全文HTML
--> --> -->为了抑制LPI的增长, 包括美国里弗莫尔国家实验室(LLNL)在内的全世界多家实验室进行了数十年的研究, 开展了大量的理论和实验研究工作[7-14]. 尽管如此, 国家点火装置(NIF)的实验中产生的背向散射仍旧远超预估数值. 表明目前对LPI的认识仍是远远不够的, 仍需要进一步开展细致的研究. 多年来的研究主要集中在纳秒激光驱动的条件, 然而SBS, SRS等LPI过程的发生、发展的时间尺度均为皮秒量级, 在纳秒较长的尺度下, 研究的是综合效果. 对于高度非线性的LPI过程, 综合效果可能难以反映真实的物理规律.
近些年, 随着啁啾脉冲放大(CPA)等技术的发展, 皮秒或飞秒超短脉冲激光装置建立起来[15,16], 这为更细致地研究LPI过程提供了可能. 在皮秒时间尺度内, 相关LPI过程经历了发生、发展、饱和等过程, 皮秒激光的时间与之相匹配, 可望获得更微观和细致的结果, 对于进一步理解相关LPI的发展过程具有更好的条件[17]. Rousseaux等[18,19]多年来一直持续地开展皮秒激光驱动LPI的研究, 他们首先利用数百皮秒的长脉冲激光辐照百纳米的CH薄膜靶产生大尺度等离子体, 其后, 1—2 ps左右的激光注入, 诱发LPI, 在背向、前向等测量SBS, SRS等散射信号. 实验中发现, SBS和SRS的发生阈值明显升高, 并且强度随着功率密度的提高而增加, 到1017 W·cm–2以后逐渐进入饱和. 其后, 为更准确和细致地研究LPI相关过程, 他们专门发展了基于皮秒激光探针的汤姆逊散射技术, 通过直接测量等离子体中的波来研究SBS和SRS的发展规律[20]. 利用该方法, 还研究了侧向散射、多光束LPI相互影响现象等, 获得了一系列重要的进展[21,22]. 但整体来说, 此类研究还太少.
基于神光-Ⅱ升级及皮秒激光装置, 建立了皮秒激光驱动LPI的实验研究平台, 并开展了相应的实验研究. 针对背向SBS, 进行了仔细的研究, 发现在测量到的背向信号中, 除了真正的背向SBS信号, 还存在明显的来自于驱动激光的干扰信号. 对这些不同来源成分的甄别对于细致了解SBS等过程非常重要.
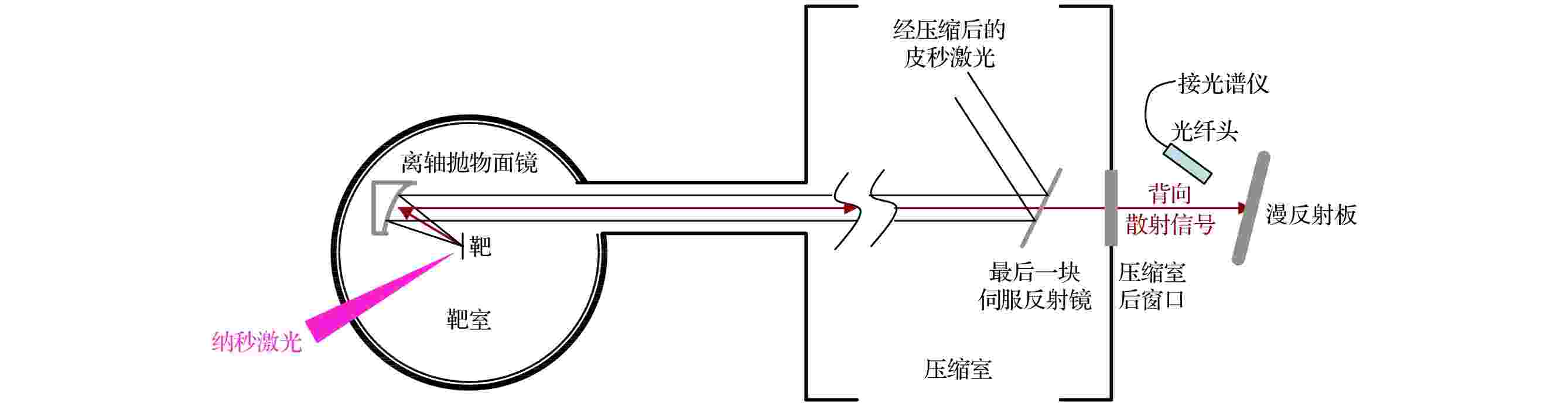
Figure1. Schematic diagram of backward SBS measurement scheme driven by a picosecond laser.
利用漫反射板来测量散射信号是一种常规的方法, 已经过多年验证, 在NIF等装置上已经成为常规的诊断技术[23]. 漫反射板是一种表面经过特殊处理的平板, 光束照射在漫反射板上, 发生漫反射, 漫反射的波谱不变, 强度则按照特定的规律分布, 因此通过在特定的方向上记录漫反射的信号, 经过标定和后期处理, 就可以得到入射光束的波谱、能量等信息.
在实验中, 漫反射板放置在皮秒激光的压缩室后面. 皮秒激光在靶位大尺度等离子体处诱发的背向SBS散射沿着皮秒激光的光路反向传播, 依次经过离轴抛物面镜反射、压缩室中最后一块伺服反射镜透射、压缩室后窗口透射后, 照射到漫反射板上. 在漫反射板之前, 设置有光纤头对着漫反射板被照射的区域, 背向SBS信号被光纤头收集后, 连接到光谱仪, 进行光谱信息的记录.
实验中采用三明治结构的平面靶, 如图2(a)所示, 三层分别为Al, C8H8和Al, 厚度分别为100, 2000和100 nm. 纳秒激光选用神光-Ⅱ升级装置的第5路单束激光, 波长351 nm(三倍频), 脉宽约1.2 ns, 每束能量约600—800 J, 以连续相位板(CPP)匀滑的方式聚焦在平面靶上, 焦斑约Φ600 μm, 靶面功率密度约1014 W·cm–2. 皮秒激光利用神光-Ⅱ升级装置的第9路皮秒激光束, 波长约1053 nm, 脉宽约8 ps, 能量30—50 J, 以离焦方式注入平面靶位置, 离焦后的焦斑约Φ200 μm, 靶面功率密度约 5 × 1015—8 × 1015 W·cm–2. 激光入射结构及焦斑示意图如图2(b)和图2(c)所示.
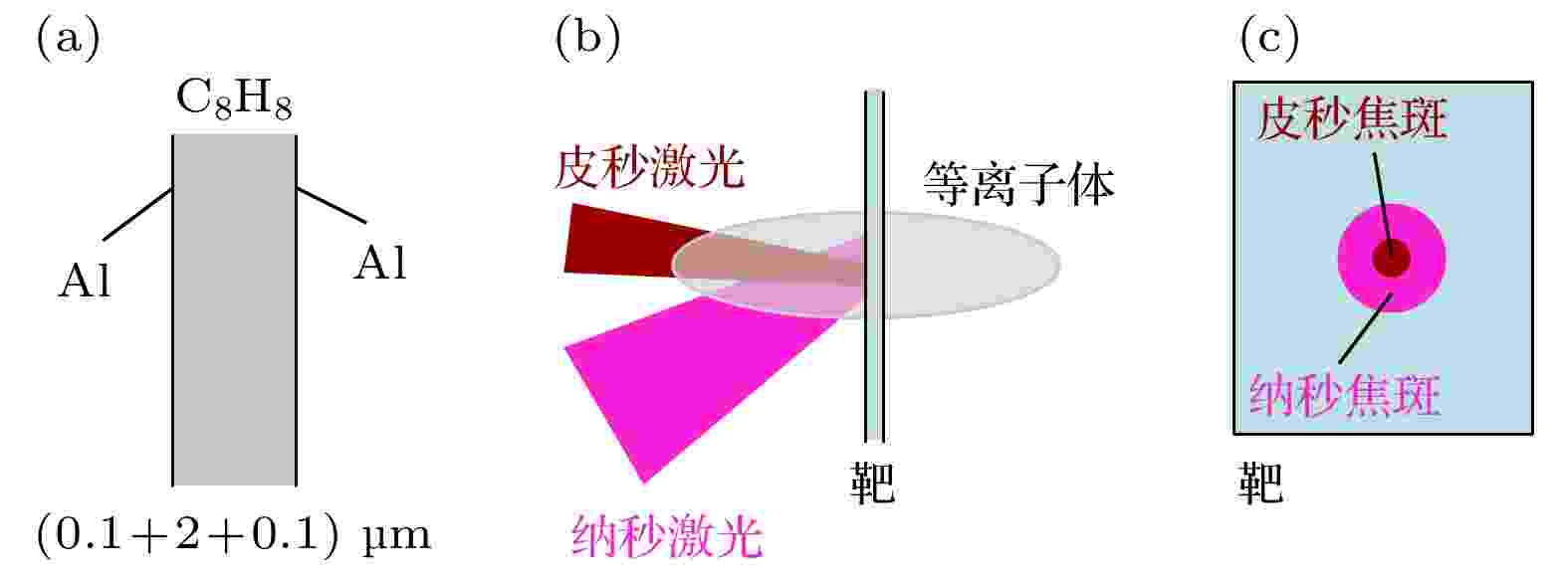
Figure2. Schematic diagram of experimental targets: (a) Sandwich structure; (b) target and driving laser; (c) focal spots of lasers.
图3是实验中获得的典型背向SBS光谱, 对应的驱动条件是第5路纳秒激光波长351 nm, 脉宽约1.2 ns, 能量约670 J, 第9路皮秒激光波长1053 nm, 脉宽约8.2 ps, 能量约30.3 J, 皮秒激光落后纳秒激光后延1.5 ns. 可以看出, 有效信号主要集中在 (1048—1058)nm之间, 其他区域无明显的信号痕迹. 这个光谱形状比较奇怪, 与传统的背向SBS光谱不同, 也与文献中的不同[11], 其中可能包含了不同的成分或来源. 经过仔细分析和多次迭代, 对光谱的结构和成分进行了分解和溯源. 通常激光的光谱形状接近高斯型, 因此以高斯函数进行拟合. 标准的高斯函数为
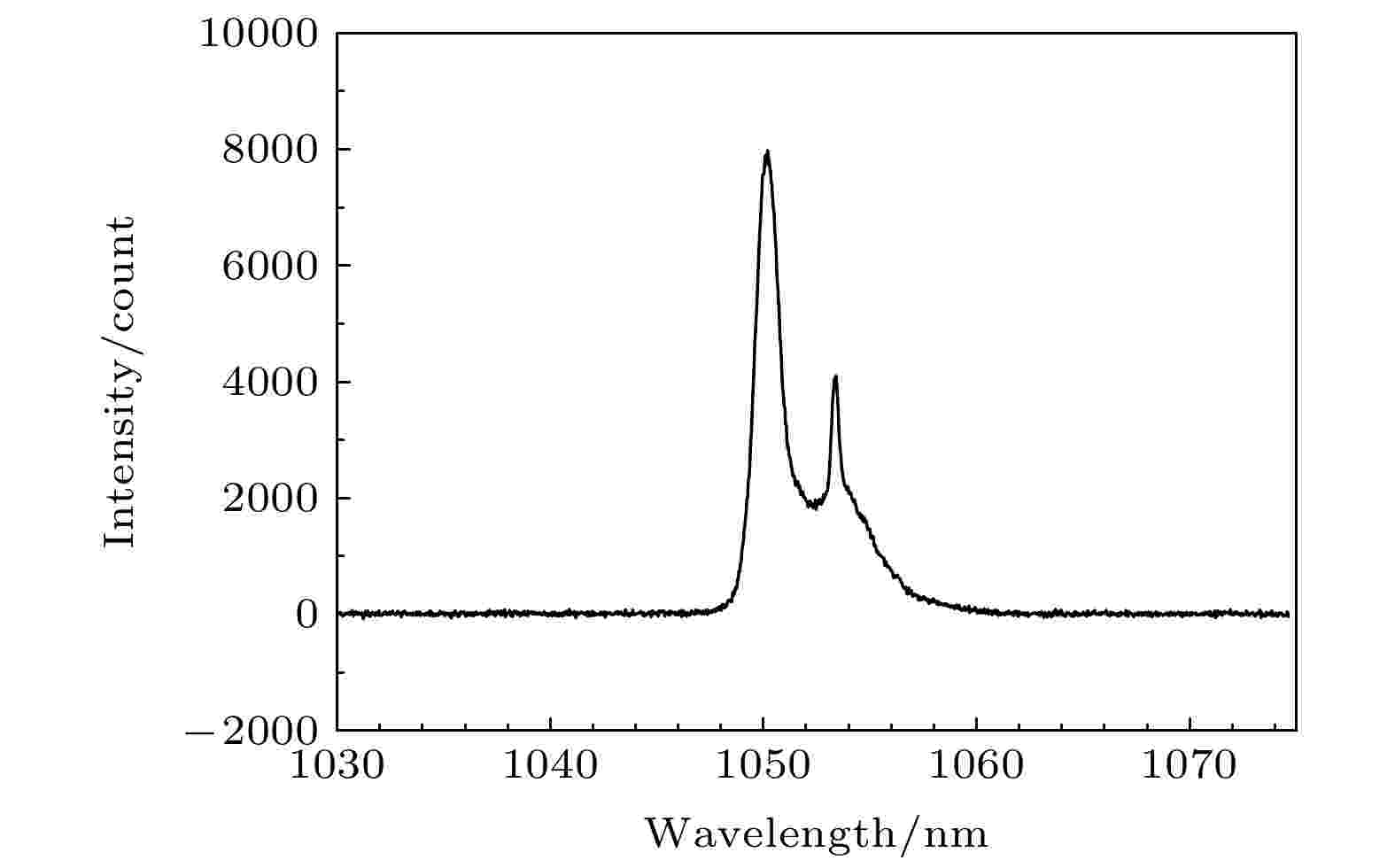
Figure3. Integrated spectroscopy of backward SBS induced by a picosecond laser.


图4(a)是针对图3光谱进行分解和拟合的结果, 可以看出, 整个光谱可以被分为三部分, 分别采用高斯函数拟合, 可以得到三个不同宽度和峰值的高斯函数, 如图4(a)所示, 分别记作P1(绿色方块标记)、P2(蓝色正三角标记)和P3(红色实心圆标记). 图4(b)给出了实验数据曲线(黑色空心圆标记)与三部分之和(P1 + P2 + P3)的曲线, 可以看出, 两者符合得非常好, 仅在中间交界的区域略有差异, 如图中蓝色虚线圈所示. 这表明, 这一分解和拟合基本上是合理、可信的.
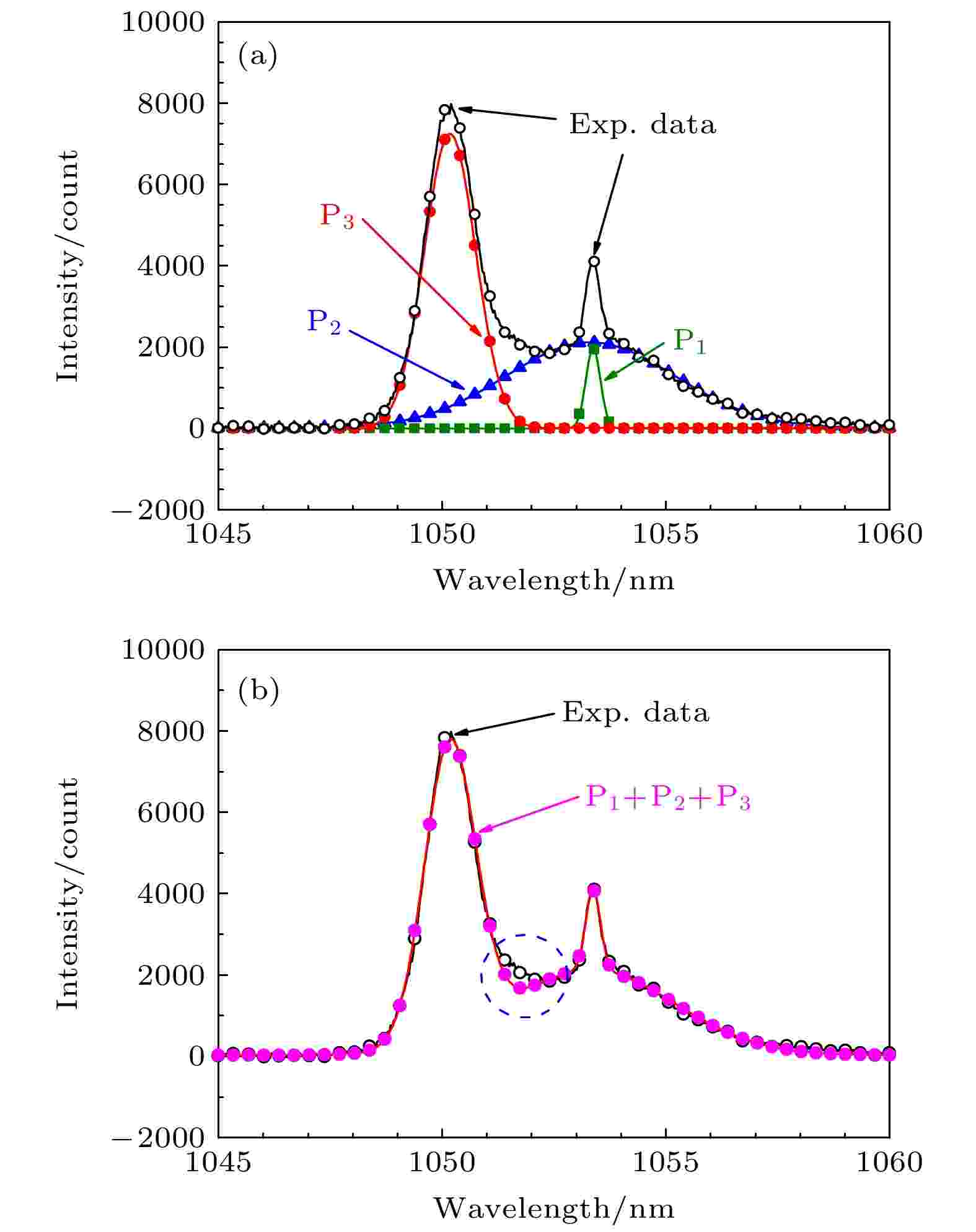
Figure4. Component decomposition of backward SBS spectrum: (a) Decomposed into three parts; (b) sum of the three parts and the experimental data.
峰值高度 | 中心波长 | 半高全宽 | |
a/count | x0/nm | τ/nm | |
P1 | 1976 | 1053.4 | 0.39 |
P2 | 2101 | 1053.3 | 4.4 |
P3 | 7243 | 1050.2 | 1.3 |
表1图4中各部分高斯拟合曲线参数
Table1.Gaussian fitting curve parameters of each part in Fig.4.
对于P1, 中心波长1053.4 nm, 光谱半高全宽约0.39 nm. 结合实验的条件, 我们认为这是由用于产生大尺度等离子体的神光-Ⅱ升级装置的纳秒激光引起的. 神光-Ⅱ升级装置的纳秒激光中心波长约1053 nm, 带宽约0.3 nm. 考虑到一般情况下驱动激光的实际运行波长可能与1053 nm有一定的偏差, 同时光栅谱仪狭缝全开导致的谱分辨率变差和波长校准的偏差, 在一定的误差范围内, 两者还是比较接近的. 用于产生等离子体的纳秒激光采用的是三倍频激光, 但由于采用了楔形透镜的终端系统, 仍有大量剩余的基频和二倍频激光进入靶室内部. 这些无用的基频和二倍频激光在靶附近与三倍频激光略微偏离, 不会影响正常的实验. 但这些光有可能照射到靶室内壁、底板、元件支架等处, 产生一定的噪声背景, 如果恰好有一部分进入到了背向散射的测量光路, 就可能被记录下来.
对于P2, 中心波长1053.3 nm, 光谱半高全宽约4.4 nm. 这是一个带宽较宽的峰, 与实验中用于相互作用的第9路皮秒激光的参数是类似的. 第9路皮秒激光中心波长约1053 nm, 带宽约3—4 nm. 同样考虑到驱动激光实际波长、光谱仪狭缝全开、波长校准偏差等因素, 两者还算基本符合. 这表明P2来源于皮秒激光. 另外, 如图2(c)所示, 靶的横向尺寸比焦斑大得多, 因此P2部分记录的是从靶上原路返回的信号. 皮秒激光斜入射辐照平面靶, 因此不存在直接的反射光, 可能的来源包括在等离子体临界面上的反射, 或者是波长变化很小的SBS散射等.
对于P3, 中心波长1050.2 nm, 光谱半高全宽约1.3 nm. 中心波长发生了明显的改变, 蓝移约3 nm, 半高全宽也发生了显著的变化, 在驱动激光中找不到类似的成分, 因此可以明确地认定这一部分是皮秒激光与大尺度等离子体相互作用产生的背向SBS信号.
事实上, 利用高斯函数来进行谱成分的拟合可能存在一定的问题. 对于上述P1, P2部分分别来源于本就是高斯型的纳秒和皮秒激光, 利用高斯函数来拟合是合适的. 但是SBS来源于激光与等离子体的非线性相互作用, 光谱结构通常非常复杂, 采用高斯函数拟合未必合适. 从具体结果来看, 采用高斯函数拟合P3, 造成了P1, P2和P3三部分之和与实验数据之间存在的差异(图4蓝色虚线圈部分). 更准确的方式应该是利用高斯函数拟合P1, P2, 然后利用实验数据减掉这两部分来获得纯粹的SBS信号. 原则上, 这会对SBS信号能量份额的估计有所影响, 但可以看出这一差异其实非常小, 基本上可以忽略.
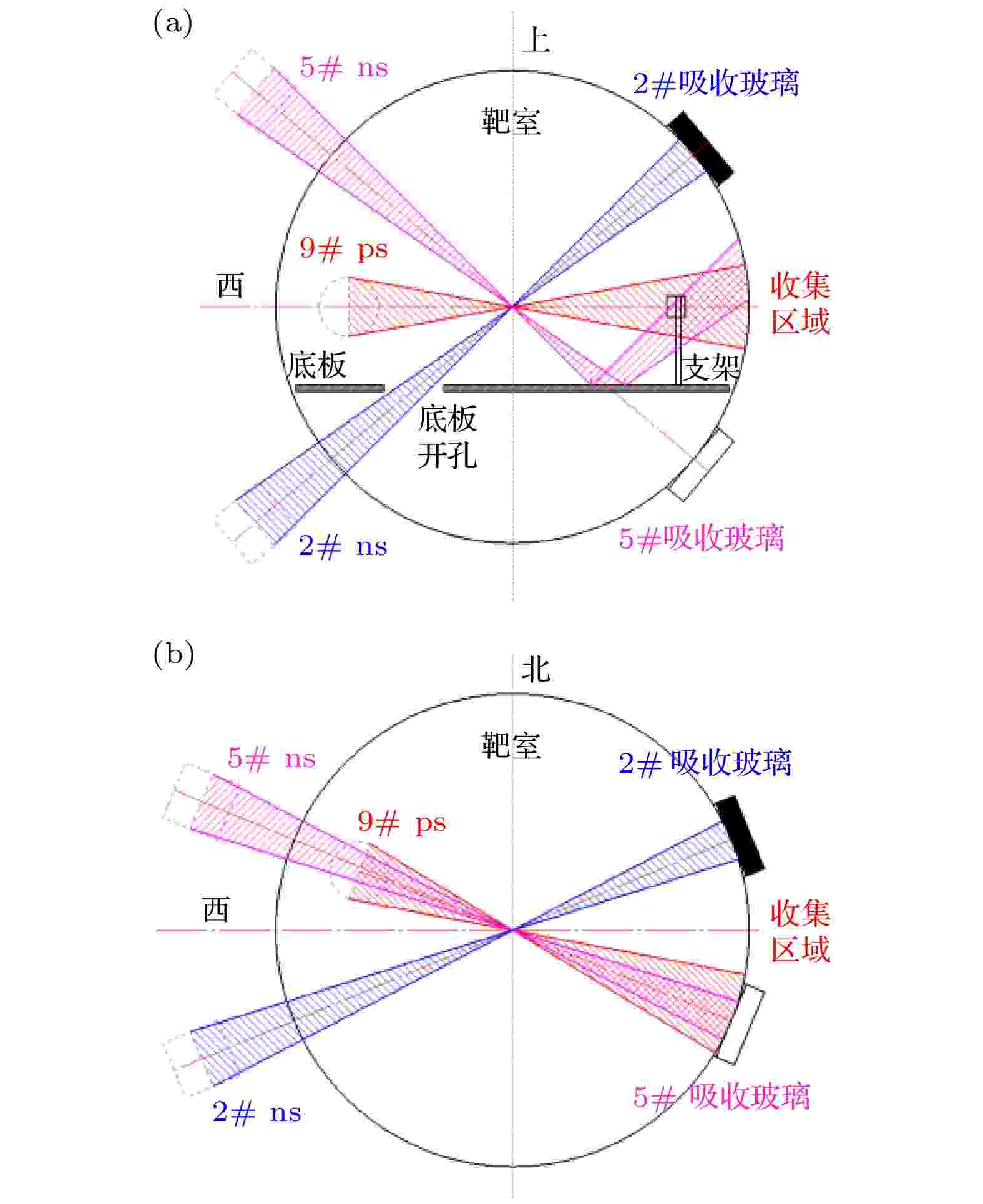
Figure5. Schematic diagram of laser beam distribution in the target chamber: (a) Side view; (b) top view.
为此, 考虑对方案进行改变, 选用第2路激光作为驱动产生大尺度等离子体的纳秒激光进行实验. 第2路纳秒激光从靶室赤道面下方入射, 与赤道面夹角40°, 西偏南22.5°入射, F# 约7.2, 与第5路相比, 除了上下入射方向不同, 对于法线正西的平面靶来说, 入射角度是一致的. 第2路纳秒激光从下方入射, 因此相应的底板必须拆卸, 光路中没有阻碍, 剩余激光直接进入吸收玻璃被吸收.
图6是利用第2路纳秒激光驱动产生大尺度等离子体获得的皮秒激光背向SBS光谱, 对应的驱动条件是第2路纳秒激光波长351 nm, 脉宽约1.2 ns, 能量约850 J, 第9路皮秒激光波长1053 nm, 脉宽约7.8 ps, 能量约44.5 J, 皮秒激光落后纳秒激光后延1.5 ns. 与图3和图4的实验结果相比, 驱动激光的能量略高, 其他条件相似. 同样, 有效信号主要集中在 (1048—1058) nm之间, 但与图4存在明显的差异, 即位于1053 nm附近的窄峰P1消失了. 这表明前文对P1来源的分析是合理的, 即P1来自于驱动大尺度等离子体的纳秒激光中的剩余基频激光在靶室中的残留背景. 图6中同时给出了各部分的拟合曲线, 参数如表2所列. 与表1相比, 在中心波长、半高全宽方面, P2, P3基本一致, 表明他们的来源与图4的实验是一致的.
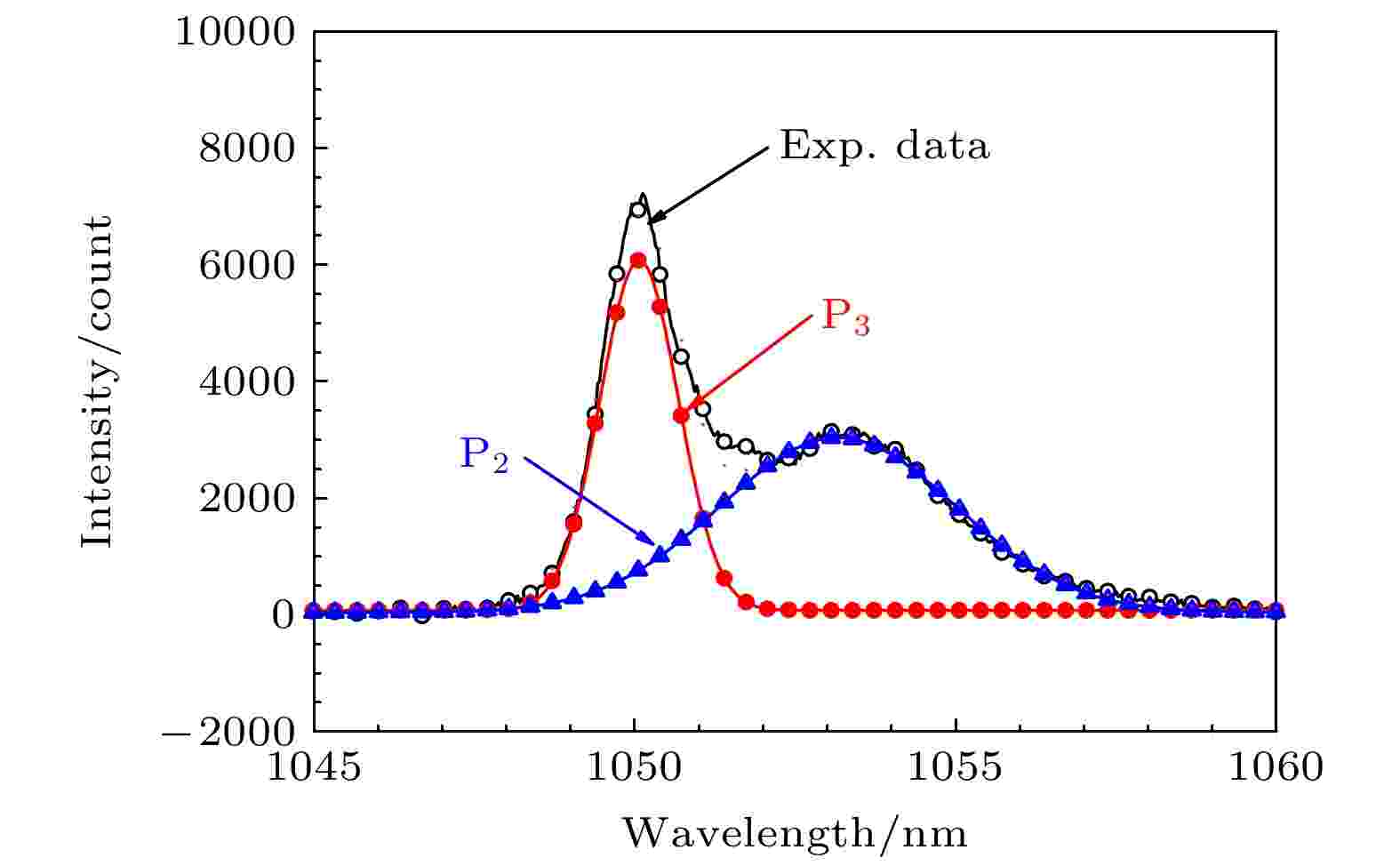
Figure6. Component decomposition of backward SBS spectrum driven by 2# nanosecond laser.
峰值高度 | 中心波长 | 半高全宽 | |
a/count | x0/nm | τ/nm | |
P1 | // | // | // |
P2 | 2994 | 1053.2 | 4.3 |
P3 | 5999 | 1050.1 | 1.4 |
表2针对图6第2路纳秒激光实验的各部分高斯拟合曲线参数
Table2.Gaussian fitting curve parameters of each part using 2# nanosecond laser shown in Fig.6.
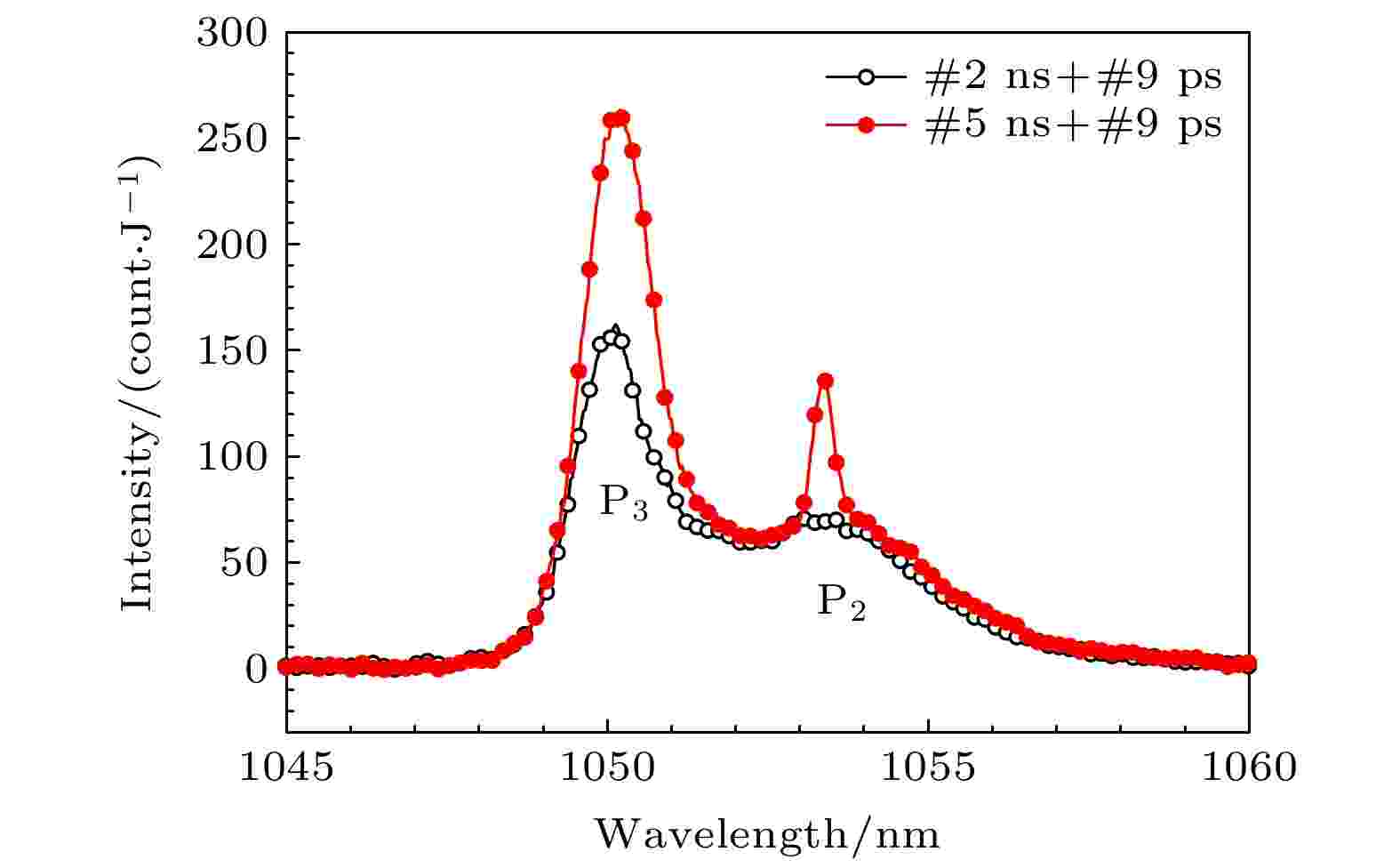
Figure7. Energy normalized spectra driven by 2# and 5# nanosecond laser respectively.
可以分别计算一下各成分的强度, 这里去除可以通过改变靶室内条件消除掉的P1成分, 只考虑皮秒激光带来的干扰信号P2和背向SBS信号P3. 对于图4发次, 拟合后的P2和P3对应的计数和分别是299120和309448, 即P3占总和的50.8%; 对于图6发次, 拟合后的P2和P3对应的计数和分别是415047和272709, 即P3占总和的39.7%. 可以看出, “纯粹”的背向SBS信号, 即P3部分, 其实在测量到的总的背向信号中的比重并不高, 有时甚至不到一半. 在本实验中, 恰好选择了纳秒激光辐照平面薄膜靶这种构型来产生大尺度的等离子体, 等离子体会向靶两侧持续膨胀, 作为相互作用的皮秒激光注入时, 遇到迎面而来的等离子体, 相互作用产生的背向SBS受到多普勒效应的影响而发生蓝移. SBS信号(P3)发生蓝移, 而作为皮秒激光噪声的P2不蓝移, 使得P2和P3信号可以分离, 进而能够比较准确地给出背向SBS的份额. 但在更普遍的情况下, 背向SBS会和干扰信号混杂在一起, 即P2和P3信号混在一起, 无法分离. 如果把测量到的激光波长附近的背向信号全部算为有效的背向SBS散射信号, 并不能反映实际的背向SBS信号的份额, 这无疑将对实验的理解产生巨大的影响. 因此在对待背向SBS散射测量数据时, 必须仔细地加以分析.
对于完全来自背向SBS的P3来说, 一个显著的特点是中心波长发生了蓝移. 蓝移的可能原因是皮秒激光注入时, 大尺度等离子体存在明显运动而引起的多普勒效应. 蓝移对应等离子体迎面而来. 在实验中, 纳秒激光辐照薄膜靶, 在其后的纳秒时间尺度内, 产生的等离子体分别向两侧膨胀, 皮秒激光在1.5 ns之后从一侧注入, 恰好遇上膨胀的等离子体相互作用, 发生蓝移的SBS. 另一个特点是P3的光谱峰的半高全宽只有 1.3—1.4 nm, 比入射的皮秒激光的半高全宽4.3—4.4 nm要窄得多. 表明其发生的区域其实是比较窄的, 那么具体发生区域密度是多少, 离子声波频率是多少? 以及峰值蓝移对应的等离子体的流速是多少等? 这些问题涉及到的因素比较复杂, 需要配合数值模拟进行深入的研究, 这也是下一步需要开展的主要工作之一.
值得注意的是, 上述实验中, 皮秒激光驱动对应的功率密度均在5 × 1015 W·cm–2甚至更高, 这一数值比ICF研究感兴趣的功率密度条件高得多. 通常情况, 对于基频激光驱动来说, 功率密度在1014 W·cm–2, 就能够激发出很强的背向SBS. 具体实验中, 也进行了几乘1014 W·cm–2等功率密度的实验, 但信号很弱或淹没在噪声中. 表明利用皮秒激光驱动可以显著地提高背向SBS的发生阈值, 与Rousseaux等[21]的结果也一致. 本实验的结论可能无法直接应用于ICF, 但对时间尺度相当的皮秒激光LPI的研究, 将有助于更深入、细致地研究SBS等LPI过程的基本原理和规律.
感谢神光-Ⅱ升级及皮秒装置全体运行人员的大力协助.