

Functional analysis of ZmCIPK24-2 gene from maize in response to salt stress
LI Jian

通讯作者:
收稿日期:2020-02-6接受日期:2020-03-24网络出版日期:2020-09-12
基金资助: |
Received:2020-02-6Accepted:2020-03-24Online:2020-09-12
Fund supported: |
作者简介 About authors
E-mail:

摘要
关键词:
Abstract
Keywords:
PDF (3671KB)元数据多维度评价相关文章导出EndNote|Ris|Bibtex收藏本文
本文引用格式
李健, 王逸茹, 张凌霄, 孙明昊, 秦阳, 郑军. 玉米ZmCIPK24-2基因在盐胁迫应答中的功能研究[J]. 作物学报, 2020, 46(9): 1351-1358. doi:10.3724/SP.J.1006.2020.03008
LI Jian, WANG Yi-Ru, ZHANG Ling-Xiao, SUN Ming-Hao, QIN Yang, ZHENG Jun.
作物生长过程中需要应对各种不良环境, 包括病原体感染、动物啃食的生物胁迫与高盐、干旱和冷害等非生物胁迫[1]。其中, 盐胁迫是造成作物减产的主要原因之一, 危害植物生长的各个方面, 在全世界范围内有超过8亿公顷的土地受到盐胁迫影响[2], 每年由于土壤盐碱化浪费了大量的土地资源[3]。高浓度盐会导致高渗胁迫、离子毒性(主要是Na+)、以及氧化损伤等次级胁迫[4]。盐胁迫初期会使植物遭受渗透胁迫, 其原因主要是根系周围盐离子浓度过高而造成植物根系的水分吸收障碍, 导致细胞伸长率降低、细胞分裂速度减缓, 并引起气孔关闭, 从而影响植物的光合速率, 抑制植物的正常代谢及生长过程, 限制其生产力。到了盐胁迫后期, 由于Na+浓度过高, 使Na+在植物体内积累过多, 打破了植物体内Na+/K+的离子平衡, 造成离子毒害, 加速叶片衰老[2]。
钙是植物体内必需矿质元素之一, 细胞内游离的钙离子(Ca2+)是植物体内普遍的第二信使, 细胞内Ca2+浓度的变化是植物对许多胁迫的初始响应[5]。Ca2+浓度独特的时空变化, 也影响着诸如气孔运动、根毛伸长和花粉管生长的生理过程[6]。此外, 在内质网、液泡膜以及质膜上都存在Ca2+泵或者Ca2+通道。缺钙时, 也会影响正常的细胞分裂以及膜结构的稳定。植物细胞内存在着多种Ca2+感受器, Ca2+感受器在植物生长发育的过程中发挥着重要的作用。钙调蛋白(CaM)是钙结合蛋白, 几乎存在于所有的真核生物中, 并且参与调节转录及酶活性; Ca2+依赖性蛋白激酶(CDPK)是由一个N端可变域、丝氨酸/苏氨酸蛋白激酶域、假底物区段和结合Ca2+的EF手组成[7]。有研究证明在水稻中过表达OsCDPK7增强了对盐、干旱和寒冷胁迫的耐受性[8]; Ca2+信号也可以被CBL解码, CBL钙感受器是一种小型的EF手型蛋白[9]。拟南芥CBL1突变后损害植株对盐胁迫和干旱胁迫的应答[10]。SOS3 (CBL4)肉豆蔻酰化基序的破坏使得突变体表现出盐敏感的表型[11]。CIPK是由N端保守的激酶催化结构域和C端的调解结构域构成的, 其N端包含自抑制NAF/FISL基序, 介导与CBL的结合[12]。拟南芥中有10个CBL与26个CIPK, 这些蛋白相互作用, 形成了复杂的信号网络, 在调节离子通量、信号转导和非生物胁迫响应中发挥关键作用[13]。拟南芥对抗盐胁迫的过程中, 可以通过SOS途径的钙依赖蛋白激酶途径介导盐胁迫信号, 激活的SOS2 (CIPK24)发生磷酸化进而激活质膜Na+/H+转运体SOS1, 从而增强植物对Na+的耐受性[14]。最近的研究结果表明盐诱导激活了SOS途径, SCaBP8通过与ATANN4互作抑制ATANN4介导的钙瞬变, 表明通过反馈调节, SOS途径也可以影响盐诱导条件下钙信号的产生[15]。在玉米中ZmCIPK21在盐胁迫后对于维持植物体内Na+、K+平衡具有重要作用[16]。
尽管已经报道了一些玉米中CIPK家族基因的相关功能, 但知之甚少。本文报道一个玉米基因ZmCIPK24-2, 该基因在玉米各组织部位均表达, 并受到盐诱导表达。将ZmCIPK24-2转入拟南芥sos2突变体中, 转基因植株的耐盐性增强。亚细胞定位实验表明: ZmCIPK24-2定位在细胞膜、细胞质以及核膜。通过互作分析, 发现ZmCIPK24-2与玉米中的4个ZmCBLs互作, 包括ZmCBL1、ZmCBL4、ZmCBL8和ZmCBL9。
1 材料与方法
1.1 蛋白序列获取与分析
在TAIR (1.2 植物材料与生长条件
玉米B73自交系, 卷纸发芽3 d后, 水培育苗, 生长至第7天时, 用200 mmol L-1的NaCl处理0、2、4、6、8、10、12 h后, 取玉米地上部, 液氮速冻, -80℃冰箱保存, 设置3个生物学重复。B73自交系不同时期不同组织部位分别取样后液氮速冻, -80℃冰箱保存。野生型拟南芥(Columbia-0)、拟南芥atsos2突变体和本氏烟草(N. benthamian)为本实验室保存。将NaClO消毒的拟南芥种子定量点种于MS培养基上, 4℃春化3 d后置22℃人工气候室内, 每日光照16 h, 黑暗8 h。1.3 基因组DNA、RNA的提取及cDNA的制备
使用TIANGEN公司的植物DNA提取试剂盒、RNAprep pure植物总RNA提取试剂盒, 分别提取拟南芥叶片和玉米植物DNA及总RNA。用全式金公司的TransScript II One-Step gDN A Removal and cDNA Synthesis SuperMix试剂盒进行cDNA的制备。1.4 基因表达分析
利用实时荧光定量聚合酶链式反应(RT-qPCR)分析玉米中ZmCIPK24-2基因的表达, 所用上游引物为ZmCIPK24-2-Q-F3, 下游引物为ZmCIPK24- 2-Q-R3 (表1), 以玉米GAPDH基因为内参。实验所用试剂盒TransStart Green qPCR SuperMix购自北京全式金公司。加入模板cDNA 1 μL, 总反应体积为20 μL, 放入Applied Biosystem 7300仪进行反应, 反应条件为95℃预变性2 min, 95℃ 10 s, 58℃ 20 s, 72℃ 30 s, 循环数为40个, 72℃延伸30 s收集荧光, 并添加熔解曲线。2次生物学重复, 每次生物学重复进行3次技术重复, 结果用2-ΔΔCt法计算。半定量聚合酶链式反应(RT-PCR)实验所用引物为ZmCIPK24-2- RT-F/R (表1), 以拟南芥ACTIN基因作为内参。2×Es Taq MasterMix购自江苏康为世纪生物科技有限公司, 反应条件为94℃预变性5 min, 94℃ 30 s, 58℃ 30 s, 72℃延伸, 扩增拟南芥ACTIN基因延伸时间为30 s,循环数为28个。扩增ZmCIPK24-2基因延伸时间为90 s,循环数为30个。Table 1
表1
表1本研究所用的引物
Table 1
引物 Primer | 正向序列 Forward sequence (5'-3') | 反向序列 Reverse sequence (5'-3') |
---|---|---|
ZmCIPK24-2-F/R | ATGGCGGGCGCGGGCGCGGG | CTAGCAGGTGGTCGTCCTCA |
ZmCIPK24-2-Q-F3/R3 | AAGGTCCAGCGTCA | GGCGTAGATTTGGCA |
GAPDH-F/R | AGGATATCAAGAAAGCTATTAAGGC | GTAGCCCCACTCGTTGTCG |
ZmCIPK24-2-RT-F/R | TGCCACAACAAAGGAGTTTATCATA | TGAGAGATCCAAACCTTGAGATAGT |
AtACTIN-F/R | GCCAATCCGGTGCTGGTAACA | CATACCAGATCCAGTTCCTCCTCCC |
ZmCIPK24-2-IF-F/R | AGCAGGCTTTGACTTTATGGCGGGCGCGGGCGCGGG | TGGGTCTAGAGACTTTCTGCAGGTGGTCGTCCTCA |
CBL1-nluc-F/R | CACGGGGGACGAGCTCGGTACCATGGGGTGCTTCCATTCCAC | ACGCGTACGAGATCTGGTCGACCGTGACGAGATCGTCGA |
CBL4-nluc-F/R | CACGGGGGACGAGCTCGGTACCATGGGCTGCGCGACGTCCAA | ACGCGTACGAGATCTGGTCGACGTCACTGGCTTCTGAAC |
CBL8-nluc-F/R | CACGGGGGACGAGCTCGGTACCATGGGGTGTGTGTCCTCCAA | ACGCGTACGAGATCTGGTCGACCAACTCGTCGTCACTGG |
CBL9-nluc-F/R | CACGGGGGACGAGCTCGGTACCATGGGGTGCTTCCATTCCAC | ACGCGTACGAGATCTGGTCGACCGTGACGAGATCGTCGA |
CIPK24-2-cluc-F/R | TACGCGTCCCGGGGCGGTACCATGGCGGGCGCGGGCGCGGG | TCCTTGTAGTCCATTTGTTGGCAGGTGGTCGTCCTCA |
新窗口打开|下载CSV
1.5 ZmCIPK24-2互补材料的获得
将具有草铵膦抗性基因的pEarlyGate100载体与ZmCIPK24-2完整CDS区构建重组质粒, 所用引物为ZmCIPK24-2-F/R和ZmCIPK24-2-IF-F/R(表1)。转化到根癌农杆菌菌株GV3101中, 用蘸花法侵染拟南芥atsos2突变体, 获得T0代植株, 收获种子后用草铵膦筛选, 再繁种筛选直至获得纯合阳性株系用于实验。1.6 亚细胞定位
构建重组质粒pEarlyGate101-ZmCIPK24-2, 所用引物为ZmCIPK24-2-F/R和ZmCIPK24-2-IF-F/R (表1)。将构建好的载体转化到根癌农杆菌菌株GV3101中, 在具有抗性的YEB培养基中, 28℃过夜孵育, 次日接种于新的具有抗性的YEB培养基中, 并加入0.2 mmol L-1的乙酰丁香酮和10 mmol L-1的2-吗啉乙磺酸(pH 5.6)。然后将培养物用0.2 mmol L-1乙酰丁香酮、10 mmol L-1 (N-吗啉)-乙磺酸(pH 5.6)和10 mmol L-1 MgCl2的重悬液重悬, 终浓度OD600=1.0。室温避光2 h后, 注射烟草。烟草于黑暗情况下生长48 h后, 用激光共聚焦显微镜观察(Zeiss LSM700)。1.7 酵母双杂交实验
本实验所用载体为实验室保存的pDEST22和pDEST32, 所用酵母菌株为MAV203。ZmCBL1、ZmCBL2-1、ZmCBL2-2、ZmCBL3、ZmCBL4、ZmCBL5、ZmCBL6-1、ZmCBL8、ZmCBL9和ZmCBL10 [17], 分别与pDEST22构建融合载体。ZmCIPK24-2与pDEST32构建融合载体。用YPDA在30℃温度条件下过夜培养酵母菌株, 按照 Frozen-EZ Yeast Transformation II-试剂盒(ZYMO RESEARCH)进行酵母感受态的制备及载体共转化, 置于30℃培养箱生长3 d, 挑取单菌落溶解于灭菌超纯水中, 进行梯度稀释, 分别在二缺和四缺培养基上观察培养。所用培养基为Clontech公司的YPDA、Minimal SD Agar Base、-Leu/-Trp DO Supplement和-Ade/-His/-Leu/- Trp DO Supplement。1.8 LUC互补成像实验
LUC互补成像实验所用的nLUC、cLUC载体为中国农业科学院作物科学研究所孙加强研究员惠赠。LUC互补成像实验验证蛋白互作的方法如前人所述[18]。将ZmCBL1、ZmCBL4、ZmCBL8和ZmCBL9四个基因完整的CDS区分别与nLUC构建融合载体, 将ZmCIPK24-2基因完整的CDS区插入到cLUC载体的多克隆位点区域内, 所用引物见表1。融合载体转化入根癌农杆菌GV3101菌株内, 培养方法同亚细胞定位实验, 侵染本生烟草叶片, 烟草于黑暗情况下生长48 h后, 用NightSHADE LB 985 (Berthold)观察荧光素酶活性。2 结果与分析
2.1 ZmCIPK24-2的序列特征分析
根据拟南芥SOS2 (AT5G35410)的基因及蛋白序列, 经数据库序列比对分析, 在玉米中鉴定到SOS2的同源基因Zm00001d000407, 该基因位于9号染色体上, CDS序列1356 bp, 共14个外显子, 编码451个氨基酸。但经文献分析发现已有报道SOS2的同源基因ZmCIPK24, 位于玉米6号染色体上[19]。因此将本研究鉴定的基因Zm00001d000407命名为ZmCIPK24-2。对SOS2、ZmCIPK24和ZmCIPK24-2氨基酸序列比对发现, ZmCIPK24-2与ZmCIPK24高度同源, 二者与拟南芥SOS2的序列相似性分别为68%和67%, 且具有CIPK蛋白激酶重要的连接域和保守的FISL基序(图1)。图1
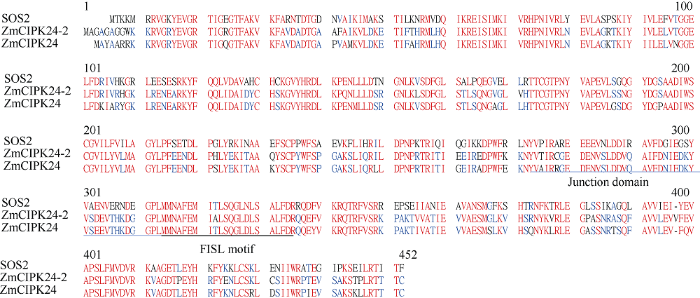
图1ZmCIPK24-2蛋白的序列分析
Fig. 1Sequence analysis of ZmCIPK24-2 protein
2.2 ZmCIPK24-2基因的表达分析
用200 mmol L-1的NaCl处理玉米幼苗, 发现在处理2 h时, ZmCIPK24-2基因的表达被显著诱导。处理4 h后, ZmCIPK24-2基因的表达恢复到初始水平(图2-A)。进一步对ZmCIPK24-2基因在玉米不同生长期、不同组织部位的表达量分析发现, 在玉米多个时期、组织部位都可以检测到ZmCIPK24-2基因的转录表达, 且在花粉(R2)中表达量最高, 其次是叶片(V1)和茎秆(V7、R2)(图2-B)。图2
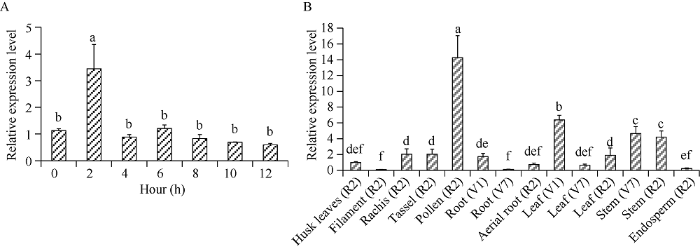
图2ZmCIPK24-2基因的表达模式分析
A: NaCl处理后ZmCIPK24-2基因的相对表达量; B: ZmCIPK24-2基因在玉米不同组织器官中的相对表达量。Husk leaves: 苞叶; Filament: 花丝; Rachis: 穗轴; Tassel: 雄穗; Pollen: 花粉; Root: 根; Aerial root: 气生根; Leaf: 叶; Stem: 茎; Endosperm: 胚乳。V1: 第1片叶完全展开; V7: 第7片叶完全展开; R2: 籽粒建成。标以不同小写字母的柱值在P<0.05水平差异显著。
Fig. 2Analysis of the expression pattern of ZmCIPK24-2
A: relative expression of ZmCIPK24-2 after NaCl treatment for indicated time; B: relative expression levels of ZmCIPK24-2 in different tissues of maize. V1: the first leaf is fully expanded; V7: the seventh leaf is fully expanded; R2: the kernel is completed. Bars with different lowercase are significantly different at the 0.05 probability level.
2.3 ZmCIPK24-2互补拟南芥sos2突变体能提高sos2的耐盐性
已报道拟南芥atsos2突变体表现出对盐胁迫敏感的特征[20], 推测ZmCIPK24-2与SOS2基因可能具有相似的功能特性。通过转基因, 获得了多个ZmCIPK24-2转化拟南芥突变体atsos2的株系。用PCR方法鉴定COM1和COM2两个转化株系中目标基因ZmCIPK24-2, 如图3-A显示, 从2个株系都可以扩增出清晰的条带, 表明ZmCIPK24-2基因已转入atsos2突变体。进一步提取2个转基因株系总RNA反转录获得cDNA后, 以拟南芥ACTIN作为参考基因, 进行RT-PCR检测, 如图3-B所示, 在转基因互补株系中检测到了ZmCIPK24-2基因的转录。图3
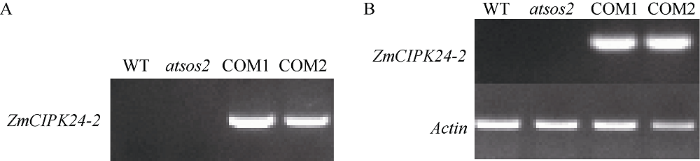
图3COM1和COM2转基因互补株系的分子检测
A: ZmCIPK24-2转基因株的检测; B: ZmCIPK24-2的转录检测。WT: 野生型; sos2: 突变体。
Fig. 3Molecular analysis of two complementary transgenic lines COM1 and COM2
A: detection of ZmCIPK24-2 transgenic lines; B: transcriptional level of ZmCIPK24-2.
对获得的转基因互补株系进行表型鉴定, 观察ZmCIPK24-2基因在拟南芥中是否可以恢复atsos2突变体的盐敏感特性。从图4-A和4-B中可以看到, WT、atsos2、COM1和COM2在无NaCl的培养基中生长正常, 存活率不存在显著差异; 当培养基中NaCl浓度达到75 mmol L-1时, WT存活率为99.1%, atsos2的存活率显著下降, 大约为62.8%, COM1和COM2的存活率分别为86.8%和90.6%左右, 显著高于突变体sos2; 当培养基中NaCl浓度达到100 mmol L-1时, WT的存活率为96.2%左右, atsos2最低, 为24.4%, COM1和COM2的存活率分别是70.1%和65.8%, 与sos2存活率相比存在显著差异。在无NaCl的MS培养基上, WT、atsos2、COM1与COM2的根长伸长无显著差异。在50 mmol L-1 NaCl培养基中, 与WT相比, atsos2对胁迫条件表现得更为敏感, 根长较短, 为9.5 mm左右。COM1和COM2根长大约为18.5 mm和20.9 mm, 显著高于突变体atsos2 (图4-C, D)。以上结果表明, ZmCIPK24-2可以部分恢复拟南芥atsos2突变体的盐敏感表型。
图4
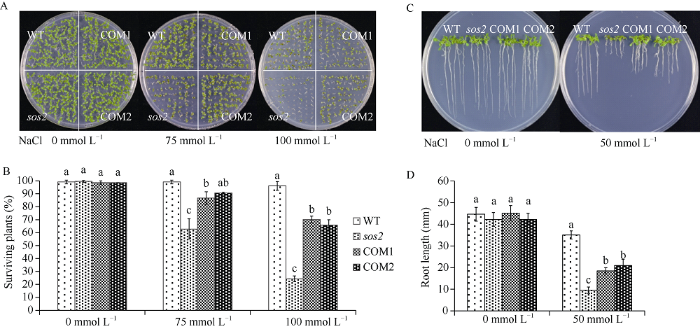
图4ZmCIPK24-2转化拟南芥atsos2突变体能提高atsos2的耐盐性
A, B: 盐胁迫对WT、atsos2、COM1和COM2植株存活率的影响; C, D: 盐胁迫对WT、atsos2、COM1和COM2根长的影响。误差线表示±标准差, 存活率n > 70, 根长n > 5。标以不同小写字母的柱值在P < 0.05水平差异显著。
Fig. 4Salt tolerance of atsos2 can be improved by ZmCIPK24-2
A, B: survival rate of WT, atsos2, COM1 and COM2 plants under salt stress; C, D: root length of WT, atsos2, COM1, and COM2 under salt stress. Mean values and SE were shown from multiple independent experiment, survival rate: n > 70, root length: n > 5. Bars with different lowercase are significantly different at the 0.05 probability level.
2.4 ZmCIPK24-2的亚细胞定位
为了进一步研究ZmCIPK24-2的亚细胞定位情况, 我们构建了ZmCIPK24-2与YFP的融合载体, 转化农杆菌后, 侵染烟草叶片, 观察ZmCIPK24-2在烟草叶片中的荧光信号, 如图5显示, ZmCIPK24- 2蛋白定位在烟草细胞中的细胞质、细胞膜与核膜。图5
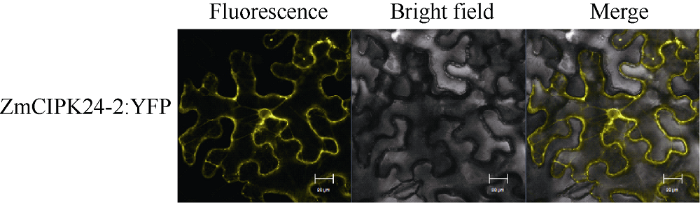
图5ZmCIPK24-2蛋白的亚细胞定位
黄色表示YFP蛋白在激光共聚焦显微镜下所发出的黄色荧光。
Fig. 5Subcellular localization of ZmCIPK24-2 protein
Yellow indicates the yellow fluorescence of YFP protein under confocal laser scanning microscope; Scale bars = 20 μm.
2.5 ZmCIPK24-2与ZmCBLs互作
前人研究已发现植物CIPK与Ca2+感受器CBL互作进行信号传递[21]。为了研究玉米ZmCIPK24-2与玉米CBL互作情况, 我们利用酵母双杂的方法鉴定ZmCIPK24-2与ZmCBL1、ZmCBL2-1、ZmCBL2- 2、ZmCBL3、ZmCBL4、ZmCBL5、ZmCBL6-1、ZmCBL8、ZmCBL9、ZmCBL10共10个玉米CBLs的互作, 结果发现ZmCBL1、ZmCBL4、ZmCBL8和ZmCBL9可以与ZmCIPK24-2互作(图6-A)。进一步利用LUC互补成像实验, 也证明在植物体内ZmCIPK24-2分别与ZmCBL1、ZmCBL4、ZmCBL8和ZmCBL9互作(图6-B)。图6
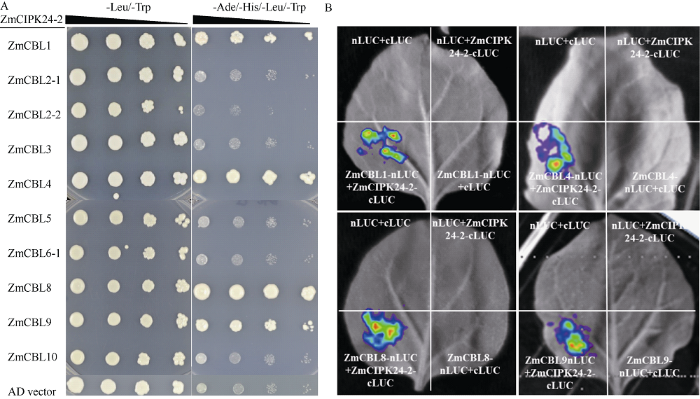
图6ZmCIPK24-2与ZmCBLs互作
A: 酵母双杂交实验检测ZmCIPK24-2与ZmCBLs的互作; B: LUC互补成像分析ZmCIPK24-2与ZmCBLs之间的相互作用。
Fig. 6Interaction between ZmCIPK24-2 and ZmCBLs
A: interaction between ZmCIPK24-2 and ZmCBLs by yeast two-hybrid experiment; B: interaction between ZmCIPK24-2 and ZmCBLs by Firefly LCI assay.
3 讨论
玉米是全世界最重要的C4作物, 盐胁迫是非生物胁迫中限制玉米产量的重要因素[22]。在植物响应盐胁迫过程中, CBL-CIPK复合体起到非常重要的作用[23]。CBL与CIPK的作用最早是通过拟南芥中的SOS途径揭示的[16], 而玉米中有关CIPK基因在耐盐信号通路中的功能还知之甚少。本研究鉴定到一个与拟南芥SOS2同源的基因ZmCIPK24-2, 通过序列分析ZmCIPK24-2与ZmCIPK24高度同源, 与AtSOS2的序列相似性为68%, 且ZmCIPK24-2、ZmCIPK24与AtSOS2都具有保守的自抑制结构域FISL基序, 因此ZmCIPK24-2也可能参与盐胁迫响应。用NaCl对B73材料进行处理, RT-qPCR检测ZmCIPK24-2基因的表达量, 我们发现ZmCIPK24-2基因能迅速响应盐胁迫, 此后逐渐恢复至初始水平, 但在盐诱导6 h、12 h和24 h后, RT-PCR结果显示ZmCIPK24基因转录表达上调[19], 与ZmCIPK24-2盐诱导后表达量变化趋势不同; 且在玉米B73材料不同组织部位中均可以检测到ZmCIPK24-2的转录表达, 表明ZmCIPK24-2参与了植物体内耐盐信号的响应, 与ZmCIPK24、AtSOS2的表达受到盐诱导的结果一致[19,24]。植物对盐分的适应性分为3种不同的类型, 即渗透胁迫耐受性, Na+或Cl-外排, 及组织对累积的Na+或Cl-的耐受性[2]。以往的研究表明在拟南芥中CBL4和CIPK24互作形成蛋白复合体, 通过SOS1将植物体内的Na+排出体外从而提高植物的耐盐性[25]。类SOS3钙结合蛋白SCABP8在体内与质膜相互作用并将SOS2招募到质膜中, 增强了拟南芥耐盐性[26]。将ZmCIPK24-2转入拟南芥atsos2突变体, 分别将WT、atsos2、COM1和COM2在不同NaCl浓度条件下培养, 对幼苗的存活率和根长进行分析, 与atsos2突变体相比, COM1和COM2在高NaCl浓度条件下存活率、根长显著高于atsos2突变体, 但还没有完全恢复到WT水平。结果表明ZmCIPK24-2基因可以在功能上部分互补SOS2基因的功能, 使拟南芥atsos2突变体耐盐性提高。拟南芥中ZmCIPK24-2可能与AtSOS2相同, 在盐胁迫条件下参与了相似的信号传递机制, 被SOS3招募到质膜中, 与SOS1互作增加Na+外排, 进而增强了atsos2突变体的耐盐性。酵母双杂实验和LUC互补成像实验表明ZmCIPK24-2与ZmCBL1、ZmCBL4、ZmCBL8和ZmCBL9互作。与前人的研究结果相同, 同一个CIPK可以与几个CBL成员互作[27]。过表达ZmCBL9提高了植物对ABA, 葡萄糖, 盐和渗透胁迫的耐受性[17], 推测ZmCIPK24-2与ZmCBL9互作, 参与ZmCBL9对非生物胁迫信号的响应。由此可见, CBL和CIPK基因功能具有特异性和冗余性, 形成了复杂的调控网络, 参与植物对非生物胁迫的应答[21]。前期研究证明拟南芥SOS2与SOS3互作依靠FISL基序[28], sos3突变体对高Na+造成的生长抑制高度敏感[29], 推测玉米中ZmCIPK24-2与4个ZmCBLs的互作需要通过保守的FISL基序, 共同调解植物对盐胁迫的响应, 但其分子机制还有待进一步研究。
4 结论
在玉米中鉴定到一个与拟南芥AtSOS2同源的基因ZmCIPK24-2, 其转录表达受到NaCl的诱导, 可部分互补拟南芥AtSOS2基因的功能, ZmCIPK24- 2与ZmCBL1、ZmCBL4、ZmCBL8和ZmCBL9均能互作。本研究结果为解析玉米CBL-CIPK响应盐胁迫的分子网络机制提供了新的实验证据。参考文献 原文顺序
文献年度倒序
文中引用次数倒序
被引期刊影响因子
DOI:10.1016/j.cell.2016.08.029URLPMID:27716505 [本文引用: 1]

As sessile organisms, plants must cope with abiotic stress such as soil salinity, drought, and extreme temperatures. Core stress-signaling pathways involve protein kinases related to the yeast SNF1 and mammalian AMPK, suggesting that stress signaling in plants evolved from energy sensing. Stress signaling regulates proteins critical for ion and water transport and for metabolic and gene-expression reprogramming to bring about ionic and water homeostasis and cellular stability under stress conditions. Understanding stress signaling and responses will increase our ability to improve stress resistance in crops to achieve agricultural sustainability and food security for a growing world population.
DOI:10.1146/annurev.arplant.59.032607.092911URLPMID:18444910 [本文引用: 3]

The physiological and molecular mechanisms of tolerance to osmotic and ionic components of salinity stress are reviewed at the cellular, organ, and whole-plant level. Plant growth responds to salinity in two phases: a rapid, osmotic phase that inhibits growth of young leaves, and a slower, ionic phase that accelerates senescence of mature leaves. Plant adaptations to salinity are of three distinct types: osmotic stress tolerance, Na(+) or Cl() exclusion, and the tolerance of tissue to accumulated Na(+) or Cl(). Our understanding of the role of the HKT gene family in Na(+) exclusion from leaves is increasing, as is the understanding of the molecular bases for many other transport processes at the cellular level. However, we have a limited molecular understanding of the overall control of Na(+) accumulation and of osmotic stress tolerance at the whole-plant level. Molecular genetics and functional genomics provide a new opportunity to synthesize molecular and physiological knowledge to improve the salinity tolerance of plants relevant to food production and environmental sustainability.
DOI:10.1073/pnas.0910798107URLPMID:20212128 [本文引用: 1]

Soil salinity limits agricultural production and is a major obstacle for feeding the growing world population. We used natural genetic variation in salt tolerance among different Arabidopsis accessions to map a major quantitative trait locus (QTL) for salt tolerance and abscisic acid (ABA) sensitivity during seed germination and early seedling growth. A recombinant inbred population derived from Landsberg erecta (Ler; salt and ABA sensitive) x Shakdara (Sha; salt and ABA resistant) was used for QTL mapping. High-resolution mapping and cloning of this QTL, Response to ABA and Salt 1 (RAS1), revealed that it is an ABA- and salt stress-inducible gene and encodes a previously undescribed plant-specific protein. A premature stop codon results in a truncated RAS1 protein in Sha. Reducing the expression of RAS1 by transfer-DNA insertion in Col or RNA interference in Ler leads to decreased salt and ABA sensitivity, whereas overexpression of the Ler allele but not the Sha allele causes increased salt and ABA sensitivity. Our results suggest that RAS1 functions as a negative regulator of salt tolerance during seed germination and early seedling growth by enhancing ABA sensitivity and that its loss of function contributes to the increased salt tolerance of Sha.
DOI:10.1104/pp.124.3.941URL [本文引用: 1]
URLPMID:17092313 [本文引用: 1]
DOI:10.1074/jbc.M111.279331URL [本文引用: 1]
DOI:10.1111/nph.14966URLPMID:29332310 [本文引用: 1]

Content Summary 414 I. Introduction 415 II. Ca(2+) importer and exporter in plants 415 III. The Ca(2+) decoding toolkit in plants 415 IV. Mechanisms of Ca(2+) signal decoding 417 V. Immediate Ca(2+) signaling in the regulation of ion transport 418 VI. Ca(2+) signal integration into long-term ABA responses 419 VII Integration of Ca(2+) and hormone signaling through dynamic complex modulation of the CCaMK/CYCLOPS complex 420 VIII Ca(2+) signaling in mitochondria and chloroplasts 422 IX A view beyond recent advances in Ca(2+) imaging 423 X Modeling approaches in Ca(2+) signaling 424 XI Conclusions: Ca(2+) signaling a still young blooming field of plant research 424 Acknowledgements 425 ORCID 425 References 425 SUMMARY: Temporally and spatially defined changes in Ca(2+) concentration in distinct compartments of cells represent a universal information code in plants. Recently, it has become evident that Ca(2+) signals not only govern intracellular regulation but also appear to contribute to long distance or even organismic signal propagation and physiological response regulation. Ca(2+) signals are shaped by an intimate interplay of channels and transporters, and during past years important contributing individual components have been identified and characterized. Ca(2+) signals are translated by an elaborate toolkit of Ca(2+) -binding proteins, many of which function as Ca(2+) sensors, into defined downstream responses. Intriguing progress has been achieved in identifying specific modules that interconnect Ca(2+) decoding proteins and protein kinases with downstream target effectors, and in characterizing molecular details of these processes. In this review, we reflect on recent major advances in our understanding of Ca(2+) signaling and cover emerging concepts and existing open questions that should be informative also for scientists that are currently entering this field of ever-increasing breath and impact.
DOI:10.1046/j.1365-313x.2000.00787.xURLPMID:10929125 [本文引用: 1]

A rice gene encoding a calcium-dependent protein kinase (CDPK), OsCDPK7, was induced by cold and salt stresses. To elucidate the physiological function of OsCDPK7, we generated transgenic rice plants with altered levels of the protein. The extent of tolerance to cold and salt/drought stresses of these plants correlated well with the level of OsCDPK7 expression. Therefore, OsCDPK7 was shown to be a positive regulator commonly involved in the tolerance to both stresses in rice. Over-expression of OsCDPK7 enhanced induction of some stress-responsive genes in response to salinity/drought, but not to cold. Thus, it was suggested that the downstream pathways leading to the cold and salt/drought tolerance are different from each other. It seems likely that at least two distinct pathways commonly use a single CDPK, maintaining the signalling specificity through unknown post-translational regulation mechanisms. These results demonstrate that simple manipulation of CDPK activity has great potential with regard to plant improvement.
DOI:10.1105/tpc.021311URLPMID:15208400 [本文引用: 1]

Calcium plays a pivotal role in plant responses to several stimuli, including pathogens, abiotic stresses, and hormones. However, the molecular mechanisms underlying calcium functions are poorly understood. It is hypothesized that calcium serves as second messenger and, in many cases, requires intracellular protein sensors to transduce the signal further downstream in the pathways. The calcineurin B-like proteins (CBLs) represent a unique family of calcium sensors in plant cells. Here, we report our analysis of the CBL9 member of this gene family. Expression of CBL9 was inducible by multiple stress signals and abscisic acid (ABA) in young seedlings. When CBL9 gene function was disrupted in Arabidopsis thaliana plants, the responses to ABA were drastically altered. The mutant plants became hypersensitive to ABA in the early developmental stages, including seed germination and post-germination seedling growth. In addition, seed germination in the mutant also showed increased sensitivity to inhibition by osmotic stress conditions produced by high concentrations of salt and mannitol. Further analyses indicated that increased stress sensitivity in the mutant may be a result of both ABA hypersensitivity and increased accumulation of ABA under the stress conditions. The cbl9 mutant plants showed enhanced expression of genes involved in ABA signaling, such as ABA-INSENSITIVE 4 and 5. This study has identified a calcium sensor as a common element in the ABA signaling and stress-induced ABA biosynthesis pathways.
URLPMID:14617077 [本文引用: 1]
URLPMID:11006339 [本文引用: 1]
DOI:10.1104/pp.16.00949URLPMID:27899535 [本文引用: 1]

Calcineurin B-like interacting protein kinases (CIPKs) decode calcium signals upon interaction with the calcium sensors calcineurin B like proteins into phosphorylation events that result into adaptation to environmental stresses. Few phosphorylation targets of CIPKs are known and therefore the molecular mechanisms underlying their downstream output responses are not fully understood. Tomato (Solanum lycopersicum) Cipk6 regulates immune and susceptible Programmed cell death in immunity transforming Ca(2+) signals into reactive oxygen species (ROS) signaling. To investigate SlCipk6-induced molecular mechanisms and identify putative substrates, a yeast two-hybrid approach was carried on and a protein was identified that contained a Universal stress protein (Usp) domain present in bacteria, protozoa and plants, which we named
DOI:10.1105/tpc.18.00568URLPMID:30962395 [本文引用: 1]

Saline-alkali soil is a major environmental constraint impairing plant growth and crop productivity. In this study, we identified a Ca(2+) sensor/kinase/plasma membrane (PM) H(+)-ATPase module as a central component conferring alkali tolerance in Arabidopsis (Arabidopsis thaliana). We report that the SCaBP3 (SOS3-LIKE CALCIUM BINDING PROTEIN3)/CBL7 (CALCINEURIN B-LIKE7) loss-of-function plants exhibit enhanced stress tolerance associated with increased PM H(+)-ATPase activity and provide fundamental mechanistic insights into the regulation of PM H(+)-ATPase activity. Consistent with the genetic evidence, interaction analyses, in vivo reconstitution experiments, and determination of H(+)-ATPase activity indicate that interaction of the Ca(2+) sensor SCaBP3 with the C-terminal Region I domain of the PM H(+)-ATPase AHA2 (Arabidopsis thaliana PLASMA MEMBRANE PROTON ATPASE2) facilitates the intramolecular interaction of the AHA2 C terminus with the Central loop region of the PM H(+)-ATPase to promote autoinhibition of H(+)-ATPase activity. Concurrently, direct interaction of SCaPB3 with the kinase PKS5 (PROTEIN KINASE SOS2-LIKE5) stabilizes the kinase-ATPase interaction and thereby fosters the inhibitory phosphorylation of AHA2 by PKS5. Consistently, yeast reconstitution experiments and genetic analysis indicate that SCaBP3 provides a bifurcated pathway for coordinating intramolecular and intermolecular inhibition of PM H(+)-ATPase. We propose that alkaline stress-triggered Ca(2+) signals induce SCaBP3 dissociation from AHA2 to enhance PM H(+)-ATPase activity. This work illustrates a versatile signaling module that enables the stress-responsive adjustment of plasma membrane proton fluxes.
DOI:10.1105/tpc.000596URLPMID:12045276 [本文引用: 1]
DOI:10.1016/j.devcel.2019.02.010URLPMID:30861376 [本文引用: 1]

Calcium signals act as universal second messengers that trigger many cellular processes in animals and plants, but how specific calcium signals are generated is not well understood. In this study, we determined that AtANN4, a putative calcium-permeable transporter, and its interacting proteins, SCaBP8 and SOS2, generate a calcium signal under salt stress, which initially activates the SOS pathway, a conserved mechanism that modulates ion homeostasis in plants under salt stress. After activation, SCaBP8 promotes the interaction of protein kinase SOS2 with AtANN4, which enhances its phosphorylation by SOS2. This phosphorylation of AtANN4 further increases its interaction with SCaBP8. Both the interaction with and phosphorylation of AtANN4 repress its activity and alter calcium transients and signatures in HEK cells and plants. Our results reveal how downstream targets are required to create a specific calcium signal via a negative feedback regulatory loop, thereby enhancing our understanding of the regulation of calcium signaling.
中国农业大学研究生院博士学位论文,
[本文引用: 2]
PhD Dissertation of China Agricultural University,
[本文引用: 2]
DOI:10.1016/j.plantsci.2016.09.011URLPMID:27968980 [本文引用: 2]

In plants, calcineurin B-like proteins (CBLs) play crucial roles in regulating calcium-signaling in response to various abiotic stresses by interacting with specific CBL-interacting protein kinases (CIPKs). However, the identities and functions of CBL gene family members in maize are largely unknown. Here, we identified from the maize genome 12 CBL genes. All 12 CBLs have conserved EF-hand domains, and half harbor myristoylation motifs. We further characterized the function of one CBL gene, ZmCBL9, which can be induced by salt, dehydration, glucose and abscisic acid (ABA) treatments. Overexpression of ZmCBL9 enhanced resistance or tolerance to ABA, glucose, salt and osmotic stress in Arabidopsis and complemented the hypersensitive phenotype of the Arabidopsis cbl9 mutant in response to ABA and abiotic stress. The ZmCBL9 gene negatively regulates the expression of genes in the ABA signaling, biosynthesis and catabolism pathways. Moreover, the ZmCBL9 protein is found to interact with eight maize CIPKs and these ZmCIPK genes were up-regulated by different stress treatments, including salt, dehydration, glucose, low potassium and ABA. These results suggest that ZmCBL9 may interact with various ZmCIPKs to regulate the abiotic stress and ABA response signaling in plants.
DOI:10.1104/pp.17.00445URLPMID:28526703 [本文引用: 1]

Genetic and environmental factors affect bread wheat (Triticum aestivum) plant architecture, which determines grain yield. In this study, we demonstrate that miR156 controls bread wheat plant architecture. We show that overexpression of tae-miR156 in bread wheat cultivar Kenong199 leads to increased tiller number and severe defects in spikelet formation, probably due to the tae-miR156-mediated repression of a group of SQUAMOSA PROMOTER BINDING PROTEIN-LIKE (SPL) genes. Furthermore, we found that the expression of two genes TEOSINTE BRANCHED1 (TaTB1) and BARREN STALK1 (TaBA1), whose orthologous genes in diverse plant species play conserved roles in regulating plant architecture, is markedly reduced in the tae-miR156-OE bread wheat plants. Significantly, we demonstrate that the strigolactone (SL) signaling repressor DWARF53 (TaD53), which physically associates with the transcriptional corepressor TOPLESS, can directly interact with the N-terminal domains of miR156-controlled TaSPL3/17. Most importantly, TaSPL3/17-mediated transcriptional activation of TaBA1 and TaTB1 can be largely repressed by TaD53 in the transient expression system. Our results reveal potential association between miR156-TaSPLs and SL signaling pathways during bread wheat tillering and spikelet development.
DOI:10.1016/j.jcg.2011.01.005URLPMID:21356527 [本文引用: 3]

Calcium (Ca) plays a crucial role as a second messenger in intracellular signaling elicited by developmental and environmental cues. Calcineurin B-like proteins (CBLs) and their target proteins, CBL-interacting protein kinases (CIPKs) have emerged as a key Ca(2+)-mediated signaling network in response to stresses in plants. Bioinformatic analysis was used to identify 43 putative ZmCIPK (Zea mays CIPK) genes in the genome of maize inbred line B73. Based on gene structures, these ZmCIPKs were divided into intron-rich and intron-poor groups. Phylogenetic analysis indicated that the ZmCIPK family had a high evolutionary relationship with the rice CIPK family of 30 members. Microarray data and RT-PCR assay showed that ZmCIPK genes transcriptionally responded to abiotic stresses, and that 24, 31, 20 and 19 ZmCIPK genes were up-regulated by salt, drought, heat and cold stresses, respectively. There were different expression patterns of ZmCIPKs between cold-tolerant inbred line B73 and cold-sensitive inbred line Mo17 under cold stress. Our findings will aid further molecular dissection of biological functions of the CIPKs in maize, and provide new insight into the CBL-CIPK signaling network in plants.
URLPMID:10725350 [本文引用: 1]
DOI:10.1111/j.1469-8137.2009.02938.xURLPMID:19860013 [本文引用: 2]

Calcium serves as a versatile messenger in many adaptation and developmental processes in plants. Cellular calcium signals are detected and transmitted by calcium-binding proteins functioning as sensor molecules. The family of calcineurin B-like (CBL) proteins represents a unique group of calcium sensors and contributes to the decoding of calcium transients by interacting with and regulating the family of CBL-interacting protein kinases (CIPKs). In higher plants, CBL proteins and CIPKs form a complex signaling network that allows for flexible but specific signal-response coupling during environmental adaptation reactions. This review presents novel findings concerning the evolution of this signaling network and key insights into the physiological function of CBL-CIPK complexes. These aspects will be presented and discussed in the context of emerging functional principles governing efficient and specific information processing in this signaling system.
URLPMID:31819228 [本文引用: 1]
DOI:10.1016/j.tplants.2008.10.005URLPMID:19054707 [本文引用: 1]

Calcium (Ca2+) is a ubiquitous second messenger in all eukaryotes. An outstanding question is how this cation serves as a messenger for numerous signals and confers specific cellular responses. Recent studies have established a concept termed 'Ca2+ signature' that specifies Ca2+ changes triggered by each signal. How do cells recognize these signatures (codes) and translate them into the correct cellular responses? The initial step in this 'decoding' process involves sensor proteins that bind Ca2+ and activate the downstream targets, thereby regulating the specific biochemical processes. Here, I review and discuss a set of Ca2+ sensors (calcineurin B-like proteins [CBLs]) and their targets (CBL-interacting protein kinases [CIPKs]) as an emerging paradigm for Ca2+ decoding in plants. The principles governing the action of the CBL-CIPK signaling network could be generally applicable to many other signaling networks in plants and other organisms.
[本文引用: 1]
DOI:10.3390/genes7090062URL [本文引用: 1]
DOI:10.1105/tpc.106.042291URLPMID:17449811 [本文引用: 1]

The SOS (for Salt Overly Sensitive) pathway plays essential roles in conferring salt tolerance in Arabidopsis thaliana. Under salt stress, the calcium sensor SOS3 activates the kinase SOS2 that positively regulates SOS1, a plasma membrane sodium/proton antiporter. We show that SOS3 acts primarily in roots under salt stress. By contrast, the SOS3 homolog SOS3-LIKE CALCIUM BINDING PROTEIN8 (SCABP8)/CALCINEURIN B-LIKE10 functions mainly in the shoot response to salt toxicity. While root growth is reduced in sos3 mutants in the presence of NaCl, the salt sensitivity of scabp8 is more prominent in shoot tissues. SCABP8 is further shown to bind calcium, interact with SOS2 both in vitro and in vivo, recruit SOS2 to the plasma membrane, enhance SOS2 activity in a calcium-dependent manner, and activate SOS1 in yeast. In addition, sos3 scabp8 and sos2 scabp8 display a phenotype similar to sos2, which is more sensitive to salt than either sos3 or scabp8 alone. Overexpression of SCABP8 in sos3 partially rescues the sos3 salt-sensitive phenotype. However, overexpression of SOS3 fails to complement scabp8. These results suggest that SCABP8 and SOS3 are only partially redundant in their function, and each plays additional and unique roles in the plant salt stress response.
DOI:10.1093/mp/ssn003URLPMID:19825536 [本文引用: 1]

Calcium plays a vital role as a second messenger in many signaling pathways in plants. The calcineurin B-like proteins (CBLs) represent a family of plant calcium-binding proteins that function in calcium signaling by interacting with their interacting protein kinases (CIPKs). In our previous study, we have reported a role for one of the CBLs (CBL9) and one of the CIPKs (CIPK3) in ABA signaling. Here, we have shown that CBL9 and CIPK3 physically and functionally interact with each other in regulating the ABA responses. The CBL9 and CIPK3 proteins interacted with each other in the yeast two-hybrid system and when expressed in plant cells. The double mutant cbl9cipk3 showed the similar hypersensitive response to ABA as observed in single mutants (cbl9 or cipk3). The constitutively active form of CIPK3 genetically complemented the cbl9 mutant, indicating that CIPK3 function downstream of CBL9. Based on these findings, we conclude that CBL9 and CIPK3 act together in the same pathway for regulating ABA responses.
DOI:10.1105/tpc.13.6.1383URLPMID:11402167 [本文引用: 1]

The SOS3 (for SALT OVERLY SENSITIVE3) calcium binding protein and SOS2 protein kinase are required for sodium and potassium ion homeostasis and salt tolerance in Arabidopsis. We have shown previously that SOS3 interacts with and activates the SOS2 protein kinase. We report here the identification of a SOS3 binding motif in SOS2 that also serves as the kinase autoinhibitory domain. Yeast two-hybrid assays as well as in vitro binding assays revealed a 21-amino acid motif in the regulatory domain of SOS2 that is necessary and sufficient for interaction with SOS3. Database searches revealed a large family of SOS2-like protein kinases containing such a SOS3 binding motif. Using a yeast two-hybrid system, we show that these SOS2-like kinases interact with members of the SOS3 family of calcium binding proteins. Two-hybrid assays also revealed interaction between the N-terminal kinase domain and the C-terminal regulatory domain within SOS2, suggesting that the regulatory domain may inhibit kinase activity by blocking substrate access to the catalytic site. Removal of the regulatory domain of SOS2, including the SOS3 binding motif, resulted in constitutive activation of the protein kinase, indicating that the SOS3 binding motif can serve as a kinase autoinhibitory domain. Constitutively active SOS2 that is SOS3 independent also was produced by changing Thr(168) to Asp in the activation loop of the SOS2 kinase domain. Combining the Thr(168)-to-Asp mutation with the autoinhibitory domain deletion created a superactive SOS2 kinase. These results provide insights into regulation of the kinase activities of SOS2 and the SOS2 family of protein kinases.
DOI:10.1126/science.280.5371.1943URLPMID:9632394 [本文引用: 1]

Excessive sodium (Na+) in salinized soils inhibits plant growth and development. A mutation in the SOS3 gene renders Arabidopsis thaliana plants hypersensitive to Na+-induced growth inhibition. SOS3 encodes a protein that shares significant sequence similarity with the calcineurin B subunit from yeast and neuronal calcium sensors from animals. The results suggest that intracellular calcium signaling through a calcineurin-like pathway mediates the beneficial effect of calcium on plant salt tolerance.