂系数都在1000 ppm/K以上, 且一般只能工作在室温环境. InAs材料为主的窄禁带半导体虽然电流相关灵敏度较大, 但其温漂系数同样很大, 工作温度往往小于400 K或者只能在特定低温条件下工作. 而现有报道的GaN基霍尔传感器能工作在大于400 K的高温环境, 但其主要是水平型, 垂直型结构由于设计和研制工艺难度都较大, 目前其实验数据或者仿真结果都还未见报道. 即使是水平型器件, 其感测磁场的电流敏感度实验数据还普遍较低. 与上述这些器件相比, 本文所设计的GaN基霍尔传感器为垂直型结构, 填补了宽禁带材料垂直型器件研究的空白, 为实现同一芯片三维磁场探测奠定了理论和技术支持. 所设计的传感器能稳定工作在大于500 K的高温环境, 经过器件结构仿真优化后, 得了较大的磁场感测灵敏度和较低的温漂系数, 因此该类型器件将具有良好的工作稳定性和可靠性.
器件类别 | 工作温度/K | 温漂系数ST/ppm·K–1 | 灵敏度SI/V·(A·T)–1 |
Si基垂直型[33] | T < 350 | $\sim $1000 | 41 (x方向) |
Si基垂直型[34] | T < 350 | 4545 | 77.5 (x方向) |
Si基垂直型[35] | T < 350 | 1500 | N/A |
InAs/AlGaSb水平型[7] | T < 400 | 1710 | 250 |
InAs/AlGaSb水平型[7] | T < RT | 2690 | 302 |
AlGaN/GaN水平型[19] | T > 400 | $\sim $1000 | 113 |
AlGaN/GaN水平型[36] | T > 400 | 820 | 46 |
AlGaN/GaN垂直型(本文) | T > 500 | $\sim $600 | 75.7 (w = 3 μm) |
113.7 (w = 2 μm) |
表2基于不同材料的霍尔传感器关键性能指标对比
Table2.Comparisons of key performances of Hall sensors based on various materials.