
The Measurement Methods and Principles of P700 Redox Kinetics
Chunyan Zhang
通讯作者: *E-mail:zhangchunyan@ibcas.ac.cn
责任编辑: 朱亚娜
收稿日期:2020-04-20接受日期:2020-08-26网络出版日期:2020-11-01
基金资助: |
Corresponding authors: *E-mail:zhangchunyan@ibcas.ac.cn
Received:2020-04-20Accepted:2020-08-26Online:2020-11-01

摘要
P700氧化还原动力学技术可快速且无损地检测植物光系统I (PSI)的活性, 是光合研究领域中广泛使用的一种技术。该文系统归纳了P700氧化还原动力学的主要测量方法, 详细阐述其原理并探讨该技术的局限性, 旨在为深入研究光合作用机理提供技术支持。
关键词:
Abstract
P700 redox technique is referred to examine plant photosystem I (PSI) function quickly and non-intrusively, and widely used in the field of photosynthesis research. In this paper, we summarize the main measurement methods of the P700 redox kinetics systematically, expound its principles, and discuss the limitations. The aim is to provide a technical support for in-depth study of photosynthesis mechanisms.
Keywords:
PDF (979KB)元数据多维度评价相关文章导出EndNote|Ris|Bibtex收藏本文
引用本文
张春艳. P700氧化还原动力学的测量方法及原理. 植物学报, 2020, 55(6): 740-748 doi:10.11983/CBB20064
Zhang Chunyan.
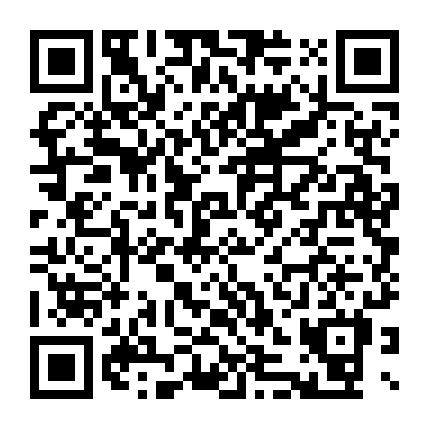
光合作用是地球上进行的最大规模的化学反应。放氧光合生物吸收太阳能并裂解水分子, 释放出地球上绝大多数生命活动所需的氧气, 同时固定大气或水中的CO2并合成有机物, 为新陈代谢提供能量。在高等植物和真核藻类中, 叶绿体是光合作用的场所。叶绿体的类囊体膜上排布着4个膜蛋白复合物: 光系统II (PSII)、细胞色素b6f (Cytb6f)、光系统I (PSI)和腺苷三磷酸合酶(ATP synthase)。它们紧密协作, 共同完成光能吸收、电子传递和能量转化, 最终合成腺苷三磷酸(ATP)和还原型烟酰胺腺嘌呤二核苷酸磷酸(辅酶II) (NADPH)。其中, PSI主要参与光合电子传递的最终步骤, 即氧化类囊体囊腔侧的质体蓝素(PC), 还原基质侧的铁氧还蛋白(Fd) (Brettel and Leibl, 2001)。PSI由核心复合物(即反应中心)及其周围的捕光天线复合物(light-harvesting complex I, LHCI)组成, 核心复合物负责电荷分离和电子传递, 而外周捕光天线复合物负责捕获和传递光能, 并进行光保护(郁飞等, 2001)。目前, 豌豆(Pisum sativum)和绿藻(假根羽藻(Bryopsis corticulans)) PSI-LHCI、红藻(Cyanidioschyzon merolae) PSI-LHCR、蓝细菌(Synechococcus elongatus) PSI三聚体以及蓝细菌(Synechococcus 7942) PSI-IsiA和PSI-IsiA-Fld超级复合物的晶体结构已得到解析, 为深入研究PSI捕获光能以及电子传递和光能转化奠定了结构基础(Jordan et al., 2001; Amunts et al., 2007; Amunts and Nelson, 2009; Qin et al., 2015, 2019; Pi et al., 2018; Cao et al., 2020)。此外, 人们对PSI组装的分子机制(Liu et al., 2012; Yang et al., 2015, 2017)以及LHCI的功能也进行了相关研究(Mozzo et al., 2010)。
PSI的反应中心有1个具有光化学反应性的叶绿素a分子二聚体, 即P700 (Kok, 1957)。P700在300-830 nm光谱范围内有广泛的吸收, 其中在430和700 nm处有吸收负峰, 最大吸收负峰在700 nm处, 而在800 nm左右有吸收正带(Ke, 1973)。此外, P700可被不同波段的光(又称活化光)激发, 转变为具有高能电子的激发态分子P700*, P700*射出电子后变成P700+, P700+从还原的PC捕获电子重新变成P700 (沈同等, 1991)。对于P700而言, 可见光范围内的光和720 nm的远红光都可作为活化光, 其中红光既可激发PSII, 使PSII反应中心进行电荷分离产生电子, 又可以使一部分P700将电子传递给PSI的电子受体, 从而变成P700+, 而远红光仅激发PSI。P700的氧化与还原不仅反映流经和围绕PSI的电子传递, 还反映PSI甚至PSII的功能。
P700的氧化与还原可通过700 nm (最大吸收负峰)的光吸收变化来检测, 但对叶片来说, 其光散射比较严重, 加之叶绿素荧光的干扰, 所以在700 nm处的光吸收非常复杂, 难以表征P700的氧化与还原(Schreiber et al., 1988)。Weis等(1987)发现, 用820 nm处的近红外光吸收变化可以很好地测量稳态光照下活体叶片P700的氧化状态, 而且820 nm处的光不会被PSII吸收, 因此也不会有叶绿素荧光的干扰。Schreiber等(1988)通过测量单一波长820 nm处的光吸收变化检测P700的氧化还原, 该方法适合叶绿体、单细胞藻类和活体叶片。但是离体叶绿体以及藻类悬浮液存在的光散射以及PC在800-900 nm范围内的光吸收等干扰因素都会影响P700的检测, 从而导致P700检测信号弱、信噪比低及信号漂移等问题。Klughammer和Schreiber (1998)利用双波长差示吸收技术检测P700的光吸收变化(810-860 nm), 很好地降低了由单一波长测量导致的影响。此外, Klughammer和Schreiber (1994)利用饱和脉冲技术检测P700在830 nm处的光吸收变化, 区分出了有活性的、具有开放受体侧的PSI反应中心以及具有还原受体侧、关闭的PSI反应中心, 从而估算PSI的光化学量子产量。Klughammer和Schreiber (2008)用饱和脉冲技术, 通过检测P700在近红外(830-875 nm)的差示吸收变化计算PSI的能量转化。
P700氧化还原动力学技术可实现快速、无损、原位和活体检测。目前, 检测P700氧化还原动力学的仪器主要有LED激发-探测光谱仪(JTS-10, Bio-Logic SAS, France)、调制叶绿素荧光仪(PAM-101, Walz, Germany)、双通道调制叶绿素荧光仪(DUAL-PAM- 100, Walz, Germany)和四通道动态LED阵列近红外光谱仪(DUAL-KLAS-NIR, Walz, Germany)等, 上述4种仪器检测的时间分辨率分别为10、10、30和35微秒。LED激发-探测光谱仪可分别检测P700在705- 730/740 nm和810-880 nm的光吸收变化, 活化光可选择绿光、橙光、红光或远红光等。该仪器信噪比高, 尤其适合测量较低浓度的藻类悬浮液样品, 且软件可校正飘移的基线、在线比较样品间P700氧还能力及氧还速率的差异等。其它3种设备由德国Walz公司研发, 可分别检测P700在810-870 nm、830-875 nm和820-870 nm的光吸收变化, 活化光均可选择蓝光、红光或远红光。这3种仪器非常适合测量高等植物叶片的P700氧化还原变化, 尤其是双通道调制叶绿素荧光仪和四通道动态LED阵列近红外光谱仪可将所需的光源进行整合, 不再需要更换滤光片和检测模块等配件, 且软件既可运行固定程序又可进行手动操作, 灵活性强。目前, 上述仪器常用的活化光光源是LED、氙灯或激光, 活化光的光强可根据需要灵活选择。下面介绍4种主要的P700氧化还原动力学的测量方法和原理。
1 活化光诱导的P700氧化还原动力学
活化光诱导的P700氧化还原动力学是将暗适应的藻类或高等植物进行光-暗诱导, 以此确定P700+的氧化程度、P700的氧化速率和P700+再还原速率(Harbinson and Hedley, 1993)。活化光可选择仅激发PSI的远红光, 也可选择激发PSII和PSI的红光、橙光和绿光等。测量过程如图1所示。将暗适应的莱茵衣藻(Chlamydomonas reinhardtii)放在LED激发-探测光谱仪的样品池内, 检测P700在705 nm处的光吸收变化, 活化光是620 nm的红光。莱茵衣藻经过活化光激发后, P700在705 nm处的光吸收迅速增加, P700开始氧化并将电子传递给铁氧还蛋白(Fd)和烟酰胺腺嘌呤二核苷酸磷酸(NADP)等PSI的电子受体。随着光照时间的延长, 逐渐形成稳定的P700+库(姚正菊等, 2002)。根据样品的不同, P700+在几秒到几分钟内缓慢增加至稳态的P700+库(Andreeva and Tikhonov, 1983; Harbinson and Woodward, 1987)。活化光的光强会影响P700的氧化速率以及稳态P700+库的量(图1) (Alric et al., 2010)。活化光关闭后, 光下积累的电子(包括来源于线式和环式电子传递链)经过光合链上的电子递体质体醌(PQ)和Cytb6/f复合体传递给P700+, P700在705 nm处的吸收也逐渐降至活化光打开前的稳定状态, 此时P700+还原为P700 (姚正菊等, 2002)。体外(Haehnel, 1984)和体内(Maxwell and Biggins, 1976; Harbinson and Hedley, 1989)实验表明, 稳态的P700+再还原到P700的t1/2时间是微秒级。为减少非特异性光吸收的影响, P700在705 nm处的光吸收要减去其在730 nm或740 nm处的光吸收变化(Iwai et al., 2010; Takahashi et al., 2013)。图1
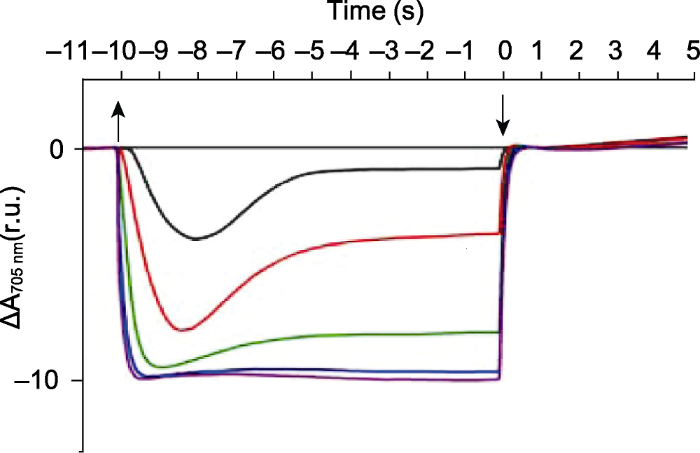
图1活化光诱导的P700氧化还原动力学(改自Alric et al., 2010)
620 nm的红光光强为5 μmol·m-2·s-1 (黑色)、20 μmol·m-2·s-1 (红色)、50 μmol·m-2·s-1 (绿色)、200 μmol·m-2·s-1 (蓝色)、500 μmol·m-2·s-1 (紫色)。莱茵衣藻野生型加入10 μmol·L-1二氯苯基二甲脲(DCMU)和1 mmol·L-1羟胺(HA)。
Figure 1The curves of P700 redox kinetics induced by actinic lights (modified from Alric et al., 2010)
The red light intensity (620 nm) is approximately 5 μmol·m-2·s-1 (black), 20 μmol·m-2·s-1 (red), 50 μmol·m-2·s-1 (green), 200 μmol·m-2·s-1 (blue), 500 μmol·m-2·s-1 (purple). Wild type algae is incubated with 10 μmol·L-1 3-(3,4-dichloro- phenyl)-1,1-dimethylurea (DCMU) and 1 mmol·L-1 hydroxylamine (HA).
根据活化光打开后P700达到2/3稳定氧化态时的时间倒数可计算PSI的天线大小, 在不同生长光强下培养的莱茵衣藻, 其PSI的天线大小并未发生变化(Bonente et al., 2012)。体外分离的PSI核心蛋白以及外周天线复合物(Ballottari et al., 2014; Cao et al., 2020)或者类囊体膜样品在加入电子供体(如抗坏血酸)和电子受体(如甲基紫精)后也可检测PSI的天线大小。根据活化光关闭后稳态P700+库的再还原速率等于流经PSI的电子传递速率(包括线式和环式), 在加入线式电子传递阻断剂后, 可判断围绕PSI的环式电子传递活性(Joliot and Joliot, 2005; Iwai et al., 2010; 高坤山, 2014)。二溴百里香醌(DBMIB, Cytb6/f抑制剂)和二氯苯基二甲脲(DCMU, PSII抑制剂)是常用的研究环式电子传递活性的抑制剂, 可极大降低或阻断来自PSI上游的线式电子, 从而导致P700氧化速率变快, 稳态的P700+库增多(Alric et al., 2010)。将莱茵衣藻厌氧处理35分钟后, 其环式电子传递速率比有氧条件下明显提高(Takahashi et al., 2013)。根据远红光照光后稳态P700+库的大小可判断PSI的光合功能是否受到损伤(Liu et al., 2012; Fristedt et al., 2014; Zhou et al., 2017)。此外, Nguyen等(2017)通过分析嗜热蓝藻(Thermosynechococcus elongatus) PSI三体与其电子供体-细胞色素C6组合体的P700氧化还原动力学, 发现P700氧化与还原受很多因素的影响, 如检测温度、pH值和离子浓度, 且不同的活化光光源会造成结果的差异性, 改变活化光的光强和持续时间可消除此影响。该方法的缺点是, 由于环式电子流的存在, 活化光很难将P700完全氧化。
2 饱和脉冲法计算PSI的光化学量子产量
饱和脉冲(saturation pulse, SP)是一种特殊的活化光, 光强可达40 000 μmol·m-2·s-1, 持续时间一般在1-800毫秒, 这个时间可使一个PSII反应中心发生多次电荷分离, 产生的电子足以将PSII次级电子受体PQ完全还原, PSII反应中心完全关闭。同样, SP也可暂时阻断流经PSI的电子传递。SP关闭后, 电子传递又会在极短的时间内恢复运转, 而不会对样品造成破坏, 因此SP是P700氧化还原动力学研究中常用的一种光。PSI吸收光能后用于光化学反应的能量可以用光化学量子产量表示, 它反映了PSI的光合功能, 其测量过程如图2所示。将暗适应20分钟的拟南芥(Arabidopsis thaliana)叶片剪下, 放置在有镜面的比色杯内, 使用PAM-101双波长检测单元ED-P700DW (810-870 nm) (Walz, Germany)进行P700氧化还原动力学测定。630 nm的活化光(约80 μmol·m-2·s-1, 持续30秒)可将一小部分P700氧化, 该活化光开关引起P700在820 nm处光吸收的变化(即ΔA)。之后720 nm的远红光(约20 μmol·m-2·s-1, 持续30秒)会再次诱导P700的氧化。在远红光照光期间施加饱和脉冲(约3 000 μmol·m-2·s-1, 持续800毫秒), P700在820 nm处的光吸收瞬间达到峰值, P700被完全氧化。随后饱和脉冲诱导产生的大量电子使P700+瞬间还原, 达到完全还原状态。饱和脉冲开关引起P700在820 nm处的光吸收的变化(即ΔAmax)。Klughammer和Schreiber (1994)认为, 样品经活化光照光后处于还原状态的PSI反应中心(即ΔAmax-ΔA)都是开放且有活性的, 依此可以估算PSI的光化学量子产量(ΔAmax-ΔA)/ΔAmax。该方法的缺点是无法区分出由于PSI受体侧受限制导致的处于关闭状态的PSI反应中心。需要特别指出的是, 有研究将ΔAmax定义为远红光开关引起的P700光吸收的变化(Meurer et al., 1996; Varotto et al., 2000)。图2
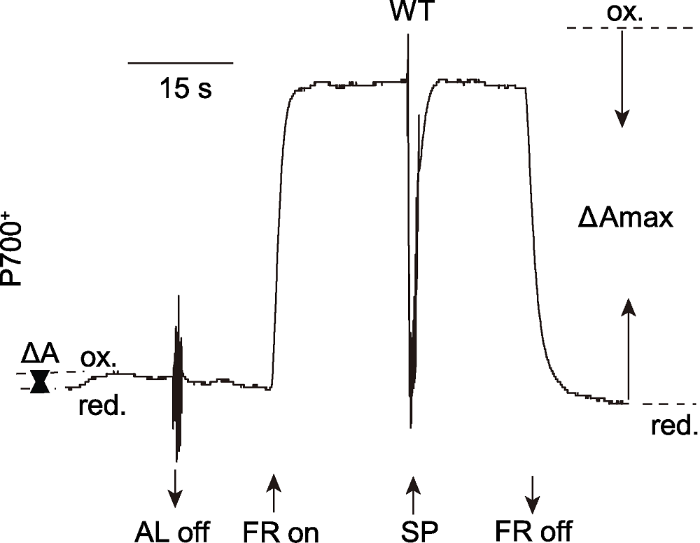
图2饱和脉冲法计算PSI光化学量子产量Y(I) (改自Yang et al., 2017)
AL: 活化光(80 μmol·m-2·s-1); FR: 远红光(720 nm, 20 μmol·m-2·s-1); SP: 饱和脉冲(3000 μmol·m-2·s-1); ΔA: 活化光诱导的部分氧化的P700; ΔAmax: 最大光氧化的P700; ox.: 氧化状态的P700; red.: 还原状态的P700
Figure 2The saturation pulse method for determination of Y(I) (modified from Yang et al., 2017)
AL: Actinic light (80 μmol·m-2·s-1); FR: Far-red light (720 nm, 20 μmol·m-2·s-1); SP: Saturation pulse (3000 μmol·m-2·s-1); ΔA: Partial photo-oxidizable P700 induced by AL; ΔAmax: Maximum photo-oxidizable P700; ox.: P700 oxidation; red.: P700 reduction
根据P700氧化还原动力学并结合叶绿素荧光参数可判断PSI功能是否受到影响, 并区分这些影响是来自PSI本身、电子传递链上游的PSII、还是电子传递链间的电子递体。例如, 拟南芥ppd1突变体在820 nm处检测不到任何光吸收(Liu et al., 2012), 或者仅有很少的光吸收(pyg7突变体) (Yang et al., 2017), 表明突变体PSI功能完全丧失或严重受损, 且导致上游PSII功能受到影响, 表现出强荧光。拟南芥hcf107突变体的P700氧化还原动力学表现出较高的ΔA值和较低的ΔAmax值, 而且在远红光照光过程中施加饱和脉冲光, P700完全没有被还原, 且检测不到叶绿素荧光参数可变荧光Fv, 表明PSII功能完全缺失, PSI接收不到任何来自上游PSII的电子, PSI功能也因此受到损伤。如果饱和脉冲能够还原P700, 但并没有将其完全还原, 叶绿素荧光参数Fv/Fm在0.5以上, 表明PSII与PSI之间的电子传递可能受阻, 从而导致异常的P700氧化还原动力学(hcf118突变体) (Meurer et al., 1996)。
3 饱和脉冲法计算PSI的能量转化
该方法可区分开放的PSI反应中心P700 A (b)和受体侧受限制导致的关闭PSI反应中心P700 A- (c) (图3A)。当样品处于持续的活化光照光时, 活化光会使一部分P700氧化, 引起一部分PSI反应中心关闭, 从而产生PSI电子供体侧受限制的状态, 这部分PSI反应中心为P700+ A (a)。活化光关闭, 同时施加强饱和脉冲(10 000 μmol·m-2·s-1)时则可以使全部有活性的、开放的PSI反应中心P700 A (b)完全氧化, 变成P700+ A-。随着电子不断传递给下游开放状态的PSI受体侧, P700+ A-很快转变成P700+ A。由于强饱和脉冲使得PSI供体侧各电子递体完全氧化, 因此被氧化的P700+不会被还原, 而且在施加强饱和脉冲后的几微秒内, PSI的下游电子受体不会累积电子, 所以P700+A-不太可能转变成P700A- (Klughammer and Schreiber, 1994)。饱和脉冲关闭后, 样品处于黑暗状态, P700回到完全还原状态。远红光打开后, P700逐渐形成稳定的P700+库, 远红光关闭时施加强饱和脉冲(10 000 μmol·m-2·s-1, 持续200毫秒), P700在几微秒内就已达到完全氧化状态, 在此期间来自PSII的电子尚未到达PSI (Klughammer and Schreiber, 2008)。饱和脉冲法将P700的反应中心分成3部分: 由于供体侧受限制导致的关闭的PSI反应中心P700+ A (a)、有活性且开放的PSI反应中心P700 A (b)和由于受体侧受限制导致的关闭的PSI反应中心P700 A- (c)。因此, 有活性的、开放的PSI反应中心的光化学量子产量是Y(I)=b/(a+b+c)=(Pm'-P)/Pm, 由于PSI电子供体侧受限制引起的非光化学量子产量是Y(ND)=a/(a+b+c)=(P-Po)/Pm, 由于PSI电子受体侧受限制引起的非光化学量子产量是Y(NA)=c/(a+b+ c)=(Pm-Pm')/Pm, 而且Y(I)+Y(ND)+Y(NA)=1 (Klughammer and Schreiber, 2008)。图3
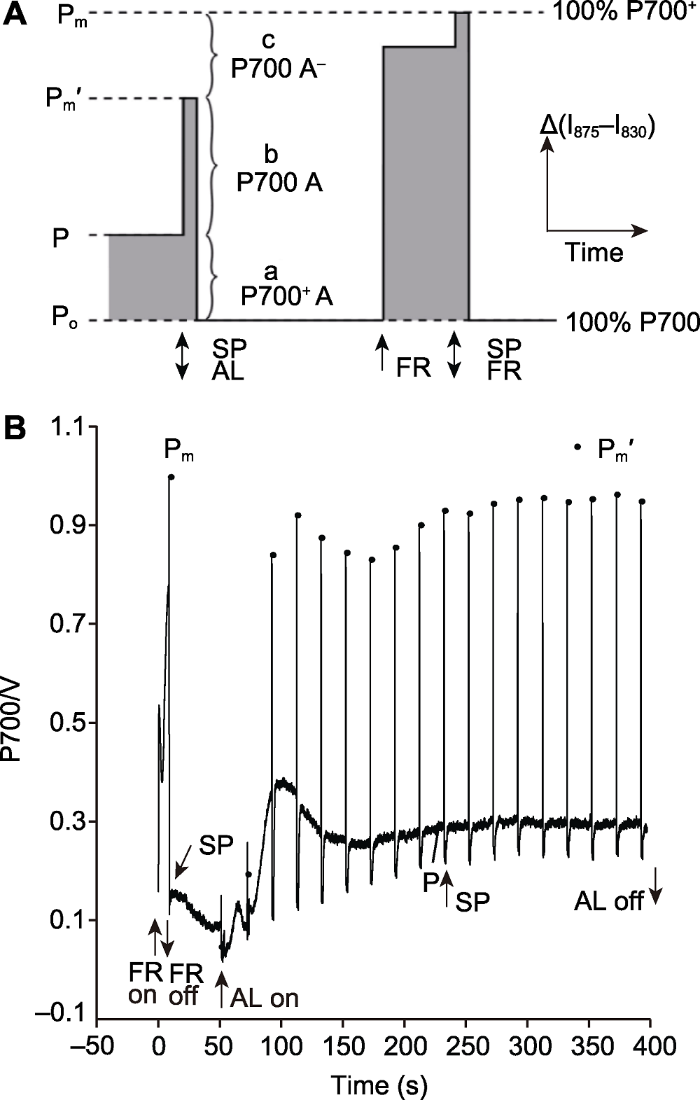
图3利用饱和脉冲法计算PSI能量转化
(A)利用饱和脉冲法计算PSI能量转化的原理示意图(改自Klughammer and Schreiber, 2008); (B) 利用饱和脉冲法计算PSI能量转化的测量曲线。AL: 活化光; SP: 饱和脉冲(10000 μmol·m-2·s-1); FR: 远红光(720 nm); Po: 远红光和饱和脉冲关闭后, 全部PSI反应中心处于完全还原状态, 此时P700信号是0; P: 活化光照光过程中, 部分PSI反应中心被氧化的P700信号; Pm': 活化光关闭, 具有开放、有活性的PSI反应中心被饱和脉冲完全氧化的P700信号; Pm: 远红光关闭, 全部PSI反应中心被饱和脉冲完全氧化的P700信号; a P700+ A: 由于PSI电子供体侧限制导致的关闭的PSI反应中心; A: PSI的下游电子受体; b P700 A: 开放的PSI反应中心; c P700 A-: 由于PSI电子受体侧限制导致的关闭的PSI反应中心; a、b、c是PSI反应中心的3个部分, b是开放的、有活性的, 其光化学量子产量定义为1, 而a和c是关闭的、没有活性的, 其光化学量子产量定义为0。
Figure 3The saturation pulse method for determination of efficiency of energy conversion in PSI
(A) Principle of saturation pulse method for determination of efficiency of energy conversion in PSI (modified from Klughammer and Schreiber, 2008); (B) Curve of saturation pulse method for determination of efficiency of energy conversion in PSI. AL: Actinic light; SP: Saturation pulse (10000 μmol·m-2·s-1); FR: Far-red light (720 nm); Po: Complete reduction is induced after the cessation of SP and far-red light with the zero P700 signal; P: In the presence of AL, a part of the PSI centers are oxidized by the AL resulting in an intermediate P700 signal; Pm': A part of the PSI centers are oxidized completely by the SP with the maximal P700 signal after the AL is turned off; Pm: All the PSI centers are oxidized completely by the SP with the maximal P700 signal after FR pre-illumination; a P700+ A: Donor-side limited closed centers; A: The effective ensemble of PSI acceptors, the same as below; b P700 A: Open centers P700A; c P700 A-: Acceptor-side limited closed centers. a, b, c are the three parts of all PSI centers, b is open and active, and the photochemical quantum yield is unity, while a and c are closed and inactive, and the photochemical quantum yield is 0.
用饱和脉冲法计算PSI能量转化的具体测量过程如图3B所示。将暗适应20分钟的拟南芥叶片剪下并放置在叶室内, 利用饱和脉冲法进行P700氧化还原动力学测定(DUAL-PAM-100, Walz, Germany)。测量开始时打开测量光(830-875 nm), 待基线平稳后, 打开远红光, P700逐渐形成稳定的P700+库, 远红光(约20 μmol·m-2·s-1)持续10秒后关闭, 同时打开强饱和脉冲(10 000 μmol·m-2·s-1, 持续200毫秒), P700达到完全氧化状态(Pm)。饱和脉冲关闭后, P700的光吸收迅速下降并且达到平稳, 此时P700处于完全还原状态(Po)。约40秒后, 打开630 nm的活化光(约80 μmol·m-2·s-1), 一部分P700开始被氧化, 活化光打开1秒后再打开强饱和脉冲, 有活性的、开放的PSI反应中心被完全氧化(Pm'), 之后每隔20秒打开强饱和脉冲(饱和脉冲的间隔时间可根据样品和饱和脉冲的光强等调整), 直至Pm'达到稳定。根据Po、P、Pm'和Pm计算PSI的能量转化。可靠的Pm测定是正确计算PSI能量转化的前提, 因此饱和脉冲的光强设置和持续时间非常关键。藻类和苔藓等样品饱和脉冲光强一般在6 000 μmol·m-2·s-1左右, 高等植物饱和脉冲光强一般在10 000 μmol·m-2·s-1左右。当测定P700氧化还原动力学时出现Pm'大于Pm时, 说明饱和脉冲的光强不够高, P700没有达到最大氧化态。此外, 在饱和脉冲持续时间内, 根据样品有无达到稳定的氧化状态可判断饱和脉冲的持续时间是否合适。对于高等植物样品, 饱和脉冲的持续时间一般为200毫秒左右, 藻类等悬浮液样品的饱和脉冲持续时间一般在50-200毫秒。
当PSI功能受损时, Y(I)会降低, 而Y(ND)和Y(NA)也会发生相应的变化。拟南芥vte6是特异影响PSI功能的突变体, 该突变体中PSI的第2个电子受体——叶绿醌(A1)含量极低。用饱和脉冲法检测该突变体的PSI能量转化时发现突变体Y(I)比野生型低, 而Y(NA)与野生型相比则处于较高的水平, 说明在vte6突变体中, 由于A1的缺失导致A0到FX的电子传递受阻, PSI反应中心P700不能被有效地氧化, 从而产生大量的能量耗散。在vte6突变体中, Y(ND)很小, 说明PSI反应中心P700可有效接收PSII传来的电子, 并完全被还原(Wang et al., 2017)。拟南芥pyg7突变体RNAi系表现出与vte6突变体相似的PSI量子产量变化(Yang et al., 2017)。在照光条件下, 缺乏叶绿素b的大麦(Hordeum vulgare)突变体, 其PSII天线小, 吸光差, PSII的电荷分离低, 从而导致PSI供体侧受限制的能量耗散Y(ND)比野生型高3倍; 而在黑暗条件下, 大麦突变体的Y(NA)却比野生型高2倍多, 可能是其PSI受体库库容较小所致(Pfündel et al., 2008)。
4 P700氧化还原动力学检测光系统间的电子载体库
P700氧化还原动力学不仅可以检测PSI的光合功能, 还可以检测光系统间电子载体库的大小, 其测量过程是在远红光诱导P700氧化还原动力学的基础上施加单周转饱和脉冲(single turnover flash, ST)和多周转饱和脉冲(multiple turnover flash, MT) (图4)。ST光照强度很高(约200 000 μmol·m-2·s-1, 与仪器配置相关), 持续时间一般在5-50微秒, 这个时间可使每个PSI反应中心发生多次电荷分离; 而PSII反应中心仅发生1次电荷分离, PSII产生的1个电子经过光系统间各电子递体被迅速传递给PSI (约几毫秒), 使得氧化态的P700快速还原。由于远红光的持续照光, P700又快速达到原来的稳定氧化状态。MT (光照强度约20 000 μmol·m-2·s-1)持续时间一般在1-800毫秒, 这个时间可使每个PSII反应中心发生多次电荷分离且产生大量电子。MT会诱导P700瞬间达到最大氧化态, 然后被流经PSI的大量电子完全还原。远红光持续照光会使P700再次氧化, 同时还有足够多来自PSII的电子又使氧化态的P700不断还原, 故此时P700的再氧化速度明显低于ST关闭后的再氧化速度。MT和ST引起的曲线下降与水平线(稳定P700+库)之间的面积称为P700氧化还原动力学面积, 两者的面积之比可衡量光系统间电子载体库的大小(Schreiber et al., 1988; Asada et al., 1992)。比值的大小与植物物种以及生长的光环境相关(Schreiber et al., 1988)。由ST引起的P700氧化还原动力学面积可以反映活性PSII的相对含量(Losciale et al., 2008; Kou et al., 2012)。图4
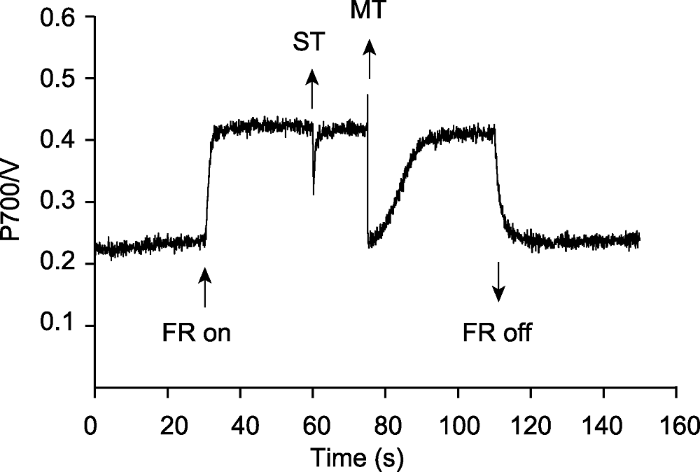
图4光系统间的电子载体库
FR: 远红光(720 nm, 10 μmol·m-2·s-1); ST: 单周转饱和脉冲(200000 μmol·m-2·s-1); MT: 多周转饱和脉冲(20000 μmol·m-2·s-1)
Figure 4The capacity of the intersystem electron carrier pool
FR: Far-red light (720 nm, 10 μmol·m-2·s-1); ST: Single turnover flash (200000 μmol·m-2·s-1); MT: Multiple turnover flash (20000 μmol·m-2·s-1)
5 P700氧化还原动力学技术的局限性
P700氧化还原动力学技术的局限性主要集中在2个方面: 检测设备的灵敏性和检测过程的干扰因素。目前, 检测P700氧化还原动力学的设备, 其最高时间分辨率是10微秒。在光合电子传递链中, PC被PSI氧化大约需要10微秒, 而PSI被光激发后, 产生的电子依次传递给叶绿素分子(A0)、A1, 以及铁硫簇反应中心的FX、FA和FB, 最后传递给Fd, 整个过程在皮秒到纳秒内完成(Hiyama and Ke, 1971; Vassiliev et al., 1998; Díaz-Quintana et al., 1998)。P700的氧化与还原可在极短的时间内完成, 因此提高检测设备的时间分辨率很关键。在测量过程中有诸多因素会干扰测量结果的准确性。PC是含铜的水溶性蛋白质, 位于类囊体囊腔侧, 穿梭在细胞色素b6f复合体与PSI之间转运电子。当在820 nm处检测P700的吸收变化时, PC的吸收变化约占总变化的23% (Joliot and Johnson, 2011), 而且当P700处于完全还原状态时, 会同时存在约30%-50%的氧化态PC (Schreiber, 2017), 因此PC是检测P700的氧化还原时较大的影响因素。Fd是可溶性铁硫蛋白, 位于类囊体基质侧, 通过4个半胱氨酸残基与PSI结合, 可将电子传递给铁氧还蛋白(Fd)或黄素蛋白(Sétif, 2001)。Fd的氧化与还原可以引起其在可见光和近红外光少量的吸收, 该吸收变化与P700的吸收变化有部分重叠。此外, PSI反应中心发生电荷分离后, 如果缺少氧化态的Fd, 或者缺少外源的电子受体, 该电子传递过程中各个电子载体会在100毫秒内发生电荷重组(Brettel, 1997; Kadota et al., 2019), 导致100% P700+信号被低估(图3A), 最终导致Y(I)被高估。
对于悬浮样品, 尤其是绿藻, 其P700氧化还原动力学信号较弱。为增强P700信号, 可适当增加藻细胞浓度(20-50 μg·mL-1), 但是单纯增加悬浮液浓度, 会导致其偏离比尔定律, 造成测量结果不准确。为防止细胞沉降, 可加入10%-20%聚蔗糖, 增加溶液的黏稠度。
致谢
感谢中国科学院植物研究所温晓刚研究员、田利金研究员和中国科学院分子植物科学卓越创新中心(植物生理生态研究所)米华玲研究员在文章撰写过程中提出的宝贵意见以及对文章内容的修改。参考文献 原文顺序
文献年度倒序
文中引用次数倒序
被引期刊影响因子
[本文引用: 1]
[本文引用: 1]
[本文引用: 2]
[本文引用: 1]
URLPMID:19651104 [本文引用: 4]
DOI:10.1038/nature05687URLPMID:17476261 [本文引用: 1]

All higher organisms on Earth receive energy directly or indirectly from oxygenic photosynthesis performed by plants, green algae and cyanobacteria. Photosystem I (PSI) is a supercomplex of a reaction centre and light-harvesting complexes. It generates the most negative redox potential in nature, and thus largely determines the global amount of enthalpy in living systems. We report the structure of plant PSI at 3.4 A resolution, revealing 17 protein subunits. PsaN was identified in the luminal side of the supercomplex, and most of the amino acids in the reaction centre were traced. The crystal structure of PSI provides a picture at near atomic detail of 11 out of 12 protein subunits of the reaction centre. At this level, 168 chlorophylls (65 assigned with orientations for Q(x) and Q(y) transition dipole moments), 2 phylloquinones, 3 Fe(4)S(4) clusters and 5 carotenoids are described. This structural information extends the understanding of the most efficient nano-photochemical machine in nature.
URLPMID:19446520 [本文引用: 1]
[本文引用: 1]
[本文引用: 1]
DOI:10.1073/pnas.1404377111URLPMID:24872450 [本文引用: 1]

In oxygenic photosynthetic eukaryotes, the hydroxylated carotenoid zeaxanthin is produced from preexisting violaxanthin upon exposure to excess light conditions. Zeaxanthin binding to components of the photosystem II (PSII) antenna system has been investigated thoroughly and shown to help in the dissipation of excess chlorophyll-excited states and scavenging of oxygen radicals. However, the functional consequences of the accumulation of the light-harvesting complex I (LHCI) proteins in the photosystem I (PSI) antenna have remained unclarified so far. In this work we investigated the effect of zeaxanthin binding on photoprotection of PSI-LHCI by comparing preparations isolated from wild-type Arabidopsis thaliana (i.e., with violaxanthin) and those isolated from the A. thaliana nonphotochemical quenching 2 mutant, in which violaxanthin is replaced by zeaxanthin. Time-resolved fluorescence measurements showed that zeaxanthin binding leads to a previously unrecognized quenching effect on PSI-LHCI fluorescence. The efficiency of energy transfer from the LHCI moiety of the complex to the PSI reaction center was down-regulated, and an enhanced PSI resistance to photoinhibition was observed both in vitro and in vivo. Thus, zeaxanthin was shown to be effective in inducing dissipative states in PSI, similar to its well-known effect on PSII. We propose that, upon acclimation to high light, PSI-LHCI changes its light-harvesting efficiency by a zeaxanthin-dependent quenching of the absorbed excitation energy, whereas in PSII the stoichiometry of LHC antenna proteins per reaction center is reduced directly.
URLPMID:22205699 [本文引用: 1]
[本文引用: 1]
DOI:10.1016/s0005-2728(01)00202-xURLPMID:11687210 [本文引用: 1]

This mini-review focuses on recent experimental results and questions, which came up since the last more comprehensive reviews on the subject. We include a brief discussion of the different techniques used for time-resolved studies of electron transfer in photosystem I (PS I) and relate the kinetic results to new structural data of the PS I reaction centre.
DOI:10.1038/s41477-020-0593-7URLPMID:32042157 [本文引用: 2]

Under iron-deficiency stress, which occurs frequently in natural aquatic environments, cyanobacteria reduce the amount of iron-enriched proteins, including photosystem I (PSI) and ferredoxin (Fd), and upregulate the expression of iron-stress-induced proteins A and B (IsiA and flavodoxin (Fld)). Multiple IsiAs function as the peripheral antennae that encircle the PSI core, whereas Fld replaces Fd as the electron receptor of PSI. Here, we report the structures of the PSI3-IsiA18-Fld3 and PSI3-IsiA18 supercomplexes from Synechococcus sp. PCC 7942, revealing features that are different from the previously reported PSI structures, and a sophisticated pigment network that involves previously unobserved pigment molecules. Spectroscopic results demonstrated that IsiAs are efficient light harvesters for PSI. Three Flds bind symmetrically to the trimeric PSI core-we reveal the detailed interaction and the electron transport path between PSI and Fld. Our results provide a structural basis for understanding the mechanisms of light harvesting, energy transfer and electron transport of cyanobacterial PSI under stressed conditions.
URLPMID:9521664 [本文引用: 1]
URLPMID:25228689 [本文引用: 1]
[本文引用: 1]
[本文引用: 1]
DOI:10.1104/pp.103.2.649URLPMID:12231970 [本文引用: 1]

Following dark adaptation, the response to irradiance of chlorophyll (Chl) fluorescence, the light-induced absorbance change around 820 nm (to measure reaction center Chl of photosystem I [PSI] P-700 oxidation), and CO2 fixation were examined in pea (Pisum sativum L.) leaves under a range of conditions. Initially, P-700 oxidation is restricted by a lack of regeneration of PSI electron acceptors, and the increase of oxidized P-700 (P-700+) that occurs during approximately the first 60 s of irradiation is largely independent of the resistance to electron flow between the two photosystems. Under these conditions, the quantum efficiency for linear electron flow is directly positively related to P-700+ accumulation, which is in contrast to the direct negative correlation that is the most frequently reported relationship between P-700+ accumulation and the quantum efficiency for linear electron flow.
[本文引用: 1]
DOI:10.1073/pnas.68.5.1010URLPMID:4995817 [本文引用: 1]

The technique of flash kinetic spectrophotometry was used to demonstrate a broad absorption band around 430 nm, which was kinetically different from P700, in several photosystem-I particles from spinach and blue-green algae. The component represented by this absorption band, designated as
DOI:10.1038/nature08885URLPMID:20364124 [本文引用: 2]

Photosynthetic light reactions establish electron flow in the chloroplast's thylakoid membranes, leading to the production of the ATP and NADPH that participate in carbon fixation. Two modes of electron flow exist-linear electron flow (LEF) from water to NADP(+) via photosystem (PS) II and PSI in series and cyclic electron flow (CEF) around PSI (ref. 2). Although CEF is essential for satisfying the varying demand for ATP, the exact molecule(s) and operational site are as yet unclear. In the green alga Chlamydomonas reinhardtii, the electron flow shifts from LEF to CEF on preferential excitation of PSII (ref. 3), which is brought about by an energy balancing mechanism between PSII and PSI (state transitions). Here, we isolated a protein supercomplex composed of PSI with its own light-harvesting complex (LHCI), the PSII light-harvesting complex (LHCII), the cytochrome b(6)f complex (Cyt bf), ferredoxin (Fd)-NADPH oxidoreductase (FNR), and the integral membrane protein PGRL1 (ref. 5) from C. reinhardtii cells under PSII-favouring conditions. Spectroscopic analyses indicated that on illumination, reducing equivalents from downstream of PSI were transferred to Cyt bf, whereas oxidised PSI was re-reduced by reducing equivalents from Cyt bf, indicating that this supercomplex is engaged in CEF (Supplementary Fig. 1). Thus, formation and dissociation of the PSI-LHCI-LHCII-FNR-Cyt bf-PGRL1 supercomplex not only controlled the energy balance of the two photosystems, but also switched the mode of photosynthetic electron flow.
DOI:10.1073/pnas.1110189108URLPMID:21784980 [本文引用: 1]

Cyclic electron flow is increasingly recognized as being essential in plant growth, generating a pH gradient across thylakoid membrane (DeltapH) that contributes to ATP synthesis and triggers the protective process of nonphotochemical quenching (NPQ) under stress conditions. Here, we report experiments demonstrating the importance of that DeltapH in protecting plants from stress and relating to the regulation of cyclic relative to linear flow. In leaves infiltrated with low concentrations of nigericin, which dissipates the DeltapH without significantly affecting the potential gradient, thereby maintaining ATP synthesis, the extent of NPQ was markedly lower, reflecting the lower DeltapH. At the same time, the photosystem (PS) I primary donor P700 was largely reduced in the light, in contrast to control conditions where increasing light progressively oxidized P700, due to down-regulation of the cytochrome bf complex. Illumination of nigericin-infiltrated leaves resulted in photoinhibition of PSII but also, more markedly, of PSI. Plants lacking ferredoxin (Fd) NADP oxidoreductase (FNR) or the polypeptide proton gradient regulation 5 (PGR5) also show reduction of P700 in the light and increased sensitivity to PSI photoinhibition, demonstrating that the regulation of the cytochrome bf complex (cyt bf) is essential for protection of PSI from light stress. The formation of a DeltapH is concluded to be essential to that regulation, with cyclic electron flow playing a vital, previously poorly appreciated role in this protective process. Examination of cyclic electron flow in plants with a reduced content of FNR shows that these antisense plants are less able to maintain a steady rate of this pathway. This reduction is suggested to reflect a change in the distribution of FNR from cyclic to linear flow, likely reflecting the formation or disassembly of FNR-cytochrome bf complex.
URLPMID:15781857 [本文引用: 1]
DOI:10.1038/35082000URLPMID:11418848 [本文引用: 1]

Life on Earth depends on photosynthesis, the conversion of light energy from the Sun to chemical energy. In plants, green algae and cyanobacteria, this process is driven by the cooperation of two large protein-cofactor complexes, photosystems I and II, which are located in the thylakoid photosynthetic membranes. The crystal structure of photosystem I from the thermophilic cyanobacterium Synechococcus elongatus described here provides a picture at atomic detail of 12 protein subunits and 127 cofactors comprising 96 chlorophylls, 2 phylloquinones, 3 Fe4S4 clusters, 22 carotenoids, 4 lipids, a putative Ca2+ ion and 201 water molecules. The structural information on the proteins and cofactors and their interactions provides a basis for understanding how the high efficiency of photosystem I in light capturing and electron transfer is achieved.
DOI:10.3390/plants8060152URL [本文引用: 1]
DOI:10.1016/0304-4173(73)90010-4URLPMID:4350387 [本文引用: 1]
[本文引用: 3]
[本文引用: 1]
[本文引用: 5]
[本文引用: 1]
URLPMID:22644475 [本文引用: 1]
URLPMID:23221595 [本文引用: 3]
URLPMID:18251867 [本文引用: 1]
DOI:10.1021/bi00663a011URLPMID:963015 [本文引用: 1]

The light-induced turnover of P700 was measured spectrophotometrically in a wide variety of algae and some photosynthetic mutants. Analysis of the postillumination recovery of P700+ revealed that the apparent first-order rate constant for reduction via the cyclic pathway was much lower that that via the noncyclic pathway. After activation of photosystems 1 and 2 the half-time for reduction of P700+ was 5-20 ms, whereas after activation of primarily photosystem 1 a longer half-time of ca. 150 ms was observed. The extent of the photooxidation of P700 was the same in both regimes of illumination. The longer half-time was also noted after inhibition of photosystem 2 by 3-(3,4-dichlorophenyl)-1,1-dimethylurea or mild heat shock and in mutant algae known to lack a functional photosystem 2. No signal was observed in mutants lacking P700 itself but those strains lacking either plastocyanin or cytochrome f were capable of a very slow turnover (reduction t 1/2 greater than 500 ms at room temperature). This very slow turnover was not affected by carbonyl cyanide m-chlorophenylhydrazone or the plastoquinone antagonist, 2,5-dibromo-3-methyl-6-isopropyl-p-benzoquinone, indicating that the pathway for reduction of P700+ in these mutants is not energy linked and does not utilize the intersystem electron transport chain. The slow, 150 ms, reduction of P700+ due to cyclic flow was not observed when cells were engaged in photosynthesis at high-light intensities. The data are interpreted as evidence for the involvement of the total functional pool of P700 in both electron transport pathways, and we suggest that cyclic electron transport does not contribute to photosynthesis in oxygen-evolving autotrophs.
DOI:10.1007/BF00620055URLPMID:8717135 [本文引用: 2]

Thirty-four recessive photosynthetic mutants of the high-chlorophyll-fluorescence (hcf) phenotype have been isolated by screening 7700 M2 progenies of ethyl methane sulfonate-treated seeds of Arabidopsis thaliana. Most of the mutants isolated were found to be seedling-lethal, but could be grown on sucrose-supplemented media. Chlorophyll (Chl) fluorescence induction, absorption changes in the reaction-centre chlorophyll of PS I (P700) at 830 nm and Chl a/Chl b ratios were recorded in order to probe the photosynthetic functions and to define the mutational lesion. These studies were complemented by immunoblot and Northern analyses which finally led to the classification of the mutants into six different groups. Four classes of mutants were affected in PS I, PS II (two different classes) or the intersystem electron-transport chain, respectively. A fifth mutant class was of pleiotropic nature and the sixth class comprised a Chl b-deficient mutant. Several of the mutants showed severe deficiencies in the levels of subunits of PS I, PS II or the cytochrome b6/f complex. Thus the mutational lesions could be located precisely. Only one mutant was defective in the transcript patterns of some plastid-encoded photosynthesis genes. Hence most of the mutants isolated appear to be affected in translational and post-translational regulatory processes of thylakoid membrane biogenesis or in structural genes encoding constituent subunits of the thylakoid protein complexes.
DOI:10.1016/j.bbabio.2009.10.005URLPMID:19853576 [本文引用: 1]

The outer antenna system of Chlamydomonas reinhardtii Photosystem I is composed of nine gene products, but due to difficulty in purification their individual properties are not known. In this work, the functional properties of the nine Lhca antennas of Chlamydomonas, have been investigated upon expression of the apoproteins in bacteria and refolding in vitro of the pigment-protein complexes. It is shown that all Lhca complexes have a red-shifted fluorescence emission as compared to the antenna complexes of Photosystem II, similar to Lhca from higher plants, but less red-shifted. Three complexes, namely Lhca2, Lhca4 and Lhca9, exhibit emission maxima above 707 nm and all carry an asparagine as ligand for Chl 603. The comparison of the protein sequences and the biochemical/spectroscopic properties of the refolded Chlamydomonas complexes with those of the well-characterized Arabidopsis thaliana Lhcas shows that all the Chlamydomonas complexes have a chromophore organization similar to that of A. thaliana antennas, particularly to Lhca2, despite low sequence identity. All the major biochemical and spectroscopic properties of the Lhca complexes have been conserved through the evolution, including those involved in
DOI:10.1007/BF02619630URLPMID:6097529 [本文引用: 1]

Na+,K+-ATPase, HCO3(-)-ATPase, Ca2+,Mg2+,-ATPase, Ca2+-ATPase, and alkaline phosphatase activities were measured in cultures of osteoblastlike cells treated with fluoride and cortisol separately and in combinations. Low concentrations of cortisol increased HCO3- -ATPase (10(-11) to 10(-18) M cortisol) and alkaline phosphatase (10(-11) to 10(-9) M cortisol) activities, but higher cortisol concentrations reduced these activities. Na+,K+-ATPase, Ca2+,Mg2+-ATPase, and Ca2+-ATPase activities tended only to be reduced by cortisol. Fluoride (10(-6) and 5 X 10(-6) M) increased HCO3(-)-ATPase and alkaline phosphatase activities, but these activities were similar to controls in the presence of 10(-5) M fluoride. Ca2+,Mg2+-ATPase activity was decreased and Na+,K+-ATPase activity was increased as the concentration of fluoride increased (10(-6) to 10(-5) M). Preliminary experiments with fluoride indicated that lower concentrations (10(-7) M) were without effect. Cortisol concentrations of 10(-9) and 10(-8) M were chosen for studies with combinations of cortisol and fluoride because the effects of these concentrations on alkaline phosphatase activity were opposite, i.e. 10(-9) M increased whereas 10(-8) M decreased activity. Fluoride concentrations of 10(-6), 5 X 10(-6), and 10(-5) M were chosen because a peak of alkaline phosphatase activity occurred at 5 X 10(-6) M fluoride. Higher (10(-4) M) and lower (10(-7) M) fluoride concentrations were without effect. The effects of combinations of cortisol and fluoride depend on the enzyme activity measured. Fluoride (10(-6) M) combined with cortisol (10(-9) M) produced a peak of Na+,K+-ATPase activity. The increased activity obtained with all concentrations of fluoride alone was preserved when fluoride was combined with 10(-8) M cortisol, although the activity tended to be reduced at 5 X 10(-6) and 10(-5) M fluoride. HCO3(-)-ATPase activity was increased by fluoride combined with 10(-8) M cortisol and decreased by fluoride combined with 10(-9) M cortisol compared to the activities obtained with fluoride alone. The decrease in Ca2+,Mg2+-ATPase activity caused by fluoride alone was prevented by 10(-9) and enhanced by 10(-8) M cortisol, although all treatments produced the same activity at 10(-5) M fluoride. Ca2+-ATPase activity tended to be increased by combinations of fluoride and cortisol, but significantly so only at 10(-5) M fluoride in combinations with 10(-8) and 10(-9) M cortisol.(ABSTRACT TRUNCATED AT 400 WORDS)
[本文引用: 1]
DOI:10.1073/pnas.1722482115URLPMID:29632169 [本文引用: 1]

Photosystem I (PSI) is one of the two photosystems present in oxygenic photosynthetic organisms and functions to harvest and convert light energy into chemical energy in photosynthesis. In eukaryotic algae and higher plants, PSI consists of a core surrounded by variable species and numbers of light-harvesting complex (LHC)I proteins, forming a PSI-LHCI supercomplex. Here, we report cryo-EM structures of PSI-LHCR from the red alga Cyanidioschyzon merolae in two forms, one with three Lhcr subunits attached to the side, similar to that of higher plants, and the other with two additional Lhcr subunits attached to the opposite side, indicating an ancient form of PSI-LHCI. Furthermore, the red algal PSI core showed features of both cyanobacterial and higher plant PSI, suggesting an intermediate type during evolution from prokaryotes to eukaryotes. The structure of PsaO, existing in eukaryotic organisms, was identified in the PSI core and binds three chlorophylls a and may be important in harvesting energy and in mediating energy transfer from LHCII to the PSI core under state-2 conditions. Individual attaching sites of LHCRs with the core subunits were identified, and each Lhcr was found to contain 11 to 13 chlorophylls a and 5 zeaxanthins, which are apparently different from those of LHCs in plant PSI-LHCI. Together, our results reveal unique energy transfer pathways different from those of higher plant PSI-LHCI, its adaptation to the changing environment, and the possible changes of PSI-LHCI during evolution from prokaryotes to eukaryotes.
DOI:10.1038/s41477-019-0379-yURLPMID:30850820 [本文引用: 1]

Photosystem I (PSI) is a highly efficient natural light-energy converter, and has diverse light-harvesting antennas associated with its core in different photosynthetic organisms. In green algae, an extremely large light-harvesting complex I (LHCI) captures and transfers energy to the PSI core. Here, we report the structure of PSI-LHCI from a green alga Bryopsis corticulans at 3.49 A resolution, obtained by single-particle cryo-electron microscopy, which revealed 13 core subunits including subunits characteristic of both prokaryotes and eukaryotes, and 10 light-harvesting complex a (Lhca) antennas that form a double semi-ring and an additional Lhca dimer, including a novel four-transmembrane-helix Lhca. In total, 244 chlorophylls were identified, some of which were located at key positions for the fast energy transfer. These results provide a firm structural basis for unravelling the mechanisms of light-energy harvesting, transfer and quenching in the green algal PSI-LHCI, and important clues as to how PSI-LHCI has changed during evolution.
DOI:10.1126/science.aab0214URLPMID:26023133 [本文引用: 1]

Photosynthesis converts solar energy to chemical energy by means of two large pigment-protein complexes: photosystem I (PSI) and photosystem II (PSII). In higher plants, the PSI core is surrounded by a large light-harvesting complex I (LHCI) that captures sunlight and transfers the excitation energy to the core with extremely high efficiency. We report the structure of PSI-LHCI, a 600-kilodalton membrane protein supercomplex, from Pisum sativum (pea) at a resolution of 2.8 angstroms. The structure reveals the detailed arrangement of pigments and other cofactors-especially within LHCI-as well as numerous specific interactions between the PSI core and LHCI. These results provide a firm structural basis for our understanding on the energy transfer and photoprotection mechanisms within the PSI-LHCI supercomplex.
DOI:10.1007/s11120-017-0394-7URLPMID:28497192 [本文引用: 1]

Properties and performance of the recently introduced Dual/KLAS-NIR spectrophotometer for simultaneous measurements of ferredoxin (Fd), P700, and plastocyanin (PC) redox changes, together with whole leaf chlorophyll a (Chl) fluorescence (emission >760, 540 nm excitation) are outlined. Spectral information on in vivo Fd, P700, and PC in the near-infrared region (NIR, 780-1000 nm) is presented, on which the new approach is based. Examples of application focus on dark-light and light-dark transitions, where maximal redox changes of Fd occur. After dark-adaptation, Fd reduction induced by moderate light parallels the Kautsky effect of Chl fluorescence induction. Both signals are affected analogously by removal of O2. A rapid type of Fd reoxidation, observed after a short pulse of light before light activation of linear electron transport (LET), is more pronounced in C4 compared to C3 leaves and interpreted to reflect cyclic PS I (CET). Light activation of LET, as assessed via the rate of Fd reoxidation after short light pulses, occurs at very low intensities and is slowly reversed (half-time ca. 20 min). Illumination with strong far-red light (FR, 740 nm) reveals two fractions of PS I, PS I (LET), and PS I (CET), differing in the rates of Fd reoxidation upon FR-off and the apparent equilibrium constants between P700 and PC. Parallel information on oxidation of Fd and reduction of P700 plus PC proves essential for identification of CET. Comparison of maize (C4) with sunflower and ivy (C3) responses leads to the conclusion that segregation of two types of PS I may not only exist in C4 (mesophyll and bundle sheath cells), but also in C3 photosynthesis (grana margins plus end membranes and stroma lamellae).
[本文引用: 4]
DOI:10.1016/s0005-2728(01)00205-5URLPMID:11687213 [本文引用: 1]

Ferredoxin and flavodoxin are soluble proteins which are reduced by the terminal electron acceptors of photosystem I. The kinetics of ferredoxin (flavodoxin) photoreduction are discussed in detail, together with the last steps of intramolecular photosystem I electron transfer which precede ferredoxin (flavodoxin) reduction. The present knowledge concerning the photosystem I docking site for ferredoxin and flavodoxin is described in the second part of the review.
DOI:10.1038/ncomms2954URLPMID:23760547 [本文引用: 2]

Photosynthesis is the biological process that feeds the biosphere with reduced carbon. The assimilation of CO2 requires the fine tuning of two co-existing functional modes: linear electron flow, which provides NADPH and ATP, and cyclic electron flow, which only sustains ATP synthesis. Although the importance of this fine tuning is appreciated, its mechanism remains equivocal. Here we show that cyclic electron flow as well as formation of supercomplexes, thought to contribute to the enhancement of cyclic electron flow, are promoted in reducing conditions with no correlation with the reorganization of the thylakoid membranes associated with the migration of antenna proteins towards Photosystems I or II, a process known as state transition. We show that cyclic electron flow is tuned by the redox power and this provides a mechanistic model applying to the entire green lineage including the vast majority of the cases in which state transition only involves a moderate fraction of the antenna.
DOI:10.1046/j.1365-313x.2000.00717.xURLPMID:10792827 [本文引用: 1]

The psae1-1 mutant of Arabidopsis was identified on the basis of a decrease in the effective quantum yield of photosystem II, among a collection of plants subjected to transposon tagging with the Enhancer element. The steady-state redox level and the rate of re-oxidation of P700 are significantly altered in psae1-1 mutants. The responsible mutation was localised to psaE1, one of two Arabidopsis genes that encode subunit E of photosystem I. An additional mutant allele, psae1-2, was identified by reverse genetics. In wild-type plants, the psaE1 transcript is expressed at a higher level than psaE2 mRNA. In the mutants, however, the E1 transcript was barely detectable, and was expressed only in small groups of wild-type cells resulting from somatic reversions. As a consequence, the amount of PsaE protein present in the mutant is significantly reduced. Concomitantly, the levels of other stromal photosystem I subunits (PsaC and PsaD) are also affected. Mutant plants showed a marked increase in light sensitivity and photoinhibition. Additional effects of the psae1 mutation include light green pigmentation, an increase in chlorophyll fluorescence and a decrease of approximately 50% in growth rate under greenhouse conditions.
DOI:10.1016/S0006-3495(98)77909-3URLPMID:9545061 [本文引用: 1]

The PsaC subunit of photosystem I (PS I) binds two [4Fe-4S] clusters, F(A) and F(B), functioning as electron carriers between F(X) and soluble ferredoxin. To resolve the issue whether F(A) or F(B) is proximal to F(X), we used single-turnover flashes to promote step-by-step electron transfer between electron carriers in control (both F(A) and F(B) present) and HgCl2-treated (F(B)-less) PS I complexes from Synechococcus sp. PCC 6301 and analyzed the kinetics of P700+ reduction by monitoring the absorbance changes at 832 nm in the presence of a fast electron donor (phenazine methosulfate (PMS)). In control PS I complexes exogenously added ferredoxin, or flavodoxin could be photoreduced on each flash, thus allowing P700+ to be reduced from PMS. In F(B)-less complexes, both in the presence and in the absence of ferredoxin or flavodoxin, P700+ was reduced from PMS only on the first flash and was reduced from F(X)- on the following flashes, indicating lack of electron transfer to ferredoxin or flavodoxin. In the F(B)-less complexes, a normal level of P700 photooxidation was detected accompanied by a high yield of charge recombination between P700+ and F(A)- in the presence of a slow donor, 2,6-dichlorophenol-indophenol. This recombination remained the only pathway of F(A)- reoxidation in the presence of added ferredoxin, consistent with the lack of forward electron transfer. F(A)- could be reoxidized by methyl viologen in F(B)-less PS I complexes, although at a concentration two orders of magnitude higher than is required in wild-type PS I complexes, thus implying the presence of a diffusion barrier. The inhibition of electron transfer to ferredoxin and flavodoxin was completely reversed after reconstituting the F(B) cluster. Using rate versus distance estimates for electron transfer rates from F(X) to ferredoxin for two possible orientations of PsaC, we conclude that the kinetic data are best compatible with PsaC being oriented with F(A) as the cluster proximal to F(X) and F(B) as the distal cluster that donates electrons to ferredoxin.
DOI:10.1016/j.molp.2016.12.006URLPMID:28007557 [本文引用: 1]

Phytyl-diphosphate, which provides phytyl moieties as a common substrate in both tocopherol and phylloquinone biosynthesis, derives from de novo isoprenoid biosynthesis or a salvage pathway via phytol phosphorylation. However, very little is known about the role and origin of the phytyl moiety for phylloquinone biosynthesis. Since VTE6, a phytyl-phosphate kinase, is a key enzyme for phytol phosphorylation, we characterized Arabidopsis vte6 mutants to gain insight into the roles of phytyl moieties in phylloquinone biosynthesis and of phylloquinone in photosystem I (PSI) biogenesis. The VTE6 knockout mutants vte6-1 and vte6-2 lacked detectable phylloquinone, whereas the phylloquinone content in the VTE6 knockdown mutant vte6-3 was 90% lower than that in wild-type. In vte6 mutants, PSI function was impaired and accumulation of the PSI complex was defective. The PSI core subunits PsaA/B were efficiently synthesized and assembled into the PSI complex in vte6-3. However, the degradation rate of PSI subunits in the assembled PSI complex was more rapid in vte6-3 than in wild-type. In vte6-3, PSI was more susceptible to high-light damage than in wild-type. Our results provide the first genetic evidence that the phytol phosphorylation pathway is essential for phylloquinone biosynthesis, and that phylloquinone is required for PSI complex stability.
[本文引用: 1]
DOI:10.1016/j.bbabio.2014.12.011URLPMID:25582571 [本文引用: 1]

Photosystem I, an integral membrane and multi-subunit complex, catalyzes the oxidation of plastocyanin and the reduction of ferredoxin by absorbed light energy. Photosystem I participates in photosynthetic acclimation processes by being involved in cyclic electron transfer and state transitions for sustaining efficient photosynthesis. The photosystem I complex is highly conserved from cyanobacteria to higher plants and contains the light-harvesting complex and the reaction center complex. The assembly of the photosystem I complex is highly complicated and involves the concerted assembly of multiple subunits and hundreds of cofactors. A suite of regulatory factors for the assembly of photosystem I subunits and cofactors have been identified that constitute an integrative network regulating PSI accumulation. This review aims to discuss recent findings in the field relating to how the photosystem I complex is assembled in oxygenic organisms. This article is part of a Special Issue entitled: Chloroplast Biogenesis.
DOI:10.1111/tpj.13618URLPMID:28636143 [本文引用: 5]

Although progress has been made in determining the structure and understanding the function of photosystem I (PSI), the PSI assembly process remains poorly understood. PsaC is an essential subunit of PSI and participates in the transfer of electrons to ferredoxin. However, how PsaC is assembled during accumulation of the PSI complex is unknown. In the present study, we showed that Pyg7 localized to the stromal thylakoid and associated with the PSI complex. We also showed that Pyg7 interacted with PsaC. Furthermore, we found that the PSI assembly process was blocked following formation of the PsaAB heterodimer in the pyg7 mutant. In addition, the analyses of PSI stability in Pyg7RNAi plants showed that Pyg7 is involved in maintaining the assembled PSI complex under excess-light conditions. Moreover, we demonstrated that decreased Pyg7 content resulted in decreased efficiency of PSI assembly in Pyg7RNAi plants. These findings suggest that the role of Pyg7 in PSI biogenesis has evolved as an essential assembly factor by interacting with PsaC in Arabidopsis, in addition to being a stability factor for PSI as seen in Synechocystis.
DOI:10.1073/pnas.1612460114URLPMID:28167782 [本文引用: 1]

Numerous attempts have been made to identify and engineer sequence-specific RNA endonucleases, as these would allow for efficient RNA manipulation. However, no natural RNA endonuclease that recognizes RNA in a sequence-specific manner has been described to date. Here, we report that SUPPRESSOR OF THYLAKOID FORMATION 1 (SOT1), an Arabidopsis pentatricopeptide repeat (PPR) protein with a small MutS-related (SMR) domain, has RNA endonuclease activity. We show that the SMR moiety of SOT1 performs the endonucleolytic maturation of 23S and 4.5S rRNA through the PPR domain, specifically recognizing a 13-nucleotide RNA sequence in the 5' end of the chloroplast 23S-4.5S rRNA precursor. In addition, we successfully engineered the SOT1 protein with altered PPR motifs to recognize and cleave a predicted RNA substrate. Our findings point to SOT1 as an exciting tool for RNA manipulation.
1
2014
... 根据活化光打开后P700达到2/3稳定氧化态时的时间倒数可计算PSI的天线大小, 在不同生长光强下培养的莱茵衣藻, 其PSI的天线大小并未发生变化(
1
1991
... PSI的反应中心有1个具有光化学反应性的叶绿素a分子二聚体, 即P700 (
光诱导叶片P-700氧化还原的测量
2
2002
... 活化光诱导的P700氧化还原动力学是将暗适应的藻类或高等植物进行光-暗诱导, 以此确定P700+的氧化程度、P700的氧化速率和P700+再还原速率(
... 还原为P700 (
光系统I (PSI)的结构与功能研究进展
1
2001
... 光合作用是地球上进行的最大规模的化学反应.放氧光合生物吸收太阳能并裂解水分子, 释放出地球上绝大多数生命活动所需的氧气, 同时固定大气或水中的CO2并合成有机物, 为新陈代谢提供能量.在高等植物和真核藻类中, 叶绿体是光合作用的场所.叶绿体的类囊体膜上排布着4个膜蛋白复合物: 光系统II (PSII)、细胞色素b6f (Cytb6f)、光系统I (PSI)和腺苷三磷酸合酶(ATP synthase).它们紧密协作, 共同完成光能吸收、电子传递和能量转化, 最终合成腺苷三磷酸(ATP)和还原型烟酰胺腺嘌呤二核苷酸磷酸(辅酶II) (NADPH).其中, PSI主要参与光合电子传递的最终步骤, 即氧化类囊体囊腔侧的质体蓝素(PC), 还原基质侧的铁氧还蛋白(Fd) (
Redox and ATP control of photosynthetic cyclic electron flow in Chlamydomonas reinhardtii (I) aerobic conditions
4
2010
... 活化光诱导的P700氧化还原动力学是将暗适应的藻类或高等植物进行光-暗诱导, 以此确定P700+的氧化程度、P700的氧化速率和P700+再还原速率(
... 活化光诱导的P700氧化还原动力学(
... 620 nm的红光光强为5 μmol·m-2·s-1 (黑色)、20 μmol·m-2·s-1 (红色)、50 μmol·m-2·s-1 (绿色)、200 μmol·m-2·s-1 (蓝色)、500 μmol·m-2·s-1 (紫色).莱茵衣藻野生型加入10 μmol·L-1二氯苯基二甲脲(DCMU)和1 mmol·L-1羟胺(HA).
... 根据活化光打开后P700达到2/3稳定氧化态时的时间倒数可计算PSI的天线大小, 在不同生长光强下培养的莱茵衣藻, 其PSI的天线大小并未发生变化(
The structure of a plant photosystem I supercomplex at 3.4 ? resolution
1
2007
... 光合作用是地球上进行的最大规模的化学反应.放氧光合生物吸收太阳能并裂解水分子, 释放出地球上绝大多数生命活动所需的氧气, 同时固定大气或水中的CO2并合成有机物, 为新陈代谢提供能量.在高等植物和真核藻类中, 叶绿体是光合作用的场所.叶绿体的类囊体膜上排布着4个膜蛋白复合物: 光系统II (PSII)、细胞色素b6f (Cytb6f)、光系统I (PSI)和腺苷三磷酸合酶(ATP synthase).它们紧密协作, 共同完成光能吸收、电子传递和能量转化, 最终合成腺苷三磷酸(ATP)和还原型烟酰胺腺嘌呤二核苷酸磷酸(辅酶II) (NADPH).其中, PSI主要参与光合电子传递的最终步骤, 即氧化类囊体囊腔侧的质体蓝素(PC), 还原基质侧的铁氧还蛋白(Fd) (
Plant photosystem I design in the light of evolution
1
2009
... 光合作用是地球上进行的最大规模的化学反应.放氧光合生物吸收太阳能并裂解水分子, 释放出地球上绝大多数生命活动所需的氧气, 同时固定大气或水中的CO2并合成有机物, 为新陈代谢提供能量.在高等植物和真核藻类中, 叶绿体是光合作用的场所.叶绿体的类囊体膜上排布着4个膜蛋白复合物: 光系统II (PSII)、细胞色素b6f (Cytb6f)、光系统I (PSI)和腺苷三磷酸合酶(ATP synthase).它们紧密协作, 共同完成光能吸收、电子传递和能量转化, 最终合成腺苷三磷酸(ATP)和还原型烟酰胺腺嘌呤二核苷酸磷酸(辅酶II) (NADPH).其中, PSI主要参与光合电子传递的最终步骤, 即氧化类囊体囊腔侧的质体蓝素(PC), 还原基质侧的铁氧还蛋白(Fd) (
Comparative study on the kinetics of electron transport and the slow chlorophyll fluorescence in bean leaves
1
1983
... 活化光诱导的P700氧化还原动力学是将暗适应的藻类或高等植物进行光-暗诱导, 以此确定P700+的氧化程度、P700的氧化速率和P700+再还原速率(
Pool size of electrons that can be donated to P700+ as determined in intact leaves: donation to P700+ from stromal components via the intersystem chain
1
1992
... P700氧化还原动力学不仅可以检测PSI的光合功能, 还可以检测光系统间电子载体库的大小, 其测量过程是在远红光诱导P700氧化还原动力学的基础上施加单周转饱和脉冲(single turnover flash, ST)和多周转饱和脉冲(multiple turnover flash, MT) (
Regulation of photosystem I light harvesting by zeaxanthin
1
2014
... 根据活化光打开后P700达到2/3稳定氧化态时的时间倒数可计算PSI的天线大小, 在不同生长光强下培养的莱茵衣藻, 其PSI的天线大小并未发生变化(
Acclimation of Chlamydomonas reinhardtii to different growth irradiances
1
2012
... 根据活化光打开后P700达到2/3稳定氧化态时的时间倒数可计算PSI的天线大小, 在不同生长光强下培养的莱茵衣藻, 其PSI的天线大小并未发生变化(
Electron transfer and arrangement of the redox cofactors in photosystem I
1
1997
... 在测量过程中有诸多因素会干扰测量结果的准确性.PC是含铜的水溶性蛋白质, 位于类囊体囊腔侧, 穿梭在细胞色素b6f复合体与PSI之间转运电子.当在820 nm处检测P700的吸收变化时, PC的吸收变化约占总变化的23% (
Electron transfer in photosystem I
1
2001
... 光合作用是地球上进行的最大规模的化学反应.放氧光合生物吸收太阳能并裂解水分子, 释放出地球上绝大多数生命活动所需的氧气, 同时固定大气或水中的CO2并合成有机物, 为新陈代谢提供能量.在高等植物和真核藻类中, 叶绿体是光合作用的场所.叶绿体的类囊体膜上排布着4个膜蛋白复合物: 光系统II (PSII)、细胞色素b6f (Cytb6f)、光系统I (PSI)和腺苷三磷酸合酶(ATP synthase).它们紧密协作, 共同完成光能吸收、电子传递和能量转化, 最终合成腺苷三磷酸(ATP)和还原型烟酰胺腺嘌呤二核苷酸磷酸(辅酶II) (NADPH).其中, PSI主要参与光合电子传递的最终步骤, 即氧化类囊体囊腔侧的质体蓝素(PC), 还原基质侧的铁氧还蛋白(Fd) (
Structural basis for energy and electron transfer of the photosystem I-IsiA-flavodoxin supercomplex
2
2020
... 光合作用是地球上进行的最大规模的化学反应.放氧光合生物吸收太阳能并裂解水分子, 释放出地球上绝大多数生命活动所需的氧气, 同时固定大气或水中的CO2并合成有机物, 为新陈代谢提供能量.在高等植物和真核藻类中, 叶绿体是光合作用的场所.叶绿体的类囊体膜上排布着4个膜蛋白复合物: 光系统II (PSII)、细胞色素b6f (Cytb6f)、光系统I (PSI)和腺苷三磷酸合酶(ATP synthase).它们紧密协作, 共同完成光能吸收、电子传递和能量转化, 最终合成腺苷三磷酸(ATP)和还原型烟酰胺腺嘌呤二核苷酸磷酸(辅酶II) (NADPH).其中, PSI主要参与光合电子传递的最终步骤, 即氧化类囊体囊腔侧的质体蓝素(PC), 还原基质侧的铁氧还蛋白(Fd) (
... 根据活化光打开后P700达到2/3稳定氧化态时的时间倒数可计算PSI的天线大小, 在不同生长光强下培养的莱茵衣藻, 其PSI的天线大小并未发生变化(
Electron transfer in photosystem I reaction centers follows a linear pathway in which iron-sulfur cluster FB is the immediate electron donor to soluble ferredoxin
1
1998
... P700氧化还原动力学技术的局限性主要集中在2个方面: 检测设备的灵敏性和检测过程的干扰因素.目前, 检测P700氧化还原动力学的设备, 其最高时间分辨率是10微秒.在光合电子传递链中, PC被PSI氧化大约需要10微秒, 而PSI被光激发后, 产生的电子依次传递给叶绿素分子(A0)、A1, 以及铁硫簇反应中心的FX、FA和FB, 最后传递给Fd, 整个过程在皮秒到纳秒内完成(
A thylakoid membrane protein harboring a DnaJ-type zinc finger domain is required for photosystem I accumulation in plants
1
2014
... 根据活化光打开后P700达到2/3稳定氧化态时的时间倒数可计算PSI的天线大小, 在不同生长光强下培养的莱茵衣藻, 其PSI的天线大小并未发生变化(
Photosynthetic electron transport in higher plants
1
1984
... 活化光诱导的P700氧化还原动力学是将暗适应的藻类或高等植物进行光-暗诱导, 以此确定P700+的氧化程度、P700的氧化速率和P700+再还原速率(
The kinetics of P-700+ reduction in leaves: a novel in situ probe of thylakoid functioning
1
1989
... 活化光诱导的P700氧化还原动力学是将暗适应的藻类或高等植物进行光-暗诱导, 以此确定P700+的氧化程度、P700的氧化速率和P700+再还原速率(
Changes in P-700 oxidation during the early stages of the induction of photosynthesis
1
1993
... 活化光诱导的P700氧化还原动力学是将暗适应的藻类或高等植物进行光-暗诱导, 以此确定P700+的氧化程度、P700的氧化速率和P700+再还原速率(
The use of light-induced absorbance changes at 820 nm to monitor the oxidation state of P-700 in leaves
1
1987
... 活化光诱导的P700氧化还原动力学是将暗适应的藻类或高等植物进行光-暗诱导, 以此确定P700+的氧化程度、P700的氧化速率和P700+再还原速率(
A new photosynthetic pigment, "P430": its possible role as the primary electron acceptor of Photosystem I
1
1971
... P700氧化还原动力学技术的局限性主要集中在2个方面: 检测设备的灵敏性和检测过程的干扰因素.目前, 检测P700氧化还原动力学的设备, 其最高时间分辨率是10微秒.在光合电子传递链中, PC被PSI氧化大约需要10微秒, 而PSI被光激发后, 产生的电子依次传递给叶绿素分子(A0)、A1, 以及铁硫簇反应中心的FX、FA和FB, 最后传递给Fd, 整个过程在皮秒到纳秒内完成(
Isolation of the elusive supercomplex that drives cyclic electron flow in photosynthesis
2
2010
... 活化光诱导的P700氧化还原动力学是将暗适应的藻类或高等植物进行光-暗诱导, 以此确定P700+的氧化程度、P700的氧化速率和P700+再还原速率(
... 根据活化光打开后P700达到2/3稳定氧化态时的时间倒数可计算PSI的天线大小, 在不同生长光强下培养的莱茵衣藻, 其PSI的天线大小并未发生变化(
Regulation of cyclic and linear electron flow in higher plants
1
2011
... 在测量过程中有诸多因素会干扰测量结果的准确性.PC是含铜的水溶性蛋白质, 位于类囊体囊腔侧, 穿梭在细胞色素b6f复合体与PSI之间转运电子.当在820 nm处检测P700的吸收变化时, PC的吸收变化约占总变化的23% (
Quantification of cyclic and linear flows in plants
1
2005
... 根据活化光打开后P700达到2/3稳定氧化态时的时间倒数可计算PSI的天线大小, 在不同生长光强下培养的莱茵衣藻, 其PSI的天线大小并未发生变化(
Three-dimensional structure of cyanobacterial photosystem I at 2.5 ? resolution
1
2001
... 光合作用是地球上进行的最大规模的化学反应.放氧光合生物吸收太阳能并裂解水分子, 释放出地球上绝大多数生命活动所需的氧气, 同时固定大气或水中的CO2并合成有机物, 为新陈代谢提供能量.在高等植物和真核藻类中, 叶绿体是光合作用的场所.叶绿体的类囊体膜上排布着4个膜蛋白复合物: 光系统II (PSII)、细胞色素b6f (Cytb6f)、光系统I (PSI)和腺苷三磷酸合酶(ATP synthase).它们紧密协作, 共同完成光能吸收、电子传递和能量转化, 最终合成腺苷三磷酸(ATP)和还原型烟酰胺腺嘌呤二核苷酸磷酸(辅酶II) (NADPH).其中, PSI主要参与光合电子传递的最终步骤, 即氧化类囊体囊腔侧的质体蓝素(PC), 还原基质侧的铁氧还蛋白(Fd) (
Oxidation of P700 induces alternative electron flow in photosystem I in wheat leaves
1
2019
... 在测量过程中有诸多因素会干扰测量结果的准确性.PC是含铜的水溶性蛋白质, 位于类囊体囊腔侧, 穿梭在细胞色素b6f复合体与PSI之间转运电子.当在820 nm处检测P700的吸收变化时, PC的吸收变化约占总变化的23% (
The primary electron acceptor of photosystem I
1
1973
... PSI的反应中心有1个具有光化学反应性的叶绿素a分子二聚体, 即P700 (
An improved method, using saturating light pulses, for the determination of photosystem I quantum yield via P700+-absorbance changes at 830 nm
3
1994
... P700的氧化与还原可通过700 nm (最大吸收负峰)的光吸收变化来检测, 但对叶片来说, 其光散射比较严重, 加之叶绿素荧光的干扰, 所以在700 nm处的光吸收非常复杂, 难以表征P700的氧化与还原(
... 饱和脉冲(saturation pulse, SP)是一种特殊的活化光, 光强可达40 000 μmol·m-2·s-1, 持续时间一般在1-800毫秒, 这个时间可使一个PSII反应中心发生多次电荷分离, 产生的电子足以将PSII次级电子受体PQ完全还原, PSII反应中心完全关闭.同样, SP也可暂时阻断流经PSI的电子传递.SP关闭后, 电子传递又会在极短的时间内恢复运转, 而不会对样品造成破坏, 因此SP是P700氧化还原动力学研究中常用的一种光.PSI吸收光能后用于光化学反应的能量可以用光化学量子产量表示, 它反映了PSI的光合功能, 其测量过程如
... 该方法可区分开放的PSI反应中心P700 A (b)和受体侧受限制导致的关闭PSI反应中心P700 A- (c) (
Measuring P700 absorbance changes in the near infrared spectral region with a dual wavelength pulse modulation system
1
1998
... P700的氧化与还原可通过700 nm (最大吸收负峰)的光吸收变化来检测, 但对叶片来说, 其光散射比较严重, 加之叶绿素荧光的干扰, 所以在700 nm处的光吸收非常复杂, 难以表征P700的氧化与还原(
Saturation pulse method for assessment of energy conversion in PS I
5
2008
... P700的氧化与还原可通过700 nm (最大吸收负峰)的光吸收变化来检测, 但对叶片来说, 其光散射比较严重, 加之叶绿素荧光的干扰, 所以在700 nm处的光吸收非常复杂, 难以表征P700的氧化与还原(
... 该方法可区分开放的PSI反应中心P700 A (b)和受体侧受限制导致的关闭PSI反应中心P700 A- (c) (
... , 而且Y(I)+Y(ND)+Y(NA)=1 (
... (A)利用饱和脉冲法计算PSI能量转化的原理示意图(改自
... (A) Principle of saturation pulse method for determination of efficiency of energy conversion in PSI (modified from
Light induced absorption changes in photosynthetic organisms
1
1957
... PSI的反应中心有1个具有光化学反应性的叶绿素a分子二聚体, 即P700 (
The time course of photoinactivation of photosystem II in leaves revisited
1
2012
... P700氧化还原动力学不仅可以检测PSI的光合功能, 还可以检测光系统间电子载体库的大小, 其测量过程是在远红光诱导P700氧化还原动力学的基础上施加单周转饱和脉冲(single turnover flash, ST)和多周转饱和脉冲(multiple turnover flash, MT) (
PsbP-domain protein1, a nuclear-encoded thylakoid lumenal protein, is essential for photosystem I assembly in Arabidopsis
3
2012
... 光合作用是地球上进行的最大规模的化学反应.放氧光合生物吸收太阳能并裂解水分子, 释放出地球上绝大多数生命活动所需的氧气, 同时固定大气或水中的CO2并合成有机物, 为新陈代谢提供能量.在高等植物和真核藻类中, 叶绿体是光合作用的场所.叶绿体的类囊体膜上排布着4个膜蛋白复合物: 光系统II (PSII)、细胞色素b6f (Cytb6f)、光系统I (PSI)和腺苷三磷酸合酶(ATP synthase).它们紧密协作, 共同完成光能吸收、电子传递和能量转化, 最终合成腺苷三磷酸(ATP)和还原型烟酰胺腺嘌呤二核苷酸磷酸(辅酶II) (NADPH).其中, PSI主要参与光合电子传递的最终步骤, 即氧化类囊体囊腔侧的质体蓝素(PC), 还原基质侧的铁氧还蛋白(Fd) (
... 根据活化光打开后P700达到2/3稳定氧化态时的时间倒数可计算PSI的天线大小, 在不同生长光强下培养的莱茵衣藻, 其PSI的天线大小并未发生变化(
... 根据P700氧化还原动力学并结合叶绿素荧光参数可判断PSI功能是否受到影响, 并区分这些影响是来自PSI本身、电子传递链上游的PSII、还是电子传递链间的电子递体.例如, 拟南芥ppd1突变体在820 nm处检测不到任何光吸收(
A rapid, whole- tissue determination of the functional fraction of PSII after photoinhibition of leaves based on flash-induced P700 redox kinetics
1
2008
... P700氧化还原动力学不仅可以检测PSI的光合功能, 还可以检测光系统间电子载体库的大小, 其测量过程是在远红光诱导P700氧化还原动力学的基础上施加单周转饱和脉冲(single turnover flash, ST)和多周转饱和脉冲(multiple turnover flash, MT) (
Role of cyclic electron transport in photosynthesis as measured by the photoinduced turnover of P700 in vivo
1
1976
... 活化光诱导的P700氧化还原动力学是将暗适应的藻类或高等植物进行光-暗诱导, 以此确定P700+的氧化程度、P700的氧化速率和P700+再还原速率(
Isolation of high- chlorophyll-fluorescence mutants of Arabidopsis thaliana and their characterisation by spectroscopy, immunoblotting and northern hybridisation
2
1996
... 饱和脉冲(saturation pulse, SP)是一种特殊的活化光, 光强可达40 000 μmol·m-2·s-1, 持续时间一般在1-800毫秒, 这个时间可使一个PSII反应中心发生多次电荷分离, 产生的电子足以将PSII次级电子受体PQ完全还原, PSII反应中心完全关闭.同样, SP也可暂时阻断流经PSI的电子传递.SP关闭后, 电子传递又会在极短的时间内恢复运转, 而不会对样品造成破坏, 因此SP是P700氧化还原动力学研究中常用的一种光.PSI吸收光能后用于光化学反应的能量可以用光化学量子产量表示, 它反映了PSI的光合功能, 其测量过程如
... 根据P700氧化还原动力学并结合叶绿素荧光参数可判断PSI功能是否受到影响, 并区分这些影响是来自PSI本身、电子传递链上游的PSII、还是电子传递链间的电子递体.例如, 拟南芥ppd1突变体在820 nm处检测不到任何光吸收(
Functional analysis of Photosystem I light-harve-sting complexes (Lhca) gene products of Chlamydomonas reinhardtii
1
2010
... 光合作用是地球上进行的最大规模的化学反应.放氧光合生物吸收太阳能并裂解水分子, 释放出地球上绝大多数生命活动所需的氧气, 同时固定大气或水中的CO2并合成有机物, 为新陈代谢提供能量.在高等植物和真核藻类中, 叶绿体是光合作用的场所.叶绿体的类囊体膜上排布着4个膜蛋白复合物: 光系统II (PSII)、细胞色素b6f (Cytb6f)、光系统I (PSI)和腺苷三磷酸合酶(ATP synthase).它们紧密协作, 共同完成光能吸收、电子传递和能量转化, 最终合成腺苷三磷酸(ATP)和还原型烟酰胺腺嘌呤二核苷酸磷酸(辅酶II) (NADPH).其中, PSI主要参与光合电子传递的最终步骤, 即氧化类囊体囊腔侧的质体蓝素(PC), 还原基质侧的铁氧还蛋白(Fd) (
1
2017
... 根据活化光打开后P700达到2/3稳定氧化态时的时间倒数可计算PSI的天线大小, 在不同生长光强下培养的莱茵衣藻, 其PSI的天线大小并未发生变化(
Monitoring the effects of reduced PSII antenna size on quantum yields of photosystems I and II using the Dual-PAM-100 measuring system
1
2008
... 当PSI功能受损时, Y(I)会降低, 而Y(ND)和Y(NA)也会发生相应的变化.拟南芥vte6是特异影响PSI功能的突变体, 该突变体中PSI的第2个电子受体——叶绿醌(A1)含量极低.用饱和脉冲法检测该突变体的PSI能量转化时发现突变体Y(I)比野生型低, 而Y(NA)与野生型相比则处于较高的水平, 说明在vte6突变体中, 由于A1的缺失导致A0到FX的电子传递受阻, PSI反应中心P700不能被有效地氧化, 从而产生大量的能量耗散.在vte6突变体中, Y(ND)很小, 说明PSI反应中心P700可有效接收PSII传来的电子, 并完全被还原(
Unique organization of photosystem I- light-harvesting supercomplex revealed by cryo-EM from a red alga
1
2018
... 光合作用是地球上进行的最大规模的化学反应.放氧光合生物吸收太阳能并裂解水分子, 释放出地球上绝大多数生命活动所需的氧气, 同时固定大气或水中的CO2并合成有机物, 为新陈代谢提供能量.在高等植物和真核藻类中, 叶绿体是光合作用的场所.叶绿体的类囊体膜上排布着4个膜蛋白复合物: 光系统II (PSII)、细胞色素b6f (Cytb6f)、光系统I (PSI)和腺苷三磷酸合酶(ATP synthase).它们紧密协作, 共同完成光能吸收、电子传递和能量转化, 最终合成腺苷三磷酸(ATP)和还原型烟酰胺腺嘌呤二核苷酸磷酸(辅酶II) (NADPH).其中, PSI主要参与光合电子传递的最终步骤, 即氧化类囊体囊腔侧的质体蓝素(PC), 还原基质侧的铁氧还蛋白(Fd) (
Structure of a green algal photosystem I in complex with a large number of light- harvesting complex I subunits
1
2019
... 光合作用是地球上进行的最大规模的化学反应.放氧光合生物吸收太阳能并裂解水分子, 释放出地球上绝大多数生命活动所需的氧气, 同时固定大气或水中的CO2并合成有机物, 为新陈代谢提供能量.在高等植物和真核藻类中, 叶绿体是光合作用的场所.叶绿体的类囊体膜上排布着4个膜蛋白复合物: 光系统II (PSII)、细胞色素b6f (Cytb6f)、光系统I (PSI)和腺苷三磷酸合酶(ATP synthase).它们紧密协作, 共同完成光能吸收、电子传递和能量转化, 最终合成腺苷三磷酸(ATP)和还原型烟酰胺腺嘌呤二核苷酸磷酸(辅酶II) (NADPH).其中, PSI主要参与光合电子传递的最终步骤, 即氧化类囊体囊腔侧的质体蓝素(PC), 还原基质侧的铁氧还蛋白(Fd) (
Structural basis for energy transfer pathways in the plant PSI-LHCI supercomplex
1
2015
... 光合作用是地球上进行的最大规模的化学反应.放氧光合生物吸收太阳能并裂解水分子, 释放出地球上绝大多数生命活动所需的氧气, 同时固定大气或水中的CO2并合成有机物, 为新陈代谢提供能量.在高等植物和真核藻类中, 叶绿体是光合作用的场所.叶绿体的类囊体膜上排布着4个膜蛋白复合物: 光系统II (PSII)、细胞色素b6f (Cytb6f)、光系统I (PSI)和腺苷三磷酸合酶(ATP synthase).它们紧密协作, 共同完成光能吸收、电子传递和能量转化, 最终合成腺苷三磷酸(ATP)和还原型烟酰胺腺嘌呤二核苷酸磷酸(辅酶II) (NADPH).其中, PSI主要参与光合电子传递的最终步骤, 即氧化类囊体囊腔侧的质体蓝素(PC), 还原基质侧的铁氧还蛋白(Fd) (
Redox changes of ferredoxin, P700, and plastocyanin measured simultaneously in intact leaves
1
2017
... 在测量过程中有诸多因素会干扰测量结果的准确性.PC是含铜的水溶性蛋白质, 位于类囊体囊腔侧, 穿梭在细胞色素b6f复合体与PSI之间转运电子.当在820 nm处检测P700的吸收变化时, PC的吸收变化约占总变化的23% (
Measuring P700 absorbance changes around 830 nm with a new type of pulse modulation system
4
1988
... P700的氧化与还原可通过700 nm (最大吸收负峰)的光吸收变化来检测, 但对叶片来说, 其光散射比较严重, 加之叶绿素荧光的干扰, 所以在700 nm处的光吸收非常复杂, 难以表征P700的氧化与还原(
... 发现, 用820 nm处的近红外光吸收变化可以很好地测量稳态光照下活体叶片P700的氧化状态, 而且820 nm处的光不会被PSII吸收, 因此也不会有叶绿素荧光的干扰.
... P700氧化还原动力学不仅可以检测PSI的光合功能, 还可以检测光系统间电子载体库的大小, 其测量过程是在远红光诱导P700氧化还原动力学的基础上施加单周转饱和脉冲(single turnover flash, ST)和多周转饱和脉冲(multiple turnover flash, MT) (
... ).比值的大小与植物物种以及生长的光环境相关(
Ferredoxin and flavodoxin reduction by photosystem I
1
2001
... 在测量过程中有诸多因素会干扰测量结果的准确性.PC是含铜的水溶性蛋白质, 位于类囊体囊腔侧, 穿梭在细胞色素b6f复合体与PSI之间转运电子.当在820 nm处检测P700的吸收变化时, PC的吸收变化约占总变化的23% (
Cyclic electron flow is redox-controlled but independent of state transition
2
2013
... 活化光诱导的P700氧化还原动力学是将暗适应的藻类或高等植物进行光-暗诱导, 以此确定P700+的氧化程度、P700的氧化速率和P700+再还原速率(
... 根据活化光打开后P700达到2/3稳定氧化态时的时间倒数可计算PSI的天线大小, 在不同生长光强下培养的莱茵衣藻, 其PSI的天线大小并未发生变化(
Disruption of the Arabidopsis photosystem I gene psaE1 affects photosynthesis and impairs growth
1
2000
... 饱和脉冲(saturation pulse, SP)是一种特殊的活化光, 光强可达40 000 μmol·m-2·s-1, 持续时间一般在1-800毫秒, 这个时间可使一个PSII反应中心发生多次电荷分离, 产生的电子足以将PSII次级电子受体PQ完全还原, PSII反应中心完全关闭.同样, SP也可暂时阻断流经PSI的电子传递.SP关闭后, 电子传递又会在极短的时间内恢复运转, 而不会对样品造成破坏, 因此SP是P700氧化还原动力学研究中常用的一种光.PSI吸收光能后用于光化学反应的能量可以用光化学量子产量表示, 它反映了PSI的光合功能, 其测量过程如
PsaC subunit of photosystem I is oriented with iron-sulfur cluster FB as the immediate electron donor to ferredoxin and flavodoxin
1
1998
... P700氧化还原动力学技术的局限性主要集中在2个方面: 检测设备的灵敏性和检测过程的干扰因素.目前, 检测P700氧化还原动力学的设备, 其最高时间分辨率是10微秒.在光合电子传递链中, PC被PSI氧化大约需要10微秒, 而PSI被光激发后, 产生的电子依次传递给叶绿素分子(A0)、A1, 以及铁硫簇反应中心的FX、FA和FB, 最后传递给Fd, 整个过程在皮秒到纳秒内完成(
The phytol phosphorylation pathway is essential for the biosynthesis of phylloquinone, which is required for photosystem I stability inArabidopsis.
1
2017
... 当PSI功能受损时, Y(I)会降低, 而Y(ND)和Y(NA)也会发生相应的变化.拟南芥vte6是特异影响PSI功能的突变体, 该突变体中PSI的第2个电子受体——叶绿醌(A1)含量极低.用饱和脉冲法检测该突变体的PSI能量转化时发现突变体Y(I)比野生型低, 而Y(NA)与野生型相比则处于较高的水平, 说明在vte6突变体中, 由于A1的缺失导致A0到FX的电子传递受阻, PSI反应中心P700不能被有效地氧化, 从而产生大量的能量耗散.在vte6突变体中, Y(ND)很小, 说明PSI反应中心P700可有效接收PSII传来的电子, 并完全被还原(
Photosynthetic control of electron transport in leaves of Phaseolus vulgaris: evidence for regulation of photosystem 2 by the proton gradient
1
1987
... P700的氧化与还原可通过700 nm (最大吸收负峰)的光吸收变化来检测, 但对叶片来说, 其光散射比较严重, 加之叶绿素荧光的干扰, 所以在700 nm处的光吸收非常复杂, 难以表征P700的氧化与还原(
Molecular mechanism of photosystem I assembly in oxygenic organisms
1
2015
... 光合作用是地球上进行的最大规模的化学反应.放氧光合生物吸收太阳能并裂解水分子, 释放出地球上绝大多数生命活动所需的氧气, 同时固定大气或水中的CO2并合成有机物, 为新陈代谢提供能量.在高等植物和真核藻类中, 叶绿体是光合作用的场所.叶绿体的类囊体膜上排布着4个膜蛋白复合物: 光系统II (PSII)、细胞色素b6f (Cytb6f)、光系统I (PSI)和腺苷三磷酸合酶(ATP synthase).它们紧密协作, 共同完成光能吸收、电子传递和能量转化, 最终合成腺苷三磷酸(ATP)和还原型烟酰胺腺嘌呤二核苷酸磷酸(辅酶II) (NADPH).其中, PSI主要参与光合电子传递的最终步骤, 即氧化类囊体囊腔侧的质体蓝素(PC), 还原基质侧的铁氧还蛋白(Fd) (
Tetratricopeptide repeat protein Pyg7 is essential for photosystem I assembly by interacting with PsaC in Arabidopsis
5
2017
... 光合作用是地球上进行的最大规模的化学反应.放氧光合生物吸收太阳能并裂解水分子, 释放出地球上绝大多数生命活动所需的氧气, 同时固定大气或水中的CO2并合成有机物, 为新陈代谢提供能量.在高等植物和真核藻类中, 叶绿体是光合作用的场所.叶绿体的类囊体膜上排布着4个膜蛋白复合物: 光系统II (PSII)、细胞色素b6f (Cytb6f)、光系统I (PSI)和腺苷三磷酸合酶(ATP synthase).它们紧密协作, 共同完成光能吸收、电子传递和能量转化, 最终合成腺苷三磷酸(ATP)和还原型烟酰胺腺嘌呤二核苷酸磷酸(辅酶II) (NADPH).其中, PSI主要参与光合电子传递的最终步骤, 即氧化类囊体囊腔侧的质体蓝素(PC), 还原基质侧的铁氧还蛋白(Fd) (
... 饱和脉冲(saturation pulse, SP)是一种特殊的活化光, 光强可达40 000 μmol·m-2·s-1, 持续时间一般在1-800毫秒, 这个时间可使一个PSII反应中心发生多次电荷分离, 产生的电子足以将PSII次级电子受体PQ完全还原, PSII反应中心完全关闭.同样, SP也可暂时阻断流经PSI的电子传递.SP关闭后, 电子传递又会在极短的时间内恢复运转, 而不会对样品造成破坏, 因此SP是P700氧化还原动力学研究中常用的一种光.PSI吸收光能后用于光化学反应的能量可以用光化学量子产量表示, 它反映了PSI的光合功能, 其测量过程如
... AL: 活化光(80 μmol·m-2·s-1); FR: 远红光(720 nm, 20 μmol·m-2·s-1); SP: 饱和脉冲(3000 μmol·m-2·s-1); ΔA: 活化光诱导的部分氧化的P700; ΔAmax: 最大光氧化的P700; ox.: 氧化状态的P700; red.: 还原状态的P700
... 根据P700氧化还原动力学并结合叶绿素荧光参数可判断PSI功能是否受到影响, 并区分这些影响是来自PSI本身、电子传递链上游的PSII、还是电子传递链间的电子递体.例如, 拟南芥ppd1突变体在820 nm处检测不到任何光吸收(
... 当PSI功能受损时, Y(I)会降低, 而Y(ND)和Y(NA)也会发生相应的变化.拟南芥vte6是特异影响PSI功能的突变体, 该突变体中PSI的第2个电子受体——叶绿醌(A1)含量极低.用饱和脉冲法检测该突变体的PSI能量转化时发现突变体Y(I)比野生型低, 而Y(NA)与野生型相比则处于较高的水平, 说明在vte6突变体中, 由于A1的缺失导致A0到FX的电子传递受阻, PSI反应中心P700不能被有效地氧化, 从而产生大量的能量耗散.在vte6突变体中, Y(ND)很小, 说明PSI反应中心P700可有效接收PSII传来的电子, 并完全被还原(
PPR-SMR protein SOT1 has RNA endonuclease activity
1
2017
... 根据活化光打开后P700达到2/3稳定氧化态时的时间倒数可计算PSI的天线大小, 在不同生长光强下培养的莱茵衣藻, 其PSI的天线大小并未发生变化(