HTML
--> --> -->The extended lake breeze includes a combination of lake breeze and valley breeze during daytime and a synthesis of land breeze and mountain breeze during nighttime (Kondo, 1990; Laiti et al., 2013a). The intensity of an extended lake breeze usually depends on the extent of the lake, the local topographical features, and the mesoscale systems (McGowan et al., 1995; Gerken et al., 2013; Wagner-Riddle et al., 2015). In mountainous lake areas, lake and mountain breezes frequently occur under weak synoptic systems (Mahrt, 2017) and are normally characterized by a sudden change in wind speed and direction (Crosman and Horel, 2016; Mahrt, 2017; Wang et al., 2017). Smaller lakes produce weaker and less well-developed extended lake breezes (Boybeyi and Raman, 1992). For lakes with a characteristic dimension larger than 80 km, the extended lake breezes are considered equivalent to sea breezes (Segal et al., 2010). For smaller lakes, the dependence of breeze intensity on the lake’s characteristic dimension is low in the late morning but high in the afternoon (Crosman and Horel, 2012).
Extended lake breezes play a significant role in atmospheric boundary layer processes in mountainous lake areas, such as the advection and convection of air flow, exchanges of heat fluxes, and evaporation and precipitation (Sturman et al., 2003; Mengelkamp et al., 2006; Williamson et al., 2009; Serafin et al., 2018). Lakes in mountain basins frequently moisten the air and decrease air temperature in summer (Pu et al., 2016). The lake acts as a source of cold air at the valley bottom, leading to stronger diurnal up-valley wind (Bergstr?m and Juuso, 2006; Laiti et al., 2013b). The interactions between lake and valley breezes could even cause clockwise or anticlockwise circulation, thereby further affecting the mixing of water masses and sedimentation (Hai et al., 2002; Filonov et al., 2015). The extended lake breeze enhances advection of cold air at night, leading to a more stable stratified atmospheric boundary layer in mountainous lake areas (Laiti et al., 2014). On the downwind side, the influence of the extended lake breeze on wind field, temperature, and humidity can be identified nearly as far as 4 km (Bischoff-Gau? et al., 2006). Previous studies have shown that daytime turbulent fluxes over a lake surface can differ from land surface fluxes by up to 200 W m?2, leading to a large thermal contrast between lake and land (Biermann et al., 2014). Lake evaporation accounts for approximately 26% of total evaporation in the Yamdrok Yumtso Lake basin (Xu et al., 2009). According to observation data from the Siling Co Lake on the Tibetan Plateau, temporal variations of evaporation are controlled mainly by the wind speed of the extended lake breeze (Guo et al., 2019). The horizontal and vertical wind associated with the interaction between lake–land and mountain–valley breezes can transport water vapor and pollutants on a large scale (Rotach et al., 2016; Lehner and Rotach, 2018; Tian and Miao, 2019).
Extended lake breezes also play a pivotal role in the transport of carbon dioxide (CO2) over heterogeneous mountainous lake areas (Lin et al., 2018; Davis et al., 2020; Han et al., 2020). On an interannual scale, lakes serve as a net source of CO2 to the atmosphere (Borges et al., 2014). The CO2 flux near the lake surface becomes smaller after sunrise (Du et al., 2018a). Formation of the lake breeze promotes CO2 flux reduction (Sun et al., 1997). The downslope flow in the mountainous area is usually a positive contributor to CO2 advection at night, induced by the presence of terrain composed of forest, mosaic trees, and shrubs upwind (Arrillaga et al., 2019). An extended lake breeze can transport CO2 and airborne pollutants further than “pure” lake or valley breezes could alone (Pérez-Landa et al., 2007a).
So far, characteristics of extended lake breezes and their impacts on energy and carbon fluxes have rarely been analyzed (Nordbo et al., 2011; Alcott et al., 2012; Jeppesen et al., 2016). This lack of statistical features is the major motivation for this study. In addition, most research has focused on case studies occurring over a short period, meaning long-term statistical analyses are still rare (Gerken et al., 2014; Jiménez and Cuxart, 2014). Analysis of these issues has been performed using long-term observation data from an eddy covariance (EC) site at Erhai Lake in the Dali Basin, southwest China. The objectives of this study are to clarify the characterization of daytime and nighttime breeze events in the Dali Basin (an area that includes the Cangshan Mountain range and Erhai Lake) and the effects of these breeze events on energy and carbon fluxes. This paper is organized as follows: the observational site and methodology used to detect breeze events are presented in section 2; Section 3 describes the characteristics of daytime and nighttime breeze events; the effects of breeze events on the sensible heat flux (Hs), latent heat flux (LE), and CO2 flux (

2.1. Site and data
The Dali Basin is located in the Dali Bai Autonomous Prefecture, Yunnan Province, southwest China. The terrain of this basin is high in the northwest and low in the southeast. The average altitude is 2,090 m above sea level (ASL). The Cangshan Mountain range lies in the west of the Dali Basin, running from north to south with 19 peaks. The highest peak is 4,122 m ASL. In the middle of the basin is Erhai Lake (100°05′–100°07′E, 25°35′–25°58′N, 1,972 m ASL), which is 42 km long and 9 km wide on average. The area of Erhai Lake is more than 250 km2. The average (maximum) depth of the lake is 10 m (20.7 m). The lake is free of ice year-round (Feng et al., 2016). Its water quality is moderately eutrophic. It mainly receives water from 19 streams from the Cangshan Mountains in the west, the Miju River in the north, and the Boluo River in the southeast. The Xier River in the southwest is the only outlet. Land use around the lake is mainly cropland. The Yuan Mountain (2,200 m ASL) is situated to the east of Erhai Lake. In the northeast of the basin is the Jizu Mountain (3,200 m ASL). The basin has extremely complex topography, with large altitude differences and heterogeneous land surfaces (Xu et al., 2016).The EC observation site is located in Erhai Lake (Fig. 1). It is nearly 80 m from the lake’s west shore and 7 km from the east shore. A ?xed platform had been established here since June 2011. The EC measurements are complemented by an open-path CO2/H2O gas analyzer (LI-7500, LICOR, USA), sonic anemometers (CSAT3A, Campbell, USA), and radiation (CNR4, Kipp & Zonen, Netherlands) and photosynthetic active radiation (LI190SB, LICOR, USA) sensors. The EC measurement frequency is 10 Hz. Air temperature, relative humidity (HMP45C, Campbell, USA), wind speed (010C, Campbell, USA) and wind direction are measured at a height of 2 m (020C, Campbell, USA). The 10 Hz raw data were processed using the EddyPro software, Version 6.1. Spikes due to instrument malfunction, weather, and physical noise were removed (Vickers and Mahrt, 1997). A double rotation was applied to align the x-axis into the local streamline and turn the vertical wind speed to be zero (Kaimal and Finnigan, 1994). The 30-min Hs, LE and





The variables used in this study were obtained from the EC site and are as follows: wind speed (ws), wind direction (wd), downward solar radiation (K↓), sensible heat flux (Hs), latent heat flux (LE), CO2 flux (

2
2.2. Determination of breeze events
To analyze the extended lake breezes systematically, daytime and nighttime breeze events are determined according to Arrillaga et al. (2016) and Román-Cascón et al. (2019). The determination of breeze events has four steps, which are as follows:Step 1: Detect days with weak synoptic scale systems. Days with synoptic wind speed at 700 hPa being lower than 9 m s?1 are selected. The ECMWF ERA-Interim data is used to select these days.
Step 2: Detect days without cold fronts. The passage of cold fronts is usually associated with a decrease of equivalent potential temperature. Among days selected in step 1, days with equivalent potential temperature variation at 700 hPa being greater than ?1.45 K in 6 hours are retained. The variation of equivalent potential temperature (

where




Step 3: Detect days without precipitation. Among days selected in step 2, days with daily accumulated rainfall being less than 0.5 mm are retained. The daily accumulated rainfall is obtained from the China Meteorological Data Service Center (
Step 4: Two additional requirements are needed to detect daytime and nighttime breeze events. One is wind direction in the selected sector, and the other is a minimum persistent duration and dominant frequency of the wind. According to the geographical location of the EC site (Fig. 1), daytime breeze events are determined by wind direction from 0°–170°. Nighttime breeze events at the Dali Basin are affected by the Cangshan Mountain range and Erhai Lake and are therefore usually a combination of mountain breeze and land breeze (Xu et al., 2019). As shown in Fig. 2, nighttime breeze events can be divided into two types. Data shown in Fig. 2 is WRF (The Weather Research and Forecasting Model) simulation data. Model settings are the same as Xu et al. (2019). When the Cangshan Mountain breeze is stronger than the land breeze from the east shore of Erhai Lake, the prevailing wind direction at the EC site is southwesterly, and N1 nighttime breeze events occur (Fig. 2a). Alternatively, when the Cangshan Mountain breeze is weak, the north branch of land breeze circulation in the southern part of the lake reaches midway up its west shore. At that time, the prevailing wind direction at the EC site is southeasterly, and N2 nighttime breeze events occur (Fig. 2b). Wind sectors for N1 and N2 nighttime breeze events are 180°–350° and 90°–170°, respectively. For each breeze event detected, a minimum persistent duration of 3 hours and a frequency of dominant wind direction from the selected sector exceeding 60% are required. The required frequency of dominant wind direction from the selected sector (60%) is different to Román-Cascón et al. (2019) (80%). This is because a standard of 80% may miss the typical lake breeze circulations in summer. Lake breezes in summer usually form 1.5 hours after sunrise and end nearly 1630 LST. The duration of these lake breezes is nearly 8 hours. The EC site (25.77°N, 100.15°E, 2090 m ASL) is located in a low latitude plateau. The sunshine duration is nearly 14 hours (0620~2030 LST) in summer. A criterion of 80% (11.2 hours) is too long and cannot capture daytime breeze events. So in this study, we use a criterion of 60% in the last step.

The number of days meeting the requirements of steps 1–3 was, respectively, 309, 271, and 216. This means that 216 fair-weather days were suitable for the development of breeze events in 2015. The number of daytime breeze events in the Dali Basin was 149 and the number of N1 and N2 events was 22 and 112, respectively.
3.1. Strength of daytime and nighttime breeze events
Wind roses for daytime (Fig. 3a), N1 nighttime (Fig. 3b), and N2 nighttime (Fig. 3c) breeze events at the EC site are shown in Fig. 3. During the day, lake breezes typically blow from Erhai Lake to the foot of the Cangshan Mountain range (southeasterly wind). The mean wind direction is 137° with a mean wind speed of 2.78 m s?1. The mean wind direction for nighttime breeze events at the EC site is 236° (N1) and 135° (N2). The N2 breeze events at the EC site are normally more intense than the N1 type (3.06 m s?1 vs. 2.17 m s?1). This is partly because the N2 breezes blow from the lake surface, where roughness, and therefore friction, is much less than that of the land surface. Although both the daytime and N2 nighttime breezes blow from the lake surface, the N2 events are more intense. This is due to the fact that the daytime events are mainly lake breezes, while the N2 events are a combination of mountain and land breezes. The southeast wind of N2 breeze events is a branch of the cyclonic circulation in the south of Erhai Lake (Xu and Liu, 2015).
2
3.2. Arrival time of daytime and nighttime breeze events
Figure 4 and 5 show examples of daytime, N1, and N2 breeze events at the EC site. The representative example of the daytime breeze event starts 1.5 hours after sunrise and ends 2 hours before sunset (Fig. 4a). The lake–land breeze circulation dominates daytime breeze events at the Dali Basin. With the formation of the lake breeze after sunrise, wind direction changes from southwesterly to southeasterly and the daytime breeze event starts. At dusk, lake breeze circulation decays and gradually disappears. The downslope flow from the Cangshan Mountain range arrives at the EC site before sunset. Wind direction changes from southeasterly to southwesterly and the daytime breeze event ends. The average daytime breeze event starts at 0830 and ends at 1630 LST. The average wind direction ranges from 97°–178°.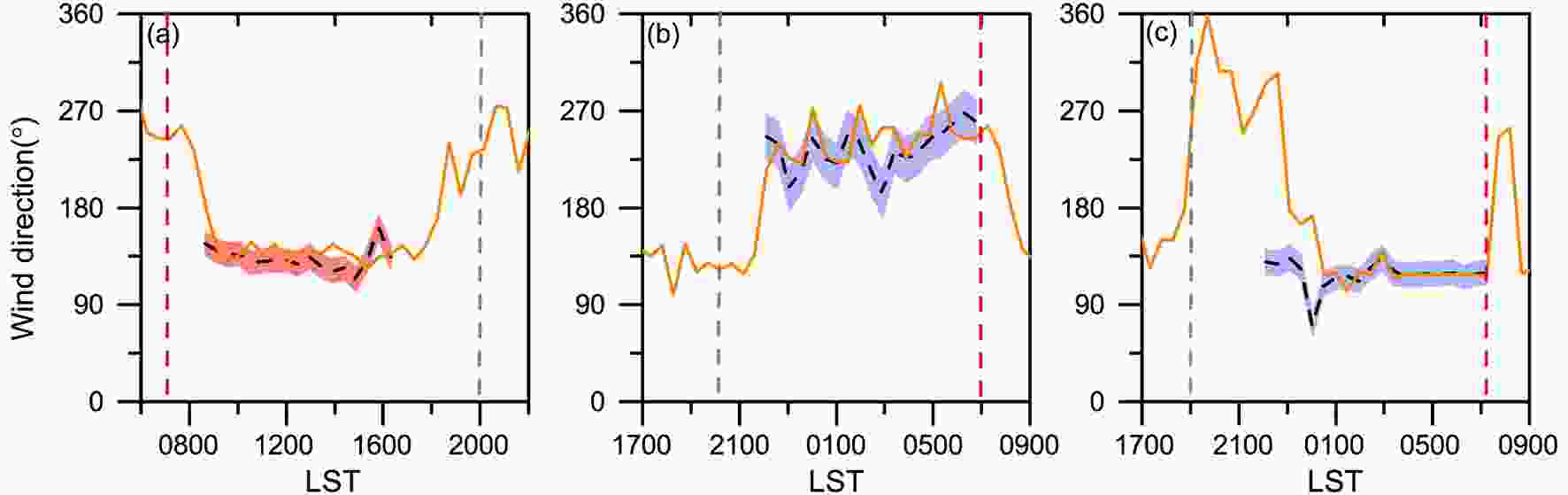

Mountain breeze circulation dominates the N1 nighttime breeze events. After sunset, mountain breeze circulation forms and develops at the foot of the Cangshan Mountain range. The N1 type breeze event begins 2.5 hours after sunset (Fig. 4b) and maintains until half an hour after sunrise. The average N1 breeze event begins at 2200 and ends at 0630 LST of the following day. The wind direction ranges between 192° and 267° with a standard deviation of nearly 12°. The N2 breeze events are mainly influenced by the land breeze circulation of Erhai Lake and mountain breeze from the Yuan Mountain. A cyclonic circulation forms at the south of Erhai Lake. When its north branch arrives at the EC site, it leads to N2 breeze events. The representative example of an N2 breeze event starts 4 hours after sunset and maintains until sunrise (Fig. 4c). The average beginning time of N2 events is 2200 LST. A southeasterly wind dominates during the entire event. The differences between N1 and N2 events result from the dominating circulation at night. When the mountain breeze from the Cangshan Mountain range is more intense, N1 events may form. When the cyclonic circulation in the southern part of the lake develops and maintains, its northern branch contributes to the formation of N2 events.
At the beginning of daytime breeze events, wind speed increases by nearly 1.3 m s?1 (Fig. 5a). The horizontal thermal contrast between lake and land could reach up to 3.5°C (Xu et al., 2019). A large thermal contrast between lake and land leads to a pressure-gradient force, which enhances the wind field (Harris and Kotamarthi, 2005). Roughness of the lake surface is less than land surface, which contributes to the higher wind speed. However, N1 events decrease wind speed from 3 m s?1 to nearly 2 m s?1 (Fig. 5b). During the afternoon transition, a large thermal difference between mountain slope and valley enhances the strength of the mountain breeze. A sharp decrease appears after that, as the thermal difference becomes smaller. The average wind speed for N1 events ranges from 1.1 m s?1 to 2.4 m s?1, which is almost 1.3 m s?1 weaker than N2 events. This means that Erhai Lake increases wind speed by at least 1.3 m s?1, which is similar to the results found in southern Manitoba, where the lake breeze increases wind speed by an average 1 m s?1 (Curry et al., 2017).
2
3.3. Duration of daytime and nighttime breeze events
The mean duration for daytime, N1, and N2 breeze events is 8, 8.3, and 8.7 hours, respectively (Fig. 6). The duration of each event depends mainly on sunlight hours (Román-Cascón et al., 2019). In general, the lake breeze circulation forms 1–2 hours after sunrise and decays a few hours before sunset. The lake breeze usually arrives at the EC site 1 hour after sunrise (Fig. 7a). This is similar to the Ora del Garda wind in the Italian Alps, for which onset usually occurs nearly 3 hours after sunrise with cessation around sunset (Giovannini et al., 2015). The mountain breeze from the Cangshan Mountain range usually arrives at the EC site 1.5 hours before sunset (Fig. 7b). As a result, nighttime events persist for longer than daytime events. The N2 events form very close to the time of sunset, although in some cases they can form 2 or 3 hours later (Fig. 7c) depending on the formation of cyclonic circulation at the southern part of the lake. The cyclonic circulation could maintain until sunrise, leading to a longer duration of N2 events compared to the other two types.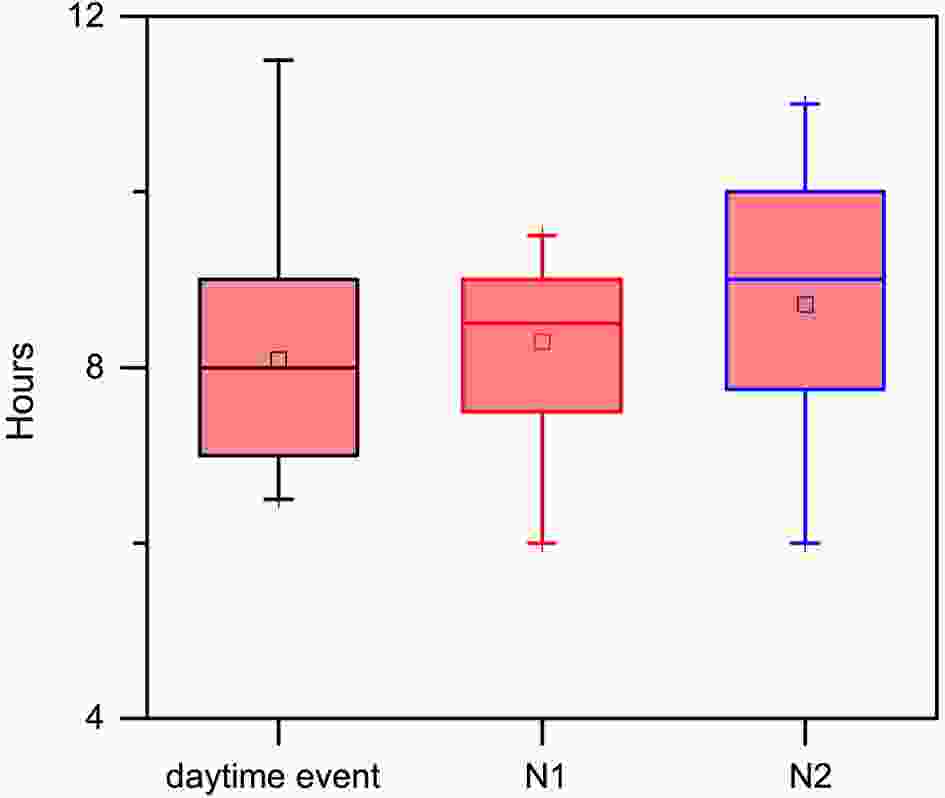
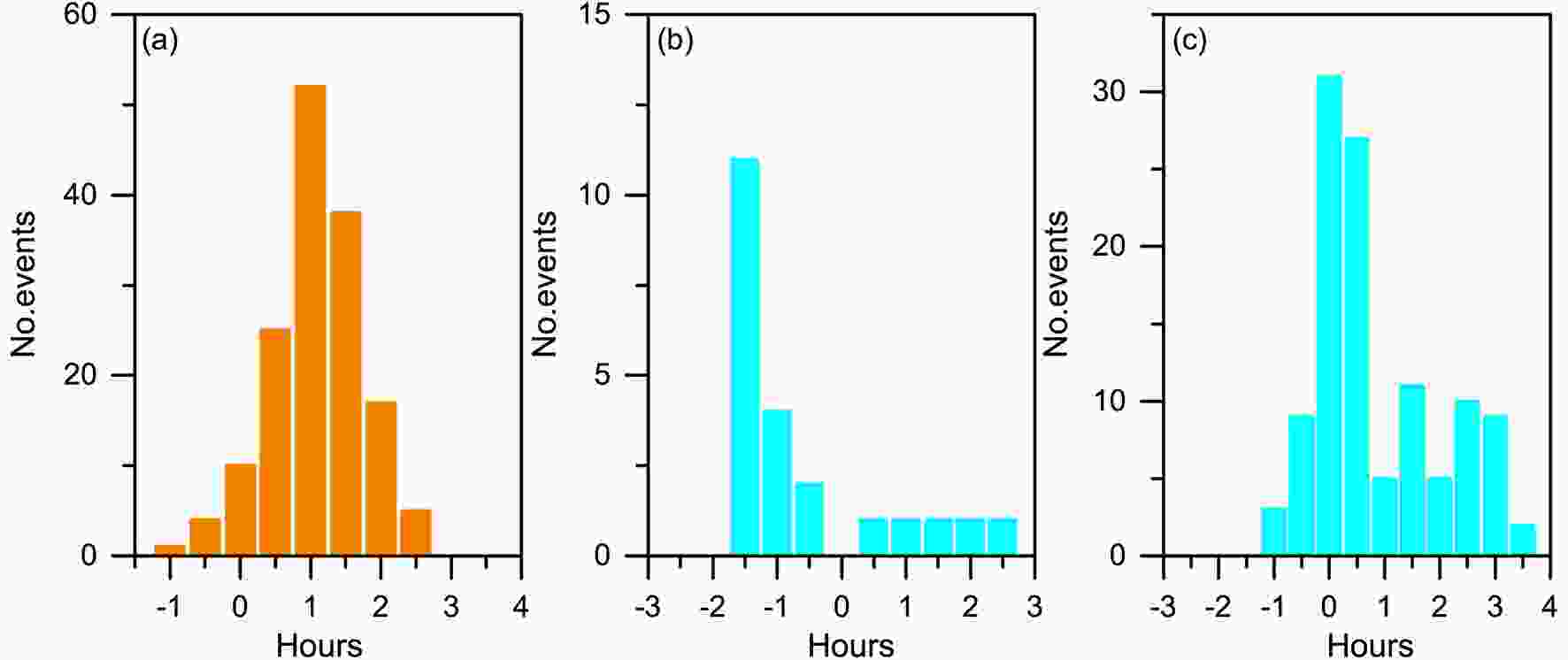
4.1. Impacts of breeze events on energy fluxes
The Hs anomalies for daytime, N1, and N2 breeze events are presented in Fig 8. The Hs anomaly is calculated by subtracting the annual average diurnal cycle from the events-mean diurnal cycle of Hs (30-min data) in 2015. The anomalies of representative examples are indicated with green solid lines. The black dotted lines show the average anomaly for each type of event. At the beginning of daytime breeze events at the EC site, the sign of the Hs anomaly changes from positive to negative (Fig. 8a). The arrival of the lake breeze reduces Hs, with the largest negative effect at 0140 LST (6.5 W m?2 for event average, 8.2 W m?2 in the daytime breeze event example). The N1 events show a larger negative effect on Hs exchange than daytime events (Fig. 8b). After sunset, downslope flow (mountain breeze from the Cangshan Mountain range) brings cold air to the EC site, leading to lower Hs. Moreover, together with the accumulation of colder nocturnal air draining down the slope to the valley, it forms a stable atmospheric boundary layer that weakens turbulent mixing (Fernando et al., 2015). The negative effect of mean N1 events could reduce Hs by an average of 15.1 W m?2 at 0100 LST(11.2 W m?2 in the N1 event example). However, N2 events increase Hs at night (Fig. 8c). Erhai Lake is warmer than the atmosphere above (Du et al., 2018b), so it transfers heat to warm the atmosphere at night. The southeast wind from the lake surface brings warm air to the EC site, increasing Hs by an average of 12.4 W m?2 at 0200 LST (18.7 W m?2 at 0130 LST in the N2 event example).
The LE anomalies for daytime, N1, and N2 breeze events are shown in Fig. 9. The LE anomaly is calculated by subtracting the annual average diurnal cycle from the events-mean diurnal cycle of LE (30-min data) in 2015. One hour after sunrise, the LE anomaly changes from negative to positive, and remains positive until sunset (Fig. 9a). The daytime breeze events increase LE by an average of 15.8 W m?2. The lake breeze increases the specific humidity and vapor pressure (Naor et al., 2017). In the representative example of a daytime event, a maximum value of 38.2 W m?2 appears at 1200 LST, coinciding with greater wind speed (2.29 m s?1) and vapor pressure difference (increased by 0.16 kPa, Fig. 10a). On average, N1 events decrease LE by 46.6 W m?2 at 0230 LST (34.8 W m?2 at 0130 LST in the N1 example). The mountain breeze brings dry air to the EC site, resulting in smaller vapor pressure difference (decreased by 0.16 kPa at 0230 LST in the average N1 events, 0.15 kPa at 0130 LST in the N1 example, Fig. 10b) and LE. N2 events increase LE by an average 34.7 W m?2. The average N2 event reaches its largest positive effect of 58.3 W m?2 at 0230 LST (59.7 W m?2 at 0130 LST in the N2 example). N2 events bring moist air to the EC site, leading to greater vapor pressure difference (increased by 0.39 kPa at 0230 LST on average for N2 events, 0.36 kPa at 0130 LST in the N2 example, Fig. 10c) and enhancement of LE. Although daytime breeze events and N2 nighttime breeze events both increase LE, the positive effect of N2 events is nearly twice as large. This is because wind speed and water vapor pressure difference are greater during N2 events than during daytime events.
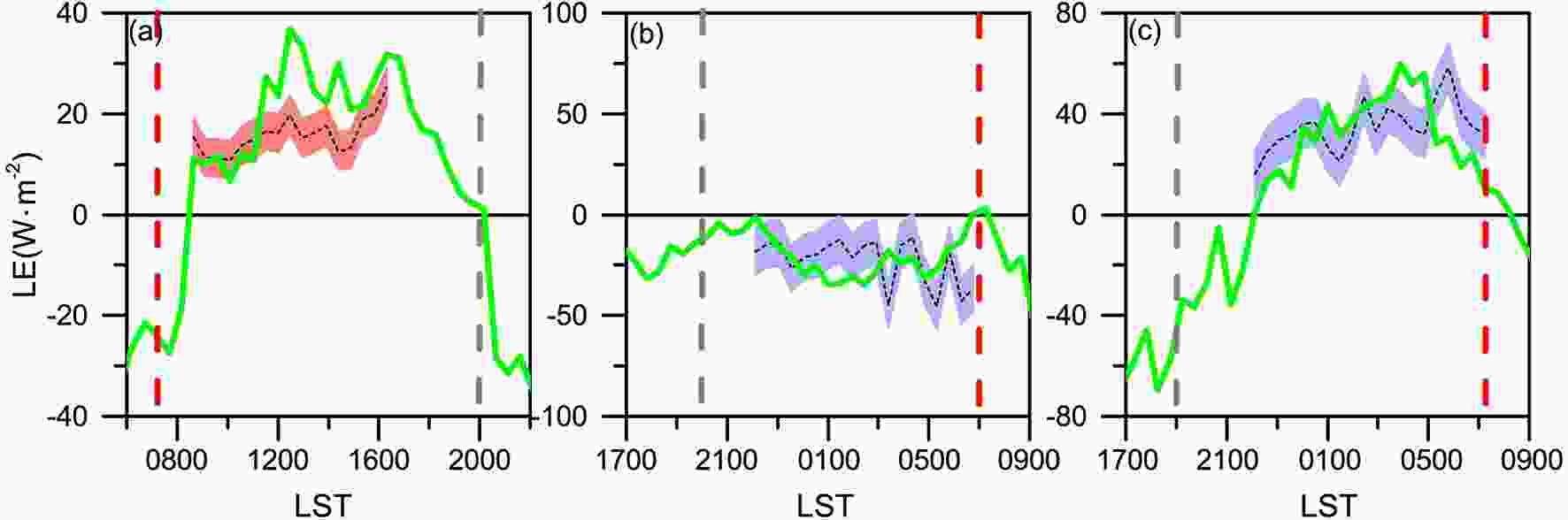

2
4.2. Impacts of breeze events on carbon dioxide flux
The
























Associated with the formation of nighttime mountain-breeze circulation, the mean N1 events increase


























































Influenced by the Cangshan Mountain range and Erhai Lake, the locality at the Dali Basin is characterized by a combination of mountain–valley and lake–land breeze circulations, leading to extended lake breezes. To analyze these extended lake breezes systematically, three types of breeze events (daytime, N1, and N2) are defined according to Arrillaga et al. (2016) and Román-Cascón et al. (2019). Due to the interactions between the mountain breeze and the land breeze, nighttime events have been divided into two types (N1 and N2 breeze events). At the EC site, daytime breeze events occur more frequently than nighttime breeze events. The number of detected daytime, and N1 and N2 nighttime breeze events is 149, 22, and 112, respectively. Daytime and N2 breeze events are dominated by the southeast wind, while N1 breeze events are mainly dominated by the west wind. Wind speed of daytime breeze events is slightly higher than N1 events, but lower than N2 events. Since the lake surface is smoother than land surface, wind speed is higher when it blows from the lake.
Nighttime breeze events last longer than daytime events. Event duration is controlled mainly by sunlight hours and the development of local circulation. The lake breeze usually forms 1–2 hours after sunrise, and then daytime breeze events begin. With the decay and disappearance of the lake breeze circulation, daytime breeze events end a few hours before the evening transition. With the arrival of the mountain breeze, N1 nighttime breeze events generally start around 1.5 hours before sunset. N2 breeze events begin during evening transition or half an hour later with the assistance of cyclonic circulation in the southern part of the lake.
The influence of daytime and nighttime breeze events on energy and carbon dioxide exchange processes at the Dali Basin has also been analyzed. Daytime breeze events decrease Hs by an average of 6.5 W m?2. N1 breeze events reduce Hs by an average of 15.1 W m?2, while N2 events increase Hs by 12.4 W m?2. The LE anomaly is positive during daytime breeze events. Coinciding with large wind speeds and wet air masses, the lake breeze increases LE exchange by 38.2 W m?2. For N1 events, the mountain breeze decreases LE by 46.6 W m?2. With the assistance of the southeast wind from the lake surface, N2 events increase Hs by 58.3 W m?2. The lake decreases




These results demonstrate several unique features of daytime and nighttime breeze events at the Dali basin based on one year’s dataset. Longer data recording would be helpful to quantify the general characteristics of breeze events in this mountainous lake area. Moreover, field experiments in the horizontal and vertical direction are needed to further investigate energy and carbon dioxide exchange at different temporal and spatial scales. The role of advection and convection in turbulence and carbon transportation will be investigated in future studies.
Acknowledgements. This study was supported by funds from the National Key Research and Development Program of China (Project no: 2017YFC1502101) and the National Natural Science Foundation of China (Projects no: 41775018, and 41805010).