1.
Introduction
Recently, GaN based light-emitting diodes have received a great deal of attention due to their high?efficiency and energy?saving properties,?environmental protection and long?service?life. LEDs have been commercialized for indoor and outdoor lighting and displays, accordingly, the performance of LEDs has also been greatly improved. As we all know, LEDs have wide applications, such as full-color displays[1, 2], photochemistry?and?photobiology[3].
However, efficiency droop[4] which is defined as a reduction in luminous efficiency with increasing current density is observed; it has now become a troublesome issue that has aroused a massive amount of interest in recent years. Many theories have been attempted to explain this phenomenon such as electron leakage[5–7], lack of hole injection[8, 9], carrier delocalization[10], and Auger recombination[11, 12]. In a large number of existing research results[13–16], nonuniform carrier distributions and the insufficient injection efficiency of carriers may lead to the efficiency droop and low external quantum efficiency (EQE), so it is essential to solve this kind of issue. Some groups have tried to address this kind of issue, including reducing the polarization effect inside the active regions of blue InGaN LEDs by using polarization matched AlGaInN EBL, which can in turn improve the confinement of electrons in the active region[17]. Liu et al. analyzed the efficiency droop in InGaN/GaN multiple-quantum-well blue light-emitting diodes grown on free-standing GaN substrate[18]. It has reported that the LEDs with GaN-AlGaN composition-decreased barriers show more significant enhancement of internal quantum efficiency and light-output power than LEDs with GaN barriers. In this letter, we compared LEDs which have double superlattices with conventional ones. The APSYS simulation program developed by the Crosslight Software Inc. was used to analyze the electrical and optical properties of the LED devices.
2.
Model and parameters
We employed the APSYS simulation program to research the above issues. This simulation program uses a?two dimensional?finite element?method to solve the Poisson equation, quantum mechanical wave equation, carrier transport equation, current continuity equations and some theoretical formula which describe carrier characteristics and boundary conditions. In this simulation, we defined the blue InGaN LEDs as structure A, which is composed of a 2.5 μm thick undoped GaN layer, and a 2.5 μm thick Si-doped n-GaN (n-doping = 5 × 1018 cm?3) layer. The InGaN/GaN MQWs consisted of six pairs of 10 nm GaN QB and 3 nm In0.16Ga0.84N QW. On the top of the last QB are a 20 nm thick p-Al0.1Ga0.9N EBL and a 170 nm thick main p-GaN layer (p-doping = 1.2 × 1017 cm?3). For comparison, we inserted In0.03GaN/GaN superlatticed between n-GaN and MQWs. Both thicknesses of the InGaN and GaN are 2 nm. Besides, the EBLs are replaced by Al0.3In0.03GaN/Al0.3GaN superlattice. The LEDs with this kind of double superlattice, which has 10 pairs of QB and QW, are called structure B. The others are identical with to structure A. In consideration of the shielding and compensation of free charge, stimulated carrier, material defect and impurity, the screening coefficient which reports the fraction of the theoretical interface charge is set to 0.6. The device geometry was designed with a rectangular shape of 300 × 300 μm2 and the operating temperature is assumed to be 300 K. To simplify the simulation, the light extraction efficiency is assumed to be 0.78[19]. In this study, we used material parameters of the semiconductors in Ref. [8] for simulation.
3.
Results and discussion
As we can see, Fig. 1 shows the energy band diagrams for sample A and sample B at an injection current of 150 mA. Because of the existence of the polarisation field, the valence band and the conduction band at the interface between the last quantum barrier (QB) and EBL would be bent down. This adverse effect leads to obstacle for holes to inject into the active region and makes electrons liable to spill out at the same time. Besides, due to the serious bending of quantum wells in the band, the wave function of the hole and electron would be separated in space. This phenomenon also aggravates the droop behavior and reduces the compound efficiency of electrons and holes. As a result, the LED's light emission would become lower. This phenomenon can be alleviated when the double superlattices were inserted. Here, structure B shows better optical and electrical performances. As shown in Figs. 1(a) and 1(b), we can see that the effective potential height for an electron in the conduction band (ΔФe) of structure A and structure B are 630 and 660 meV, respectively, which suggests that the EBL that is composed of an Al0.3In0.03GaN/Al0.3GaN superlattice can increase the effective potential heights. Hence, the leakage of electrons can be markedly suppressed. Consequently, the electron concentration is significantly increased. Moreover, the effective electron potential height for the holes between the last barrier in the valence band (ΔФh) of structure A (525 meV) is much higher than that of structure B (390 meV). This indicates that it is more difficult for the holes to be injected into the quantum well areas in the conventional LED (structure A) as a result of the blocking of the effective potential barrier. In consequence, more efficient electron blocking and the improved hole-injection efficiency for structure B will give more holes and electrons participating in radiative recombination in the active region. In addition, the Al0.3In0.03GaN/Al0.3GaN superlattice EBL can make the carriers, especially for holes, tunnel the superlattice barriers to the active region easily due to lower energy barriers, shown in Fig. 1(b). This is also useful to improve the holes injection efficiency. At the same time, In0.03GaN/GaN superlattice between n-GaN and MQWs can help to improve the surface roughness and reduce the density of the V-defects, which is normally found in InGaN/GaN MQWs[20–22]. What is more, about the 230 meV energy barrier as shown in Fig. 1(b), induced by polarization effect, accompanied by the series of interfaces in In0.03GaN/GaN superlattice structure would diffract the injection elections and mitigate the overshoot effect to some extent[23, 24], which leads to electron leakage. As a result, a higher electron concentration in the MQWs could be expected. This is consistent with the calculation results shown in Fig. 2(b). In addition, the lateral expansion of the current in LED structure will?be enhanced.

class="figure_img" id="Figure2"/>
Download
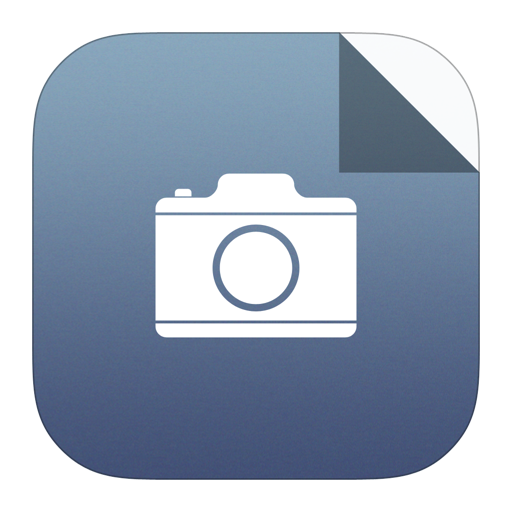
Larger image
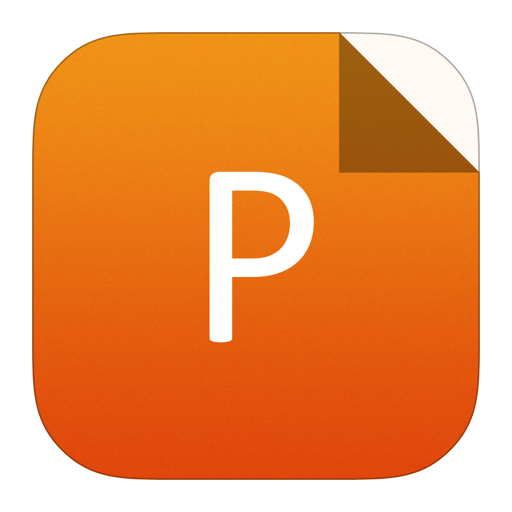
PowerPoint slide
Figure2.
(Color?online) (a) Hole and (b) Electron concentrations for the (a) AlGaN EBL structures and (b) the double superlattices EBL at 150 mA.
The electron and hole concentration distribution in quantum well areas of two structures at the current of 150 mA are illustrated in Fig. 2. It can be seen obviously that with double superlattices, more injected holes are confined in the active region compared to the conventional one. This can contribute to the lower ΔФh, as shown in Fig. 1. Furthermore, the Al0.3In0.03GaN/Al0.3GaN superlattice EBL can also help the holes tunnel to the MQWs, the hole concentration in MQW for structure B is dramatically increased. Meanwhile, it can be seen clearly in Fig. 1 that ΔФe is much lower for structure A. Hence, the higher ΔФe of structure B compared with that of structure A can strongly suppress the electrons from spilling over the quantum well areas. In Fig. 2, the electron leakage of structure A significantly decreases. Thus, such an improvement in the holes concentrations and the efficient confinement of electrons is conductive to efficiency droop alleviation. In addition, double superlattices can improve the distribution of electrons and holes more uniformly in MQWs and the emission spectrum of LED will be more stable[25].
Fig. 3(a) shows the LED output power comparison between structure A and structure B when the current injection density is increased. Obviously, the light output power of two structures increases with the rising current injection density. It is?remarkable?that the output power of structure B ascends much more rapidly with a rising injection current than that of structure A, which states that it is useful to optimize the output power of the LED, especially at a high injection current, by using the double superlattices.
Fig. 3(b) shows a contrast of internal quantum efficiency (IQE) on the forward current for the two structures. By?comparison with structure B, structure A shows a severe efficiency droop in the IQE. In this study, we defined the efficiency droop ratio as
m{peak}}}} - {eta _{600 ,,,,{
m{mA}}}})/{eta _{{
m{peak}}}}$


class="figure_img" id="Figure3"/>
Download
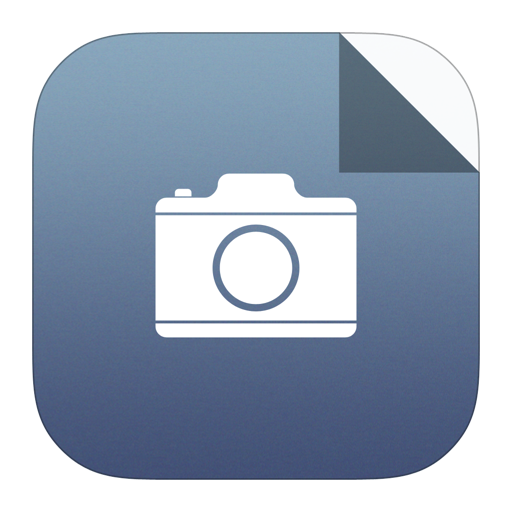
Larger image
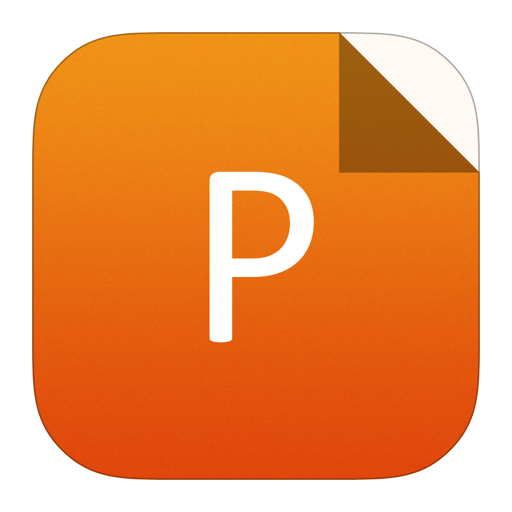
PowerPoint slide
Figure3.
(Color?online) (a) Output power and (b) IQE curves as a function of injection current for the double superlattices EBL and AlGaN EBL structures, respectively.

class="figure_img" id="Figure1"/>
Download
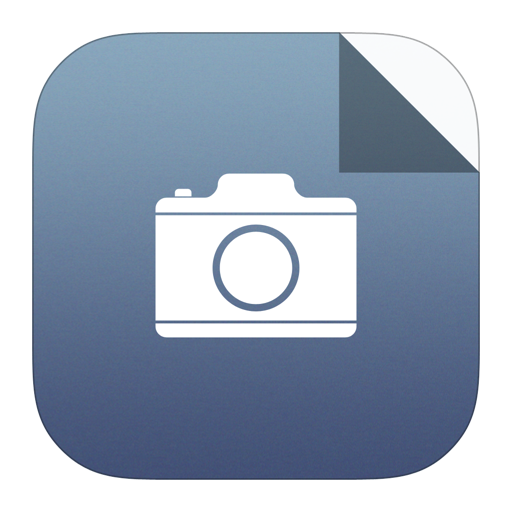
Larger image
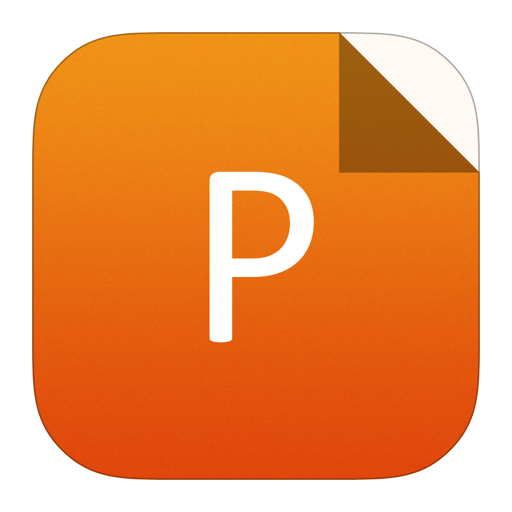
PowerPoint slide
Figure1.
(Color?online) Energy band diagrams of (a) AlGaN EBL structures and (b) the double superlattices EBL at 150 mA.
4.
Conclusions
In summary, the performance of the blue GaN-based LED with double superlattices is investigated numerically. The comparison between structure A and structure B shows that LEDs with double superlattices can enhance the output power and improve the efficiency droop behaviors. We reckon that the tremendous improvement in the efficiency droop can be put down to the more efficient electron blocking and the improved hole-injection efficiency.