全文HTML
--> --> -->最近, 许多实验和理论研究均发现镧系离子在裁剪TiO2的能带结构和增强TiO2的光催化活性方面具有特殊的作用. 如, Mazierski等[11]利用电化学方法制备了一系列的Er-, Yb-, Ho-, Gd-, Pr-掺杂的TiO2纳米管, 发现这些镧系离子掺杂均能增强TiO2的光催化活性. 在可见光解降甲苯的过程中, Ho掺杂TiO2的效率尤为显著; 进一步的理论计算表明, 能带中新出现的Ho-f能级和表面缺陷态可能是TiO2可见光催化能力增强的主要原因. Du等[12]报道Ce掺杂TiO2体系不但展现出优异的可见光吸收活性, 而且显示出良好的热稳定性. Nasir等[13]认为这种光催化活性增强的原因之一在于TiO2中形成了氧化还原电荷对(即Ce3+/Ce4+, 其中Ce3+具有4f15d0电子构型, 而Ce4+具有4f05d0电子构型), 因而在光生载流子的过程中Ce3+/Ce4+充当了“清道夫”的作用, 有效促进了电子-空穴对的分离, 提高了TiO2的光催化活性. Li等[14]发现, 当TiO2表面的Ce/Ti原子的比率为2.0%时, 在可见光照射下Ce-TiO2显示出优良的光催化活性. Xiao等[15]指出随着Ce掺杂浓度的提高, TiO2纳米纤维的光吸收边从450.0 nm大幅红移至739.0 nm, 当Ce/Ti的摩尔比增加到0.03后, 其可见光的吸收能力又逐渐变小. 而Fu等[16]通过第一性原理计算则发现, 随着Ce掺杂浓度的增加, Ti 3d轨道电子在导带底的态密度不断减弱, 从而使TiO2在180.0—600.0 nm之间的光吸收强度逐渐降低. 可见, 有关Ce掺杂TiO2的光吸收性能的理论研究与实验研究之间仍存在一定的差距. 这些差距的形成与多方面原因密切相关. 例如, 实验制备的掺杂体系, 不可避免地会产生一些缺陷(如氧空位(OV)、间隙掺杂、异种原子置换掺杂等缺陷), 而理论计算中常常却忽略了某些缺陷, 因而计算结果与实验观测之间有一定的差距. 对于金属氧化物而言, OV是一种常见的缺陷, 对金属氧化物的电子结构和物化性能可产生重要影响[17]. 最近, 占昌朝等[18]报道La3+置换TiO2中的Ti4+后形成了OV, 从而引起刃型位错, 他们认为这些位错缺陷为光催化反应活性位点提供了可能, 十分有利于增强TiO2的光催化活性. 再如, 最近Yang等[19]研究发现: 当氢原子占据OV, 形成新型的OVH缺陷时, 锐钛矿相TiO2的能带中出现了新的亚能级, 从而有效提高了TiO2的可见光吸收能力; 利用可见光辐射含OVH缺陷的TiO2光阳极, 可以把水完全裂解.
综上所述, 离子掺杂和空位缺陷均对氧化物半导体的能带结构、光吸收性能以及光催化活性具有重要的调控与影响作用. 鉴于Ce与OV共掺杂TiO2的理论和实验研究鲜有报道, 本文对Ce/OV共掺杂锐钛矿相TiO2的电子结构和光学性质进行理论计算和研究.
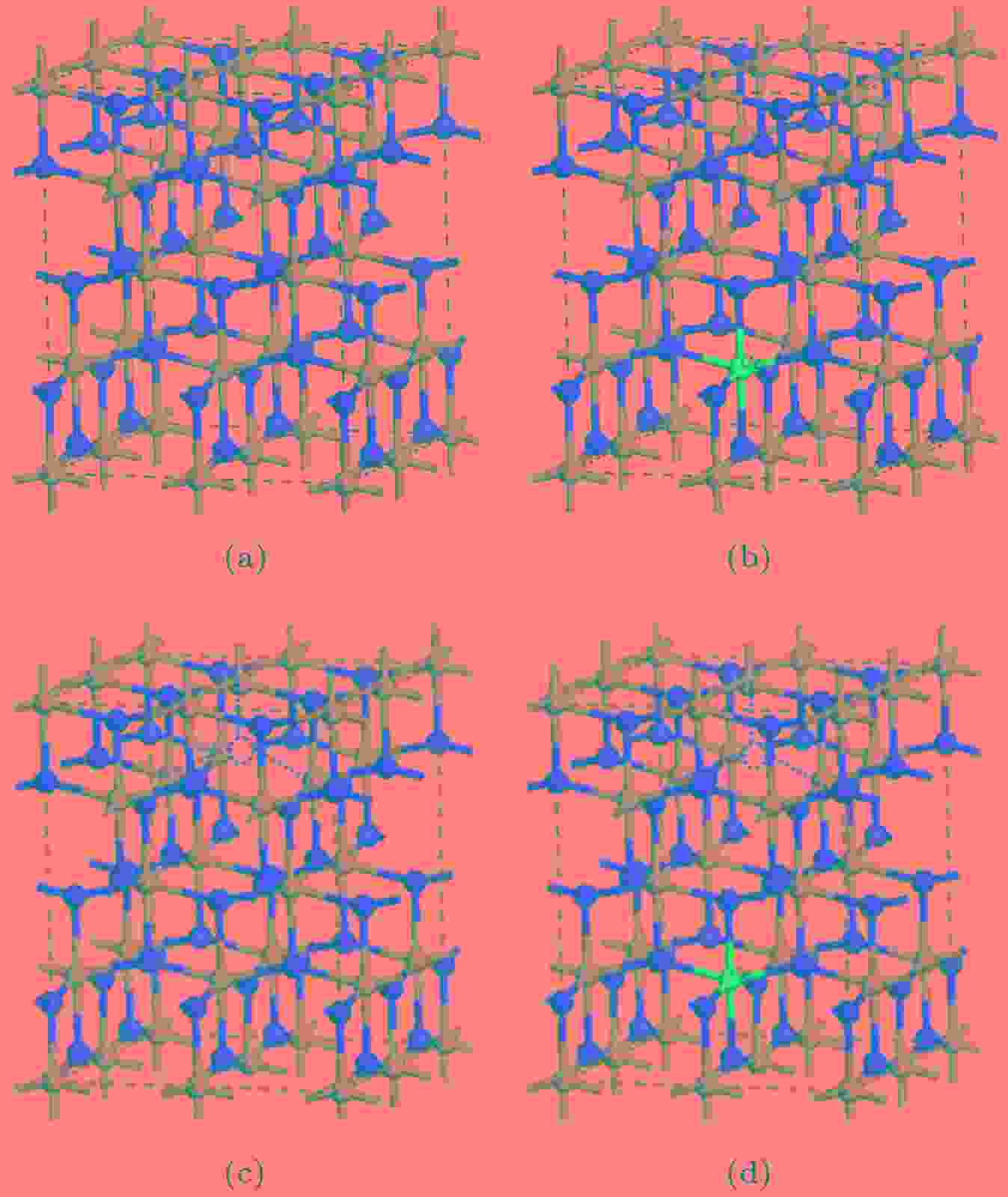
Figure1. The 2 × 2 × 1 supercell structure of various supercell models (the red, grey and blue balls represent O, Ti and Ce atoms, respectively; the imaginary red circle shows the position of oxygen vacancy): (a) Pure anatase TiO2; (b) Ce-TiO2; (c) OV-TiO2; (d) Ce/OV-TiO2.
本文采用Materials Studio软件中基于密度泛函理论(density functional theory, DFT)的平面波超软赝势法的CASTEP程序包进行计算. 考虑到计算体系含有强关联性的局域性Ti 3d和Ce 4f电子, 采用标准的DFT计算难以获得满意的电子结构和光学性质. 因此, 计算过程中交换关联泛函采用局域密度近似(local density approximation, LDA), 并对具有强关联作用的Ti 3d和Ce 4f电子用





















































计算中平面波截断动能设置为620 eV, 采取6 × 6 × 5的Monkhorst-Pack格点取样. 自洽场运算中应用Pulay密度混合法, 能量收敛标准为1.0 × 10?5 eV/atom (1 eV/atom = 9.6485 × 104 J/mol), 最大公差为0.0002 nm, 晶体内应力收敛标准为0.05 GPa, 每个原子间相互作用力小于0.2 eV/nm. 计算电子能带结构和光学性质之前, 计算所用的超胞结构先采用BFGS (Broyden-Flecher-Goldfarb-Shanno)方法进行几何优化, 然后再对优化后的模型结构进行单点能计算. 参与计算的价电子的构型分别为O: 2s22p4, Ti: 3s23p63d24s2, Ce: 4f15s25p65d16s2. 为保证计算结果的可比性, 各模型的计算均在倒易空间中进行, 其参数的设置均与上述设置相同.
为便于和实验测量结果比较, 光吸收性质计算中运用了非极化多晶模型, 并利用剪刀算符修正带隙值. 考虑到纯TiO2的实验带隙值为3.23 eV, 而本计算的值为3.12 eV, 两者间相差0.11 eV. 因此, 剪刀算符设为0.11 eV. 在能带带边的计算中亦使用0.11 eV对各体系的带隙宽度进行修正.
3.1.结构优化结果
表1为采用广义密度梯度近似(generalized gradient approximation, GGA), GGA + U, LDA及LDA + U方法, 对纯TiO2进行结构优化及能带结构计算得到的晶格参数和带隙值. 其中, 采用LDA + U计算得到的纯TiO2的晶格常数和内坐标参数(a, b, c, u) (u = dap/c)的计算误差均小于2.0%, 与实验和理论计算值[16,20,21,24,26-30]均吻合良好. 表2为采用LDA + U方法, 对Ce/OV单(共)掺杂TiO2的超晶胞结构几何优化后, 折合成单晶胞的结构参数.参量 | GGA | GGA + U | LDA | LDA + U | Experiment | Theory | Error% |
a/nm | 0.3791 | 0.3897 | 0.3745 | 0.3806 | 0.3785[26] | 0.3831[29] | 0.5548 |
b/nm | 0.3791 | 0.3897 | 0.3745 | 0.3806 | 0.3785[26] | 0.3831[29] | 0.5548 |
c/nm | 0.9805 | 0.9925 | 0.9447 | 0.9674 | 0.9514[26] | 0.9631[29] | 1.6817 |
c/a | 2.5860 | 2.5470 | 2.5230 | 2.5410 | 2.5140[26] | 2.5140[29] | 1.0739 |
u | 0.2050 | 0.2050 | 0.2080 | 0.2070 | — | 0.2050[29] | — |
dap/nm | 0.2011 | 0.2031 | 0.1966 | 0.2005 | 0.1980[27] | 0.1973[29] | 1.2626 |
deq/nm | 0.1946 | 0.2000 | 0.1914 | 0.1948 | 0.1934[28] | 0.1930[29] | 0.7238 |
Eg/eV | 2.18 | 3.18 | 2.16 | 3.12 | 3.32[24] | 3.23[29] | — |
注: dap, deq分别表示Ti—O长键和短键的键长; 误差指LDA + U方法相对于实验值的误差. |
表1采用GGA, GGA + U, LDA及LDA + U方法计算得到的纯锐钛矿相TiO2的晶格参数和带隙宽度
Table1.Lattice parameters and band gap width of pure anatase TiO2 calculated at the GGA, GGA + U, LDA and LDA + U levels of theory.
参量 | Ce-TiO2 | OV-TiO2 | Ce/OV-TiO2 | ||||
This work | Experiment | Other theory | This work | This work | |||
a/nm | 0.3822 | 0.3789[25] | 0.3980[31] | 0.3789 | 0.3827 | ||
b/nm | 0.3833 | — | 0.3980[31] | 0.3793 | 0.3798 | ||
c/nm | 0.9956 | 0.9509[25] | 0.9757[31] | 0.9824 | 1.0090 | ||
dap/nm | 0.2225 | — | 0.2273[32] | 0.2012 | 0.2233 | ||
deq/nm | 0.2183 | — | 0.2119[32] | 0.1946 | 0.2252 | ||
注: dap, deq分别表示Ce-TiO2, Ce/OV-TiO2中Ce—O长键和短键的键长, 对于OV-TiO2则表示Ti—O长键和短键的平均键长. |
表2Ce/OV单(共)掺杂锐钛矿相TiO2弛豫后的晶胞参数
Table2.Lattice parameters of Ce/OV single and co-doped anatase TiO2 after the structure relaxation.
表2数据表明, 掺杂体系的晶格参数均发生了不同程度的畸变. 由晶体场理论可知[11]: 晶格畸变可导致TiO2中八面体单元的正负电荷中心分离, 从而在晶体中形成局域内建电场. 由于产生了内建电场, TiO2中光生电子-空穴对的分离和迁移变得更加容易, 十分有利于增强TiO2的光催化活性. 这种内建电场的强弱, 可以用八面体单元的电偶极矩来度量. 表3列出了掺杂体系的平均电偶极矩的计算值.
Model | Ef/eV | q/e | p/Debye | ||||
Ti-rich | O-rich | O | Ti | Ce | |||
Pure TiO2 | — | — | ?0.720 | 1.440 | — | 0.0000 | |
Ce-TiO2 | 10.42 | 1.16 | ?0.719 | 1.405 | 1.920 | 0.0168 | |
OV-TiO2 | 1.02 | 5.65 | ?0.727 | 1.412 | — | 0.0668 | |
Ce/OV-TiO2 | ?15.84 | ?20.48 | ?0.725 | 1.373 | 1.920 | 0.0251 |
表3Ce/OV掺杂锐钛矿相TiO2的缺陷形成能(Ef)、平均净电荷(q)和平均偶极矩(p)
Table3.Defect formation energy, average net charges and average dipole moment of Ce/OV doped TiO2 supercell after the structure relaxation.
表3中平均偶极矩的计算结果表明: OV可显著提高TiO2中八面体结构的平均偶极矩; Ce掺杂TiO2中引入OV后, 八面体结构单元的平均电偶极矩的大小明显增加. 可见, 形成一定浓度的OV, 有利于增强TiO2的光催化活性. 后文中的电子能带结构、态密度和光吸收谱的计算结果均进一步印证了这一结论.
2
3.2.缺陷形成能
为评估掺杂原子嵌入TiO2晶格与空位形成的相对难易程度, 以及确定掺杂体系的稳定性, 这里采用缺陷形成能来表征[20,29]. 缺陷形成能(Ef)的计算公式如下:


在富氧条件下, 显然,












2
3.3.能带结构和态密度
基于DFT + U的计算方法, 对优化后的纯锐钛矿相TiO2和掺杂与缺陷体系的能带结构和态密度进行了计算. 为方便比较, 各体系的能带图均选取费米能级在能量零点来观察TiO2晶体沿布里渊区高对称方向的能带结构(如图2所示). TiO2掺杂前后各体系对应的总态密度(total density of states, TDOS)和分态密度(partial density of states, PDOS)图(如图3所示)中的费米能级也取为能量零点.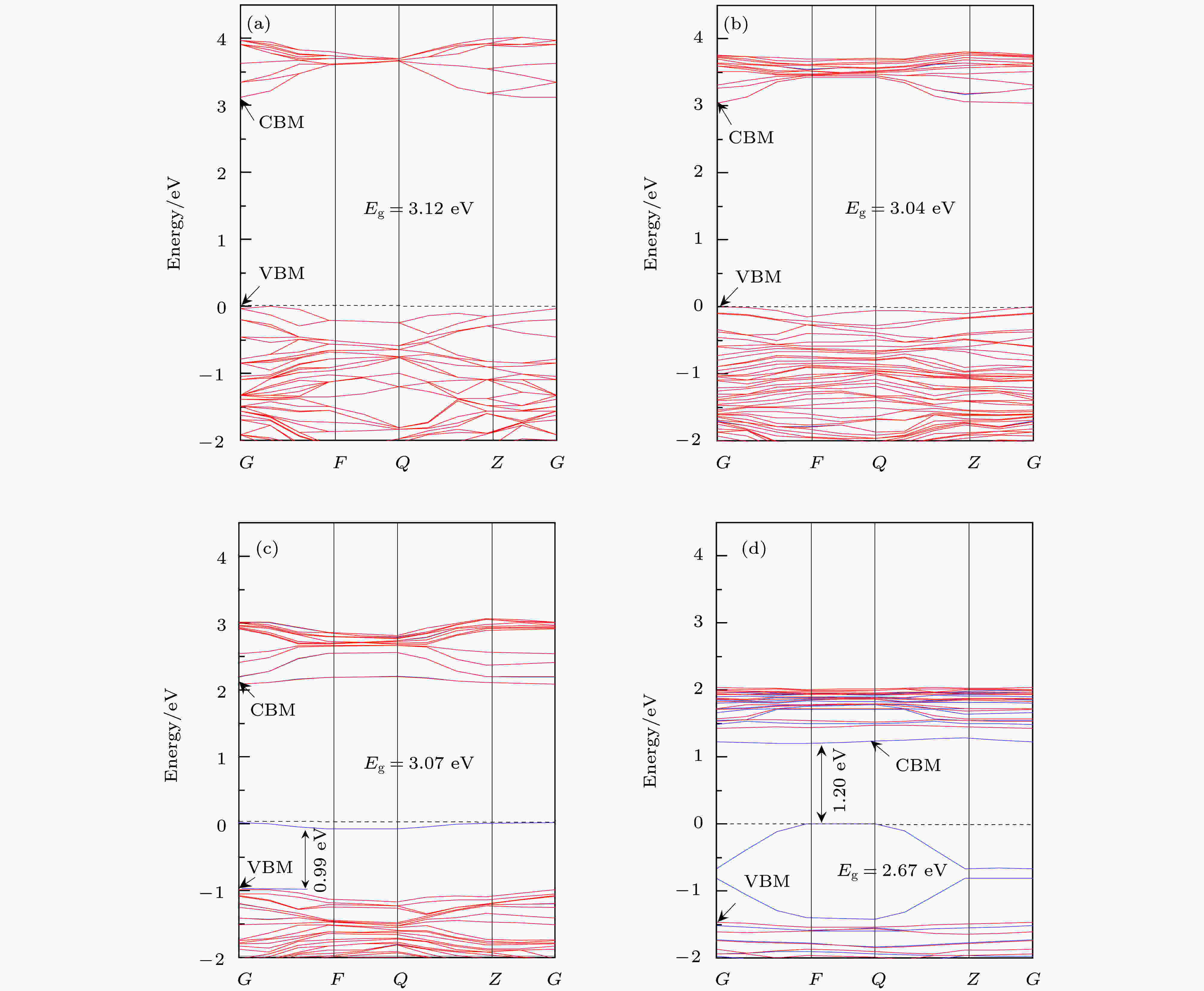
Figure2. Band structure of anatase TiO2 before and after doping (the dashed lines represent the Fermi level, EF): (a) Pure TiO2; (b) Ce-doped TiO2; (c) OV-TiO2; (d) Ce/OV-TiO2.
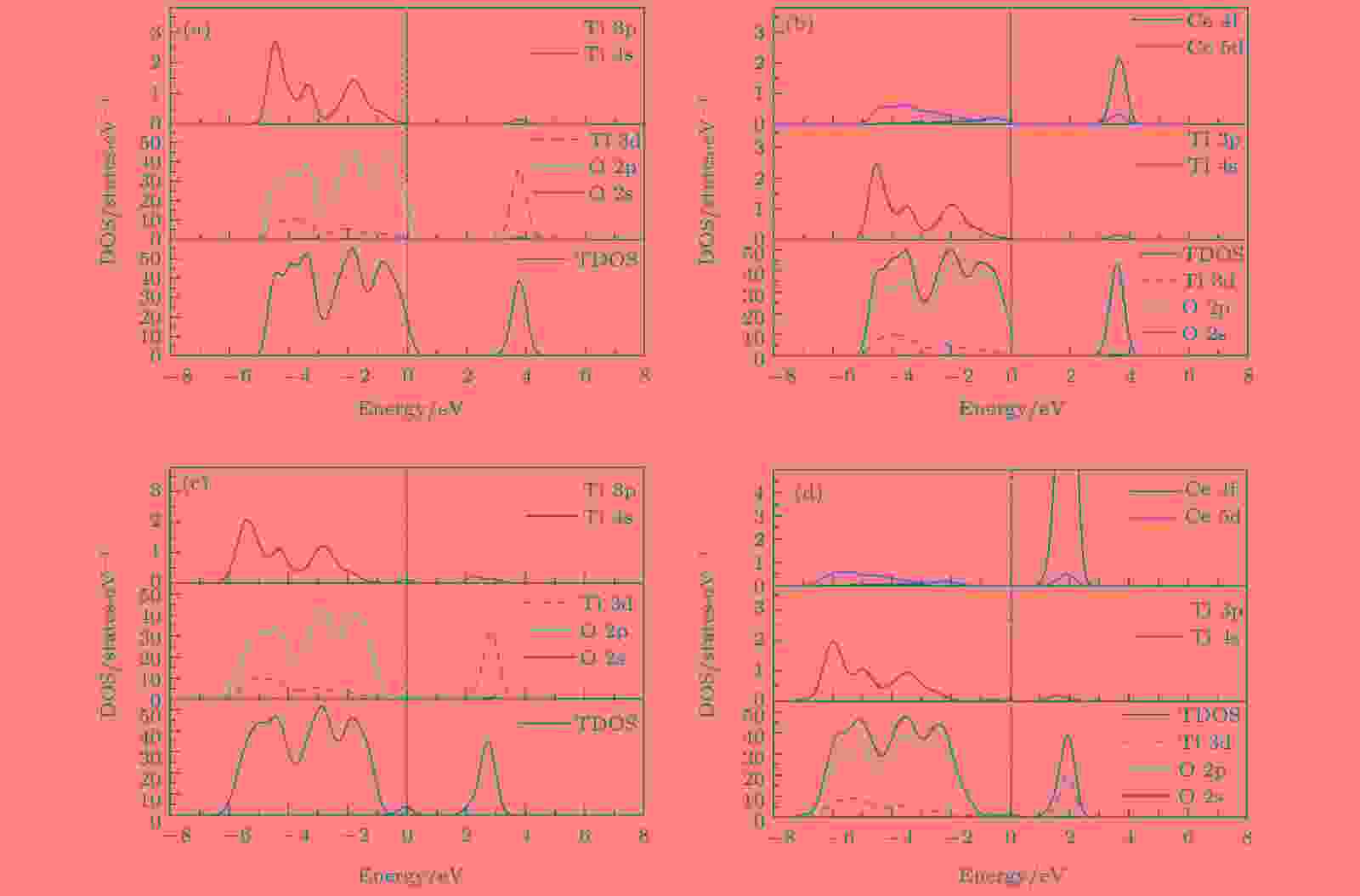
Figure3. Comparison of partial density of states of anatase TiO2 before and after doping (the dashed lines represent the Fermi level, EF): (a) Pure TiO2; (b) Ce-doped TiO2; (c) OV-TiO2; (d) Ce/OV-TiO2.
由图2(a)可知, 在高对称点G处纯锐钛矿相TiO2几乎为直接带隙, 其带隙宽度为3.12 eV, 与实验测量值3.23 eV十分接近. 对应的态密度图3(a)显示, 纯TiO2的价带(valence band, VB)和价带顶(valence band maximum, VBM)的态密度主要由O 2p轨道电子贡献, 其导带(conduction band, CB)和导带底(conduction band minimum, CBM)的态密度则主要由Ti 3d轨道电子贡献. 由此可知, 纯锐钛矿相TiO2的光吸收主要起源于电子在O 2p轨道与Ti 3d轨道之间的跃迁. 另一方面, 图3(a)表明纯TiO2的VB和CB中都含有O 2p和Ti 3d轨道电子, 这表明O原子与Ti原子之间具有部分共价键成分, 这一特性与文献[16, 33]的报道一致.
图2(b)为Ce单掺杂TiO2的能带图. 由图2(b)可知, 相对于纯TiO2而言, Ce-TiO2的VBM几乎没有移动, 而其CB整体朝低能方向发生了的移动, 从而导致Ce掺杂后TiO2的带隙宽度窄化为3.04 eV. 结合图3(b)可知, 这种变化的主要原因可归于CBM附近的Ce 4f, Ce 5d, Ti 3d及O 2p轨道之间充分混合, 致使反键峰的位置相对于未掺杂前反键峰的位置明显下移, 从而导致TiO2的CB下移、带隙收缩. 显然, 带隙窄化可引起半导体的光吸收谱发生红移. 与之相吻合是, 后文中计算所得的光吸收谱和相关理论和实验研究[16,25,34]中均观测到了这一红移现象.
如图2(c)所示, OV-TiO2的禁带宽度相对于纯TiO2发生了微小的收缩, 并且在费米能级附近出现了杂质能级. 态密度图3(c)显示, O 2p, Ti 3d, Ti 3p, Ti 4s轨道之间充分叠加耦合, 在费米能级附近形成成键峰, 从而导致OV-TiO2的费米能级下方出现了具有占据态特性的受主能级. 这一结果与Zhang等[35]采用GGA + U方法计算得到的结果完全一致. 图2(c)显示该杂质能级与VBM相距0.99 eV, 这一能量差值与Rumaiz等[36]在实验中观测的结果极其相近. 他们观测到含OV的TiO2带隙中的杂质能级比VBM高1.0 eV. 此外, 对比图3(c)与图3(a)容易发现OV-TiO2的价带宽度明显发生了宽化. 这预示着电子具有更强的离域性, 十分有利于增强载流子的移迁能力.
比较图2(d)与图2(b)可知, Ce/OV共掺杂TiO2的禁带宽度(2.67 eV)相对于Ce掺杂TiO2的禁带宽度(3.04 eV)显著窄化. 由此可见Ce掺杂TiO2中进一步引入OV, 可有效诱导Ce/OV-TiO2的带隙收缩. 态密度图3(d)显示Ce 4f和Ce 5d进入CBM, 并且与Ti 3d轨道充分混合, 致使反键峰所在位置明显向低能方向移动, 从而导致Ce/OV-TiO2的导带整体向低能方向大幅移动(如图3(b)所示); 同时, 在价带区Ce 5d, Ti 3p, Ti 4s与O 2p轨道电子之间的成键峰的位置均较单掺杂时的成键峰的位置向价带低端能移动, 从而引起TiO2的价带整体向低能明显移动. 因此, Ce与OV协同作用下可导致TiO2的带隙显著收缩. 另一方面, 能带图2(d)显示, Ce/OV-TiO2的禁带中形成了极浅的受主能级. 这些杂质能级处于费米能级下方(与CBM相距1.20 eV), 并且与VBM充分交叠, 有利于电子从VBM跃迁至CBM. 这一效果与直接减小带隙宽度相似, 极大地提高了TiO2对可见光的响应能力.
比较图3(d)与图3(b), 可观测到相对于Ce-TiO2的价带和导带而言, Ce/OV-TiO2的价带和导带区的TDOS均向价带低能端下移和延伸, 且峰形降低, 变得更为平滑和宽化. 这种价带宽化与Ce 5d, Ti 4s与O 2p轨道电子在价带低能区相互叠加引起电子的分布更为宽广密切相关; 类似地, 图3(c)中也观测到价带发生了宽化, 且其价带宽化幅度略小于Ce/OV-TiO2. 事实上, 这种价带宽化现象, 是掺杂致使晶体的对称性降低, 导致电子分布的非局域性增强而形成的. 此时, 态密度曲线的峰形降低、变得更为平滑和宽化[29,37]. 显然, OV-TiO2和Ce/OV-TiO2中电子的非局域性的增强, 对提高光生载流子的迁移能力和TiO2的光催化活性十分有益.
2
3.4.带边位置
为探索掺杂前后半导体的导带边和价带边的还原氧化能力的变化, 可采用如下公式[38]: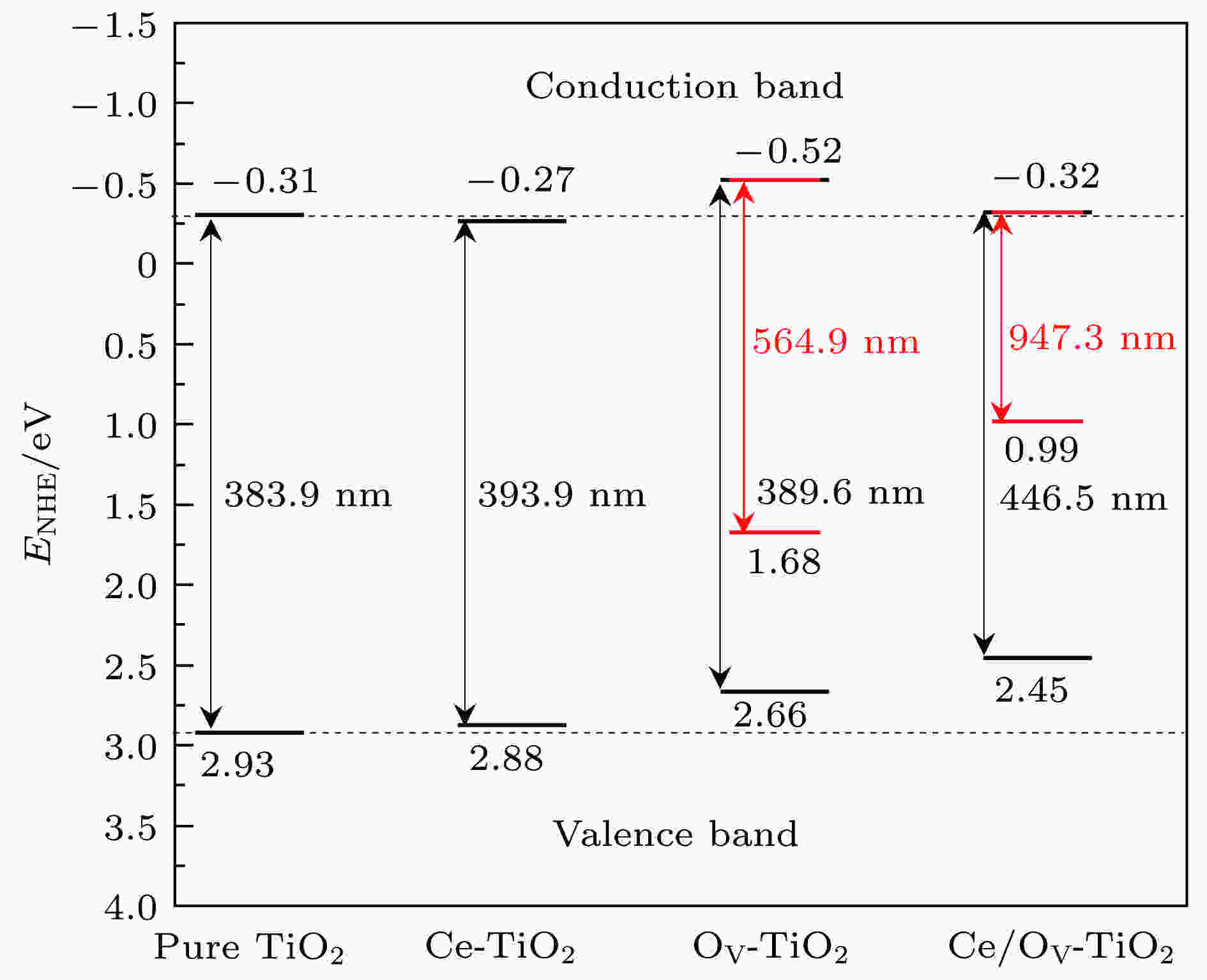
Figure4. Calculated band energy positions of pure TiO2, Ce-TiO2, OV-TiO2, Ce/OV-TiO2 (the energy scale is indicated by the normal hydrogen electrode in electron volts as a reference; the short thick lines and fine red lines represent the band energy positions of TiO2 by neglecting and considering the impurity levels, respectively).
如图4所示, 相对于纯TiO2的导带边和价带边的位置而言, Ce-TiO2 (Ce掺杂浓度为2.08%)对应的带边位置分别向下和向上移动了0.035和0.047 eV. Ce-TiO2的带边位置移动方向与Lenka等[25]报道的掺杂浓度为0.28%和3.0%的Ce/TiO2样品的带边位置的移动方向一致. 但此两种掺杂浓度的Ce/TiO2的带边移动幅度明显大于本研究中Ce-TiO2的带边移动幅度. 究其原因除了与掺杂浓度不同这个因素有关外, 更重要的在于实验制备中会形成CeO2, 因而可在TiO2的能带中产生新的能级, 对半导体的带边电势产生重要的影响. 显然, Ce-TiO2的价带边的氧化能力因其价带边上移而有所削弱, 但2.88 eV的价带边电势, 相对于氧化剂H2O2和O3的氧化电势1.77和2.07 eV而言, 可认为Ce-TiO2的价带边仍具有良好的氧化能力. 另一方面, Ce-TiO2的导带边的还原能力却因其导带边电势位置下移, 而略有减弱. 值得指出的是, OV-TiO2的导带带边显著向上移动, 这表明TiO2中引入OV可明显提高其导带边的光催化还原能力. Liu等[40]通过光致发光光谱(photoluminescence spectra, PL)检测到TiO2样品中形成了OV. 这些OV的存在可导致Ti4+向Ti3+转化, 有利于降低光生电子-空穴对的复合[13]. 类似地, 图4显示, Ce/OV共掺杂TiO2的导带边的电势位置略高于纯TiO2和Ce单掺杂的导带边的电势位置, 这说明其导带边的还原能力高于纯TiO2和Ce单掺杂的情形.
根据光解水的基本条件[41]: 光催化剂的导带边的电势应低于H+/H2的还原电势(0 eV vs. NHE); 另一方面, 光催化剂的价带边的电势应高于O2/H2O的氧化电势(1.23 eV vs NHE). 结合图2和图4可知, Ce/OV-TiO2的基本带隙明显窄化, 对可见光具有良好的响应能力, 且其带边电势可满足上述光解水的基本要求. 因此, Ce/OV-TiO2可能是一种良好的适合于可见光光解水的光催化材料.
另一方面, 图4显示Ce/OV单(共)掺杂TiO2的光吸收阈值相对于纯TiO2的光吸收阈值均向长波方向增长. 特别是, Ce/OV-TiO2的基本带隙的光吸收响应明显增长到可见光区域. 若考虑杂质能级的作用, OV-TiO2和Ce/OV-TiO2的光吸收能力分别拓展到可见光长波区域和红外光区.
2
3.5.光吸收谱
半导体的光学性质的计算基本原理是基于直接带间传输的介电函数的虚部公式[42]:


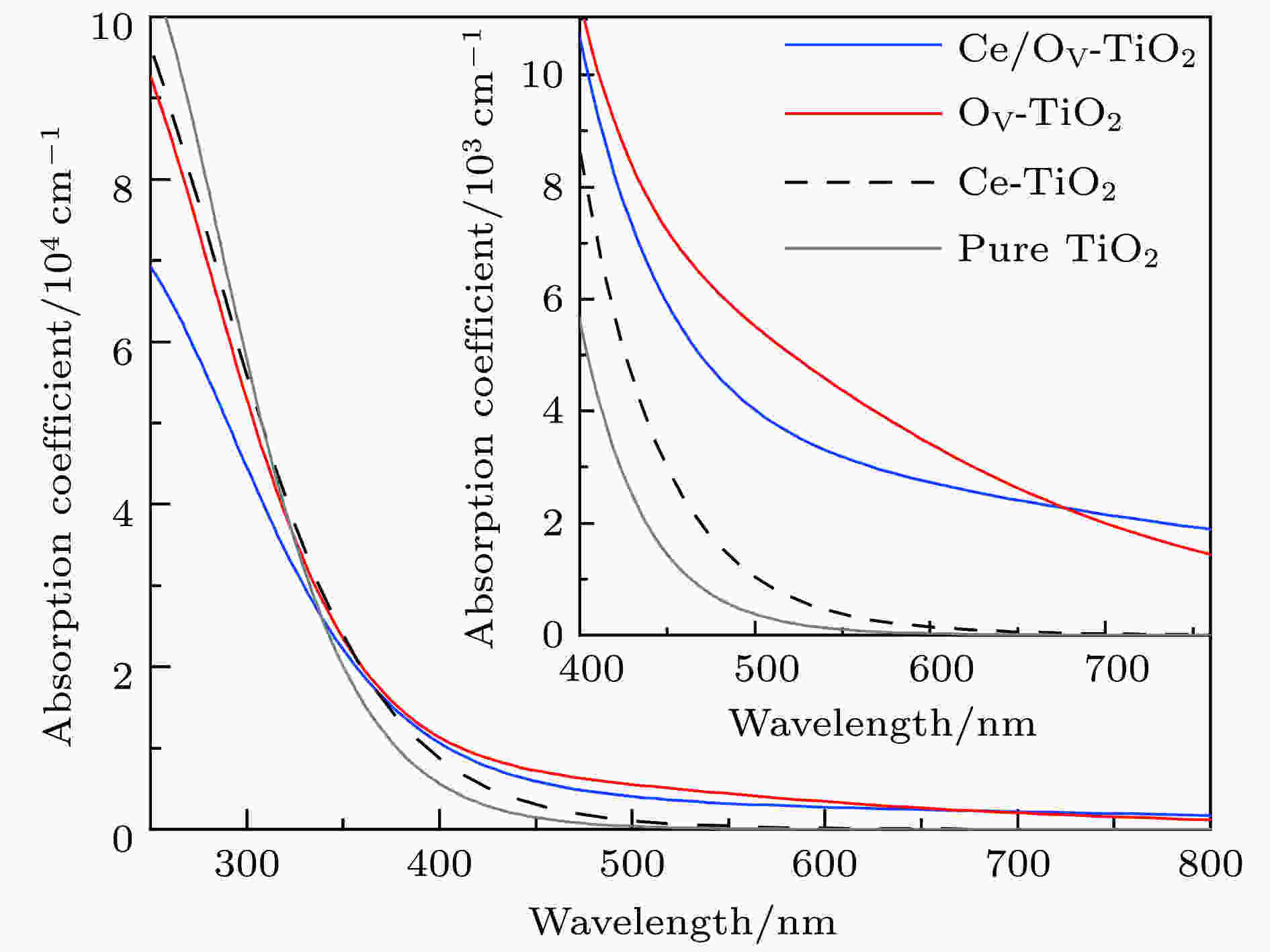
Figure5. Absorption spectra of pure and different doping TiO2 models (inset is the expanded absorption spectra in the visible region).
图5显示, 各掺杂体系的光吸收谱均发生了明显的红移, 且其在可见光区和更长波段区域的光吸收系数均高于纯锐钛矿相TiO2的光吸收系数. 其中, OV-TiO2和Ce/OV-TiO2在可见光区的吸收系数明显大于Ce-TiO2的可见光光吸收系数, 且前者在大部分的可见光区的光吸收系数均高于后者. 由此可见, Ce-TiO2中引入OV, 可有效增强TiO2对可见光的响应能力.
仔细比较Ce/OV-TiO2与OV-TiO2的光吸收谱, 可发现在400.0—677.1 nm之间, 前者比后者的光吸收系数略弱一些. 究其原因, 主要可归因于如下两个方面. 一是, 由图3(c)和图3(d)可发现, OV-TiO2与Ce/OV-TiO2在其费米能级附近的杂质能级上的电子态密度的大小存在较大差距, 前者明显高于后者. 这预示着, 相对于Ce/OV-TiO2而言, OV-TiO2中杂质能级上的电子吸收光能向导带跃迁的概率要大. 二是, 由图4可知, Ce/OV-TiO2在可见光区域的光吸收阈值约为446.5 nm, 而OV-TiO2由于杂质能级的作用, 其光吸收阈值约为564.9 nm. 这就是说, 前者对可见光的响应在一定区域内要弱于后者. 另一方面, 图5显示在波长大于677.1 nm的区域, Ce/OV-TiO2的光吸收系数略高于OV-TiO2的光吸收系数. 这一现象可以从图2(d)和图4得到解释. 图2(d)和图4显示, Ce/OV-TiO2的受主能级对更长波长的吸收响应能力显著强于OV-TiO2中的杂质能级对更长波长的吸收响应能力, 因而导致在更长波段中Ce/OV-TiO2的光吸收系数略高于OV-TiO2.
最近, 许多实验研究[25,34,44]报道, Ce掺杂TiO2可引起半导体的光吸收谱发生红移, 并显示出优良的光催化活性. 例如, Yan等[44]实验发现Ce掺杂浓度为1.0 mol%的Ce/TiO2的光吸收边的红移约为50.0 nm, 但掺杂浓度进一步增加时, TiO2的光吸收边的红移却不再增加; 而Lenka等[25]在实验中却观测到了Ce掺杂浓度增至10 mol%时, TiO2的光吸收边的红移约为81.0 nm. 相比于Yan等[44]和Lenka等[25]的实验结果, 本文研究的Ce/OV-TiO2的光吸收边的红移约为62.0 nm. 可见, 本研究的Ce/OV-TiO2的红移幅度与实验研究的Ce/TiO2的红移幅度相近. 这是因为实验制备的Ce掺杂TiO2不可避免地会产生一定浓度的OV, 本计算已考虑了这一因素, 因而计算结果与实验相近; 若计算中不考虑OV的作用, 本研究发现Ce-TiO2的红移幅度仅约为10.0 nm. 另一方面, 尽管本研究的Ce/OV-TiO2的红移幅度与实验结果相近, 但仍然存在明显差距. 究其原因有: 实验中TiO2样品的表面可形成CeO2, 因而OV和Ce的4f轨道在TiO2的能带中形成了新的能级, 从而促进了TiO2对可见光的响应能力. 此外, 实验制备中因Ce离子氧化不充分, Ce4+和Ce3+可以同时存在, 从而形成氧化还原电对Ce3+/Ce4+[25,44], 因而十分有利于提高光生电子-空穴对的迁移率和光量子产率[13]. 因此, 实验中观测到的Ce掺杂TiO2表现出了更优异的可见光光催化活性.
1) 在Ce/OV共掺杂TiO2的导带区Ce 4f, Ce 5d, Ti 3d轨道之间充分混合, 致使反键峰的位置和导带整体下移; 同时, 价带区的Ce 5d, Ti 3p, Ti 4s, Ti 3d, O 2p轨道电子之间交叠耦合, 引起成键态峰位朝低能方向移动. 最终, 共掺杂TiO2的价带明显宽化, 在费米能级附近形成受主能级, 带隙窄化为2.67 eV, 明显小于纯的和单掺杂TiO2的带隙宽度. 因此, Ce/OV共掺杂可有效提高TiO2对可见光的响应能力和增加其光吸收范围.
2) Ce/OV共掺杂使TiO2的晶格畸变, 并在其八面体单元中诱生出显著的电偶极矩, 从而可有效提高光生电子-空穴对的迁移能力和半导体的光催化活性; 另一方面, Ce掺杂TiO2中引入OV可增强导带边的还原能力, 共掺杂后TiO2的能带边的电势位置满足光解水的基本条件, 且在可见光区的光吸收强度明显高于纯TiO2和Ce单掺杂TiO2.
综上所述, Ce/OV共掺杂TiO2的能带结构可满足可见光光解水的基本要求. 本文的研究为相关理论和实验的进一步研究提供了良好的理论基础.