全文HTML
--> --> -->国内外若干研究单位相继开展了关于单层MoS2性能受应变影响的研究工作. 例如, Liu等[10]研究了单层MoS2原子层中的应变和结构异质性, 并证明了单晶单层MoS2中的不均匀应变和应变诱导的带隙工程. Pak等[11]研究了单层MoS2接触能垒的应变工程和光响应行为. Conley等[12]研究了机械剥落的单层和双层MoS2应变的带隙变化. Dadgar等[13]研究了单层过渡金属二硫属元素化物的应变工程和拉曼光谱. 李明林等[14]研究了单层MoS2性能随温度的变化趋势以及手性效应的影响. Kuc等[15]研究了MoS2层间相互作用对其能带结构的影响. 吴木生等[16]研究了2.5%拉伸范围内应变对单层MoS2能带影响. Wu等[17]研究了晶粒尺寸对单层多晶MoS2拉伸强度的影响. 由于单层MoS2样品仅0.65 nm厚, 在制备和测试过程中存在诸多困难和挑战[18-20], 因此以第一性原理计算为代表的纳米尺度计算模拟方法成为分析单层MoS2材料结构和力学性能的重要手段[21,22].
本文基于第一性原理计算得出了本征单层MoS2的电子能带结构和吸收系数. 分析了拉应变和压应变的影响; 并结合实验和表征研究了拉伸对单层MoS2光学特性的影响.
2.1.计算方法
本文计算采用基于密度泛函理论的第一性原理方法[23], 使用VASP (Vienna ab-initio simulation package)程序包完成[24], 选择广义梯度近似平面波赝势方法, 结合Perdew-Burke-Ernzerhof交换关联势, 原子实和价电子之间的相互作用通过映射缀加波势来描述. VASP软件采用超原胞模型, 在实际计算中, 将超原胞划分为足够细的网格点, 在每个网格点上给定一组初始波函数, 并计算出电荷密度ρ(r)和交换关联势, 然后根据本征方程求出一组波函数和本征能量. 根据求得的波函数, 再次求出下一组波函数和本征能量, 一直迭代直到两次求出的本征能量之差小于某一预设值(自洽), 则得到体系的总能量. 计算过程中, 把Mo原子的4d电子作为价电子处理, 计算时平面波截断能量为550 eV, 采取9 × 9 × 1的倒空间k点网格, 这些参数使得总能收敛. 使用共轭梯度法优化MoS2结构, 直到每个原子间的受力收敛精度不超过0.01 eV/? (1 ? = 0.1 nm). 为模拟单层MoS2, 把单层MoS2放置在超原胞结构中, 为消除层间相互作用引起的干扰, 真空层大小取为2 nm.单层MoS2是由一层Mo原子层和上下两层S原子堆积而成, 单层MoS2的结构图如图1所示. 蓝色虚线平行四边形表示MoS2的原胞, 每个原胞中含有1个Mo原子和2个S原子, 无应变时2个硫原子层的垂直距离为d0.
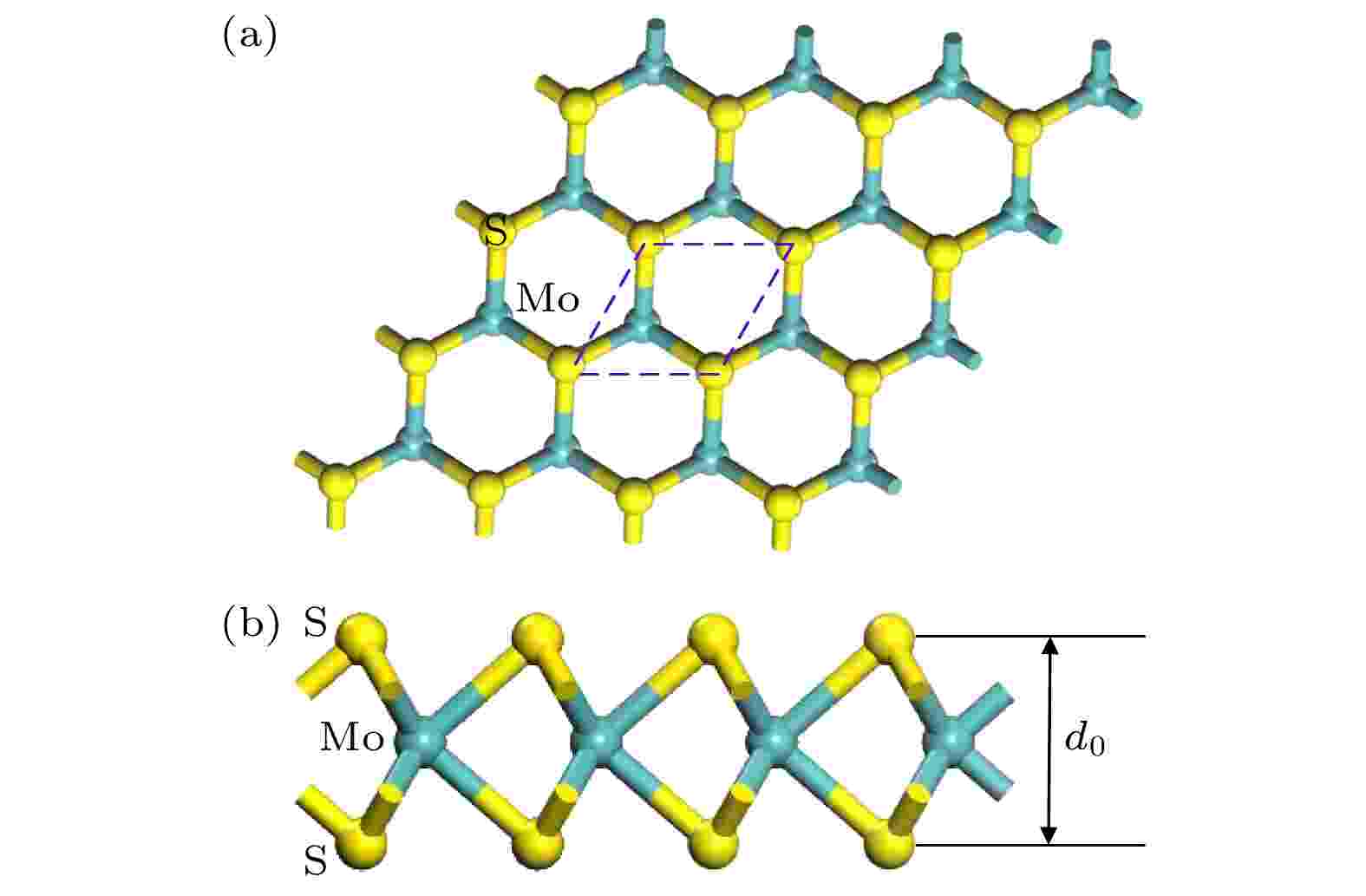
Figure1. Structure diagram of monolayer MoS2: (a) Top view; (b) side view.
本文研究了在0%—10%范围的平拉伸应变对单层MoS2的物理性质的影响, 由于在水平方向的应变会引起平面内晶格常数的变化, 在每个应变之下, 都优化了平面内的晶格常数, 采用的方法是计算一系列的平面内晶格常数, 得到该应变之下总能与平面内晶格常数的关系, 总能最小值所对应的平面内晶格常数, 即为该水平应变之下平面内的晶格常数. 体系在平面内晶格常数a0在3.183 ?时的总能最低. 此时, 单层MoS2中的S—Mo键长为2.413 ?, 两个硫原子层的垂直间距h0为3.127 ?, 和Ramakrishna等[25]的计算结果比较接近.
2
2.2.实验流程
实验所用的单层MoS2连续薄膜通过化学气相沉积法生长在具有300 nm厚的SiO2的Si衬底上. 将单层MoS2转移到柔性聚二甲基硅氧烷(PDMS)衬底上并测试光学性能. 柔性PDMS制备流程如下: 1)制作样品模板, 倒入质量比为10∶1的PDMS原液∶固化剂, 放置在100 ℃加热台上加热0.5 h; 2)加脱模剂, 继续热熏1 h; 3)通过超声波、真空脱泡机进行脱泡处理; 4)放置在80 ℃干燥箱中固化6 h, 冷却完毕, 取样.采用湿法转移的方法将MoS2膜转移到柔性PDMS衬底上, 利用聚甲基丙烯酸甲酯(PMMA)作为支撑层. 单层MoS2的转移流程如图2所示. 1)在MoS2膜上旋涂一层40 mg/mL的PMMA, 然后放置在加热台(100 °C)上加热30 min固化PMMA; 2)用2 mol/L的NaOH溶液腐蚀SiO2层直到薄膜分离为止. 将漂浮的PMMA/MoS2膜转移到DI水中清洗残留的NaOH溶液; 3)取合适大小的PDMS衬底, 用丙酮、乙醇进行清洗; 4)通过等离子体清洗机进行PDMS衬底表面亲水性处理, 用PDMS衬底把漂浮的MoS2薄膜捞出, 放入手套箱倾斜放置自然晾干4 h以上, 再放入100 °C的干燥箱中1 h蒸发残留水分, 改善MoS2和衬底的结合; 5)分多次放入丙酮溶液浸泡2 h以除去PMMA, 然后用乙醇、去离子水清洗, 最后用氮气吹干.
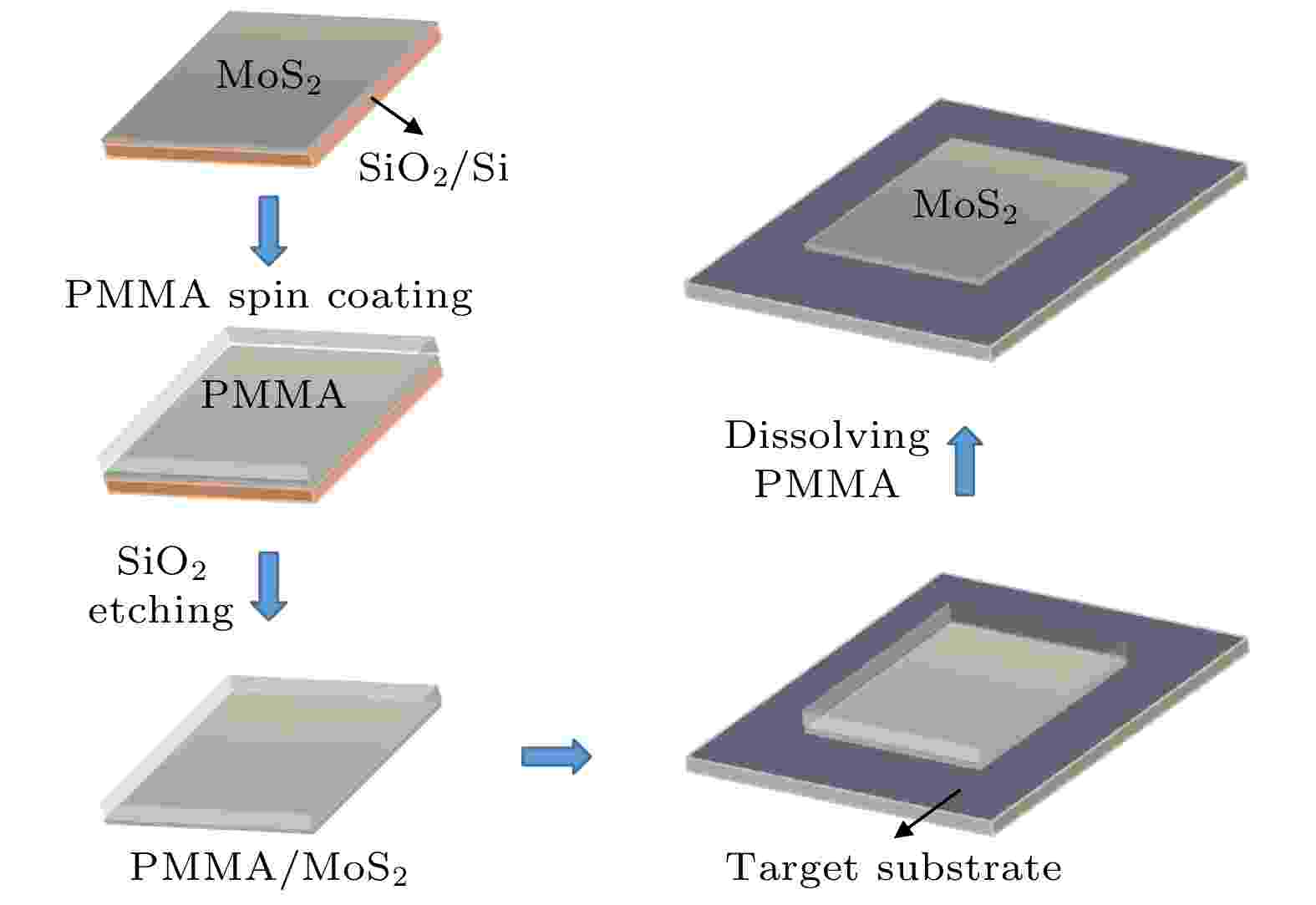
Figure2. Flow chart of wet transfer of monolayer MoS2.
实验采用的单层MoS2膜由深圳六碳科技公司通过化学气相沉积法进行生长. 转移过后的薄膜通过Renishaw公司生产的、型号InVia的拉曼光谱仪进行拉曼光谱(Raman spectra)和光致发光光谱(photoluminescence spectroscopy, PL)的测量, 使用532 nm DPSS激光进行激发.
3.1.计算结果及分析
图3(a)是计算得到的本征单层MoS2的能带结构(以费米能级为0 eV), 可以看出, 单层MoS2为直接带隙, 价带最高点和导带最低点均为K点, 禁带宽度为1.68 eV, 略低于实验值1.73 eV[26]. 因为在使用密度泛函方法求解方程的过程中, 没有考虑激发态, 这是采用密度泛函理论计算能带的常见现象, 并不会影响对能带和电子结构的分析.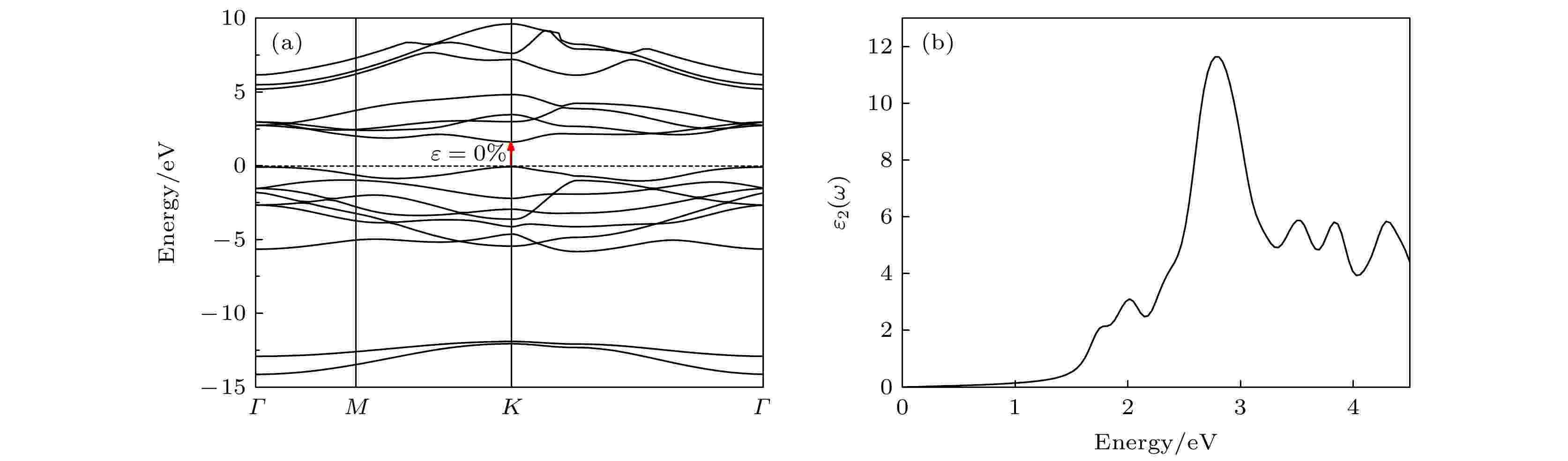
Figure3. (a) Energy band structure of intrinsic MoS2; (b) imaginary part of the intrinsic monolayer MoS2 dielectric function.
在能带计算的基础上, 对单层MoS2的光学性质进行了计算. 介电函数ε(ω)能够反映出固体的光谱信息, ε(ω)是1个虚数, 分为实部和虚部两部分[27]:







对虚部ε2(ω)进行Kramer-Kronig变换能得到介电函数的实部ε1(ω) [27]:


计算得到的本征单层MoS2的介电函数的虚部ε2(ω)如图3(b)所示. 最大峰值出现在2.7 eV处, 是由价带顶到导带底的跃迁所决定的, 代表单层MoS2在入射光频率为2.7 eV左右时达到最大吸收峰值. 对介电函数的实部ε1(ω)与虚部ε2(ω)进行计算可以得到固体的吸收系数α(ω):
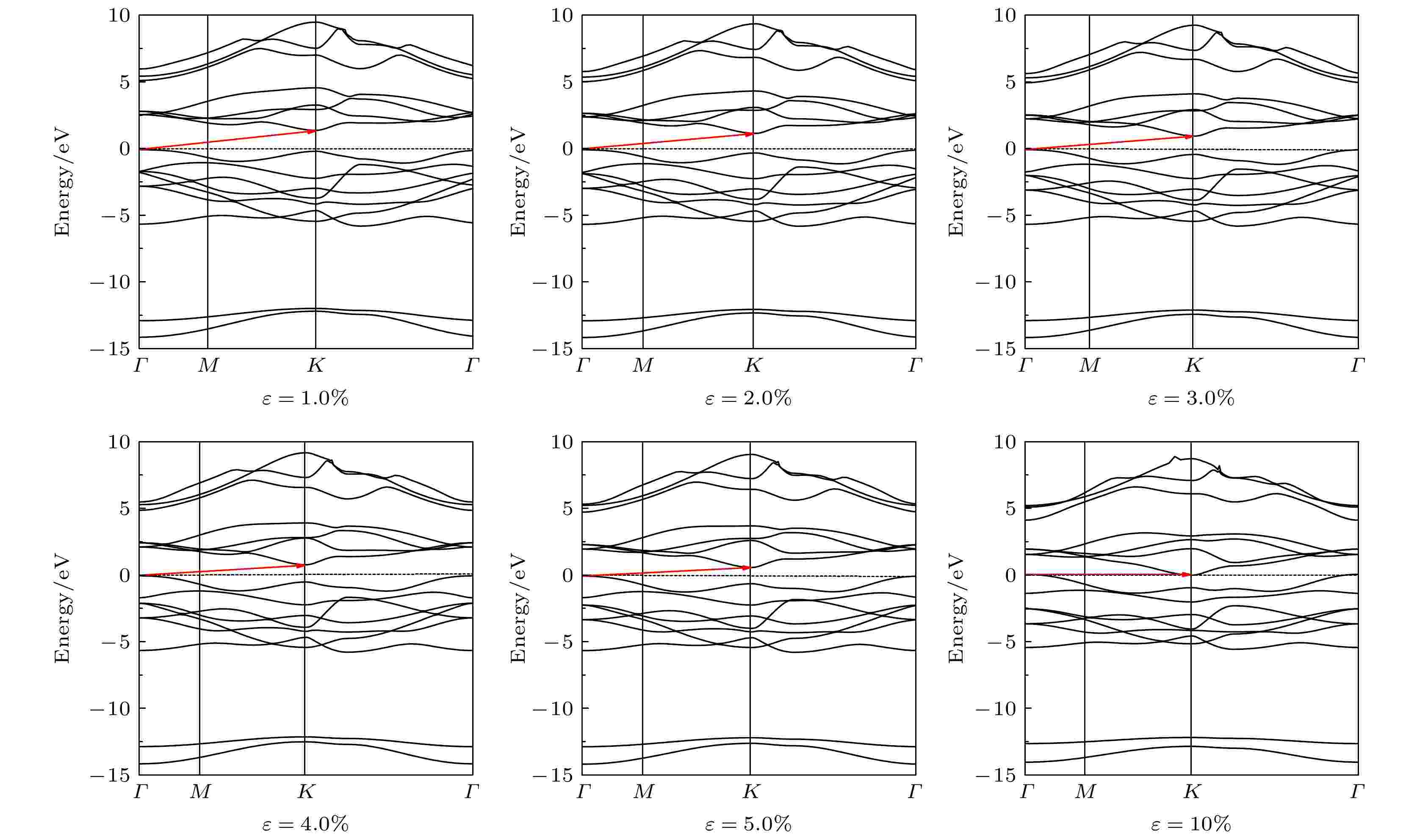
Figure4. Band structure diagram of monolayer molybdenum disulfide under different tensile strains (1.0%?10%).
图5(a)给出了价带最高点K和次高点Γ点随应力变化的能量值. 当拉伸不断施加时, 价带次高点Γ点的能量值仅从–0.085 eV缓慢上升到–0.081 eV; 而K点的绝对能量由–0.066 eV下降到–0.645 eV. 可以得知转变为间接带隙的原因: 价带位置处的K点和Γ点的能量变化对拉伸的敏感程度不同. 其根本原因在于拉伸应变改变了原子位置的弛豫, 间接影响原子间的成键性质和成键强度, 能带结构随之发生改变[28]. 单层MoS2的禁带宽度随拉应变的变化关系如图5(b)所示, 数据显示禁带宽度随拉应变的增大而线性减小.
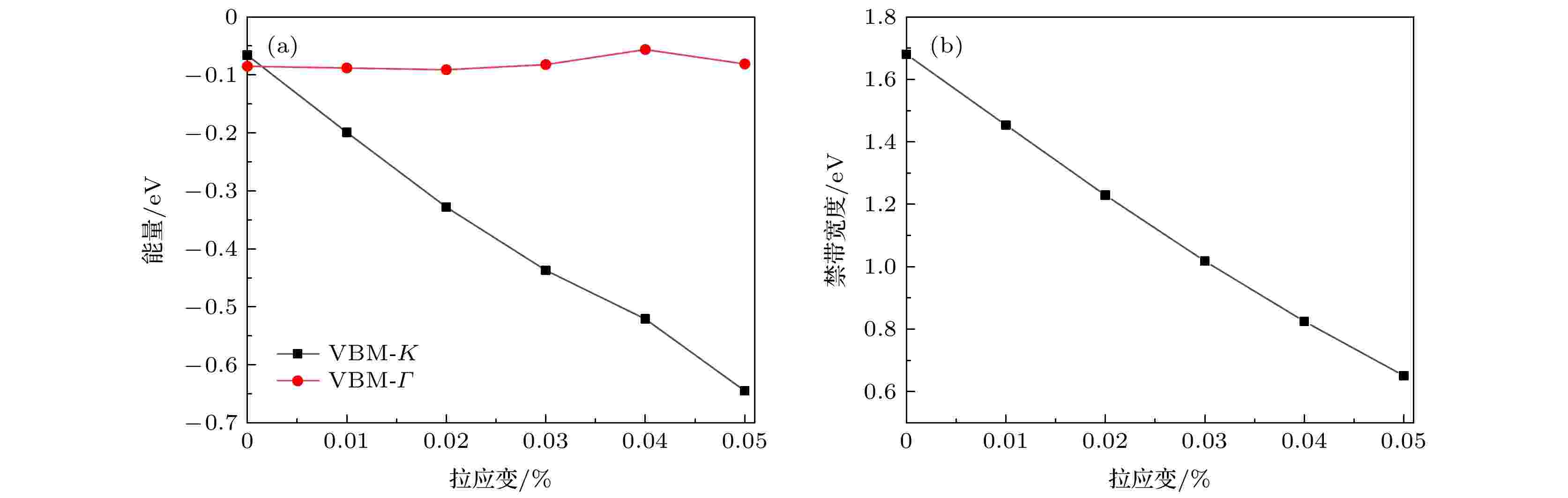
Figure5. (a) Variation of energy of point Γ and point K on the maximum price band (VBM) with the increase of stress; (b) variation curve of band gap width of monolayer MoS2 with tensile strain.
图6为单层MoS2在本征状态和拉应变5%下的总态密度(DOS)和部分态密度(PDOS)图, 其中黑色为DOS图, 彩色为PDOS图. 价带的最高点主要有两种原子的轨道贡献, 分别为钼原子的d轨道和硫原子的p轨道. 在能级–6 eV到–1 eV的范围内, Mo-d轨道和S-p轨道态密度形状相似, 表明两者间共用电子程度高, 对应于Mo-d轨道和S-p轨道间形成的类似于σ键的相互作用. 在–1 eV到Fermi能级范围内, Mo-d轨道和S-p轨道间形成强度较弱的类π键的相互作用. 这种作用对应变极为敏感, 导致施加应变后能带结构发生显著变化[16,29].
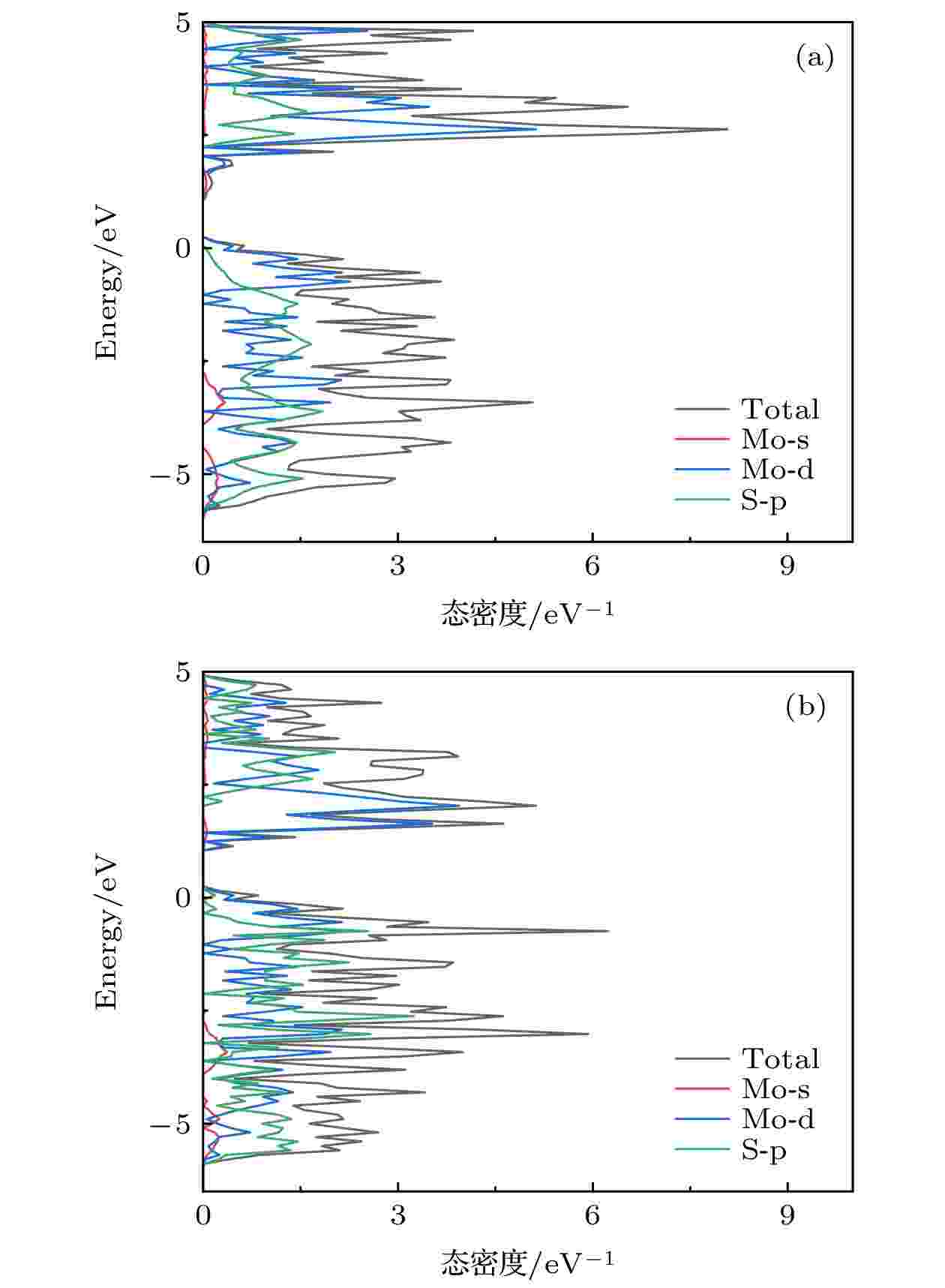
Figure6. Electron density of states of monolayer MoS2 for (a) zero strain and (b) 5% tensile strain.
图7给出了计算得到的单层MoS2在不同拉应变下的吸收系数, 由本征吸收系数曲线(0.0%)可见, 长波吸收边对应的光子能量约为1.5 eV, 对应于导带和价带之间的电子直接跃迁能量, 其中最强峰位于10.92 eV附近, 最大吸收系数为1.66 × 105 cm–1, 吸收系数在8.478 eV处有1个低谷. α(ω)的第1个峰值在2.8 eV附近, 处于可见光中紫光的能量附近, 这解释了MoS2晶体呈现深紫色的原因. 单层MoS2拉应变的吸收系数的结果都与本征MoS2吸收系数图像在趋势上非常接近, 拉应变吸收系数曲线随应变增大而整体红移.
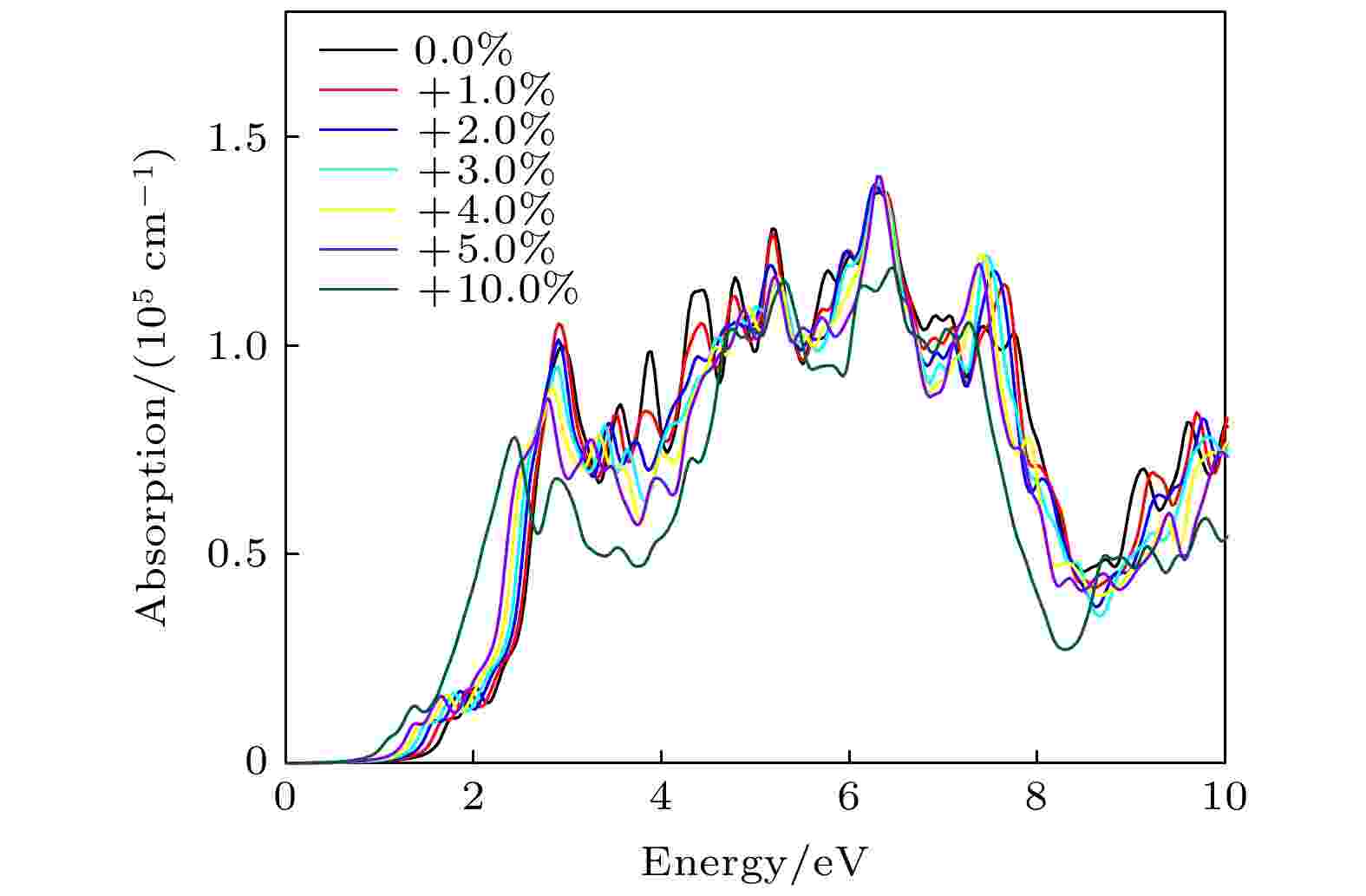
Figure7. Monolayer MoS2 absorption coefficients under different tensile and compressive strains.
2
3.2.实验结果及分析
图8(a)和图8(b)分别为通过湿法转移到Si衬底的单层MoS2的光学显微镜(optical microscope, OM)图像和PL光谱图, 图8(c)和图8(d)分别为转移到PDMS衬底上的单层MoS2的OM图像和实物图. 在PL光谱的1.83 eV处观察到单层 MoS2的A激子的强发射峰, 这表明转移后的单层MoS2膜仍然是高质量的. 单层MoS2的A激子的PL峰可归因于1.84 eV的负三极峰和1.88 eV的中性激子峰的共同作用, PL光谱中负三极峰的存在能表明1L MoS2具有电n型特征[30].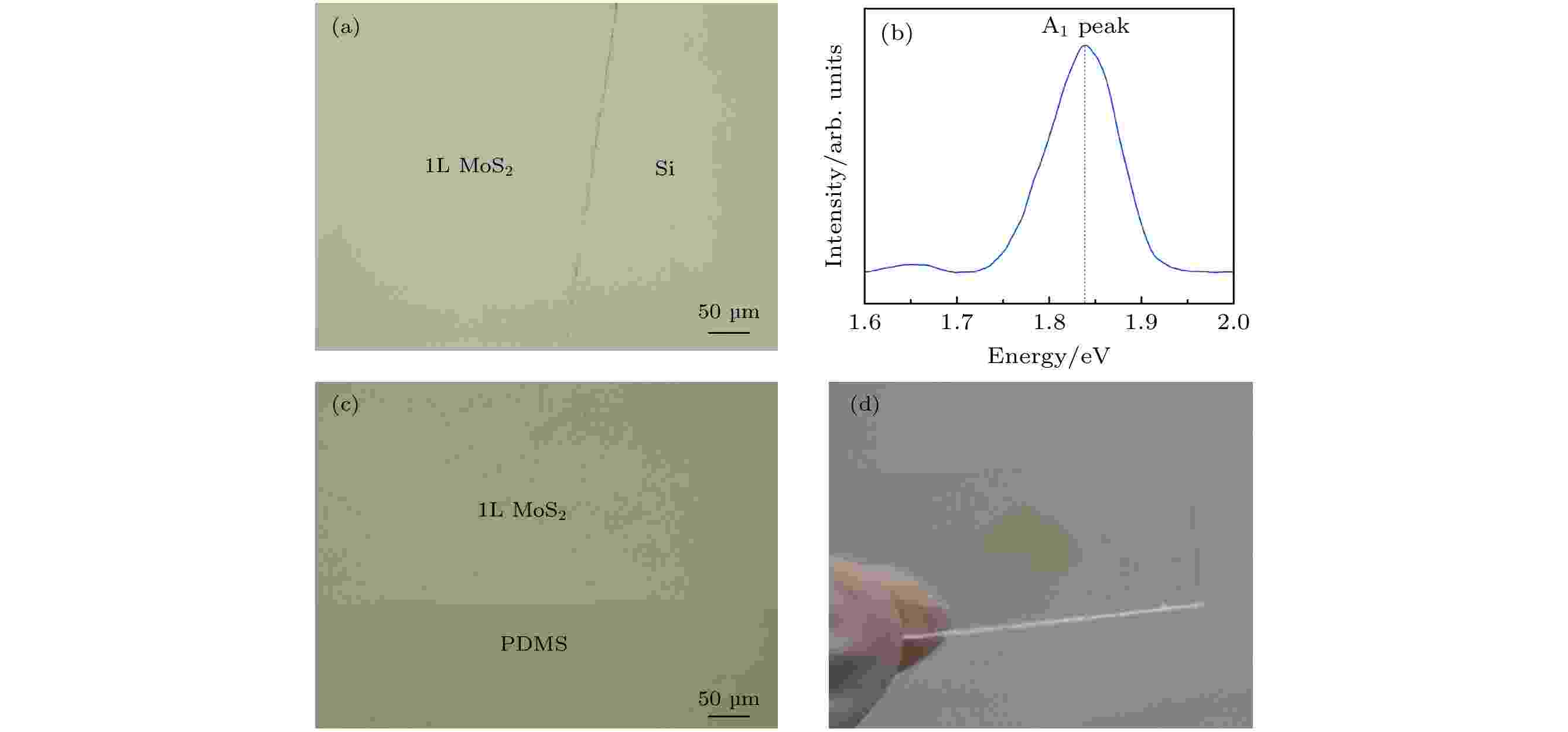
Figure8. (a) OM images transferred to Si substrates, (b) PL spectra transferred to Si substrates, (c) OM images transferred to PDMS substrates, (d) real figures transferred to PDMS substrates when monolayer MoS2 continuous film is transferred by wet method.
对转移到柔性PDMS上的单层MoS2通过拉曼光谱仪(使用532 nm DPSS激光进行激发)下进行不同拉伸应变下的测试, 所施加的应变近似为ε = τ/R, 其中2τ是这项工作中使用的PDMS衬底的厚度. 图9给出了单层MoS2/PDMS的拉曼光谱、PL光谱及相应峰位在不同拉伸应变下的变化趋势图. 随着拉应变的增大, 拉曼光谱图中面内模式

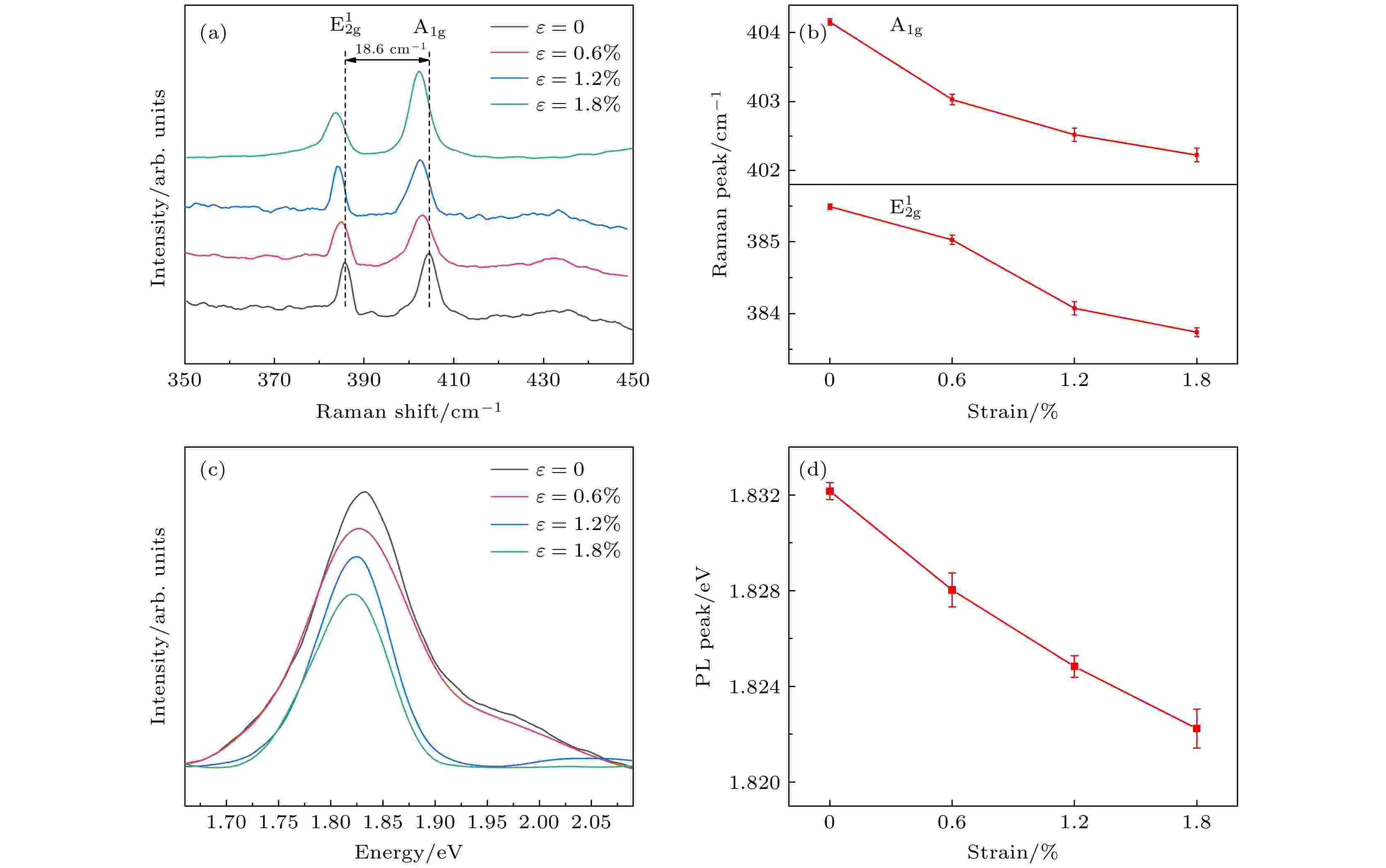
Figure9. (a) Raman spectroscopy, (b) change of Raman peak position, (c) PL spectroscopy, (d) change of PL peak position when monolayer MoS2/PDMS is stretched by 0%?1.8%.
