HTML
--> --> -->Extreme rainfall events have caused great losses in recent years as a result of their high frequency and widespread impact, but are still a great challenge in operational forecasting. Extreme rainfall is generally defined by either the relative (95th or 99th percentiles) or absolute (daily or accumulated rainfall during the precipitation process) threshold methods. The relative method focuses on the climatology of extreme rainfall to determine a pattern (Chris et al., 2002; Knight and Davis, 2009). The absolute method is commonly used in synoptic studies. For example, a typhoon-induced extraordinary rainstorm in China with 24-h rainfall ≥1000 mm was defined as an extreme rainfall by Chen and Xu (2017), although there have been seven extreme rainfall associated with typhoons that have met this criterion since 1960. Six of these extreme rainfall occurred in Taiwan, but only one in the Chinese mainland. A maximum daily rainfall ≥50 mm is also used to define an extreme rainfall during a typhoon (hoon (Jiang et al., 2018; Qiu et al., 2019). Using this definition, the operational forecasting terms of typhoon-induced torrential rain, heavy torrential rain and extraordinary storm all refer to the extreme rainfall associated with typhoons.
As a crucial component of the Earth’s atmospheric circulation, monsoons are essential for the occurrence of rainfall. China is located in the world’s largest monsoonal climate zone, the Asian?Australian monsoon region, and therefore the Asian monsoon has a great impact on rainstorms in China (Zhao et al., 2019). Statistical and numerical studies have shown that low-level jets (LLJs) mainly consist of boundary layer jets (BLJs) and synoptic system-related LLJs (SLLJs) over southern China, which are the key factors in regulating heavy rainfall (Du and Chen, 2019a), convection initiation (Du and Chen, 2019b) and the subsequent upscale convective growth (Du et al., 2020). Chen et al. (2010) concluded that the heavy rainfall from landfalling typhoons depends upon the transport of water vapor, the extratropical transition process, land surface processes, topography, and mesoscale convective systems. The relationship between the rainfall associated with landfalling tropical cyclones and summer monsoon jets has also been investigated. Results show that the tropical cyclones causing widespread heavy rainfall are often consistently associated with a low-level jet after landfall and the water vapor flux and latent heat are significantly higher than tropical cyclones that only cause weak rainfall (Cheng et al., 2012). The summer monsoon is not constant in its intensity after onset and shows marked low-frequency oscillations. A monsoon surge occurs when the wind speed increases dramatically, followed by significant changes in the weather (Dictionary of Atmospheric Science 1994). Monsoon surge is usually defined as the band-pass filtered zonal wind at 850 hPa (Ju et al., 2005, 2007) or regional mean of total wind at 850 hPa over a specific area (Dong et al., 2010). In addition, the monsoon surge is always identified with southeast oriented cloud clusters in infrared satellite imagery in real time operational forecasting. When the southwest monsoonal is strong, a low-level jet tends to form and approach typhoons from their south side to transport sufficient water vapor, which is conducive to the formation and maintenance of heavy rainfall (Tao, 1980). Monsoon surges are crucial in rainstorms that cause flooding and provide the water vapor required for the rainstorm (Tao and Wei, 2007).
The southeastern coast of China is the most active area in the East Asian summer monsoon region, and landfalling typhoons often occur where monsoon surges and typhoons have more opportunity to interact with each other (Chen and Xu, 2017). Dong et al. (2010) reported that a southwesterly monsoonal surge can intensify the transport of water vapor to westward-moving typhoons, and that the enhanced southwesterly flow increases the convergence near the typhoon, which favors the development of ascending motion and intensifies the rainfall. Based on these studies, it is easy to associate monsoon surges with LTCER and the surges may influence the occurrence of such rainfall. Wang et al. (2010) also showed that the monsoon surge can increase the torrential rains induced by the landfalling typhoon.
Extreme rainfall, including ERLTC, has increased in frequency within the current backdrop of global warming, hence we need to improve our understanding of the causative mechanisms for these events. The main objective of this study is to conduct a dynamic composite analysis of the occurrence of ERLTC and to focus on the impact of the monsoonal surge on it. Landfalling tropical cyclones with non-extreme rainfall (NERLTC) are also analyzed.
The rest of the paper is organized as follows. Section 2 describes the data and methods. Section 3 details the water vapor flux composite and comparative analysis in terms of the vertically integrated water vapor transport (Qvt), the Qvt convergence, lateral boundary budget and the vertical distribution. Section 4 discusses the impacts of the low-level jet and steering flow associated with the monsoon surge. Our discussion and conclusions are presented in section 5.
Our study has taken more factors into consideration in defining typhoon extreme rainfall, including the number of samples and the representativeness of the tropical cyclones triggering the extreme rainfall, the differences among typhoon-induced torrential rain, heavy torrential rain and extraordinary storms, and extreme rainfall caused by typhoons on Taiwan, Hainan Island and the Chinese mainland. We defined typhoon-induced extraordinary rainstorms in China as a rainfall event in which the 24-h rainfall was ≥600 mm at a single rain gauge station. Statistically, there are 38 records of single-station typhoon extreme rainfall from 1960 to 2019, caused by a total of 26 typhoons. Among these 26 typhoons, 14 resulted in extreme rainfall in Taiwan, 5 resulted in extreme rainfall in Hainan and 7 resulted in extreme rainfall in the Chinese mainland. Seven ERLTC occurred at the same rain gauge station at Ali Mountain, Taiwan Island, which is more than 25% of all the typhoons according to our definition. These typhoons are the main object of study in this paper. We carried out comparative analysis and dynamic diagnosis of the large-scale circulations of selected tropical cyclones using dynamic composite analysis (e analysis (Li et al., 2004). The ERLTC and NERLTC were identified based on the similarities of the season of occurrence (major season, July?September), track (northwestward), and location (Ali Mountain in Taiwan Island) of the tropical cyclones. On this basis, typhoons 9608, 0908, 6312, 1307 and 0813 were selected as associated with ERLTC, whereas typhoons 9417, 1315, 0713, 0505 and 0605 were selected as associated with NERLTC. This research focuses on the precipitation induced by tropical cyclones over land. The term “landfalling tropical cyclones” refers to the landfall of either the center of the tropical cyclone or the tropical cyclone rain belt. Thus, it covers both the landfalling tropical cyclones and side-swiping tropical cyclones in the operational forecasting definition. The average daily rainfall during ERLTC and NERLTC is 1223.0 and 165.7 mm, respectively (Table 1). We are more concerned with the differences between extreme rainfall and ordinary torrential rain accompanied with landfalling typhoons, not the so-called “dry typhoon” which can only generate precipitation over 24-h≤50 mm.
Tropical cyclone number | Extremes of daily rainfall (mm) | Time at which extreme rainfall occurred (LST, LST=UTC+8) | Center of tropical cyclone | Central minimum pressure (hPa) | Maximum wind speed (m s?1) | |
ERLTC | 9608 | 1748.5 | 7-31-02:00?8-1-02:00 | (121.0°E, 24.9°N) | 960 | 40 |
0908 | 1623.5 | 8-8-20:00?8-9-20:00 | (119.8°E, 26.7°N) | 975 | 30 | |
6312 | 1248.0 | 9-10-20:00?9-11-20:00 | (121.6°E, 25.6°N) | 945 | 55 | |
1307 | 757.0 | 7-12-20:00?7-13-20:00 | (118.8°E, 26.1°N) | 985 | 30 | |
0813 | 738.0 | 9-13-20:00?9-14-20:00 | (121.3°E, 25.4°N) | 970 | 33 | |
NERLTC | 9417 | 52.7 | 8-31-20:00?9-1-20:00 | (119.7°E, 25.3°N) | 970 | 35 |
1315 | 96.5 | 8-28-20:00?8-29-20:00 | (122.3°E, 26.6°N) | 990 | 23 | |
0713 | 255.0 | 9-17-20:00?9-18-20:00 | (121.4°E, 26.2°N) | 940 | 50 | |
0505 | 350.0 | 7-17-20:00?7-18-20:00 | (121.2°E, 24.7°N) | 955 | 40 | |
0605 | 74.5 | 7-24-20:00?7-25-20:00 | (117.7°E, 24.4°N) | 985 | 28 |
Table1. Overview of selected ERLTCs and NERLTCs.
Figure 1 shows the tracks and intensity categories of the tropical cyclones during the ERLTC (Fig. 1a) and NERLTC (Fig. 1b). These tropical cyclones occurred during the peak season of tropical cyclone activity (July?September) and showed a mainly northwestward movement. All these tropical cyclones made landfall or affected Taiwan and the Chinese mainland, reaching the category of a typhoon or above before landfall. To avoid the influence of terrain and to make the tropical cyclone samples more comparable, we selected the tropical cyclones associated with ERLTC and NERLTC in Ali Mountain, Taiwan. This paper focuses on the main differences between the tropical cyclones that bring extreme rainfall and those that bring non-extreme rainfall under similar backgrounds of atmospheric circulation and the same terrain.
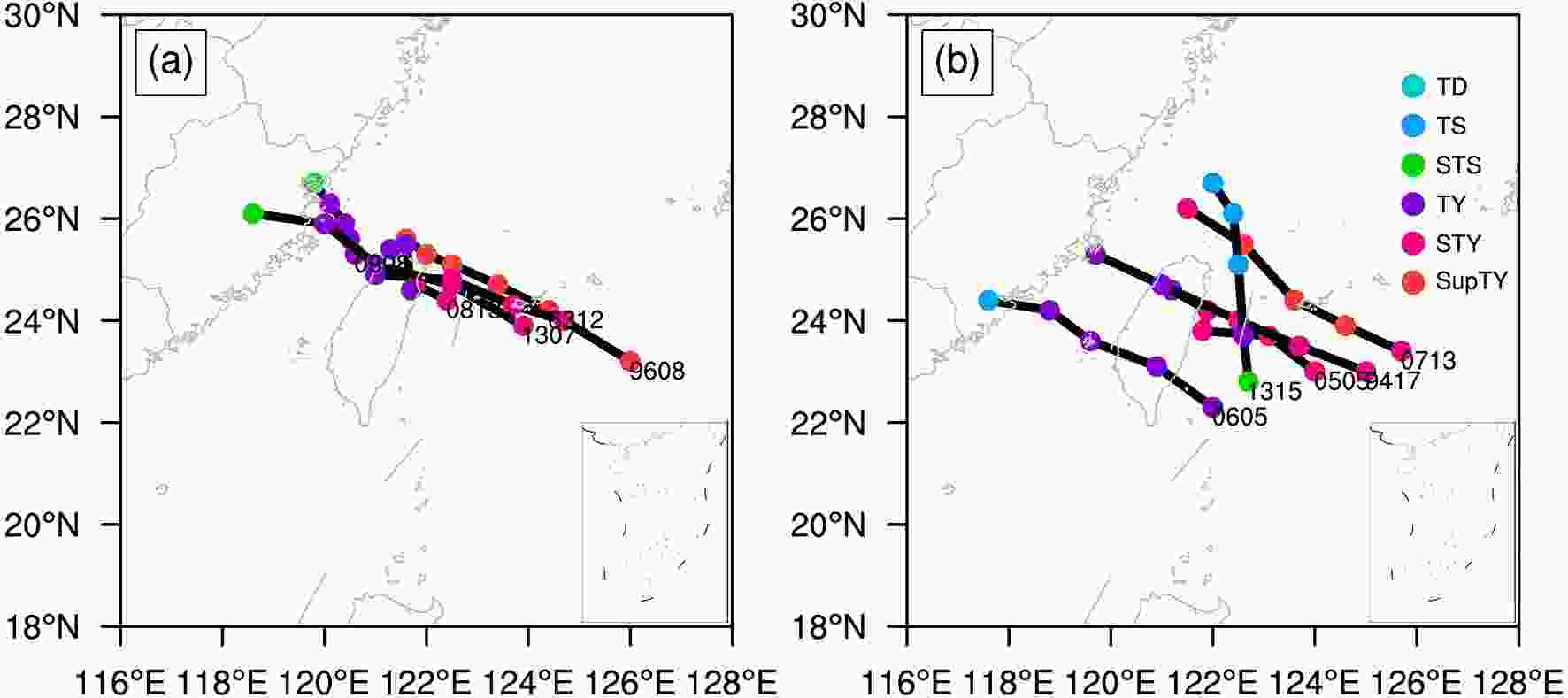
3.1. Vertically integrated water vapor transport
The vertically integrated water vapor transport (Qvt) of an air column is defined as (Ding, 1989; Chen and Huang, 2007):where







We aimed to explore the evolutionary characteristics and compare the differences of water vapor between ERLTC and NERLTC. Figure 2 shows the evolution of the area-averaged Qvt during ERLTC and NERLTC, where rectangles of different colors indicate the overall evolution of the water vapor flux of the typhoon and the surrounding environmental field as a function of the side lengths relative to the eye of the typhoon center.
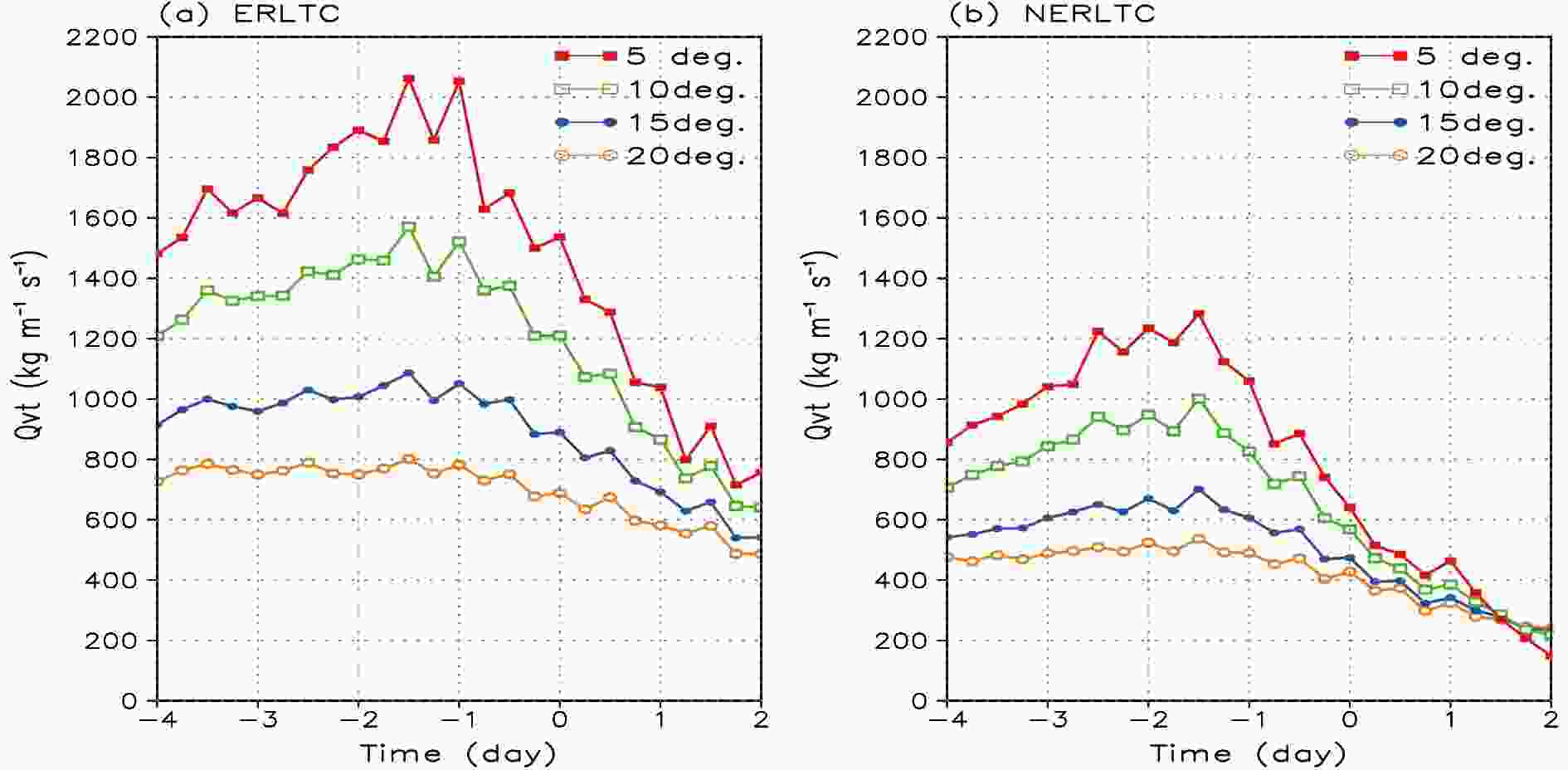
The different variation trends for the Qvt are consistent with each other. The Qvt increases continuously, reaching a maximum 1.5 days before the occurrence of extreme rainfall and then decreases rapidly. This evolutionary trend is most pronounced within the rectangles with side lengths of 5° and 10°, indicating that there are significant differences in the water vapor fluxes within the typhoon circulation before and after the occurrence of extreme rainfall. This is an indication of the occurrence of extreme rainfall. A comparison between ERLTC (Fig. 2a) and NERLTC (Fig. 2b) shows that the Qvt in the tropical cyclone circulation and the surrounding environmental field is significantly higher for the ERLTC than for the NERLTC.
Differences in the water vapor fields can result in very different intensities and distributions of rainfall, which could, in turn, determine the spatiotemporal distribution and intensity of typhoon rainstorms (Ye and Li, 2011). To further investigate the main water vapor transport channels in the tropical cyclones that trigger extreme rainfall, Fig. 3 shows the Qvt between 1000 and 300 hPa during ERLTC and NERLTC and their differences. There are two major channels for the transport of water vapor from the southwest and southeast regions for both types of tropical cyclone, but the southwestern water vapor channel of a tropical cyclone associated with the ERLTC is much broader and continuously provides water vapor for the development of the cyclone. However, the southeastern oriented water vapor channel interacts with the two types of tropical cyclones in different quadrants—in the first quadrant (northeast relative to the typhoon center) for ERLTC and in the fourth quadrant (southeast relative to the typhoon center) for NERLTC. In other words, the environmental southeastern oriented water vapor channel combines with the typhoon in its northeast (southeast) direction in ERLTC (NERLTC). Tropical cyclones associated with the ERLTC have a continuous and stable inflow of water vapor from the southeastern sector. The differences in water vapor transport (Figs. 3c, 3f, 3i and 3l) show that the southwestern channels for the transport of water vapor is powerful and long-lasting in the environmental field for tropical cyclones associated with the ERLTC. A continuous inflow of water vapor from the southwest for tropical cyclones associated with the ERLTC occurs from two days before the extreme rainfall to one day after its occurrence, intensifying the water vapor flux on the southwestern side of the tropical cyclones. Thus, a more intense southwesterly transport of water vapor in the environment is a key feature differentiating tropical cyclones associated with the ERLTC from those associated with the NERLTC.

2
3.2. Qvt divergence and decomposition terms
The Qvt can reflect the source of the water vapor for rainfall and the relationship between the transport of water vapor and the weather systems in the environment. The location and intensity of the rainfall are more closely related to the divergence of the Qvt.The


where
















Figure 4 shows the spatial distributions of the Qvt divergence and the two components (convergence and advection of water vapor) during the ERLTC and the NERLTC on the date when extreme rainfall occurred. On the day on which the extreme rainfall occurred, strong convergence of water vapor was located within 10° of the center of the tropical cyclone related to ERLTC, where there are large areas of water vapor convergence on the southwestern and southeastern sides, associated with the southwestern and southeastern water vapor fluxes, respectively (Fig. 4a). Although there is also a strong area of convergence of water vapor near the center of the tropical cyclone related to NERLTC (Fig. 4b), the corresponding intensity and range are significantly smaller than those in ERLTC. There is also an area of strong water vapor divergence within 10° northeast of the center of tropical cyclone related to the NERLTC. These characteristics are unchanged during the two days before the occurrence of extreme rainfall (figure not shown). The distribution of the water vapor flux divergence for both types of tropical cyclone is mainly determined by the wind field convergence term (Figs. 4c-f), indicating that the contribution of wind convergence to water vapor flux convergence is significantly greater than that of water vapor advection. Specifically, wind convergence is mainly within the circulation of the two types of tropical cyclones as well as the environmental fields on their southeastern and southwestern sides (Figs. 4c-d). The wind convergence is much wider and stronger within the circulation of tropical cyclones associated with ERLTC and within the environmental field on its southwestern side compared with tropical cyclones associated with NERLTC. For the water vapor advection term, wind convergence (Figs. 4e, 4f) contributes less (relatively more) to the total water vapor flux convergence in ERLTC (NERLTC). However, such difference does not have a significant effect on the total water vapor flux convergence. Therefore, tropical cyclones associated with ERLTC are associated with strong wind convergence to their southwestern and southeastern sides—that is, with the presence of stronger moisture advection in the environment—resulting in a stronger water vapor flux convergence in the circulation of the tropical cyclones. However, the wind convergence in the environment to the southwest and southeast of tropical cyclones associated with NERLTC is relatively weak and the northern side of the tropical cyclones is dominated by dry advection, making a negative contribution to the water vapor flux convergence in NERLTC.
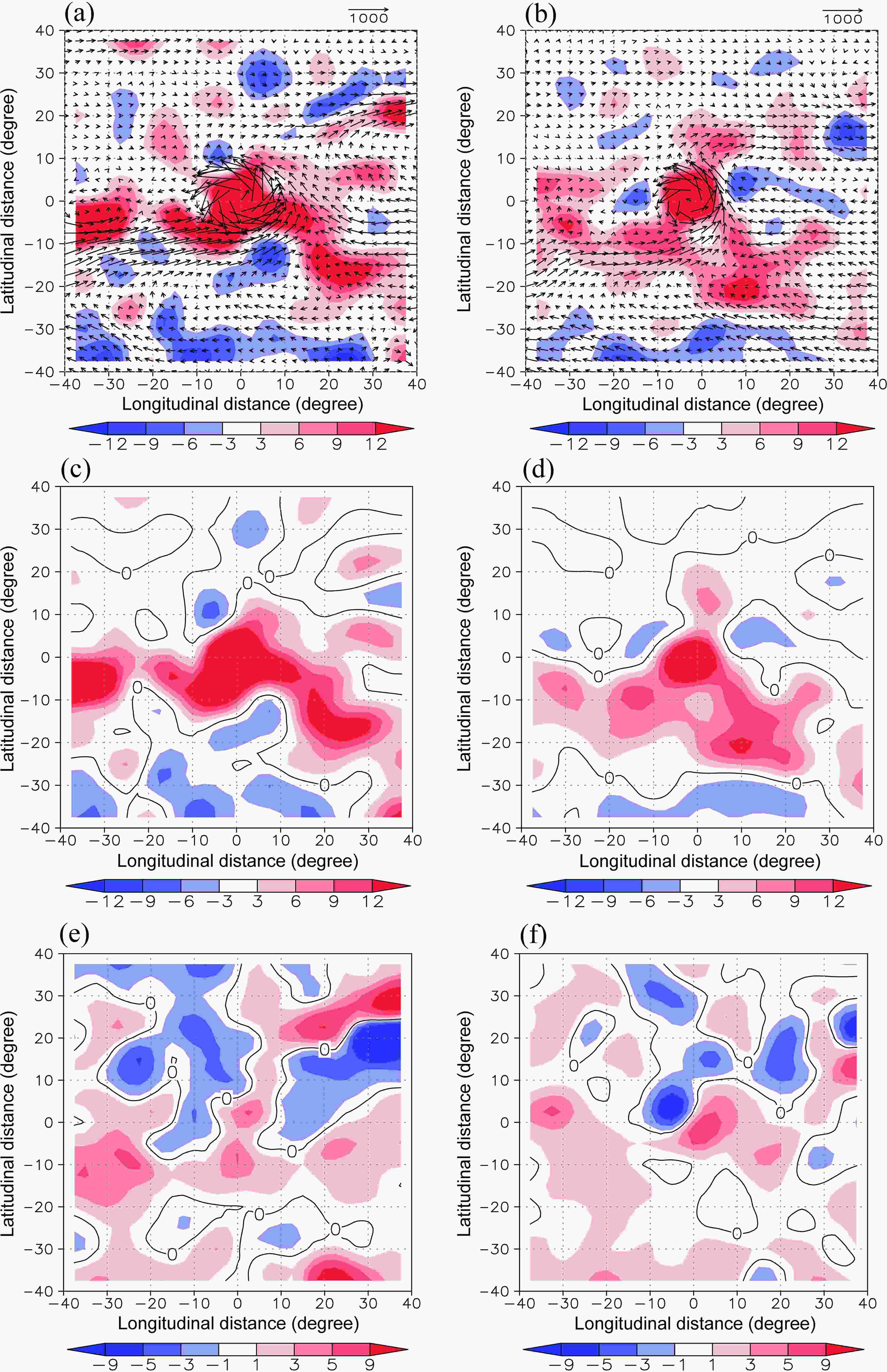
2
3.3. Qvt budget on the lateral boundaries of tropical cyclones
We used the regional average water vapor budget proposed by Ding (1989):where














The four terms on the right-hand side of Eq. (4) represent the amount of water vapor entering the selected region from the southern, northern, western and eastern boundaries, respectively, where






We investigated the evolution of the Qvt in ERLTC and NERLTC along the four boundaries (east, west, south and north) of the rectangular region with side lengths of 10° from the center of the typhoon to quantitatively determine the transport of water vapor between the environment and the tropical cyclones (Fig. 5). The water vapor flows enter through all four boundaries during ERLTC (Fig. 5a), with the largest (smallest) inflow on the southern (northern) boundary. The inflow of water vapor increases on the southern and western boundaries before the occurrence of ERLTC, reaching a peak 1?2 days before the occurrence of extreme rainfall, respectively, and then decreases rapidly once the extreme rainfall begins. However, there is no significant difference between the inflow of water vapor on the northern and eastern boundaries before and after the occurrence of extreme rainfall. Similarly, there are also water vapor inflows on the southern, western and eastern boundaries of the rectangular region for tropical cyclones associated with NERLTC. However, there is a continuous outflow of water vapor on the northern boundary before the occurrence of rainfall (Fig. 5b), which is consistent with previous findings (Ding and Liu, 1986). The inflows of water vapor on both the western and southern boundaries during ERLTC are larger than those during NERLTC, but there is no obvious difference in the water vapor budget between the two types of tropical cyclones on the eastern boundary. Therefore the inflow of water vapor on the northern boundary is an important feature that distinguishes ERLTC from NERLTC.
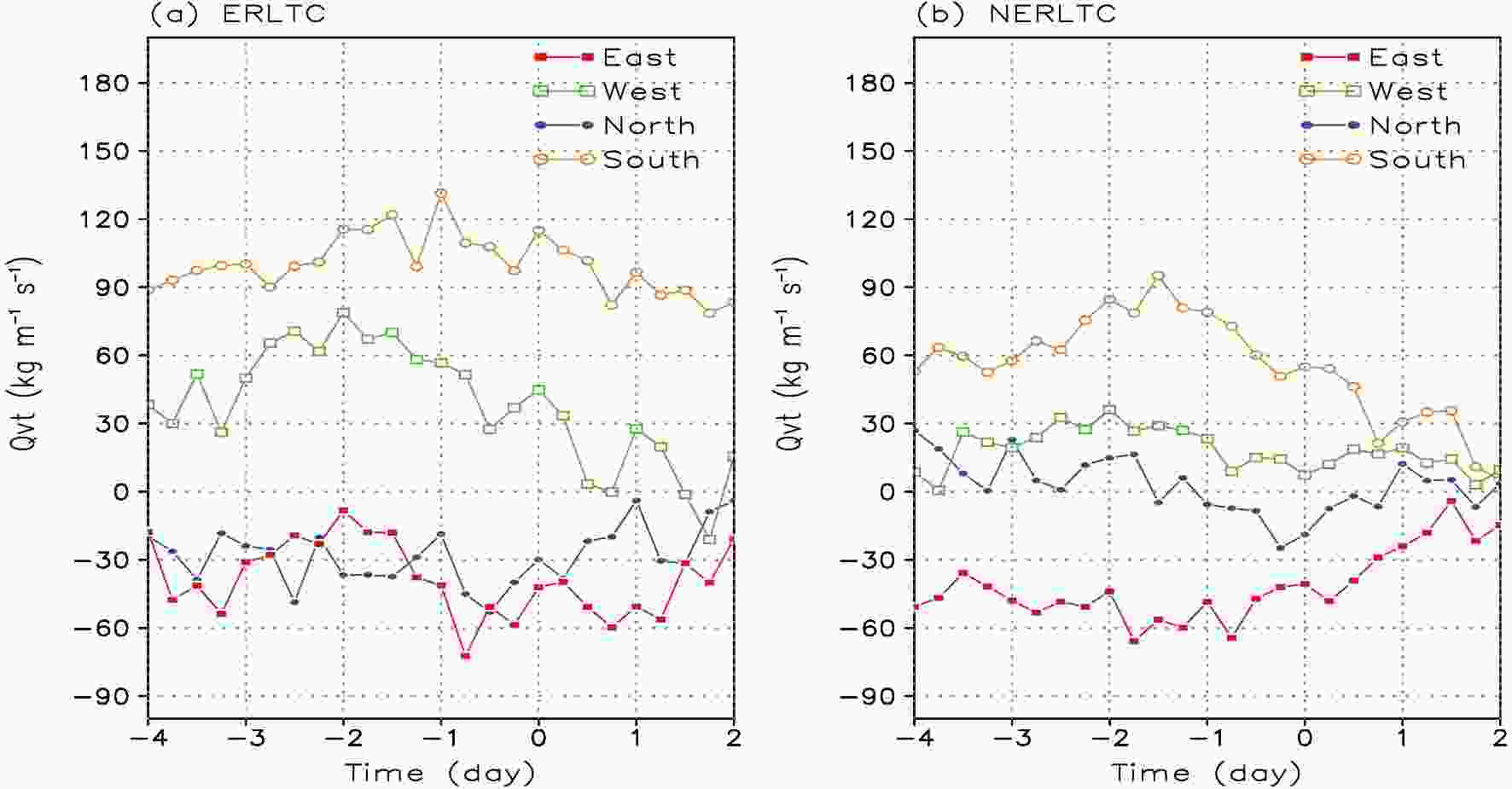
2
3.4. Vertical distribution of water vapor flux
Figure 6 shows the vertical profiles of the water vapor fluxes averaged over the rectangular area with side lengths of 10° from the center of the typhoon for the two types of tropical cyclones. These profiles were used to determine the distribution of water vapor fluxes in different layers.
The fluxes of water vapor in both types of tropical cyclones decrease with height as a result of their structural characteristics—i.e., areas with high wind speeds and large amounts of water vapor are located in the lower and middle troposphere. However, the water vapor flux is significantly higher in the tropical cyclones associated with ERLTC than those associated with NERLTC and can be almost twice as large in the same layer. The regionally averaged water vapor flux below 850 hPa in ERLTC is at a maximum from two days before the occurrence of extreme rainfall until the day on which the extreme rainfall occurs. By contrast, the regionally averaged water vapor flux in NERLTC reaches a maximum three days before the occurrence of rainfall and then decreases from two days before the occurrence of rainfall. Combined with the results for the Qvt (Fig. 3), this shows that the lower troposphere makes the highest contribution to the Qvt, and therefore the water vapor flux in the lower troposphere is significantly different between the ERLTC and NERLTC.
4.1. Monsoon surge and low-level jet
Our analyses show the strong convergence of Qvt during ERLTC, which is mainly caused by the strong convergence of wind. This section further explains the configuration of circulation that allows for such a strong convergence of the water vapor flux during ERLTC. Figure 7 shows the configuration of the wind and humidity fields at 850 hPa and the west Pacific subtropical high at 500 hPa for the same time period.
There is no significant difference between the humidity fields of the two types of tropical cyclones. The tropical cyclone itself and its western side are both areas of high humidity and these areas are slightly larger in regions with ERLTC. However, the configurations of the wind field are markedly different for the two types of tropical cyclone. During ERLTC, the tropical cyclones are linked to the southwestern low-level jet and interact with each other for a long time (Figs. 7a and 7c), causing the southern monsoonal trough to advance eastward and the subtropical high to retreat eastward and then move northward. This is consistent with the conclusions in Cheng et al. (2012) that the water vapor and instability energy associated with low-level jet have an important influence on landfalling typhoon induced extraordinary rainstorms. However, the tropical cyclone associated with the NERLTC does not interact with the southwestern low-level jet. The area near the tropical cyclone with high wind speeds is always to the east, mainly as a result of the strong pressure gradient between the subtropical high and the tropical cyclone.
2
4.2. Monsoon surge slows the movement of tropical cyclones
Many previous studies have documented that a slower translation speed of a tropical cyclone favors local extreme rainfall by extending the impact period of the typhoon, especially on Taiwan Island (Chien and Kuo, 2011; Su et al., 2012; Wu, 2013; Chen and Xu, 2017). Figure 8 shows the translation speed of our selected examples of ERLTC (NERLTC) and the average speed calculated using the latitudinal and longitudinal position at 6-h intervals in the best-track dataset.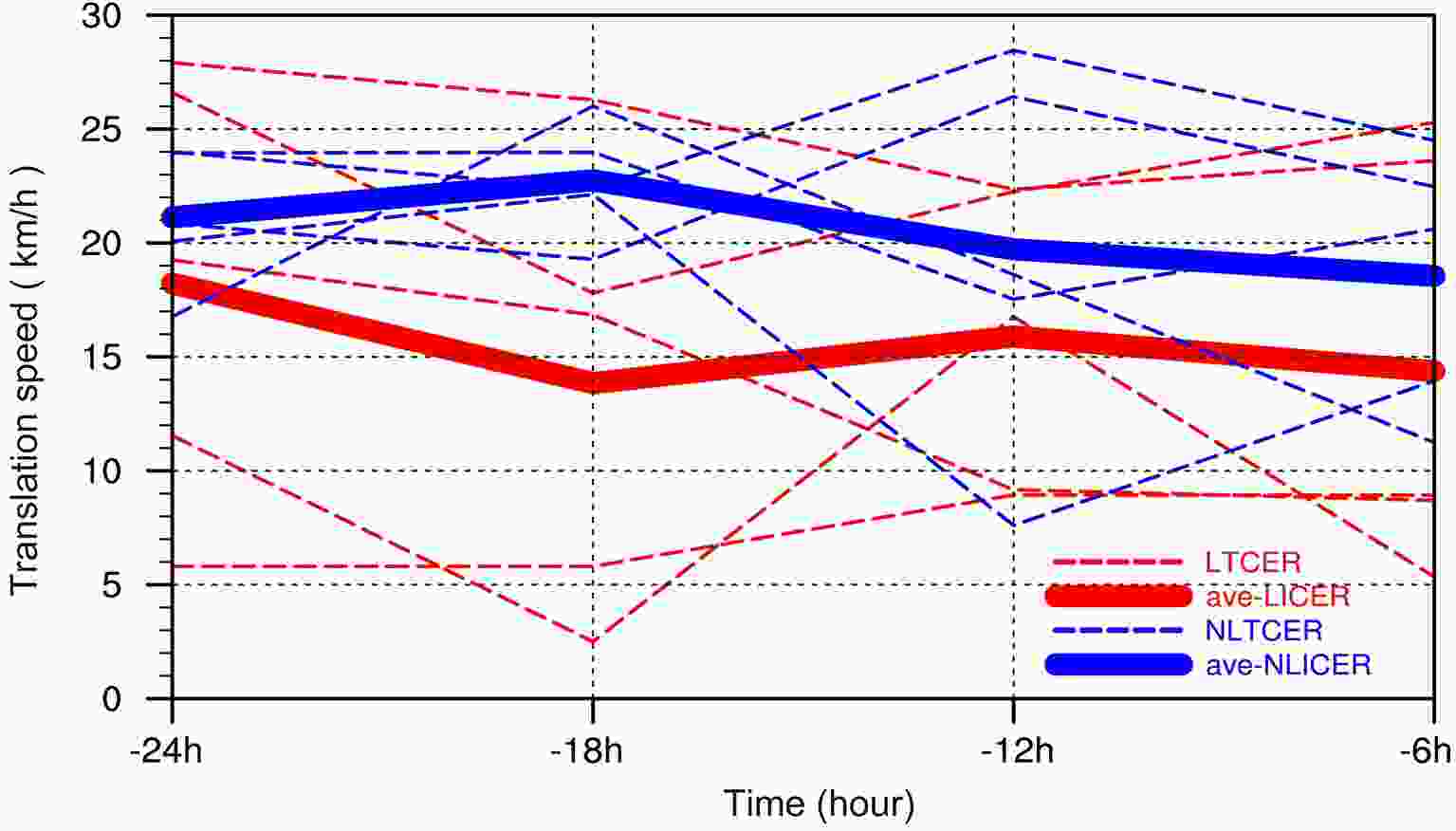
Figure 8 clearly shows that the average translation speed of the ERLTC (NERLTC) is slower (faster) during the period of extreme rainfall. Specifically, the average translation speed of the ERLTC (NERLTC) is 15.5 (20.5) km h?1. The average translation speed of the ERLTC is only 75% of the average for the NERLTC. Tu and Chou (2013) suggested that intense and long-lasting typhoon rainfall is mainly a result of this slower translation speed, which may be associated with weakening of the steering flow. Figure 9 shows the zonal component of the steering flow of ERLTC and NERLTC at each level between 1000 and 200 hPa and the whole-layer zonal mean component of the steering flow. Before the occurrence of extreme rainfall, the direction of the zonal steering flow in ERLTC and NERLTC were similar at the middle and higher levels, but were different in magnitude. The tropical cyclones associated with ERLTC had a much stronger westward zonal steering flow while the NERLTC had an eastward zonal steering flow in the lower troposphere. This directly affected the whole-layer zonal mean steering flow and therefore the ERLTC had a relatively weak eastward zonal mean steering flow, which contributed to the slower translation speed relative to the NERLTC.
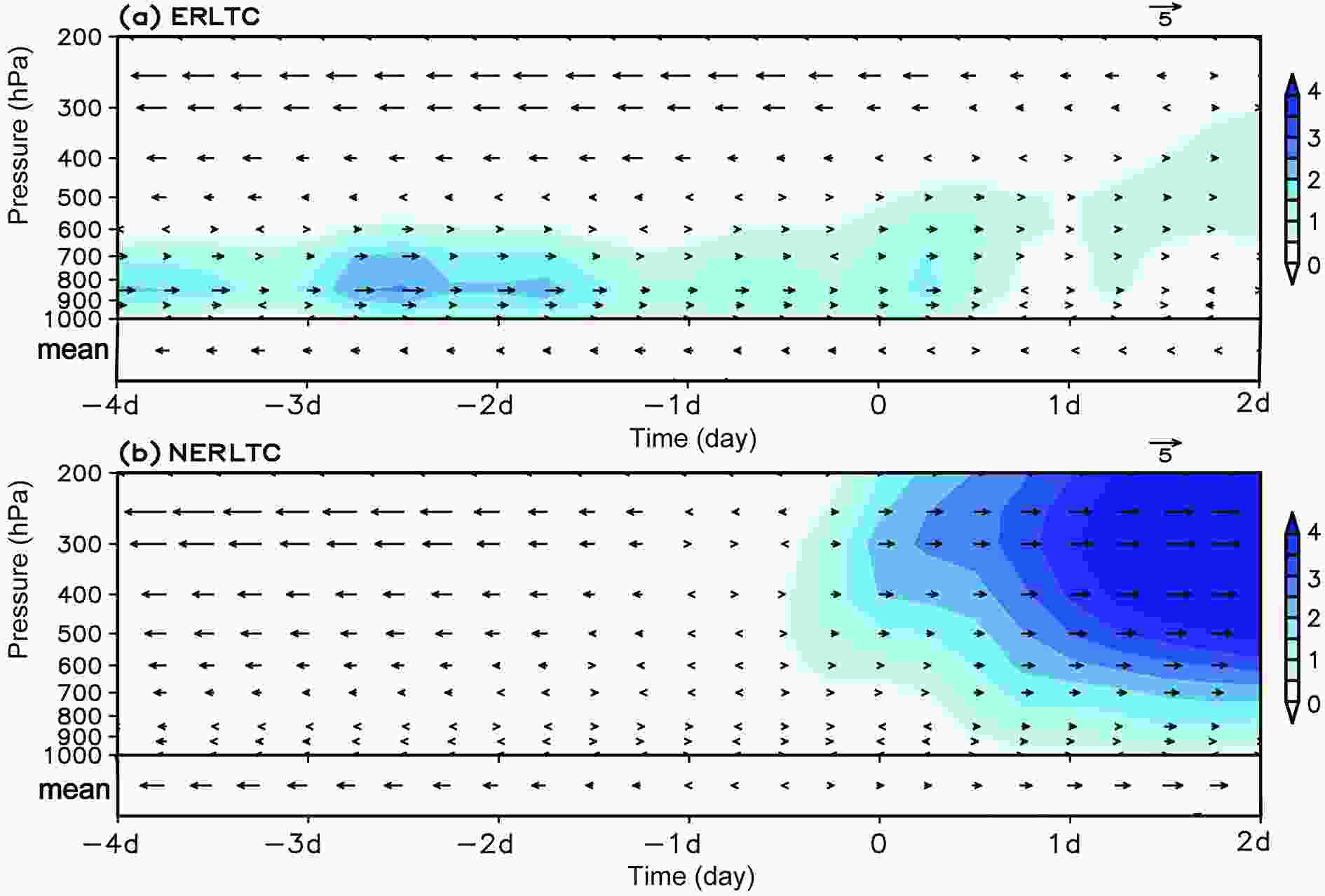
2
4.3. Dynamic monsoon surge index
Within the background of the East Asian summer monsoon, the activities of monsoonal surges are characterized by a clear increase in wind speed, possibly associated with the activity of low-level jets. Monsoon surges have previously been defined as the regional average of the zonal wind at 850 hPa in a given region (Dong et al., 2010; Hai et al., 2017). As a landfalling typhoon generally moves from east to west after formation, it is often difficult to distinguish the circulation of the typhoon itself from the monsoon surge circulation during and after the landfalling. We therefore define a dynamic monsoon surge index (
where






Figure 10b shows the evolution of the DMSI during the ERLTC and NERLTC. The DMSI clearly distinguishes ERLTC from NERLTC, with the DMSI of ERLTC being significantly greater than that of NERLTC before and after rainfall. The DMSI increases continuously three days before the extreme rainfall in ERLTC, peaks on the day on which the rainfall occurs, and then decreases rapidly. The DMSI also increases before the occurrence of rainfall in NERLTC, but with a relatively small magnitude. The DMSI proposed here indicates significance for ERLTC and could be taken as a predictor of ERLTC in operational forecasting.
(1) The Qvt peaks 1?2 days before the occurrence of the ERLTC and decreases dramatically after the occurrence of rainfall. The Qvt in the circulation of the tropical cyclone and its surrounding environmental field during the ERLTC is significantly larger than that during the NERLTC. In terms of the regionally averaged water vapor fluxes, both the circulation of the ERLTC and its environment are moister than that of the NERLTC.
(2) There is a wider and more persistent water vapor channel to the southwest of the tropical cyclone during the ERLTC than during the NERLTC. The low-level jet to the southwest of the tropical cyclone during the ERLTC is connected to the tropical cyclone for a long time and continuously enhances the cyclonic circulation of the tropical cyclone. The Qvt convergence is stronger during the ERLTC than during the NERLTC. The wind convergence term contributes the most to the environmental fields in both types of tropical cyclones, whereas the water vapor advection term has a relatively minor role. The strong wind convergence in the ERLTC is mainly caused by the low-level jet overlain by the circulation of the tropical cyclone, whereas there is no low-level jet in the environment of cyclones associated with NERLTC.
(3) The water vapor flux budget on the boundaries indicates that there is inflow of water vapor from the four boundaries 10° from the center of the tropical cyclone during the ERLTC, whereas there is a continuous outflow of water vapor on the northern boundary during the NERLTC before the rainfall occurs. Quantitatively, the inflow of water vapor is larger on both western and southern boundaries during the ERLTC than during the NERLTC, but there is little difference on the eastern boundary.
(4) The southwest monsoonal surge can decrease the zonal mean steering flow, which leads to a slower translation speed and extends the period of influence of the ERLTC. The circulation of the landfalling typhoon and the monsoon circulation can be clearly distinguished by our newly defined DMSI. The DMSI is significantly higher during the ERLTC than during the NERLTC and increases dramatically before the occurrence of ERLTC. Given its clear physical implications, the DMSI could be used as a predictor for ERLTC and could be used in operational forecasting.
Our conclusions suggest that monsoon surge activity has a significant impact on ERLTC. Previous reports of ERLTC have mainly focused on a single case (Ge et al., 2010; Chien and Kuo, 2011; Ding, 2015), whereas our findings are based on several and are an extension of previous studies concluding that low-level jets have an important influence on the formation of typhoon-induced extraordinary storms (Cheng et al., 2012). From the perspective of monsoon surges, understanding the formation and development of low-level jets and their interaction with tropical cyclones can help to improve our understanding of the mechanisms of ERLTC. Nevertheless, this study is limited to synoptic-scale analyses and more detailed processes; in particular, mesoscale, convective, and microphysical processes during the ERLTC have not been investigated as a result of the coarse resolution of the data. We are planning numerical experiments to explore this further in future studies.
Acknowledgements. This work was supported by the National Science Foundation of China (Grant Nos. 41775048, 42030611), National Basic Research Program of China (Grant No. 2015CB452804), the Open Grants of the State Key Laboratory of Severe Weather (Grant No. 2020LASW-B06). The authors thank Dr. Fumin REN for providing the TC-induced OSAT precipitation dataset over China in this study. The best-track data is from