
Characteristics of annual climate resource distribution and utilization in high-yielding winter wheat-summer maize double cropping system
ZHOU Bao-Yuan, MA Wei, SUN Xue-Fang, DING Zai-Song, LI Cong-Feng, ZHAO Ming
通讯作者:
收稿日期:2018-09-22接受日期:2019-01-12网络出版日期:2019-02-01
基金资助: |
Received:2018-09-22Accepted:2019-01-12Online:2019-02-01
Fund supported: |
作者简介 About authors

摘要
关键词:
Abstract
Keywords:
PDF (385KB)元数据多维度评价相关文章导出EndNote|Ris|Bibtex收藏本文
本文引用格式
周宝元, 马玮, 孙雪芳, 丁在松, 李从锋, 赵明. 冬小麦-夏玉米高产模式周年气候资源分配与利用特征研究[J]. 作物学报, 2019, 45(4): 589-600. doi:10.3724/SP.J.1006.2019.81067
ZHOU Bao-Yuan, MA Wei, SUN Xue-Fang, DING Zai-Song, LI Cong-Feng, ZHAO Ming.
在我国耕地面积不断下降、水资源日益短缺的情况下[1], 提高作物单位面积产量, 最大限度挖掘作物的生产潜力和气候资源利用效率, 是确保我国的粮食安全和农业可持续发展的重要途径。近年来, 我国高产栽培理论与技术研究取得了重要进展, 主要粮食作物高产纪录不断出现[2,3,4,5]。黄淮海平原是我国重要的粮食产区, 也是典型的两熟制区域, 冬小麦-夏玉米为该区主要种植模式, 在该区高产创建过程中, 冬小麦-夏玉米周年产量达20,000 kg hm-2以上。其中河南冬小麦产量最高, 达到12,315 kg hm-2以上, 山东夏玉米产量最高, 达到14,000 kg hm-2以上, 对该区冬小麦-夏玉米整体产量水平的提升起到了重要的引领作用。
作物高产的形成是一个非常复杂的过程, 受环境、作物和措施三因素共同作用的影响[6,7,8]。由于黄淮海冬小麦-夏玉米模式高产产量是在两季光温资源合理分配, 与作物生长发育匹配度较高, 且水肥资源供应较充足的条件下获得的[9], 因此生态条件是决定作物高产形成的最主要因素。然而, 近年来受全球气候变化影响, 黄淮海地区秋、冬季温度持续增加[10,11], 日照时数减少, 干旱、涝渍灾害频发, 严重影响作物生长发育。另外, 气候资源的变化造成了传统冬小麦-夏玉米模式季节间气候资源配置不合理, 使得作物品种、播期、密度、生育期等与光、温、水资源变化不匹配, 进而影响作物的生长发育[12,13,14,15], 限制了周年产量及资源利用效率的进一步提升。为合理配置冬小麦-夏玉米两季间气候资源, 实现作物生长发育与光温水等资源的匹配, 20世纪90年代王树安[16,17]在华北平原建立了冬小麦-夏玉米“双晚”技术模式, 即将冬小麦播种期由10月初推迟至10月中旬, 夏玉米收获期由9月中旬推迟至9月底, 其周年产量达到15,000 kg hm-2以上, 光、温资源生产力分别提高64%和124%。Sun等[18]和付雪丽等[19]研究也证明, 在保持原有投入成本不变的情况下, 夏玉米收获期和冬小麦播期分别推迟5~7 d, 可显著提高周年产量和光温水资源利用效率。可见, 在不增加任何投入的基础上通过播/收期的调节实现冬小麦-夏玉米季节间气候资源的优化配置, 是进一步提升黄淮海周年产量和资源利用效率的重要途径。然而, 当前通过改变播/收期来调节冬小麦-夏玉米周年气候资源配置的方法尚缺乏相应的理论支撑和定量化指标的指导, 且由于区域间气候条件差异造成播/收期变化较大, 难以形成整个黄淮海区冬小麦-夏玉米模式统一的资源优化配置定量标准, 从而限制了该技术的大面积精准应用。
本研究定量分析9个高产点共45个面积3.3 hm2以上的高产典型的冬小麦-夏玉米模式产量和生育期间气候资源数据, 明确黄淮海不同生态区该模式周年气候资源分配定量化特征, 及其高产形成与气候资源分配的定量关系, 并建立季节间资源分配率与资源分配比值等定量化指标及其相应的定量标准, 以期为进一步挖掘黄淮海区周年产量潜力和气候资源利用效率提供理论依据。
1 材料与方法
1.1 试验数据来源
主要来源于2006—2010年国家粮食丰产工程中黄淮海区9个代表性高产点共45个田间试验的冬小麦-夏玉米周年高产数据, 包括产量和生育期。1.2 试验地概况
试验地点为河南浚县、兰考和温县, 山东兖州、滕州、诸城和莱州, 河北吴桥和藁城, 均属暖温带大陆性季风气候, 年平均气温14°C, 全年≥10°C积温4647.2°C, 年降水量573.4 mm, 且多在7、8月间, 年日照时数2323.9 h, 能够基本满足冬小麦-夏玉米一年两熟种植。选用当地土壤条件较好的田块作试验田, 土壤类型、基础地力情况详见表1。Table 1
表1
表1各高产地块土壤条件
Table 1
地点 Experiment site | 土壤质地 Soil texture | 土层深度 Soil depth (cm) | pH | 全氮 Total N (%) | 碱解氮 Effective N (mg kg-1) | 速效磷 Effective P (mg kg-1) | 速效钾 Effective K (mg kg-1) | 有机质 Organic matter (%) |
---|---|---|---|---|---|---|---|---|
山东莱州 Laizhou, Shandong | 黏壤土 Clay loam | 0-10 | 6.9 | 0.077 | 66.5 | 61.9 | 130.0 | 1.6 |
10-20 | 7.1 | 0.088 | 70.8 | 64.9 | 110.0 | 1.4 | ||
山东滕州 Tengzhou, Shandong | 潮土 Moisture soil | 0-10 | 7.1 | 0.076 | 63.8 | 73.9 | 132.1 | 1.6 |
10-20 | 6.9 | 0.089 | 66.0 | 83.8 | 115.3 | 1.2 | ||
山东诸城 Zhucheng, Shandong | 黏壤土 Clay loam | 0-10 | 6.8 | 0.092 | 76.0 | 34.6 | 120.3 | 1.4 |
10-20 | 7.0 | 0.086 | 84.4 | 36.1 | 105.0 | 1.5 | ||
山东兖州 Yanzhou, Shandong | 壤土 Loam soil | 0-10 | 6.8 | 0.083 | 74.0 | 31.4 | 110.0 | 1.7 |
10-20 | 7.0 | 0.064 | 58.5 | 24.2 | 70.0 | 1.1 | ||
河北吴桥 Wuqiao, Hebei | 壤土 Loam soil | 0-10 | 7.8 | 0.066 | 76.2 | 38.3 | 160.0 | 1.6 |
10-20 | 8.1 | 0.075 | 51.4 | 15.1 | 86.7 | 2.1 | ||
河北藁城 Gaocheng, Hebei | 壤土 Loam soil | 0-10 | 7.2 | 0.091 | 60.0 | 16.6 | 105.0 | 2.3 |
10-20 | 7.0 | 0.095 | 49.2 | 18.5 | 80.0 | 2.4 | ||
河南浚县 Xunxian, Henan | 黏壤土 Clay loam | 0-10 | 7.5 | 0.092 | 76.0 | 34.6 | 150.0 | 1.9 |
10-20 | 7.6 | 0.086 | 84.4 | 36.1 | 185.0 | 1.9 | ||
河南兰考 Lankao, Henan | 沙壤土 Sandy loam | 0-10 | 8.4 | 0.085 | 53.8 | 76.9 | 120.0 | 1.1 |
10-20 | 8.3 | 0.092 | 46.0 | 91.8 | 115.0 | 1.2 | ||
河南温县 Wenxian, Henan | 潮土 Moisture soil | 0-10 | 8.2 | 0.099 | 46.6 | 64.3 | 128.0 | 1.5 |
10-20 | 8.1 | 0.108 | 90.2 | 25.35 | 170.6 | 1.8 |
新窗口打开|下载CSV
1.3 田间管理
为充分挖掘作物的产量潜力, 在各高产点选用当地主栽的高产稳产的小麦、玉米品种, 按当地超高产栽培方式, 采用最佳作物管理方案, 最佳作物播种与收获时期、耕作方式、水肥管理措施、种植密度和种植方式等。主要种植方案见表2。Table 2
表2
表2各高产地块作物种植方案
Table 2
地点 Experiment site | 作物 Crop | 品种 Variety | 播种期 Sowing date (month/day) | 收获期 Harvest date (month/day) |
---|---|---|---|---|
山东莱州 Laizhou, Shandong | 小麦 Winter wheat | 烟农19, 烟2415 Yannong 19, Yan 2415 | 10/10-10/12 | 6/9-6/10 |
玉米Summer maize | 金海5号, 莱农14 Jinhai 5, Lainong 14 | 6/11-6/12 | 10/8-10/10 | |
山东滕州 Tengzhou, Shandong | 小麦Winter wheat | 济麦19, 鲁麦21 Jimai 19, Lumai 21 | 10/6-10/8 | 6/8-6/10 |
玉米Summer maize | 登海3号, 郑单958 Denghai 3, Zhengdan 958 | 6/9-6/11 | 10/5-10/7 | |
山东诸城 Zhucheng, Shandong | 小麦Winter wheat | 济麦20, 山农12 Jimai 20, Shannong 12 | 10/5-10/7 | 6/6-6/8 |
玉米Summer maize | 鲁单981, 登海9号 Ludan 981, Denghai 9 | 6/8-6/10 | 10/3-10/5 | |
山东兖州 Yanzhou, Shandong | 小麦Winter wheat | 济南17, 山农664 Jinan 17, Shannong 664 | 10/6-10/8 | 6/8-6/9 |
玉米Summer maize | 鲁单981, 农大108 Ludan 981, Nongda 108 | 6/10-6/12 | 10/5-10/8 | |
河北吴桥 Wuqiao, Hebei | 小麦Winter wheat | 轮选987, 石家庄8号 Lunxuan 987, Shijiazhuang 8 | 10/6-10/10 | 6/5-6/8 |
玉米Summer maize | 农大108, 蠡玉16 Nongda 108, Liyu 16 | 6/6-6/8 | 10/5-10/8 | |
河北藁城 Gaocheng, Hebei | 小麦Winter wheat | 石新828, 石麦14 Shixin 828, Shimai 14 | 10/8-10/11 | 6/8-6/10 |
玉米Summer maize | 郑单958, 蠡玉16 Xundan 20, Liyu 16 | 6/10-6/12 | 10/8-10/10 | |
河南浚县 Xunxian, Henan | 小麦Winter wheat | 周麦22, 矮抗58 Zhoumai 22, Aikang 58 | 10/10-10/12 | 6/8-6/10 |
玉米Summer maize | 浚单20, 浚单18 Xundan 20, Xundan 18 | 6/9-6/12 | 10/8-10/10 | |
河南兰考 Lankao, Henan | 小麦Winter wheat | 兰考矮早八, 周麦16 Lankaoaizao 8, Zhoumai 16 | 10/13-10/16 | 6/9-6/10 |
玉米Summer maize | 浚单22, 郑单958 Xundan 22, Zhengdan 958 | 6/9-6/12 | 10/10-10/12 | |
河南温县 Wenxian, Henan | 小麦Winter wheat | 豫麦49-198, 周麦18 Yumai 49-198, Zhoumai 18 | 10/15-10/18 | 6/8-6/10 |
玉米Summer maize | 浚单20, 先玉335 Xundan 20, Xianyu 335 | 6/10-6/12 | 10/12-10/15 |
新窗口打开|下载CSV
1.4 气象数据及其测定指标
气象数据来源于国家气象局网站(http://www.cma. gov.cn/)。主要包括平均气温、日照时数和降雨量等指标。表3为各试验点及其对应的气象台站的地理位置。Table 3
表3
表3高产地块地理分布及相应气象台站位置
Table 3
试验点 Experiment site | 经度 Longitude (E) | 纬度 Latitude (N) | 海拔 Altitude (m) | 气象站点 Weather station | 经度 Longitude (E) | 纬度 Latitude (N) | 海拔 Altitude (m) |
---|---|---|---|---|---|---|---|
莱州 Laizhou | 119.94 | 37.18 | 48.35 | 龙口 Longkou | 120.20 | 37.38 | 28.42 |
滕州 Tengzhou | 117.16 | 35.08 | 69.81 | 滕州 Tengzhou | 117.12 | 35.06 | 74.89 |
诸城 Zhucheng | 119.40 | 35.59 | 64.77 | 日照 Rizhao | 119.52 | 35.42 | 37.26 |
兖州 Yanzhou | 116.40 | 35.41 | 46.10 | 兖州 Yanzhou | 116.51 | 35.34 | 51.70 |
吴桥 Wuqiao | 116.39 | 37.63 | 20.18 | 陵县 Lingxian | 116.57 | 37.34 | 22.72 |
藁城 Gaocheng | 114.84 | 38.02 | 58.75 | 石家庄 Shijiazhuang | 114.51 | 38.04 | 84.01 |
浚县 Xunxian | 114.55 | 35.68 | 62.79 | 安阳 Anyang | 114.39 | 36.10 | 62.90 |
兰考 Lankao | 114.81 | 34.82 | 66.27 | 开封 Kaifeng | 114.30 | 34.80 | 75.56 |
温县 Wenxian | 113.07 | 34.94 | 108.70 | 温县 Wenxian | 113.02 | 34.57 | 106.40 |
新窗口打开|下载CSV
1.4.1 季节间资源分配率与资源分配比值 为了定量指导两熟制季节间资源分配, 提出了资源分配率和资源分配比值等指标, 并建立了相应的计算公式。
积温分配率(TDR) = 季节内积温量(Tx)/周年积温总量(T)
辐射分配率(RDR) = 季节内辐射量(Rx)/周年辐射总量(R)
降雨分配率(PDR) = 季节内降雨量(Px)/周年降雨总量(P)
积温比值(TR) = 第一季积温量(T1)/第二季积温量(T2)
辐射比值(RR) = 第一季辐射量(R1)/第二季辐射量(R2)
降雨比值(PR) = 第一季降雨量(P1)/第二季降雨量(P2)
太阳总辐射Q = Q0 (a+bS/S0)
式中, Q为太阳总辐射, Q0为天文辐射, S为实测日照时数, S0为太阳可照时数, S/S0为日照百分率, a、b为待定系数[20]。
积温计算过程中, 小麦季下限温度取值为0°C, 玉米季下限温度取值为10°C [21]。
1.4.2 光、温、水生产效率 按下面公式计算光、温、水生产效率[12,22]。
光能生产效率(g MJ-1) = 籽粒产量/单位面积的太阳辐射
积温生产效率(kg hm-2 °C-1) = 单位面积籽粒产量/生育期间积温总量
降水生产效率(kg hm-2 mm-1) = 籽粒产量/单位面积的降水量
1.5 数据分析
利用Microsoft Excel 2007和SPSS 16.0软件处理和统计分析数据, 采用SigmaPlot 10.0软件作图。2 结果与分析
2.1 高产冬小麦-夏玉米模式周年籽粒产量
由表4可以看出, 河南、山东和河北三省冬小麦-夏玉米周年产量均达到20,000 kg hm-2以上, 其中河南(23,805.3 kg hm-2)和山东(24,741.9 kg hm-2)周年产量差异不显著, 但显著高于河北(20,359.3 kg hm-2), 增幅分别为16.9%和21.5%。对于单季作物来说, 河南冬小麦产量最高, 平均为10,626.9 kg hm-2, 与山东差异不显著, 显著高于河北, 增幅分别为13.0%和10.5%; 山东夏玉米产量最高, 为14,350.4 kg hm-2, 分别高于河南和河北8.9%和31.0%。Table 4
表4
表42006-2010年不同试验点冬小麦-夏玉米模式高产产量
Table 4
试验点 Experiment site | 冬小麦 Winter wheat | 夏玉米 Summer maize | 周年 Annual | |
---|---|---|---|---|
河南 Henan | 浚县Xunxian | 10875.0 | 13678.9 | 24553.9 |
兰考Lankao | 10577.3 | 12993.0 | 23570.3 | |
温县Wenxian | 10428.5 | 12863.3 | 23291.8 | |
山东 Shandong | 兖州Yanzhou | 10434.3 | 14977.9 | 25412.2 |
滕州Tengzhou | 10857.5 | 13983.3 | 24840.8 | |
诸城Zhucheng | 10256.1 | 12763.2 | 23019.3 | |
莱州Laizhou | 10018.5 | 15677.1 | 25695.6 | |
河北 Hebei | 吴桥Wuqiao | 9356.5 | 11297.4 | 20653.9 |
藁城Gaocheng | 9459.0 | 10605.6 | 20064.6 | |
平均值 Mean | 河南Henan | 10626.9 a | 13178.4 b | 23805.3 a |
山东Shandong | 10391.6 a | 14350.4 a | 24741.9 a | |
河北Hebei | 9407.8 b | 10951.5 c | 20359.3 b |
新窗口打开|下载CSV
各省不同试验田之间小麦产量变异较小, 三省小麦产量变幅分别为446.5 kg hm-2(河南)、639.0 kg hm-2 (山东)和102.5 kg hm-2 (河北); 而与小麦比, 玉米产量变异较大, 三省玉米产量变幅分别为2138.2 kg hm-2 (河南)、2913.9 kg hm-2 (山东)和691.8 kg hm-2 (河北)。以上结果表明, 黄淮海高产冬小麦-夏玉米产量区域间差异较大, 尤其是玉米产量的变幅最大, 小麦产量变异较小。由于高产田采取的是最佳的土壤和作物管理方案, 作物生长不受水、肥、病虫草害的限制, 因此, 地区间产量的差异主要来自于光温等生态条件的差异。
2.2 高产冬小麦-夏玉米模式季节间光、温、水资源分配
黄淮海地区不同省份之间积温变异较大(表5)。三省中河南冬小麦-夏玉米模式周年积温量最高, 为5488.6°C, 其次是山东(5245.8°C), 河北最低(5000.9°C), 变幅为469.1°C, 差异显著。对于单季作物来说, 河南小麦季分配积温量最高, 为2346.2°C, 显著高于山东(2263.6°C)和河北(2135.0°C), 变幅为211.2°C, 且山东与河北之间也存在显著差异。与小麦季趋势相同, 三省玉米季积温量分别为3142.3°C (河南)、2983.0°C (山东)和2865.9°C (河北), 差异显著, 变幅为276.4°C。Table 5
表5
表5冬小麦-夏玉米模式季节间积温分配
Table 5
试验点 Experiment site | 冬小麦 Winter wheat | 夏玉米 Summer maize | 周年Annual | ||||||
---|---|---|---|---|---|---|---|---|---|
积温 AT (°C) | 分配率 TDR (%) | 积温 AT (°C) | 分配率 TDR (%) | 积温 AT (°C) | 两季比 TR | ||||
河南 Henan | 浚县Xunxian | 2229.2 | 42 | 3062.4 | 58 | 5291.6 | 0.7 | ||
兰考Lankao | 2452.9 | 43 | 3219.5 | 57 | 5672.4 | 0.8 | |||
温县Wenxian | 2356.6 | 43 | 3145.2 | 57 | 5501.8 | 0.7 | |||
山东 Shandong | 兖州Yanzhou | 2233.9 | 43 | 2985.5 | 57 | 5219.4 | 0.7 | ||
滕州Tengzhou | 2249.6 | 43 | 2999.8 | 57 | 5249.4 | 0.7 | |||
诸城Zhucheng | 2295.4 | 44 | 2962.8 | 56 | 5258.2 | 0.8 | |||
莱州Laizhou | 2275.6 | 43 | 2980.4 | 57 | 5256.0 | 0.7 | |||
河北 Hebei | 吴桥Wuqiao | 2122.8 | 43 | 2838.2 | 57 | 4961.0 | 0.7 | ||
藁城Gaocheng | 2157.1 | 43 | 2883.6 | 57 | 5040.7 | 0.7 | |||
平均值 Mean | 河南Henan | 2346.2 a | 43 | 3142.3 a | 57 | 5488.6 a | 0.7 | ||
山东Shandong | 2263.6 b | 43 | 2983.0 b | 57 | 5245.8 b | 0.7 | |||
河北Hebei | 2135.0 c | 43 | 2865.9 c | 57 | 5000.9 c | 0.7 |
新窗口打开|下载CSV
由表5可以看出, 相对于实际积温量, 各地区小麦、玉米季节间积温分配率(TDR)和两季间积温比值(TR)变异较小。三省小麦季积温分配率均为43%, 变异系数为1.9%; 玉米季积温分配率均为57%, 变异系数为1.1%; 两季间积温比值均为0.7。以上结果表明, 虽然各地区小麦、玉米生长季实际积温量变异较大, 但冬小麦-夏玉米季节间积温分配率和积温比值变异较小。由此可知, 黄淮海不同地区高产冬小麦-夏玉米种植模式两季积温分配率和积温比值具有统一的定量标准值, 依据此指标可指导该区冬小麦-夏玉米种植模式周年气候资源优化配置, 及评价其资源分配的合理性。
各省之间辐射量变异较大(表6)。三省中山东省冬小麦-夏玉米模式周年辐射量最高, 为4547.6 MJ m-2, 其次是河北(4494.0 MJ m-2), 河南辐射量(4132.2 MJ m-2)最低, 变幅为415.4 MJ m-2, 差异显著。对于单季作物来说, 山东小麦季辐射量最高, 为2659.7 MJ m-2, 与河北(2636.3 MJ m-2)差异不显著, 但显著高于河南(2437.7 MJ m-2), 变幅为222 MJ m-2; 与小麦季趋势相同, 山东省玉米季分配辐射量为1924.5 MJ m-2, 与河北(1857.7 MJ m-2)差异不显著, 但显著高于河南(1687.2 MJ m-2), 变幅为237.3 MJ m-2。
Table 6
表6
表6冬小麦-夏玉米模式季节间辐射分配
Table 6
试验点 Experiment site | 冬小麦 Winter wheat | 夏玉米 Summer maize | 周年 Annual | ||||||
---|---|---|---|---|---|---|---|---|---|
辐射量 Ra (MJ m-2) | 分配率 RDR (%) | 辐射量 Ra (MJ m-2) | 分配率 RDR (%) | 辐射量 Ra (MJ m-2) | 两季比 RR | ||||
河南 Henan | 浚县Xunxian | 2344.0 | 57 | 1776.1 | 43 | 4120.1 | 1.3 | ||
兰考Lankao | 2395.9 | 60 | 1616.1 | 40 | 4012.0 | 1.5 | |||
温县Wenxian | 2573.2 | 61 | 1669.5 | 39 | 4242.7 | 1.5 | |||
山东 Shandong | 兖州Yanzhou | 2629.7 | 58 | 1880.7 | 42 | 4510.4 | 1.4 | ||
滕州Tengzhou | 2591.2 | 58 | 1847.6 | 42 | 4438.8 | 1.4 | |||
诸城Zhucheng | 2714.2 | 60 | 1792.4 | 40 | 4454.7 | 1.5 | |||
莱州Laizhou | 2703.6 | 56 | 2177.2 | 44 | 4786.5 | 1.3 | |||
河北 Hebei | 吴桥Wuqiao | 2638.7 | 58 | 1852.2 | 42 | 4490.9 | 1.4 | ||
藁城Gaocheng | 2633.9 | 59 | 1863.2 | 41 | 4497.1 | 1.4 | |||
平均值 Mean | 河南Henan | 2437.7 b | 59 | 1687.2 b | 41 | 4124.9 b | 1.4 | ||
山东Shandong | 2659.7 a | 58 | 1924.5 a | 42 | 4547.6 a | 1.4 | |||
河北Hebei | 2636.3 a | 58 | 1857.7 a | 42 | 4494.0 a | 1.4 |
新窗口打开|下载CSV
由表6可以看出, 相对于实际辐射量, 各地区小麦、玉米季辐射分配率(RDR)和两季间辐射量比值(RR)变异较小。河南小麦季和玉米季辐射分配率分别为59%和41%, 两季间辐射量比值(RR)为1.4; 山东和河北小麦季辐射分配率均为58%, 玉米季辐射量分配率为42%, 两季间辐射量比值(RR)为1.4, 但三省各指标差异不显著。
由表7可以看出, 各省之间降水量变异较大。三省中山东冬小麦-夏玉米模式周年降水总量最高, 为776.0 mm, 其次是河南(554.1 mm), 河北最低(484.0 mm), 变幅为292 mm, 差异显著。对于单季作物来说, 山东小麦季降水量最高, 为193.2 mm, 显著高于河南(160.9 mm)和河北(117.5 mm); 与小麦季趋势相同, 山东省玉米季降水量为582.8 mm, 显著高于河南(393.2 mm)和河北(366.5 mm)。
与积温和辐射分配比, 各地区小麦、玉米季节间降水分配率(PDR)和两季间降水量比值(PR)变异较大, 河南、山东和河北小麦季降水量分配率分别为29%、25%和24%, 玉米季降水量分配率分别为71%、75%和76%, 两季间降水量比值(PR)分别为0.4、0.3和0.3。与积温和辐射比, 地区间降水量变异较大, 但季节间降水分配率和分配比值变异相对较小。
Table 7
表7
表7冬小麦-夏玉米模式季节间降水分配
Table 7
试验点 Experiment site | 冬小麦 Winter wheat | 夏玉米 Summer maize | 周年Annual | ||||||
---|---|---|---|---|---|---|---|---|---|
降水量 Pr (mm) | 分配率 PDR (%) | 降水量 Pr (mm) | 分配率 PDR (%) | 降水量 Pr (mm) | 两季比 PR | ||||
河南 Henan | 浚县Xunxian | 154.2 | 29 | 386.6 | 71 | 540.8 | 0.4 | ||
兰考Lankao | 161.2 | 29 | 395.1 | 71 | 556.3 | 0.4 | |||
温县Wenxian | 167.3 | 30 | 397.8 | 70 | 565.1 | 0.4 | |||
试验点 Experiment site | 冬小麦 Winter wheat | 夏玉米 Summer maize | 周年Annual | ||||||
降水量 Pr (mm) | 分配率 PDR (%) | 降水量 Pr (mm) | 分配率 PDR (%) | 降水量 Pr (mm) | 两季比 PR | ||||
山东 Shandong | 兖州Yanzhou | 172.7 | 23 | 585.6 | 77 | 758.3 | 0.3 | ||
滕州Tengzhou | 179.2 | 23 | 593.6 | 77 | 772.8 | 0.3 | |||
诸城Zhucheng | 238.4 | 27 | 643.4 | 73 | 881.8 | 0.4 | |||
莱州Laizhou | 182.6 | 26 | 508.5 | 72 | 691.1 | 0.4 | |||
河北 Hebei | 吴桥Wuqiao | 119.8 | 25 | 367.8 | 75 | 487.6 | 0.3 | ||
藁城Gaocheng | 115.2 | 24 | 365.1 | 76 | 480.3 | 0.3 | |||
平均值 Mean | 河南Henan | 160.9 b | 29 | 393.2 b | 71 | 554.1 b | 0.4 | ||
山东Shandong | 193.2 a | 25 | 582.8 a | 75 | 776.0 a | 0.3 | |||
河北Hebei | 117.5 c | 24 | 366.5 c | 76 | 484.0 c | 0.3 |
新窗口打开|下载CSV
2.3 冬小麦、夏玉米产量与光温水资源分配的定量关系
由图1可以看出, 冬小麦9000 kg hm-2以上的产量形成要求积温量为1924.2~2608°C, 辐射量为2168.5~2953.8 MJ m-2, 降水量为70.6~327.3 mm。图1
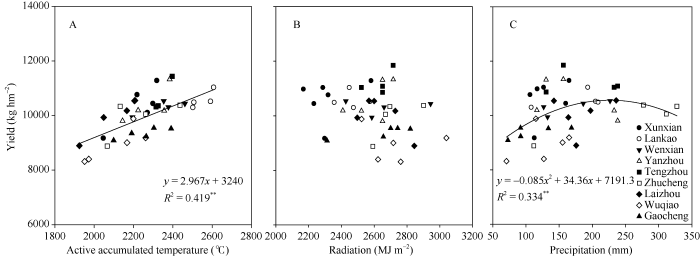
图1小麦产量与气象因子的关系**表示在0.01水平显著相关。** Significant correlation at the 0.01 probability level.
Fig. 1Relationship between wheat yield and climatic factors
小麦季积温量在1924.2~2608°C范围内, 小麦产量(y)与积温(x)呈显著线性关系(y = 2.967x+3240), 即产量随着积温量的增加而增加。降水量在70.6~327.3 mm范围内, 小麦产量(y)与降水量(x)呈二次函数的关系(y = -0.085x2+34.36x+7191.3), 即冬小麦产量随着降水量增加先升高后降低, 当降雨量为202.1 mm时, 冬小麦产量最高, 为10,663.7 kg hm-2。而辐射量在2168.5~2953.8 MJ m-2范围内, 小麦产量与辐射量无显著相关。以上结果表明, 在当前高产条件下, 冬小麦产量的形成受当季积温和降雨分配量的限制, 当降水量不超过201.1 mm时, 小麦产量随生长季积温和降水量的增加而增加。由于河南和山东小麦季积温量和降水量均高于河北, 因此小麦产量显著高于河北。
由图2可以看出,夏玉米10,500 kg hm-2以上的产量形成要求当季积温量为2759.6~3307.2°C,辐射量在1432.2 MJ m-2以上,降水量为249.3~966.7 mm。
图2
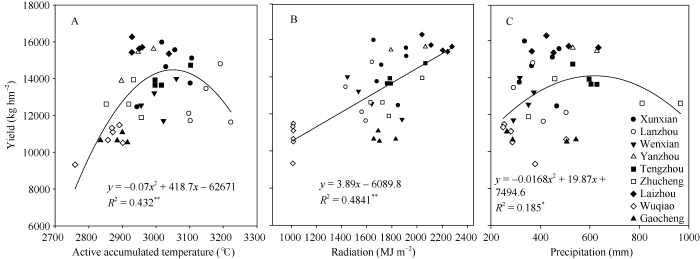
图2玉米产量与气象因子的关系
*表示在0.05水平显著相关, **表示在0.01水平显著相关。
Fig. 2Relationship between maize yield and climatic factors
* Significant correlation at the 0.05 probability level. ** Significant correlation at the 0.01 probability level.
玉米季分配积温量在2759.6~3307.2°C范围内, 玉米产量(y)与积温(x)呈二次曲线变化趋势(y = -0.0766x2+467.19x-697,988), 即随着生长季积温量增加, 玉米产量先增加后降低, 当生长季积温为2990.7°C时, 产量最高, 为14,366.2 kg hm-2。玉米产量(y)与辐射量(x)呈显著线性关系(y=3.7953x+ 6806.1), 即玉米季辐射量在1432.2~2278.7 MJ m-2范围内, 产量随着辐射量的增加而增加。降水量在249.3~966.7 mm范围内, 玉米产量(y)与降水量(x)呈二次函数关系(y = -0.017x2+20.994x+7604.4), 即随着生长季降水量增加, 玉米产量先增加后降低, 当降水量为591.3 mm时, 产量最高, 为14,086.2 kg hm-2。以上结果说明, 玉米高产形成受当地光温水资源限制, 在积温不超过2990.7°C、降雨量小于591.3 mm时, 增加玉米季的积温、辐射和降水的分配可显著提高玉米产量。由此可知, 由于山东玉米季辐射量最高, 积温量和降水量分别在不超过2990.7°C和591.3 mm的范围内最高, 因此山东夏玉米产量显著高于河南和河北。
2.4 小麦、玉米资源生产效率比较
由表8可以看出, 三省份小麦季积温生产效率无明显差异, 而玉米季差异显著, 山东省玉米季积温生产效率为4.81 kg hm-2 °C-1, 显著高于河南和山东, 增幅分别为14.8%和25.9%。因此, 山东省冬小麦-夏玉米周年积温生产效率最高, 为4.72 kg hm-2 °C-1, 分别高于河南和河北8.8%和16.0%。河北玉米季和周年积温生产效率最低, 均显著低于河南和山东。Table 8
表8
表8冬小麦-夏玉米模式光温水资源生产效率
Table 8
试验点 Experiment site | 积温生产效率 Production efficiency of AT (kg hm-2 °C-1) | 光能生产效率 Production efficiency of radiation (g MJ-1) | 降水生产效率 Production efficiency of precipitation (kg hm-2 mm-1) | |||||||||
---|---|---|---|---|---|---|---|---|---|---|---|---|
小麦 Wheat | 玉米 Maize | 周年 Annual | 小麦 Wheat | 玉米 Maize | 周年 Annual | 小麦 Wheat | 玉米 Maize | 周年 Annual | ||||
河南 Henan | 浚县Xunxian | 4.88 | 4.47 | 4.64 | 0.46 | 0.77 | 0.60 | 70.5 | 35.4 | 45.4 | ||
兰考Lankao | 4.31 | 4.04 | 4.16 | 0.44 | 0.80 | 0.59 | 65.6 | 32.9 | 42.4 | |||
温县Wenxian | 4.43 | 4.09 | 4.23 | 0.40 | 0.77 | 0.56 | 62.3 | 32.3 | 41.2 | |||
山东 Shandong | 兖州Yanzhou | 4.67 | 5.02 | 4.87 | 0.40 | 0.80 | 0.56 | 60.4 | 25.6 | 33.5 | ||
滕州Tengzhou | 4.83 | 4.66 | 4.73 | 0.42 | 0.76 | 0.56 | 60.6 | 23.6 | 32.1 | |||
诸城Zhucheng | 4.47 | 4.31 | 4.38 | 0.38 | 0.71 | 0.52 | 43.0 | 19.8 | 26.1 | |||
莱州Laizhou | 4.40 | 5.26 | 4.89 | 0.37 | 0.72 | 0.54 | 54.9 | 30.8 | 37.2 | |||
河北 Hebei | 吴桥Wuqiao | 4.41 | 3.98 | 4.16 | 0.35 | 0.61 | 0.46 | 78.1 | 30.7 | 42.4 | ||
藁城Gaocheng | 4.39 | 3.68 | 3.98 | 0.36 | 0.57 | 0.45 | 82.1 | 29.0 | 41.8 | |||
平均值 Mean | 河南Henan | 4.53 a | 4.19 b | 4.34 b | 0.44 a | 0.78 a | 0.59 a | 66.0 b | 33.5 a | 43.0 a | ||
山东Shandong | 4.59 a | 4.81 a | 4.72 a | 0.39 b | 0.75 a | 0.54 b | 53.8 c | 24.6 c | 31.9 b | |||
河北Hebei | 4.41 a | 3.82 c | 4.07 c | 0.36 c | 0.59 b | 0.45 c | 80.1 a | 29.9 b | 42.1 a |
新窗口打开|下载CSV
三省份中河南小麦季光能生产效率最高, 为0.44 g MJ-1, 分别高于山东和河北12.8%和22.2%, 河北小麦季光能生产效率最低; 河南和山东玉米季光能生产效率无显著差异, 但分别高于河北32.2%和27.1%, 差异显著。因此, 河南冬小麦-夏玉米周年光能生产效率最高, 为0.59 g MJ-1, 分别高于山东和河北9.3%和31.1%, 河北周年光能生产效率最低。
河北小麦季降水生产效率显著高于河南和山东, 增幅分别为21.4%和48.9%; 河南玉米季降水生产效率最高, 为33.5 kg hm-2 mm-1, 分别高于山东和河北36.2%和12.0%, 山东玉米季降水生产效率最低; 河南和河北冬小麦-夏玉米周年降水生产效率无显著差异, 但显著高于山东, 增幅分别为34.8%和32.0%。
3 讨论
在不增加任何投入的前提下, 通过优化冬小麦-夏玉米模式季节间资源配置来进一步挖掘作物产量潜力和资源利用效率, 已成为促进黄淮海平原农业可持续发展的重要途径[16,22]。由于冬小麦-夏玉米周年超高产产量是在两季光温资源合理分配, 与作物生长发育匹配度较高的条件下获得的[9], 因此研究黄淮海不同生态区冬小麦-夏玉米高产模式周年资源分配特征, 及其高产形成与气候资源分配的定量关系, 可为该区冬小麦-夏玉米模式建立统一的、定量化的资源优化配置方案提供理论依据。本研究中选取的2006—2010年河南、河北和山东三省9个代表性高产点共45个田间试验的冬小麦-夏玉米周年产量均达到20,000 kg hm-2以上水平, 可作为黄淮海区冬小麦-夏玉米模式高产代表。然而, 三省周年产量及小麦、玉米单季产量均存在显著差异。河北省无论单季还是周年产量均显著低于河南和山东, 而山东与河南的小麦季及周年产量无显著差异, 但山东夏玉米产量显著高于河南。这与前人关于黄淮海地区作物光温生产潜力的分析结果基本一致[26,27]。由于各省区高产田均采取的是土壤和作物最佳的管理方案, 作物生长不受水、肥、病虫草害的限制[9], 因此生态条件差异可能是造成区域间冬小麦-夏玉米高产产量变化的主要因素。前人研究表明, 作物产量形成与其所在地区的光温水等生态条件密切相关[14,23-25,28]。本研究分析黄淮海区多年多点冬小麦-夏玉米高产模式季节间资源分配特征发现, 区域间光温水资源差异较大, 河南省小麦、玉米单季及周年积温量最高, 山东省各季及周年降水量最高, 而河北除辐射量与山东差异不显著, 但显著高于河南外, 各季及周年的积温和降水量均显著低于河南和山东, 从而导致地区间超高产量差异较大。黄淮海地区作物光温生产潜力也有类似的变化趋势[26]。有人认为, 黄淮海平原夏玉米超高产所需的积温和日照时数均可得到满足, 但产量与降水呈负相关[29]。本研究分析不同地区夏玉米高产形成与光温水资源分配的定量关系发现, 当玉米季光温水分配量在一定范围内, 玉米产量与生长季积温量和降水量呈二次曲线变化趋势, 与辐射量呈显著线性关系, 即在积温不超过2990.7°C, 降水量小于591.3 mm, 辐射量在1432.2~2278.7 MJ m-2范围内, 玉米产量随生长季积温、辐射和降水的增加而显著提高。因此, 由于山东玉米季辐射量、积温量和降水量最高, 夏玉米产量显著高于河南和河北。另外, 小麦产量与开花至成熟期日均温和降水量呈二次曲线关系[30]。本研究分析不同地区小麦高产形成与光温水资源分配的定量关系发现, 当小麦季光温水量在一定范围内, 小麦产量与生长季积温量呈显著线性关系, 与降雨量呈二次函数的关系, 与辐射量无显著相关, 即小麦季积温量在1924.2~ 2608°C范围内, 降水量不超过201.1 mm时, 辐射量在2168.5~2953.8 MJ m-2范围内, 小麦产量随生长季积温和降水量的增加而增加。因此, 由于河南和山东小麦季分配积温量和降水量均高于河北, 小麦产量显著高于河北。
如上所述, 虽然黄淮海区冬小麦-夏玉米模式生长季光温水资源分配量差异较大, 但本研究发现冬小麦-夏玉米模式季节间资源分配率和分配比值差异相对较小, 其中积温分配变异最小, 三省积温分配率和积温比值均为统一的定量标准, 即小麦季和玉米季积温分配率分别为43%和57%, 两季间积温比值为0.7。以上指标与冬小麦-夏玉米“双晚”技术模式积温分配指标相同[22], 说明季节间资源的合理分配是冬小麦-夏玉米模式周年高产形成的重要条件。相对于积温分配率和积温比值, 三省区季节间辐射分配率和降水分配率差异较大, 河南小麦季和玉米季辐射分配率分别为59%和41%, 两季间辐射量比值(RR)为1.5, 山东和河北小麦季和玉米季辐射分配率均为58%和42%, 两季间辐射量比值(RR)为1.4; 河南、山东和河北小麦季降水分配率分别为29%、25%和24%, 玉米季降水分配率分别为71%、75%和76%, 两季间降水量比值(PR)分别为0.4、0.3和0.3。这主要是因为, 相对于积温来说, 黄淮海地区辐射量和降水量地区间、年际间变化更明显[31]。然而, 热量条件(积温)是影响植物生长发育的最主要生态因子, 其通过调节作物的生育进程, 影响光有效辐射截获量及生育期降水分布, 进而影响产量[32,33]。因此, 冬小麦-夏玉米模式季节间资源分配应以热量资源为主, 其次是辐射和降雨。生产实践中, 可依据上述定量指标标准, 根据区域冬小麦-夏玉米周年可利用积温量进行两季积温合理分配, 进而确定适宜熟期品种和两季合理播/收期。另外, 由于省份间冬小麦-夏玉米模式季节间热量资源配率和分配比值相对固定, 可以推测随着气候条件的变化, 该模式各季资源量绝对值和资源利用效率会发生变化, 不同熟期品种和播收期也会相应的调整, 但其季节间资源分配率和分配比值仍会保持相对固定值。综上所述, 本研究建立的以积温分配为主的资源分配指标可作为黄淮海区当前气候条件和生产条件下冬小麦-夏玉米模式季节间资源配置是否合理的评价标准, 来指导该区传统冬小麦-夏玉米种植模式的资源优化配置。
通过比较冬小麦-夏玉米模式光温水资源生产效率发现, 三省周年资源利用效率差异较大, 其中山东省周年积温生产效率最高, 河南省周年辐射生产效率最高, 河北省周年降水生产效率与河南省无显著差异, 但显著高于山东。这主要是因为区域间同一作物以及同一区域不同作物间资源生产效率存在较大差异, 说明充分挖掘C4作物(玉米)高光温水效率优势是进一步提升黄淮海冬小麦-夏玉米周年资源利用效率的重要途径[18-19,22]。可见, 虽然通过季节间资源优化配置可显著提高冬小麦-夏玉米模式周年产量及资源利用效率, 但作物生长季节内光温水资源的时空分布, 及其与作物生长发育的动态匹配程度影响单季作物产量形成及资源利用效率[11,15,25]。因此进一步研究季节内资源分配与利用的定量关系对于建立更加完善的冬小麦-夏玉米模式周年资源定量优化配置方案具有重要意义, 这也是我们下一步研究的重点。
4 结论
明确了作物高产形成与气候资源分配的定量关系, 建立了以热量资源为主的季节间资源优化配置指标及其定量标准。虽然黄淮海不同区域光温水等资源禀赋差异较大, 导致区域间冬小麦-夏玉米模式产量差异较大, 但周年资源分配合理, 季节间资源分配率和分配比值相对固定, 即小麦季和玉米季积温分配率分别为43%和57%, 两季间积温比值为0.7, 可使各地区产量均达到当前生产和生态条件下的最高水平。这些定量指标可作为黄淮海不同生态区冬小麦-夏玉米模式季节间合理资源配置的评价标准, 指导该区冬小麦-夏玉米种植模式的资源优化配置, 为进一步挖掘黄淮海周年产量潜力和资源效率提供理论支撑。参考文献 原文顺序
文献年度倒序
文中引用次数倒序
被引期刊影响因子
[本文引用: 1]
[本文引用: 1]
[本文引用: 1]
[本文引用: 1]
[本文引用: 1]
[本文引用: 1]
[本文引用: 1]
[本文引用: 1]
[本文引用: 1]
[本文引用: 1]
[本文引用: 1]
[本文引用: 1]
[本文引用: 1]
[本文引用: 1]
DOI:10.1016/j.fcr.2013.04.004URL [本文引用: 1]

Options to increase resource use efficiency and climatic yield potential of locally adapted super rice hybrids including combined water, nutrient and other agronomic management are limited. Hence, the aim of our three-year (six seasons) experiments during early-season (ESR; Luliangyou996) and late-season (LSR; C-liangyou396) rice in southern China was to identify key yield parameters and optimum resource use options to enhance the crop's climatic yield potential. Grain yield averaged across all three years with effective N management combined with post-anthesis shallow wetting and drying was 32.8% and 37.1% higher than the normal farmers’ practice in Liuyang County in ESR and LSR, respectively. More spikeletsm612 were the key to achieving high yield potential, further supported by increased leaf area index and high radiation interception and internal use efficiency. The split application of nitrogen in combination with shallow wetting and drying allowed for better N uptake, use efficiency and partitioning, leading to enhanced biomass and yield. The high yield potential, however, was not just a function of genetics and management but also depended on the climatic conditions prevailing, particularly temperature and radiation. In ESR, lower temperature during vegetative stage reduced overall biomass and sink size while subsequent higher temperature reduced the total grain filling period by 17 days compared with LSR, indicating a climatic condition-driven decline in yield potential rather than lower genetic potential of the super hybrids. A lack of correlation of spikeletspanicle611 and spikeletsm612 with grain-filling percentage in LSR provided evidence that a larger sink does not necessarily result in poor grain filling when sufficient time and assimilates for grain filling are provided, which is more climate dependent. Our work highlights the benefits of integrating nutrient, water and agronomic management options to achieve high NUE and grain yield.
DOI:10.1016/j.fcr.2012.04.008URL [本文引用: 3]

Excessive nitrogen (N) fertilizer application, outdated fertilization techniques, and non-optimal planting patterns are current cultivation problems with summer maize (Zea mays L.) among smallholders in North China. To try to solve those problems, this study examined four integrated agronomic management treatments-MT (FP: traditional farming practices; OPT-1: an optimized combination of cropping systems and fertilizer treatment; HY: treatment based on high-yield studies; and OPT-2: further optimized combination of cropping systems and fertilizer treatment) and four N application rate treatments-NT (0, 129.0, 184.5, and 300.0kgha1). Maize grain yield and N efficiency were determined under each treatment. Grain yield, yield components, individual/population dry matter weight, N partial factor productivity (PFPN), N use efficiency (NUE), and agronomic efficiency of N (AEN) were measured. Results from the NT revealed no significant increase in grain yield in response to N applied above 184.5kgha1 and increased yield was achieved by the MT. In MT, the change in sowing method from relay intercropping to direct seeding was effective in avoiding maize rough dwarf virus and in encouraging mechanized production; individual and population dry matter accumulation from the tasseling stage (VT) to physiological maturity stage (R6) increased in response to delayed sowing date and harvesting time; grain yield increased significantly from more ears per hectare due to increased planting density; and high N efficiency was achieved after optimizing fertilization patterns. In this study, OPT-2 obtained 67.0%, 104.0%, and 53.5% higher grain yield, PFPN, and NUE, respectively, compared to FP, achieving high yield and high N efficiency. Furthermore, the low AEN value suggests that further reduction in the N application rate of OPT-2 may be possible.
DOI:10.1016/j.agrformet.2006.03.014URL [本文引用: 1]

A warming trend has become pronounced since the 1980s in China and is projected to accelerate in the future. Concerns about the vulnerability of agricultural production to climate change are increasing. The impact of future climate change on crop production has been widely predicted by using crop models and climate change scenarios, but little evidence of the observed impacts of climate change on crop production has been reported. In this study, we synthesized crop and climate data from representative stations across China during 1981-2000 to investigate whether there were significant trends in changes of climate variables in different regions, and whether theses changes have had significant impact on the development and production of the staple crops (i.e. rice, wheat, and maize). Our results showed that significant warming trends were observed at most of the investigated stations, and the changes in temperature have shifted crop phenology and affected crop yields during the two decades. The observed climate change patterns, as well their impacts on crop phenology and yields are spatially diverse across China. Our study also highlights the need for further investigations of the combined impacts of temperature and CO 2 concentration on physiological processes and mechanisms governing crop growth and production.
DOI:10.1007/s10584-011-0385-1URL [本文引用: 2]

AbstractIn the North China Plain, the grain yield of irrigated wheat-maize cropping system has been steadily increasing in the past decades under a significant warming climate. This paper combined regional and field data with modeling to analyze the changes in the climate in the last 40 years, and to investigate the influence of changes in crop varieties and management options to crop yield. In particular, we examined the impact of a planned adaptation strategy to climate change -“Double-Delay” technology, i.e., delay both the sowing time of wheat and the harvesting time of maize, on both wheat and maize yield. The results show that improved crop varieties and management options not only compensated some negative impact of reduced crop growth period on crop yield due to the increase in temperature, they have contributed significantly to crop yield increase. The increase in temperature before over-wintering stage enabled late sowing of winter wheat and late harvesting of maize, leading to overall 4–6% increase in total grain yield of the wheat-maize system. Increased use of farming machines and minimum tillage technology also shortened the time for field preparation from harvest time of summer maize to sowing time of winter wheat, which facilitated the later harvest of summer maize.
DOI:10.1016/j.agee.2011.10.015URL [本文引用: 2]

Serious water deficits and excessive nitrogen (N) applications are threatening the sustainability of intensive agriculture in the North China Plain (NCP). This study examined the possibility of replacing the conventional system (Con.W/M) of winter wheat ( Triticum aestivum L.) and summer maize ( Zea mays L.), with an optimized double cropping system (Opt.W/M), a 2-year system (winter wheat/summer maize–spring maize, W/M–M), and a monoculture system (spring maize, M) based on optimal water and N management strategies. From 2004 to 2010, a long-term field experiment conducted in the NCP showed that although >70 mm of irrigation water can be saved with Opt.W/M compared with Con.W/M, annual net groundwater use under Opt.W/M was still 250 mm, 65–90% of which was consumed during the winter wheat season. When wheat production was decreased, 35% and 61% of irrigation water could be reduced in W/M–M and M compared to Con.W/M, respectively. As a result, annual groundwater use was decreased to 190 mm in W/M–M and 94 mm in M. Meanwhile, the N fertilizer rate was reduced 59% and 72% in W/M–M and M compared to Con.W/M, respectively. There were no significant differences in net economic returns between Con.W/M and W/M–M across the 6-year period. In the 6 years, no significant economic loss was observed between Con.W/M and M except in the 2008–2010 rotation. The W/M–M and M systems showed great potential to reduce water and N application and achieve groundwater use balance, and thus should be considered for economic and sustainable agricultural development in the NCP.
URL [本文引用: 1]
URL [本文引用: 2]
DOI:10.1016/j.fcr.2013.01.003URL [本文引用: 2]

Environmental conditions greatly affect the growth of maize. To examine differences in phenological responses of maize (Zea mays L.) to climatic factors under different environmental conditions as induced by latitude, experiments were conducted from 2007 to 2010 at 34 sites in seven Chinese provinces located in the north spring maize region of China between latitudes 35°11′ and 48°08′N in the cultivation of hybrid zhengdan958 (ZD958). Latitude is an important geographical factor which significantly affects temperature, sunshine hours, and the duration of crop growth. The findings of this study indicate that for every 1° increase in the latitude, northward, the growth durations of sowing to emergence and emergence to silking were significantly increased by 0.7 d and 1.25 d, respectively as a consequence of lowering temperatures (mean, maximum, and minimum temperatures). Reproductive growth duration (silking to maturity), which was significantly correlated with the precipitation, decreased by 0.8 d with each 1° increase in latitude northward. At higher latitudes, the number of growing degree days (GDD) of maize vegetative growth duration (emergence to silking) was significantly higher, and the GDD of the reproductive growth duration were significantly lower. The average photoperiod during the photoperiod-sensitive phase of maize development across all the experimental sites was 14.9h with a range of 13.7–15.6h. Total leaf numbers increased from 18.7 to 23.7 with an average of 21.0 across all experimental sites. Significant and positive linear relationships were found to occur between both latitude and photoperiods and latitude and total leaf number. In the north China spring maize region, the mean growth duration of ZD958 was 143.73 d, which constituted 82.8% of the frost free period, the percentage increasing with higher latitude. These findings strongly indicate that in order to ensure high and stable production of maize in the north spring maize region of China, with its limited heat resources, especially in the high-latitude regions, there is a need to cultivate short-growth-duration cultivars.
[本文引用: 2]
[本文引用: 2]
[本文引用: 1]
[本文引用: 1]
DOI:10.1016/j.indcrop.2006.12.003URL [本文引用: 2]

Rotation of winter wheat ( Triticum aestivum L.) and summer maize ( Zea mays L.) is the prevailing double-cropping system in the North China Plain. Typically, winter wheat is planted at the beginning of October and harvested during early June. Maize is planted immediately after wheat and harvested around 25th of September. The growing season of maize is limited to about 100-110 days. How to rectify the sowing date of winter wheat and the harvest time of summer maize are two factors to achieve higher grain yield of the two crops. Three-year field experiments were carried out to compare the grain yield, evapotranspiration (ET), water use efficiency (WUE) and economic return under six combinations of the harvest time of summer maize and sowing date of winter wheat from 2002 to 2005. Yield of winter wheat was similar for treatments of sowing before 10th of October. Afterwards, yield of winter wheat was significantly reduced ( P < 0.05) by 0.5% each day delayed in sowing. The kernel weight of maize was significantly increased ( P < 0.05) by about 0.6% each day delayed from harvest before 5th of October. After 10th of October, kernel weight of maize was not significantly increased with the delay in harvest because of the lower temperature. The kernel weight of maize with thermal time was in a quadratic relationship. Total seasonal ET of winter wheat was reduced by 2.5 mm/day delayed in sowing and ET of maize was averagely increased by 2.0 mm/day delayed in harvest. The net income, benefit ost and net profit per millimetre of water used of harvest maize at the beginning of October and sowing winter wheat around 10th of October were greater compared with other treatments. Then the common practice of harvest maize and sowing winter wheat in the region could be delayed by 5 days correspondingly.
[本文引用: 2]
[本文引用: 2]
[本文引用: 1]
[本文引用: 1]
[本文引用: 1]
[本文引用: 1]
[本文引用: 4]
[本文引用: 4]
DOI:10.2134/agronj1983.00021962007500050008xURL [本文引用: 1]

The efficient breeding and selection of corn (Zea mays L.) genotypes for different climatic regions requires a quantitative understanding of the plant's developmental responses to environmental factors such as temperature and photoperiod. This information is also essential if reliable and meaningful crop simulation models are to be developed. Plants of two corn hybrids, XL45 and W346 were grown in controlled environments under 18 day/night temperature combinations ranging from 16/6 to 38/33176;C (12-h photoperiod) and under three photoperiods (12,14, and 16 h) at two selected temperatures (constant 18 and 28176;C). Data defining the temperature response curves, including the minimum and optimum temperature limits, for germination and emergence and for the development periods from sowing to tassel initiation and sowing to anthesis were obtained. A minimum temperature of 9176;C was predicted for germination and emergence, and a requirement of 62.5 degree-days was determined for this growth stage. The optimum temperature was approximately 30176;C. Minimum temperatures of 8 and 7176;C were determined for tassel initiation and anthesis, respectively, and the optimum temperature for both was 28176;C above which the development rates declined. These temperature limits compared with minima and maxima of 10 and 30176;C, respectively, used in most current heat-sum methods. Between the limits of 7 and 28176;C, the number of degree-days required to reach tassel initiation and anthesis were, respectively, 208 and 736 for hybrid W346, and 245 and 816 for XL45. Tassel initiation occurred at approximately one-third of the time between sowing and anthesis when calculated either on the basis of heat-sums (degree-days) or from calendar-days under the steady-state temperature conditions used. An increase in photoperiod lengthened both the time between sowing and tassel initiation and that between tassel initiation and anthesis in a similar, almost equal, manner for both cultivars. Sensitivity to the photoperiod response was not altered by temperature.
DOI:10.1016/S0378-4290(98)00170-1URL

Abstract Total number of initiated leaves and duration from sowing to silking increases when photoperiod is increased during the photoperiod-sensitive phase in maize (Zea mays L.). Little is known, however, about possible other effects of photoperiod and incident photosynthetic photon flux density (PPFD) on rate of development and duration of life cycle. A study was undertaken to quantify effects of photoperiod and incident PPFD from sowing to the 15-leaf stage on rate of leaf appearance and duration of the grain-filling period. The short-season maize hybrid Pioneer 3902 was grown in growth cabinets from sowing to the 15-leaf stage with either (i) a 10h photoperiod at high PPFD (650μmolm612s611), (ii) a 20h photoperiod consisting of 10h of high PPFD followed by 10h of low PPFD (5–50μmolm612s611), or (iii) a 20h photoperiod of high PPFD. From the 15-leaf stage to maturity the plants were placed under a 16h photoperiod in a growth room. Increasing photoperiod from 10 to 20h increased final number of initiated leaves and delayed silking but did not affect rate of leaf appearance. Doubling incident PPFD to a value similar to that under Ontario field conditions during the summer resulted in a 16% increase in rate of leaf appearance and in a significant increase in total number of initiated leaves. Differences in final number of initiated leaves and in rate of leaf appearance from sowing to the 15-leaf stage among treatments resulted in a 4-day difference in silking date between the 10h photoperiod treatment and the two 20h photoperiod treatments. Duration of the grain-filling period did not differ among the three treatments.
DOI:10.2135/cropsci2012.12.0688URL [本文引用: 2]

Environmental conditions have important effects on maize (Zea mays L.) growth. To examine spatial variation in maize yield and aboveground biomass and to understand differences in the response of maize yield and aboveground biomass to climatic factors under various ecological conditions, we conducted experiments from 2007 to 2010 at 34 locations in seven provinces in the spring maize region of northern China between 35 degrees 11' N lat and 48 degrees 08' N lat. We used a most widely cultivated maize hybrid ZD958. The maize yield and aboveground biomass (presilking and postsilking) were found to be strongly influenced by locations. A nonlinear relationship existed between the maize yields and latitude. Maize yield was the greatest (12.19 Mg ha(-1)) at 39 degrees 08' N lat, and the corresponding presilking and postsilking aboveground biomass at this location were 143.41 and 215.35 g per plant, respectively. Variations in the harvest index (HI) and 1000-kernel weight were the main reasons for yield latitudinal trends. Among the climatic factors, air temperature had the best relationships with variations in maize yield, HI, and 1000-kernel weight. With latitudes increasing northward, presilking aboveground biomass affected by growth duration length and accumulated solar radiation increased significantly. The aboveground biomass of postsilking stage that was affected by the maximum temperature, daily mean temperature, and growing degree days decreased significantly with latitudes increasing northward. However, there were no significant changes of total aboveground biomass with latitudes increasing northward.
[本文引用: 2]
[本文引用: 2]
[本文引用: 1]
[本文引用: 1]
DOI:10.2134/agronj2015.0196URL [本文引用: 1]

Variations in weather conditions could alter maize (Zea mays L.) growth and development. This study was conducted to determine the eco-physiological determinants of variations in maize yield with weather conditions, and the relationship between grain yield, dry matter production, and climatic factors. Eight sowing dates were set at 15- to 20-d intervals from mid-March to mid-July during 2012 and 2013 in the Huang-Huai-Hai region of China. When the sowing date was delayed, the yield increased initially and later declined, and the greatest yield was obtained at 12 June (SD6) sowing date for both years. The increased yield for SD6 was mainly attributed to the 1000-kernel weight and post-silking dry matter production, which were mainly influenced by the post-silking plant growth rate. Variations in temperature and radiation were the primary factors that infl uenced the post-silking dry matter production of maize, and eventually influenced grain yield. High temperatures (daily maximum temperature [Tmax] > 28.1C) during postsilking under early sowing conditions and low temperatures (daily minimum temperature [Tmin] < 17.7C) under late sowing conditions combined with low radiation (accumulated radiation [Ra] < 1 005.4 MJ m2) decreased the post-silking plant growth rate, thereby decreasing the dry matter production and grain yield. Therefore, when the sowing was done from 25 May to 27 June, the relatively higher maize yield would be obtained. We conclude that variations in weather conditions (temperature and radiation) from silking to maturity significantly affect the plant growth rate of maize, influence post-silking dry matter production, and grain yield.
[本文引用: 1]
[本文引用: 1]
[本文引用: 1]
[本文引用: 1]
[本文引用: 1]
[本文引用: 1]
DOI:10.2135/cropsci1979.0011183X001900010001xURL [本文引用: 1]

Flowering date of maize (L.) was advanced, by increasing temperature or decreasing photoperiod, in order to study the effect of a decrease in length of vegetative phase on leaf area at flowering, number of female florets, and length of grain-filling period. Plants of two single-crnss hybrids were grown at temperatures of 20 or 25 C, combined with 10- or 15-hour photoperiods, until ears started developing, and then transferred to a single regime of a 14 hour photoperiod and 23/19 C light/dark temperature.
DOI:10.3354/cr00823URL [本文引用: 1]

Change in thermal conditions can substantially affect crop growth, cropping systems, agricultural production and land use. In the present study, we used annual accumulated temperatures > 10 degrees C (AAT10) as an indicator to investigate the spatio-temporal changes in thermal conditions across China from the late 1980s to 2000, with a spatial resolution of 1 x 1 km. We also investigated the effects of the spatio-temporal changes on cultivated land use and cropping systems. We found that AAT10 has increased on a national scale since the late 1980s, Particularly, 3.16 x 10(5) km(2) of land moved from the spring wheat zone (AAT10: 1600 to 3400 degrees C) to the winter wheat zone (AAT10: 3400 to 4500 degrees C). Changes in thermal conditions had large influences on cultivated land area and cropping systems. The areas of cultivated land have increased in regions with increasing AAT10, and the cropping rotation index has increased since the late 1980s. Single cropping was replaced by 3 crops in 2 years in many regions, and areas of winter wheat cultivation were shifted northward in some areas, such as in the eastern Inner Mongolia Autonomous Region and in western Liaoning and Jilin Provinces.