


Damage of AsA-GSH Cycle of Soybean Leaves Under Waterlogging Stress at Initial Flowing Stage and the Mitigation Effect of Uniconazole
WANG ShiYa


通讯作者:
责任编辑: 杨鑫浩
收稿日期:2020-03-14接受日期:2020-05-12网络出版日期:2021-01-16
基金资助: |
Received:2020-03-14Accepted:2020-05-12Online:2021-01-16
作者简介 About authors
王诗雅,E-mail:

摘要
关键词:
Abstract
Keywords:
PDF (863KB)元数据多维度评价相关文章导出EndNote|Ris|Bibtex收藏本文
本文引用格式
王诗雅, 郑殿峰, 项洪涛, 冯乃杰, 刘雅, 刘美玲, 靳丹, 牟保民. 初花期淹水胁迫对大豆叶片AsA-GSH循环的损伤及烯效唑的缓解效应[J]. 中国农业科学, 2021, 54(2): 271-285 doi:10.3864/j.issn.0578-1752.2021.02.004
WANG ShiYa, ZHENG DianFeng, XIANG HongTao, FENG NaiJie, LIU Ya, LIU MeiLing, JIN Dan, MOU BaoMin.
开放科学(资源服务)标识码(OSID):
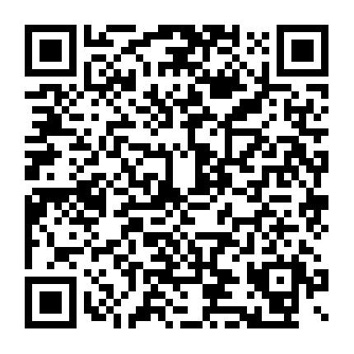
0 引言
【研究意义】大豆(Glycine max)是世界上最重要的豆科作物之一[1],在黑龙江种植较为广泛[2]。黑龙江省属典型的雨养农业种植地区,是气候变化敏感区和生态脆弱区,异常降雨频率增加,农田涝渍灾害多发,特别是7—8月正值大豆生殖生长期,对大豆生长发育构成严重威胁[3,4]。初花期(R1)是大豆的水分敏感期,也是最易受涝灾损伤的时期,研究R1期大豆涝灾损伤机制并寻求解决方案,是一个重要的现实和科学问题,意义重大。涝渍胁迫会使作物处于缺氧状态,导致作物体内活性氧的快速积累及膜脂过氧化程度加剧,抑制作物的株高、茎粗和生物量积累[5]。植物生长调节剂是一类人工合成并与植物激素相类似的活性物质,可直接调控作物本身,使作物生长发育得到定向控制,在增强作物的抗逆能力方面效果显著[6,7,8]。因此,研究淹水胁迫下喷施调节剂,对提高大豆的耐涝性具有重要意义。【前人研究进展】正常情况下,植物体内ROS水平在抗氧化酶的调节下维持在恒定水平,但在严重的逆境胁迫下,ROS的产生和清除机制将失去平衡,导致植物细胞受损[9,10]。抗坏血酸-谷胱甘肽(AsA-GSH)循环是植物体内存在的一个重要抗氧化保护系统,AsA和GSH是2种重要的抗氧化剂,参与循环中的反应,并与循环中的关键酶(APX、GR、MDHAR、DHAR)共同作用,可有效地清除逆境胁迫所产生的活性氧(ROS)和过氧化氢(H2O2)[11,12]。AsA-GSH循环作为重要的抗氧化代谢途径之一,在许多逆境胁迫下,如镉胁迫[13]、干旱胁迫[14]、盐胁迫[15]等中得到验证。但随胁迫时间的延长,作物体内的ROS清除剂能力下降,导致ROS代谢失调,ROS的过量积累导致脂质过氧化和蛋白质氧化,致使细胞完整性丧失,影响叶片的正常生长[16]。目前,植物生长调节剂的理论研究及其在生产上的应用越来越多,已成为作物优质、高产、高效栽培中的一项重要技术措施[17]。在逆境胁迫下,喷施植物生长调节剂可有效缓解逆境对作物所造成的伤害,前人已对大豆[18]、绿豆[19]、芸豆[20]和水稻[21]等作物进行了相关报道。植物生长调节剂包括促进型植物生长调节剂和延缓型植物生长调节剂等[22],其中,延缓型植物生长调节剂烯效唑(uniconazole,S3307)被广泛应用[23],S3307为三唑类植物生长调节剂,可提高植物在逆境胁迫下的抵抗能力,包括低氧[24]、高温[25]、盐胁迫[26]和干旱[27]等。项洪涛等[28]研究指出,叶面喷施S3307可通过降低MDA含量,提高保护酶活性等生理过程,抵御低温胁迫对红小豆造成的伤害。AHMED等[29]研究表明,S3307可提高植株内SOD、POD等相关保护酶活性,减少MDA的生成量和积累量提高细胞膜透性,进而增强细胞膜对抗逆境的应激防御能力。另有研究指出,S3307处理能够有效缓解盐胁迫对大豆叶片细胞膜的伤害[30]。目前,研究表明S3307可提高淹水胁迫下大豆体内抗氧化酶SOD和POD活性,降低体内膜脂过氧化物MDA含量,减少淹水胁迫对大豆造成的生理伤害[5],但关于S3307缓解淹水胁迫下AsA-GSH循环损伤的研究尚未见报道。【本研究切入点】目前,淹水胁迫对作物AsA-GSH循环的影响研究较少,且关于S3307缓解大豆淹水伤害的研究更是鲜见。【拟解决的关键问题】通过探究R1淹水胁迫对耐涝性不同的2个大豆品种叶片内活性氧和AsA-GSH循环中关键酶和抗氧化剂含量的影响,以及喷施S3307后对其调控效应,旨在揭示S3307增强大豆耐涝性的作用机理,以期为大豆的耐涝栽培提供理论依据。1 材料与方法
1.1 试验材料
选用大豆品种垦丰14和垦丰16为试验材料,其中垦丰14为耐涝品种,垦丰16为涝渍敏感品种[5,31]。供试生长调节剂选用烯效唑(S3307),由黑龙江八一农垦大学植物生长调节剂工程技术研究中心提供。
1.2 试验设计
于2019年在黑龙江八一农垦大学国家杂粮工程技术研究中心盆栽场进行盆栽试验。选用带有隔水层和排水口的树脂花盆(上口径×底径×高= 32 cm×23 cm×31 cm),试验用土按栽培土﹕腐殖土﹕沙子=7﹕2﹕1体积比例混合组成,每盆装土18 kg。5月19日进行播种,每个品种设正常水分管理(CK)、淹水胁迫处理(W)和淹水胁迫+S3307处理(W+S),采用完全随机区组设计。每盆播种10粒,子叶期定苗5株,具体设计见表1。待植株生长至R1期(播种后53 d),进行叶面喷施S3307(浓度为50 mg·L-1,喷施量为225 L·hm-2),并于喷药后第5天(播种后58 d,淹水胁迫处理第0天,记作R1+5)进行淹水处理(套盆淹水,水淹没土表面,高于土面2—3 cm为准),淹水持续5 d(淹水第5天为播种后第63天,记作R1+10),淹水胁迫处理5 d后(播种后68 d,记作R1+15)放水恢复正常水分管理。处理期间,分别对R1+5、R1+10、R1+15 3个时间点进行取样,各处理取样后立即放入液氮中,而后置于-80℃冰箱中保存,供生理指标测定。Table 1
表1
表1试验设计方案
Table 1
品种 Cultivar | 处理编号 Treatment code | 药剂处理 Pesticide treatment | 水分处理 Water treatment |
---|---|---|---|
垦丰14 Kenfeng 14 | CK | 清水喷施 Spray water | 正常水分 Normal water |
W | 清水喷施 Spray water | 淹水胁迫 Waterlogging stress | |
W+S | S3307喷施 Spray S3307 | 淹水胁迫 Waterlogging stress | |
垦丰16 Kenfeng 16 | CK | 清水喷施 Spray water | 正常水分 Normal water |
W | 清水喷施 Spray water | 淹水胁迫 Waterlogging stress | |
W+S | S3307喷施 Spray S3307 | 淹水胁迫 Waterlogging stress |
新窗口打开|下载CSV
1.3 测定项目与方法
1.3.1 MDA、H2O2和$\mathop{{O}}_{2}^{{\mathop{}_{\ ·}^{-}}}$的测定 丙二醛(malondialdehyde,MDA)含量采用硫代巴比妥酸法(TBA)测定[32]。称取0.5 g样品,加入10 mL磷酸缓冲液(0.05 mmol·L-1 PBS,pH 7.8),研磨成匀浆后转入离心管中,在6 000 r/min下离心20 min,取上清液1 mL于离心管,加入2 mL 0.6% TBA(硫代巴比妥酸)混合后在100℃沸水浴中煮15 min,用冷水迅速冷却后在4 000 r/min下离心20 min;取上清液在450、532和600 nm下测OD值。超氧阴离子产生速率(superoxide anion production rates,$\mathop{{O}}_{2}^{{\mathop{}_{\ ·}^{-}}}$)采用羟胺氧化法[33,34]进行测定。取0.5 mL提取液,加入0.5 mL PBS(0.05 mol·L-1,pH 7.8),1 mL 10 mmol·L-1盐酸羟胺溶液后摇匀,同时对照管以PBS代替样品提取液;在25℃下保温1 h,保温后需加入2 mL乙醚萃取叶绿素;依次加入1mL 17 mmol·L-1对氨基苯磺酸,1mL 7 mmol·L-1 α-萘胺,混合后涡旋,在25℃下保温20 min后3 000×g离心3 min,在530 nm下测定OD值。
过氧化氢(hydrogen peroxide,H2O2)含量采用碘化钾法[35]测定。取1 g植物样品,加入0.5 mL的0.1% TCA,在液氮下研磨,研磨后所得匀浆以19 000×g离心20 min。取上清液0.5 mL,加2 mL 1 mol·L-1 KI溶液和0.5 mL 100 mmol·L-1硫酸钾的缓冲液,暗反应1 h;反应结束后,以0.1%的TCA为参比,在390 nm下测定OD值。
1.3.2 AsA和DHA含量的测定 还原型抗坏血酸(ascorbic acid,AsA)和氧化型抗坏血酸(dehydroascorbate,DHA)采用ZHANG等[36]方法测定。称取1 g样品置于研钵中,加入5 mL的5%的偏磷酸,在4℃下研磨,所得匀浆于8 000×g离心15 min,收集上清液用于测定(AsA+DHA)和AsA的含量。取0.3 mL上清液,加入0.75 mL含5 mmol.L-1 EDTA的磷酸缓冲液(150 mmol·L-1,pH 7.4)和0.15 mL 10 mmol·L-1的二硫苏糖醇溶液(DTT)。室温下放置10 min后,加入0.15 mL 0.5% N-乙基马来酰亚胺以消除多余的DTT。加入0.6 mL的10%三氯乙酸(TCA)、0.6 mL的44%正磷酸溶液、0.6 mL的0.5% BP-乙醇溶液和0.15 mL的0.3%(W/V)FeCl3溶液。混匀后40℃水浴40 min,测525 nm处AsA+DHA的OD值。AsA的测定过程中以0.3 mL水代替DTT和N-乙基马来酰亚胺,其余操作步骤如上所述。DHA为AsA+DHA与AsA的差值。
1.3.3 GSH和GSSG含量的测定 还原型谷胱甘肽(glutathione,GSH)和氧化型谷胱甘肽(glutathiol,GSSG)含量测定采用二硫代硝基苯甲酸法[34,37]测定。取植物叶片1.0 g,加5 mL的5%偏磷酸溶液,在4℃下研磨,8 000 r/min离心25 min,上清液供测定,5℃保存。取样品母液0.2 mL,加入150 mmol·L-1磷酸二氢钠溶液(pH 7.7)2.6 mL,混匀后,加入0.2 mL DTNB溶液,在30℃保温10 min,测定412 nm 下的OD值。GSSG的测定需要在反应体系中加入0.1 mmol·L-1 2-乙烯吡啶,在27℃反应1 h,计算GSSG的含量。
1.3.4 APX、GR、MDHAR和DHAR活性的测定 过氧化氢酶(aseorbateperoxidase,APX)参考高俊凤[34]的测定方法。称取0.5 g样品,分3次加入10 mL 50 mmol·L-1预冷的磷酸缓冲液(pH 7.8),在冰浴上研磨成匀浆,转入离心管中,在4℃、12 000×g下离心20 min,上清夜即为APX粗提液。以3 mL体系测定,取0.10 mL 酶液,加入2.60 mL 含0.1 mmol·L-1 乙二胺四乙酸二钠的PBS(0.05 mol·L-1,pH 7.0),再加入0.15 mL 5 mmol·L-1的AsA,最后加入0.15 mL 20 mmol·L-1 H2O2,立即在20℃下测定290 nm下每30 sOD值的变化。
谷胱甘肽还原酶(glutathione reductase,GR)根据ZHU等[38]和WHEELER等[39]方法测定,称取0.2 g样品,分3次加入1.6 mL(0.6、0.5、0.5 mL)50 mmol·L-1预冷的磷酸缓冲液(pH 7.8),在冰浴上研磨成匀浆,转入离心管中,在4℃、12 000×g下离心20 min,上清液即为GR粗提液。取样品上清液0.1 mL,放入试管中,加入Hepes 1.7 mL,NADPH 0.1 μL及GSSG 0.1 μL,在340 nm下测定OD值。
脱氢抗坏血酸还原酶(dehydroascorbatereductase,DHAR)根据MURSHED等[40]的方法测定。称取0.8 g植株样品,在50 mmol·L-1,pH7.5的磷酸缓冲液中研磨成匀浆,定容至8 mL,4℃、12 000×g下离心20 min,提取上清液。3 mL的反应体系含有100 mmol·L-1 Hepes-KOH(pH 7.0)、2.5 mmol·L-1 H2O2、0.5 mmol·L-1 AsA及0.05 mL酶提取液,测定290 nm处OD值的变化。
APX、GR、DHAR酶活性(U·g-1·min-1)=(△A340×V0)/(0.01×V1×t×WFW)。其中,△A340为t时间内吸光值变化的代数和,V0表示酶提取液体积,V1表示测定时酶液体积,t表示为测定变化时间,WFW表示样品鲜重。以每分钟OD值变化0.01为1个酶活性单位(U)。
单脱氢抗坏血酸还原酶(monodehydroascorbate reductase,MDHAR)采用试剂盒法(Solarbio公司)。
1.4 数据处理
采用Microsoft Excel 2013进行数据录入及整理、用SPSS 25和Origin 2018进行数据统计分析及制图。2 结果
2.1 烯效唑对R1期淹水胁迫下大豆叶片膜脂过氧化程度的影响
R1+5 时,W+S处理有效降低了垦丰14和垦丰16叶片中MDA含量,但与CK相比未达显著差异水平。R1+10 时,W处理的2个大豆品种叶片MDA含量与CK相比显著增加,其中,垦丰14增加60.48%,垦丰16增加70.09%;与W处理相比,W+S处理显著降低了2个大豆品种叶片内MDA含量,其中垦丰14降低4.57%,垦丰16降低32.79%。恢复正常水分后(R1+15),2个品种W处理的MDA含量有所降低,但仍显著高于CK;W+S处理后2个品种大豆叶片恢复能力快于W处理,其中垦丰16 W+S处理恢复至CK水平,说明叶面喷施S3307能够缓解活性氧对膜脂过氧化程度的损伤,在淹水胁迫下效果显著(图1)。图1
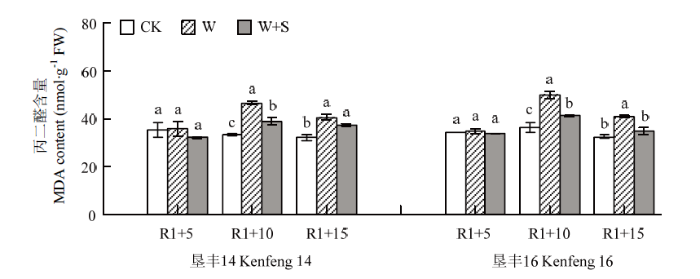
图1烯效唑对R1期淹水胁迫下大豆叶片MDA含量的影响
不同小写字母表示处理间在0.05水平差异显著。下同
Fig. 1Effects of S3307 on MDA content in leaf of soybean under waterlogging stress at R1 stage
Different small letters indicate significantly different at 0.05 probability level. The same as below
2.2 烯效唑对R1期淹水胁迫下大豆叶片$\mathop{{O}}_{2}^{{\mathop{}_{\ ·}^{-}}}$产生速率和H2O2含量的影响
R1+5时,S3307喷施抑制了2个大豆品种叶片内$\mathop{{O}}_{2}^{{\mathop{}_{\ ·}^{-}}}$产生速率和H2O2含量的积累,其中,垦丰14分别较CK显著降低10.21%和4.35%,垦丰16较CK显著降低9.36%和6.04%(图2)。R1+10 时,2个大豆品种叶片中$\mathop{{O}}_{2}^{{\mathop{}_{\ ·}^{-}}}$产生速率和H2O2含量上升趋势一致,淹水胁迫显著增加了2个大豆品种叶片内$\mathop{{O}}_{2}^{{\mathop{}_{\ ·}^{-}}}$产生速率和H2O2含量;S3307喷施可有效抑制2个大豆品种叶片内$\mathop{{O}}_{2}^{{\mathop{}_{\ ·}^{-}}}$产生速率和H2O2含量的积累,其中,垦丰14较W处理显著降低6.48%和3.65%,垦丰16较W处理显著降低18.78%和2.46%。恢复正常水分后(R1+15),2个品种W处理的$\mathop{{O}}_{2}^{{\mathop{}_{\ ·}^{-}}}$产生速率和H2O2含量明显降低,但仍显著高于CK,S3307可进一步缓解$\mathop{{O}}_{2}^{{\mathop{}_{\ ·}^{-}}}$产生速率和H2O2含量的积累,且垦丰14的降幅大于垦丰16,说明耐涝品种受淹水胁迫造成的伤害小于涝渍敏感品种,S3307对维持淹水胁迫条件下ROS代谢平衡具有积极作用(图2)。图2
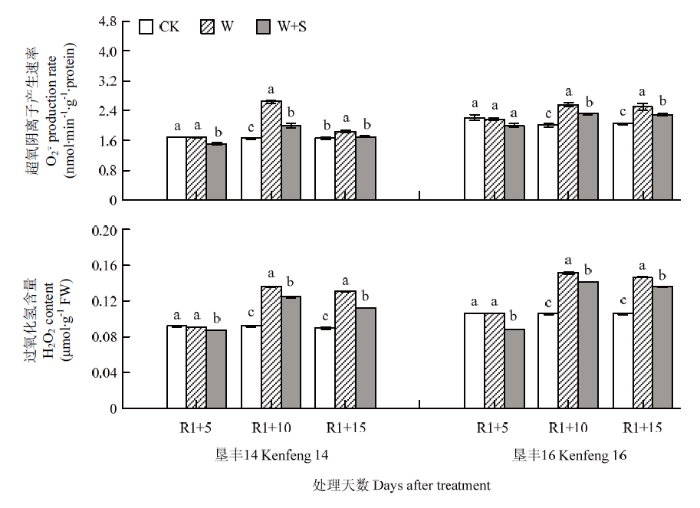
图2烯效唑对R1期淹水胁迫下大豆叶片$\mathop{{O}}_{2}^{{\mathop{}_{\ ·}^{-}}}$产生速率和H2O2含量的影响
Fig. 2Effects of S3307 on $\mathop{{O}}_{2}^{{\mathop{}_{\ ·}^{-}}}$ production rate and H2O2 content in leaf of soybean under waterlogging stress at R1 stage
2.3 烯效唑对R1期淹水胁迫下大豆叶片AsA和DHA含量的影响
R1+5时,垦丰14和垦丰16 W+S处理与各自对照CK相比,提高了2个大豆品种叶片内AsA、DHA和AsA+DHA含量。R1+10时,垦丰14和垦丰16各处理叶片的AsA、DHA和AsA+DHA含量均表现为W+S>W>CK,且差异达到显著水平。恢复正常水分后(R1+15),垦丰14和垦丰16各处理与R1+10时相比呈相反变化趋势,但垦丰14和垦丰16 AsA、DHA和AsA+DHA含量高低顺序与R1+10时相同,且各处理之间达差异显著水平。说明S3307可促进淹水胁迫下叶片内AsA、DHA和AsA+DHA含量的增加,并在恢复正常水分处理后延缓其含量的降低,以缓解淹水造成的伤害(图3)。图3
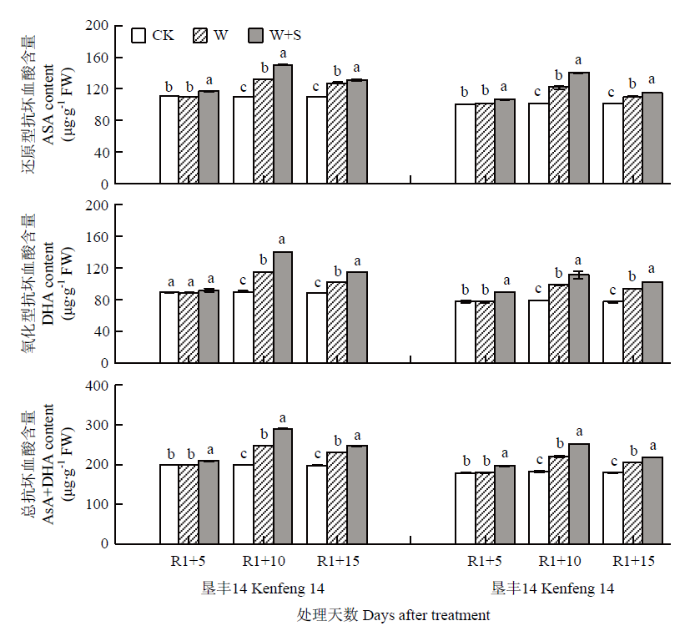
图3烯效唑对R1期淹水胁迫下大豆叶片AsA、DHA和AsA+DHA含量的影响
Fig. 3Effects of S3307 on AsA, DHA and AsA+DHA content in leaf of soybean under waterlogging stress at R1 stage
2.4 烯效唑对R1期淹水胁迫下大豆叶片GSH和GSSG含量的影响
R1+5时,垦丰14 W+S处理叶片内的GSH、GSSG和GSH+GSSG含量显著高于CK,分别较CK增加6.23%、7.80%和6.70%;垦丰16 W+S处理分别较CK增加8.65%、1.24%和6.05%,差异显著。R1+10时,垦丰14和垦丰16叶片内GSH、GSSG和GSH+GSSG含量的趋势表现为W+S>W>CK,方差分析表明各处理之间差异显著,说明S3307在淹水胁迫下可显著提高叶片内GSH、GSSG和GSH+GSSG含量。恢复正常水分后(R1+15),垦丰14和垦丰16 W和W+S处理的GSH、GSSG和GSH+GSSG含量迅速降低,但2个处理上述指标含量高低与R1+10时趋势相同,说明叶面喷施S3307具有延缓淹水胁迫下大豆叶片内GSH、GSSG和GSH+GSSG含量减少的作用(图4)。图4
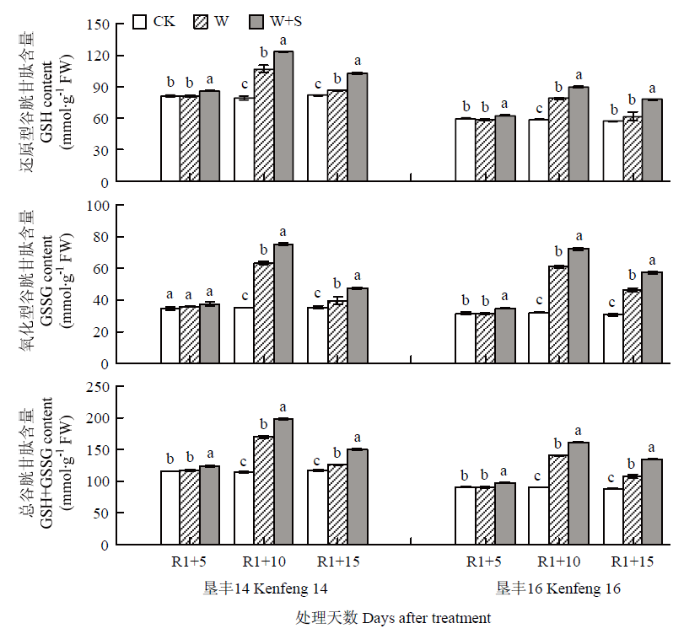
图4烯效唑对R1期淹水胁迫下大豆叶片GSH、GSSG和GSH+GSSG含量的影响
Fig. 4Effects of S3307 on GSH and GSSG and GSH+GSSG content in leaf of soybean under waterlogging stress at R1 stage
2.5 烯效唑对R1期淹水胁迫下大豆叶片关键酶活性的影响
2.5.1 烯效唑对R1期淹水胁迫下大豆叶片APX活性的影响 R1+5时,垦丰14和垦丰16 W+S处理与CK相比无显著差异。在淹水胁迫R1+10时,垦丰14和垦丰16 W处理叶片内APX活性显著增加,S3307喷施后进一步提高了垦丰14和垦丰16叶片内APX活性。恢复正常水分后(R1+15),2个大豆品种W处理的APX含量迅速降低,W+S处理延缓了APX降低,二者均显著高于CK。说明S3307可显著增加淹水胁迫下大豆叶片内APX活性并延缓其降低,有助于增强清除H2O2的能力,减轻氧化胁迫造成的伤害(图5)。图5
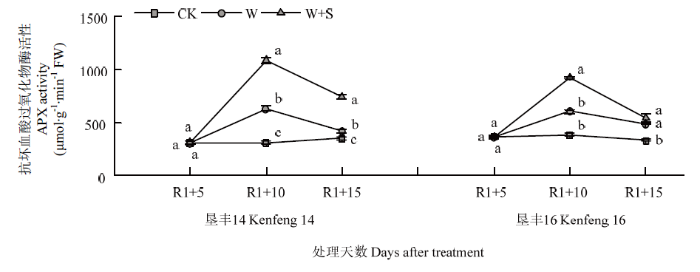
图5烯效唑对R1期淹水胁迫下大豆叶片APX活性的影响
Fig. 5Effects of S3307 on APX activity in leaf of soybean under waterlogging stress at R1 stage
2.5.2 烯效唑对R1期淹水胁迫下大豆叶片GR活性的影响 R1+5时,W+S处理可提高垦丰14和垦丰16叶片内GR活性,与2个品种CK相比分别增加5.39%和1.43%,但均未达显著差异水平。R1+10时,垦丰14和垦丰16各处理之间趋势表现为W+S>W>CK,淹水胁迫提高了2个品种叶片内GR活性,叶面喷施S3307后可进一步促进2个品种叶片内GR活性的提高,缓解大豆在淹水胁迫下所受到的伤害。R1+15时,垦丰14和垦丰16各处理之间高低趋势与R1+10时相同,说明淹水胁迫下喷施S3307具有延缓大豆叶片内GR活性降低的作用(图6)。
图6
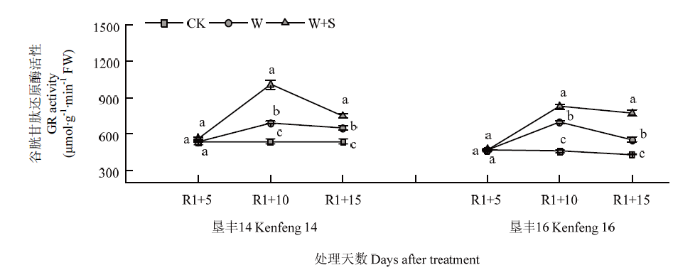
图6烯效唑对R1期淹水胁迫下大豆叶片GR活性的影响
Fig. 6Effects of S3307 on GR activity in leaf of soybean under waterlogging stress at R1 stage
2.5.3 烯效唑对R1期淹水胁迫下大豆叶片MDHAR活性的影响 R1+5时,W+S处理略提高垦丰14和垦丰16叶片内MDHAR活性,但与2个品种CK相比未达显著差异水平。R1+10时,垦丰14和垦丰16各处理之间MDHAR活性高低趋势均表现为W+S>W>CK,说明淹水胁迫提高了大豆叶片内MDHAR活性,喷施S3307后进一步提高了MDHAR活性。恢复正常水分后(R1+15),2个品种W处理MDHAR活性均呈降低趋势,但仍显著高于CK,W+S处理的2个品种MDHAR活性虽有降低,但仍维持较高水平,说明S3307可延缓MDHAR活性的降低,减缓淹水胁迫对大豆所造成的伤害(图7)。
图7
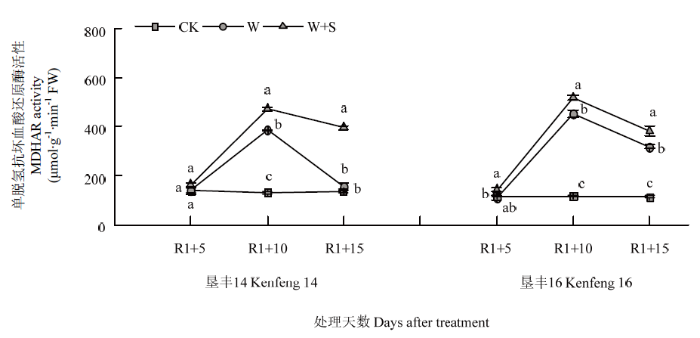
图7烯效唑对R1期淹水胁迫下大豆叶片MDHAR活性的影响
Fig. 7Effects of S3307 on MDHAR activity in leaf of soybean under waterlogging stress at R1 stage
2.5.4 烯效唑对R1期淹水胁迫下大豆叶片DHAR活性的影响 R1+5时,S3307喷施可提高垦丰14和垦丰16叶片内DHAR活性,但与CK相比无显著性差异。R1+10时,垦丰14和垦丰16叶片内DHAR活性高低趋势均表现W+S>W>CK,说明淹水胁迫提高了大豆叶片体内DHAR活性,喷施S3307后可进一步提高DHAR活性。恢复正常水分后(R1+15),垦丰14和垦丰16 W处理和W+S处理叶片内DHAR活性虽有降低,但高低趋势与R1+10时相同,W+S处理虽有降低,但显著高于W处理和CK,说明S3307具有延缓DHAR活性降低的作用,有利于增强清除活性氧的能力(图8)。
图8
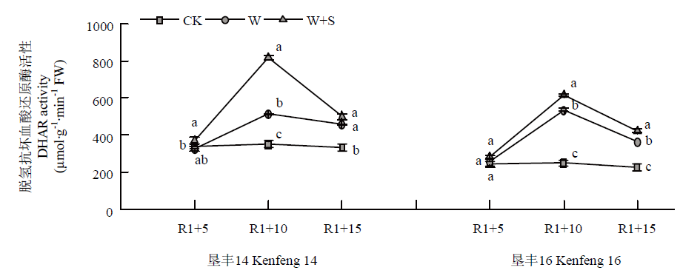
图8烯效唑对R1期淹水胁迫下大豆叶片DHAR活性的影响
Fig. 8Effects of S3307 on DHAR activity in leaf of soybean under waterlogging stress at R1 stage
2.6 R1期淹水胁迫对大豆叶片AsA-GSH的损伤及S3307的缓解效应
淹水胁迫导致$\mathop{{O}}_{2}^{{\mathop{}_{\ ·}^{-}}}$产生速率的增加,随后$\mathop{{O}}_{2}^{{\mathop{}_{\ ·}^{-}}}$被歧化为H2O2,$\mathop{{O}}_{2}^{{\mathop{}_{\ ·}^{-}}}$和H2O2含量的增加会改变膜的流动性,形成MDA等膜脂过氧化物。AsA-GSH可有效清除淹水胁迫积累的ROS。AsA可被APX催化与H2O2反应生成MDAHAR,MDHAR可协同APX清除H2O2,使H2O2分解为H2O和O2。DHAR是存在于AsA和DHA之间的关键酶,在GSH和NADH存在的条件下催化DHA转化为AsA。GSH具有清除$\mathop{{O}}_{2}^{{\mathop{}_{\ ·}^{-}}}$的作用,可被DHAR氧化生成GSSG。淹水胁迫增加了AsA、GSH、DHA、GSSG含量,并提高了关键酶(APX、GR、DHAR、MDHAR)的活性,S3307处理后可进一步增加上述指标的活性,并有效抑制了ROS和积累和MDA含量的增加,进而起到提高大豆耐涝性的作用(图9)。图9
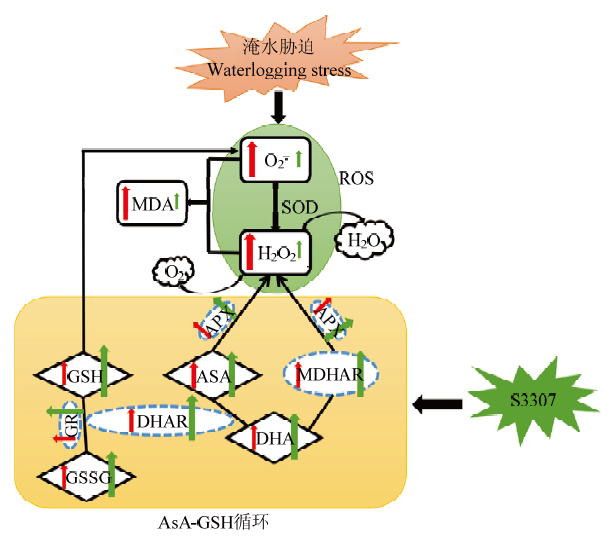
图9R1期淹水胁迫对大豆叶片AsA-GSH循环的损伤及S3307的缓解效应
红色箭头表示R1期淹水胁迫对各指标的影响(增加或减少);绿色箭头表示R1期淹水胁迫下S3307处理对各指标的影响(增加或减少)
Fig. 9Damage of AsA-GSH cycle of soybean leaves under waterlogging stress at R1 stage and the mitigation effect of S3307
The red arrow indicates the impact of R1 waterlogging stress on each indicator (increase or decrease); The green arrow indicates the impact of S3307 treatment on each index under R1 waterlogging stress (increase or decrease)
3 讨论
淹水胁迫会对植物生长发育造成严重影响,且表现为淹水时间越长伤害作用越高的趋势。初花期(R1)是大豆营养生长和生殖生长并进的时期,在R1期,大豆受到淹水胁迫,会严重抑制植株的生长发育,导致产量降低[41]。烯效唑是重要的外源控长调节剂,通过浸种[42]、叶喷等方式[21],均可提高作物的抗逆性。研究表明,烯效唑可通过提高抗氧化酶含量,清除过多活性氧,保持较高的抗氧化剂含量,增强作物抗氧化能力,减轻膜脂过氧化程度,缓解逆境胁迫对植物造成的伤害[43,44,45]。3.1 R1期淹水胁迫及S3307处理对大豆膜脂过氧化程度的影响
MDA是植物细胞膜脂过氧化产物,其含量是衡量植物细胞膜脂过氧化程度和质膜损伤的重要指标[46]。叶片内MDA含量的增加,会导致表面细胞膜受害程度加大,MDA可与膜上酶和蛋白质等结合,使细胞失活,从而破坏生物膜的结构和功能,影响细胞的物质代谢[47]。本试验结果表明,淹水胁迫下垦丰14和垦丰16 2个品种大豆叶片MDA含量增加,加剧膜脂过氧化和细胞膜破坏程度,且涝渍敏感品种垦丰16受到破坏程度大于耐涝品种垦丰14,说明耐涝品种承受淹水胁迫能力更强,这与张洪鹏等[48]研究结果一致。于奇等[49]研究表明,在淹水胁迫下应用S3307处理可降低R1期绿豆叶片MDA含量,缓解淹水胁迫对绿豆叶片细胞膜的伤害。项洪涛等[28]研究指出,S3307能够影响植物体内自由基和△-二氢吡咯-5-羧酸合成酶的合成,自由基作用于膜脂过氧化合成,最终产物是MDA,S3307处理可抑制自由基的合成,并降低MDA含量,提高作物的耐寒性。本试验研究结果表明,淹水胁迫条件下,S3307处理后,降低了2个大豆品种叶片内MDA含量,减轻了淹水胁迫下膜脂过氧化程度对大豆叶片造成的伤害,保证大豆在淹水胁迫下正常生长发育。3.2 R1期淹水胁迫及S3307处理对大豆活性氧代谢的影响
一般来说,植物在正常条件下生长,可通过自身产生的酶促和非酶促氧化防御体系来抵抗过量的ROS积累,促进植物正常生长,而淹水胁迫会抑制作物的正常生长,使植物体内相对平稳的活性氧遭到淹水胁迫的破坏,进而导致超氧阴离子($\mathop{{O}}_{2}^{{\mathop{}_{\ ·}^{-}}}$)和过氧化氢(H2O2)的增生。过多的活性氧自由基不能被及时清除,会加快膜脂过氧化进程,降低膜系统的完整性,损伤蛋白质,最终导致植物体内生理生化代谢紊乱,抑制植物生长发育[50]。本研究得出,R1期淹水胁迫显著增加2个大豆品种叶片中$\mathop{{O}}_{2}^{{\mathop{}_{\ ·}^{-}}}$产生速率和H2O2含量,与各自CK相比,R1期淹水胁迫5 d时(R1+10),垦丰14增加了1.60倍和1.30倍,垦丰16增加了1.28倍和1.16倍。研究指出,外施S3307可提高作物在逆境胁迫下的耐受性,原因可能是S3307可帮助降低由ROS过度积累引起的膜损伤[5,28-29]。本试验研究表明,S3307可有效减少淹水胁迫下2个大豆品种叶片内$\mathop{{O}}_{2}^{{\mathop{}_{\ ·}^{-}}}$产生速率和H2O2含量的积累,说明S3307对维持ROS平衡具有积极作用。3.3 R1期淹水胁迫及S3307处理对大豆AsA-GSH循环的影响
AsA-GSH循环参与植物胁迫反应,其中,AsA和GSH是循环中重要的抗氧化物质,是清除自由氧系统的重要组成物质,可有效清除H2O2和ROS[50]。APX、GR、MDHAR、DHAR是AsA-GSH循环中的关键酶,和其他抗氧化物质共同作用清除ROS,使ROS保持平衡,维持细胞膜的稳定性[51]。吴秀等[52]研究表明,APX和DHAR是AsA-GSH循环中活性最强的2个关键酶,其活性会直接影响AsA和DHA含量。APX在清除H2O2时,先将H2O2还原成H2O,从而清除H2O2,并伴随MDHAR的产生,MDHAR会氧化成DHA,当MDHAR和DHAR共同存在时,DHAR将利用GSH还原DHA,并有MDHAR和DHA重新生成AsA,AsA可直接参与$\mathop{{O}}_{2}^{{\mathop{}_{\ ·}^{-}}}$清除[53],而GSH在反应过程中被氧化成GSSG,再通过GR氧化NADPH重新还原产生[54,55]。前人研究指出,GSH在植物胁迫抵抗中的主要作用是保持较高的含量,促进膜蛋白结构稳定,因此,GSH和GR活性水平被认为是机体抗氧化状态的重要标志[56],同时GR活性可直接影响GSH含量,GR活性上升是造成GSH含量增加的一个因素之一[57]。顾帆等[58]在对高温和干旱胁迫对黄薇抗氧化系统的影响中研究表明,APX是循环中主要的清除酶类,其余的酶则维持循环内的平衡,保证活性氧的清除能力。本试验结果表明,垦丰14和垦丰16 APX、GR、MDHAR、DHAR活性在淹水胁迫第5天(R1+10)时显著增加,表明在淹水胁迫下,抗氧化酶活性急剧增加,这可能是大豆为适应淹水胁迫所产生的应激反应,叶面喷施S3307后可进一步促进APX、GR、MDHAR、DHAR活性的提高,且耐涝品种的酶活性高于涝渍敏感品种,这说明S3307能快速激活AsA-GSH中关键酶活性,保持AsA和GSH含量在较高水平,及时清除胁迫产生的H2O2和$\mathop{{O}}_{2}^{{\mathop{}_{\ ·}^{-}}}$,促进AsA-GSH循环的运转。恢复正常水分后(R1+15),淹水胁迫解除,抗氧化酶活性降低,耐涝品种较涝渍敏感品种恢复能力快,复水后可逐渐恢复至正常水平。另外,AsA、DHA、GSH和GSSG含量变化是可反映淹水胁迫下植物变化特征的重要指标。郭欣欣等[50]指出,淹水胁迫下,植物体内较高含量的抗氧化剂含量(AsA和GSH)和氧化还原力(DHA和GSSG)可保护细胞膜免受伤害,确保植物具有较强的抗逆能力,与本试验结果一致。淹水胁迫5 d(R1+10)时促进了垦丰14和垦丰16 W抗氧化剂含量(AsA和GSH)和氧化还原力(DHA和GSSG)的增加,且S3307处理可进一步促进上述指标含量的增加,说明S3307可清除淹水胁迫下大豆植株体内堆积的大量膜脂过氧化物,加强植株抵御逆境胁迫的性能和自身修复机制,恢复正常水分后(R1+15),S3307处理可保持较高的抗氧化剂含量,有助于快速恢复至正常生理状态。上述指标耐涝品种的含量均高于涝渍敏感品种,表明耐涝性强品种受到的伤害要小于涝渍敏感品种,S3307可有效防止大豆在淹水胁迫下细胞膜受损,提高耐涝性。
4 结论
淹水胁迫导致大豆叶片内ROS代谢紊乱,膜脂过氧化程度提高,喷施S3307可使淹水胁迫下ASA和GSH维持较高含量,提高关键酶活性,保证ASA-GSH循环的正常运行,并维持恢复正常水分管理后关键酶和抗氧化剂含量,提高大豆抗氧化能力,减轻膜脂过氧化程度,进而增加大豆的耐涝性。耐涝品种垦丰14受淹水胁迫造成的伤害程度低于涝渍敏感品种垦丰16。参考文献 原文顺序
文献年度倒序
文中引用次数倒序
被引期刊影响因子
[本文引用: 1]
[本文引用: 1]
[本文引用: 1]
[本文引用: 1]
[本文引用: 1]
DOI:10.13287/j.1001-9332.202004.016URLPMID:32530197 [本文引用: 1]

Under the background of climate change, the spatial-temporal distribution of precipita-tion in Heilongjiang Province is uneven, and drought and flood frequently change, which is not conducive to the safety of soybean production for the province. To clarify the influence mechanism of drought and flood in the growing season on soybean yield in Heilongjiang Province, we analyzed the time-series characteristics of drought and flood in soybean growing season and its effect on soybean yield in different growth stages, based on data of daily precipitation from 60 meteorological stations during 1961 to 2018 and soybean yield in the same period, with the standardized precipitation index (SPI) as the drought and flood evaluation index. The results showed that, from 1961 to 2018, the influence range of drought in soybean growing season in Heilongjiang Province showed a weak decreasing trend, while that of flood showed a weak increasing trend. In the same period, the intensity of both drought and flood showed a weak increasing trend, with slightly stronger role of flood intensity. The probability of the co-occurrence of drought and flood accounted for 60.3%. The soybean growing season in Heilongjiang Province may become wetter. From 2012 to 2018, the influence range and occurrence intensity of flood were significantly higher than that of drought, six years of the whole or regional flood occurred, in which five years were moderate degrees. The effects of drought and flood on soybean yield differed across regions in soybean growing season. The effect of flood on soybean yield was significantly stronger than that of drought in the Northwest, North and East, and were similar in the Midland, while in the Southwest, South and Southeast, the effect of drought was much greater than that of flood. The fluctuation of soybean yield was closely related to drought and flood during bloom-seed-filling period. Among them, in the Northwest, Southwest, Midland, South and Southeast of Heilongjiang, soybean yield would reach a high level when there was a little bit more precipitation, but the moderate and above-moderate levels of flood would cause the reduction. In the North, the fluctuation of soybean yield was mainly affected by flood, while in the East, the effects of drought and flood on soybean yield were similar.
DOI:10.13287/j.1001-9332.202004.016URLPMID:32530197 [本文引用: 1]

Under the background of climate change, the spatial-temporal distribution of precipita-tion in Heilongjiang Province is uneven, and drought and flood frequently change, which is not conducive to the safety of soybean production for the province. To clarify the influence mechanism of drought and flood in the growing season on soybean yield in Heilongjiang Province, we analyzed the time-series characteristics of drought and flood in soybean growing season and its effect on soybean yield in different growth stages, based on data of daily precipitation from 60 meteorological stations during 1961 to 2018 and soybean yield in the same period, with the standardized precipitation index (SPI) as the drought and flood evaluation index. The results showed that, from 1961 to 2018, the influence range of drought in soybean growing season in Heilongjiang Province showed a weak decreasing trend, while that of flood showed a weak increasing trend. In the same period, the intensity of both drought and flood showed a weak increasing trend, with slightly stronger role of flood intensity. The probability of the co-occurrence of drought and flood accounted for 60.3%. The soybean growing season in Heilongjiang Province may become wetter. From 2012 to 2018, the influence range and occurrence intensity of flood were significantly higher than that of drought, six years of the whole or regional flood occurred, in which five years were moderate degrees. The effects of drought and flood on soybean yield differed across regions in soybean growing season. The effect of flood on soybean yield was significantly stronger than that of drought in the Northwest, North and East, and were similar in the Midland, while in the Southwest, South and Southeast, the effect of drought was much greater than that of flood. The fluctuation of soybean yield was closely related to drought and flood during bloom-seed-filling period. Among them, in the Northwest, Southwest, Midland, South and Southeast of Heilongjiang, soybean yield would reach a high level when there was a little bit more precipitation, but the moderate and above-moderate levels of flood would cause the reduction. In the North, the fluctuation of soybean yield was mainly affected by flood, while in the East, the effects of drought and flood on soybean yield were similar.
[D].
[本文引用: 4]
[D].
[本文引用: 4]
[本文引用: 1]
URL [本文引用: 1]
URL [本文引用: 1]
[本文引用: 1]
DOI:10.1083/jcb.200409170URLPMID:15631987 [本文引用: 1]

Hydrogen peroxide (H2O2) has established itself as a key player in stress and programmed cell death responses, but little is known about the signaling pathways leading from H2O2 to programmed cell death in plants. Recently, identification of key regulatory mutants and near-full genome coverage microarray analysis of H2O2-induced cell death have begun to unravel the complexity of the H2O2 network. This review also describes a novel link between H2O2 and sphingolipids, two signals that can interplay and regulate plant cell death.
DOI:10.1016/j.plaphy.2010.08.016URLPMID:20870416 [本文引用: 1]

Various abiotic stresses lead to the overproduction of reactive oxygen species (ROS) in plants which are highly reactive and toxic and cause damage to proteins, lipids, carbohydrates and DNA which ultimately results in oxidative stress. The ROS comprises both free radical (O(2)(-), superoxide radicals; OH, hydroxyl radical; HO(2), perhydroxy radical and RO, alkoxy radicals) and non-radical (molecular) forms (H(2)O(2), hydrogen peroxide and (1)O(2), singlet oxygen). In chloroplasts, photosystem I and II (PSI and PSII) are the major sites for the production of (1)O(2) and O(2)(-). In mitochondria, complex I, ubiquinone and complex III of electron transport chain (ETC) are the major sites for the generation of O(2)(-). The antioxidant defense machinery protects plants against oxidative stress damages. Plants possess very efficient enzymatic (superoxide dismutase, SOD; catalase, CAT; ascorbate peroxidase, APX; glutathione reductase, GR; monodehydroascorbate reductase, MDHAR; dehydroascorbate reductase, DHAR; glutathione peroxidase, GPX; guaicol peroxidase, GOPX and glutathione-S- transferase, GST) and non-enzymatic (ascorbic acid, ASH; glutathione, GSH; phenolic compounds, alkaloids, non-protein amino acids and alpha-tocopherols) antioxidant defense systems which work in concert to control the cascades of uncontrolled oxidation and protect plant cells from oxidative damage by scavenging of ROS. ROS also influence the expression of a number of genes and therefore control the many processes like growth, cell cycle, programmed cell death (PCD), abiotic stress responses, pathogen defense, systemic signaling and development. In this review, we describe the biochemistry of ROS and their production sites, and ROS scavenging antioxidant defense machinery.
[本文引用: 1]
[本文引用: 1]
DOI:10.3389/fpls.2017.01061URLPMID:28674552 [本文引用: 1]

Cadmium (Cd) brings a devastating health hazard to human being as a serious consequence of agricultural and environmental contamination. We demonstrated the protective effect of silicon (Si) on cadmium (Cd)-stressed rapeseed (Brassica napus L. cv. BINA Sharisha 3) plants through regulation of antioxidant defense and glyoxalase systems. Twelve-day-old seedlings were exposed to Cd stress (0.5 and 1.0 mM CdCl2) separately and in combination with Si (SiO2, 1.0 mM) for 2 days. Cadmium toxicity was evident by an obvious oxidative stress through sharp increases in H2O2 content and lipid peroxidation (malondialdehyde, MDA content), and visible sign of superoxide and H2O2. Cadmium stress also decreased the content of ascorbate (AsA) and glutathione (GSH) as well as their redox pool. The activities of monodehydroascorbate reductase (MDHAR), dehydroascorbate reductase (DHAR) and catalase (CAT) were decreased by Cd while ascorbate peroxidase (APX) and glutathione S-transferase (GST) activities were increased. The enzymes of glyoxalase system (glyoxalase I, Gly I and glyoxalase II, Gly II) were also inefficient under Cd stress. However, exogenous application of Si in Cd treated seedlings reduced H2O2 and MDA contents and improved antioxidant defense mechanism through increasing the AsA and GSH pools and activities of AsA-GSH cycle (APX, MDHAR, DHAR and GR) and glyoxalase system (Gly I and Gly II) enzymes and CAT. Thus Si reduced oxidative damage in plants to make more tolerant under Cd stress through augmentation of different antioxidant components and methylglyoxal detoxification system.
URL [本文引用: 1]
DOI:10.1007/s00709-018-1213-5URLPMID:29372337 [本文引用: 1]

This paper investigated the roles of hydrogen sulfide (H2S) and hydrogen peroxide (H2O2) and the possible relationship between them in regulating the AsA-GSH cycle in wheat leaves under drought stress (DS). Results showed that DS markedly increased the production of H2S and H2O2, the transcript levels and activities of ascorbate peroxidase (APX), glutathione reductase (GR), monodehydroascorbate reductase (MDHAR), and dehydroascorbate reductase (DHAR); malondialdehyde (MDA) content; and electrolyte leakage (EL). Meanwhile, DS markedly reduced plant height and biomass. Above increases induced by drought stress except MDA content and EL were all suppressed by pretreatments with H2S synthesis inhibitor aminooxyaceticacid (AOA) and H2O2 synthesis inhibitor diphenylene iodonium (DPI). Besides, pretreatments with AOA and DPI further significantly increased MDA content and EL and significantly reduced plant height and biomass under DS. DPI reduced the production of H2O2 and H2S induced by DS. AOA also reduced the production of H2S and H2O2 induced by DS. Pretreatments with NaHS + AOA and H2O2 + DPI reversed above effects of AOA and DPI. Our results suggested that H2S and H2O2 all participated in the up-regulation of AsA-GSH cycle in wheat leaves by DS and possibly affected each other.
[本文引用: 1]
[本文引用: 1]
[本文引用: 1]
[本文引用: 1]
[本文引用: 1]
[本文引用: 1]
[本文引用: 1]
[本文引用: 1]
[本文引用: 1]
[本文引用: 1]
[本文引用: 2]
[本文引用: 2]
URL [本文引用: 1]
URL [本文引用: 1]
[本文引用: 1]
[本文引用: 1]
URLPMID:19176720 [本文引用: 1]
[本文引用: 1]
[本文引用: 1]
URLPMID:16769153 [本文引用: 1]
[本文引用: 3]
[本文引用: 3]
[本文引用: 2]
URL [本文引用: 1]
[本文引用: 1]
[本文引用: 1]
[本文引用: 1]
[本文引用: 1]
[本文引用: 1]
[本文引用: 1]
[本文引用: 1]
[本文引用: 3]
[本文引用: 3]
[本文引用: 1]
[本文引用: 1]
[本文引用: 1]
[本文引用: 1]
URL [本文引用: 1]
[本文引用: 1]
URLPMID:24431505 [本文引用: 1]
[本文引用: 1]
[本文引用: 1]
[本文引用: 1]
[本文引用: 1]
DOI:10.19540/j.cnki.cjcmm.20190325.101URLPMID:31359644 [本文引用: 1]

The aim of the study is to explore exogenous S3307 on alleviating low-temperature stress of coix seedlings. The coix cultivar,
DOI:10.19540/j.cnki.cjcmm.20190325.101URLPMID:31359644 [本文引用: 1]

The aim of the study is to explore exogenous S3307 on alleviating low-temperature stress of coix seedlings. The coix cultivar,
[本文引用: 1]
[本文引用: 1]
[本文引用: 1]
[本文引用: 1]
[本文引用: 1]
[本文引用: 1]
[D].
[本文引用: 1]
[D].
[本文引用: 1]
[本文引用: 1]
[本文引用: 1]
URL [本文引用: 1]
URL [本文引用: 1]
[本文引用: 3]
[本文引用: 3]
URL [本文引用: 1]
URL [本文引用: 1]
URL [本文引用: 1]
URL [本文引用: 1]
[本文引用: 1]
[本文引用: 1]
[本文引用: 1]
DOI:10.1016/j.plaphy.2005.01.002URLPMID:15820657 [本文引用: 1]

To understand the interaction between Zn, an essential micronutrient and Cd, a non-essential element, Cd-10 microM and Zn supplemented (10, 50, 100, and 200 microM) Cd 10 microM treated Ceratophyllum demersum L. (Coontail), a free floating freshwater macrophyte was chosen for the study. Cadmium at 10 microM concentration decreased thiol content, enhanced oxidation of ascorbate (AsA) and glutathione (GSH) to dehydroascorbate (DHA) and glutathione disulfide (GSSG), respectively, a clear indication of oxidative stress. Zinc supplementation to Cd (10 microM) treated plants effectively restored thiols, inhibited oxidation of AsA and GSH maintaining the redox molecules in reduced form. Cd-10 microM slightly induced ascorbate peroxidase (APX, E.C. 1.11.1.11) but inhibited monodehydroascorbate reductase (MDHAR, E.C. 1.6.5.4), dehydroascorbate reductase (DHAR, E.C. 1.8.5.1) and glutathione reductase (GR, E.C. 1.6.4.2), enzymes of ascorbate-glutathione cycle (AGC). Zn supplementation restored and enhanced the functional activity of all the AGC enzymes (APX, MDHAR, DHAR and GR). Gamma-glutamylcysteine synthetase (gamma-GCS, E.C. 6.3.2.2) was not affected by Cd as well as Zn, but Zn supplements increased glutathione-S-transferase (GST, E.C. 2.5.1.18) activity to a greater extent than Cd and simultaneously restored glutathione peroxidase (GSH-PX, E.C. 1.11.1.9) activity impaired by Cd toxicity. Zn-alone treatments did not change above investigated parameters. These results clearly indicate the protective role of Zn in modulating the redox status of the plant system through the antioxidant pathway AGC and GSH metabolic enzymes for combating Cd induced oxidative stress.
URL [本文引用: 1]
[本文引用: 1]
DOI:10.1016/S0168-9452(02)00159-0URL [本文引用: 1]
[本文引用: 1]
URL [本文引用: 1]