全文HTML
--> --> -->早在十多年前, 人们已经可以获得数十太瓦级的高功率商用激光器, 产生强度超过1018 W/cm2的超强飞秒激光脉冲, 使得光与物质相互作用进入到相对论非线性区域. 如此强的光场可以瞬间将物质电离并形成高温高密度等离子体. 等离子体中的电子被强激光场剧烈加速, 速度接近光速, 导致相对论效应变得非常显著. 强激光和等离子体相互作用伴随着高能粒子加速、高次谐波产生、超亮X/γ射线辐射等物理现象[6-8]. 随着国际上大量的数拍瓦与十拍瓦级激光装置的建造, 以及一些百拍瓦级激光装置的筹建, 未来有望在实验室中实现光强达到甚至超过1023 W/cm2的超高强度激光脉冲, 如图1所示. 我国的超强超短激光装置在过去的几十年内飞速发展, 取得了举世瞩目的成就. 例如, 上海超强激光装置在国际上率先实现了十拍瓦峰值功率的输出, 创造了世界纪录, 被国际著名《科学》杂志誉为强激光发展史上的五个里程碑工作之一. 数十拍瓦激光装置正在把光与物质作用推向极强场范畴, 由此将会激发许多新的物理效应, 诸如强辐射阻尼效应、多光子非线性Compton散射、超亮伽马辐射和正负电子对产生等非线性量子电动力学(quantum electrodynamics, QED)效应[9,10]. 本文简要回顾国内外在超强激光等离子体相互作用下产生超亮伽马辐射和稠密正负电子对方面取得的研究进展, 并对未来发展面临的主要挑战进行简单叙述, 以期让读者对该领域有一整体的了解.
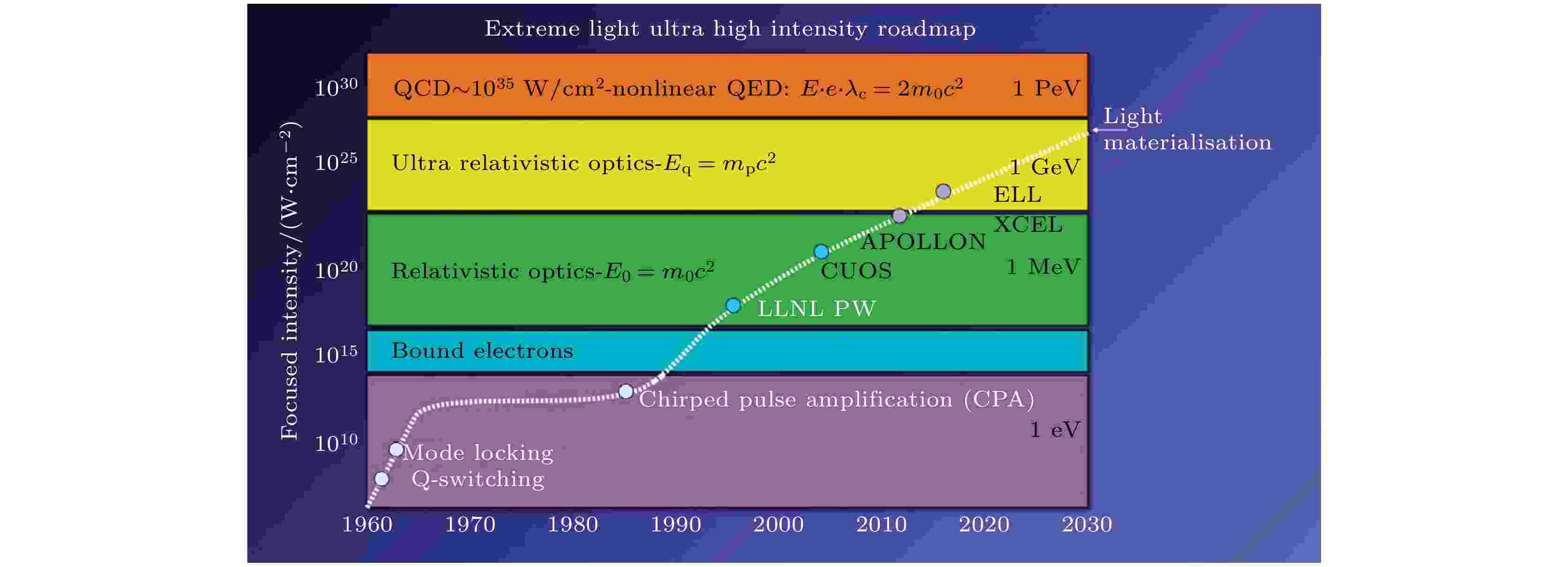
Figure1. Progress of the focused laser intensity over years and the development of laser-driven physics[4].
随着大型同步辐射装置的更新换代[15]和X射线自由电子激光(X-ray free-electron laser, XFEL)的建造[12], 人们已经可以获得X射线波段的高亮度辐射光源, 其产生的峰值亮度范围分别为1019—1024和1027—1032 (单位是photons/(s·mm2·mrad2·0.1%BW)). 并且, 由于同步辐射与XFEL光源在能谱、源尺寸、时间脉冲方面具有很好的可调控性, 因此在基础研究、新材料设计和制备、生物、工业领域有着广泛的用途. 但是, 这类大型装置高昂的造价、大尺寸规模、较少的数量等情况限制了它们的用户范围. 此外, X射线自由电子激光器和同步辐射装置所产生的辐射光子能量通常在1 MeV以下, 因此怎样产生更高光子能量的高亮度伽马射线脉冲是亟需解决的问题. 另一方面, 虽然基于超热电子与固体靶相互作用触发的轫致辐射可以产生MeV能量段的伽马射线源[16-18], 但是获得的脉宽长达皮秒量级、尺寸宽达百微米、发散角高达百毫弧度, 从而难以实现高准直性的超亮伽马射线脉冲. 为此, 2018年德国的Keitel研究组[19]提出利用数GeV能量的高品质稠密电子束与固体靶作用, 通过激发的电磁不稳定性可以产生高能类同步辐射, 比轫致辐射产生的光子数目和能量转换效率高几个数量级, 故有望实现超亮伽马射线源. 然而, 该方案需要的较高质量(6%能散、0.8 mrad发散角)稠密(约1020 cm–3) GeV电子束, 超出了现有的激光等离子体加速器技术所能够达到的水平, 所获得的伽马射线峰值亮度为1025 photons/(s·mm2·mrad2·0.1%BW). 目前还少有能够产生10 MeV、甚至GeV量级的超亮超短强伽马射线辐射源的有效方法.
2
2.1.基于激光尾场加速器产生超亮伽马辐射源的研究进展与挑战
近年来, 基于等离子体的激光尾波场加速器研究得到了迅速的发展[20-22]. 激光尾波场可以产生比传统射频加速器高三个数量级以上的超高加速场, 从而使得加速长度可以缩短到厘米甚至毫米尺度[23-25]. 这使得台面型高能粒子加速器和超亮高能辐射源成为了可能[6,8]. 目前, 基于激光尾波场加速器的单级电子加速能量已经可以达到8 GeV水平[26], 在未来有望实现将单级加速提高至10 GeV水平以及多级加速达到1 TeV量级的宏伟目标[27]. 基于激光尾波场加速器产生的高能电子, 通过其在尾波场中作剧烈的Betatron振荡运动或在外部电磁场中作同步辐射运动[28-32], 以及与强散射激光场对撞通过Thomson或Compton散射[33-36], 可以产生高亮度的X/γ射线脉冲. 所产生的辐射源脉冲宽度可短至数飞秒, 光子能量一般处于keV—MeV范围内, 峰值亮度约为1019—1023 photons/(s·mm2·mrad2·0.1%BW), 每发次获得的光子总数约为108量级. 最终, 获得的高能辐射源的能量转换效率约为10–6量级. 图2给出了基于激光等离子体方法、同步辐射源以及自由电子激光装置所产生的超亮辐射源峰值亮度和光子能量的范围. 表1列出了在当前实验条件下基于不同物理机制激光驱动的超亮X射线和伽马射线源参数的比较. 近年来, 虽然世界各地科学家付出了很多努力并提出很多方案来增强激光尾波场驱动的Betatron辐射, 例如利用高能粒子束驱动的等离子体尾波场[37,38], 或增加尾波场中电子的横向振荡振幅[39]等, 但是这些方案仍未能解决大幅度提高伽马辐射源的光子能量、能量转换效率和峰值亮度这一重大的科学难题. 然而, 在许多前沿领域研究中往往需要的是光子能量在MeV乃至GeV量级以上的超高亮度伽马射线脉冲, 例如研究光与光作用的基本物理过程[40,41]、探索伽马射线暴[42]、产生与探测基本粒子[43]、研究核结构或光核物理过程[44,45]、检验QED 效应[46]等.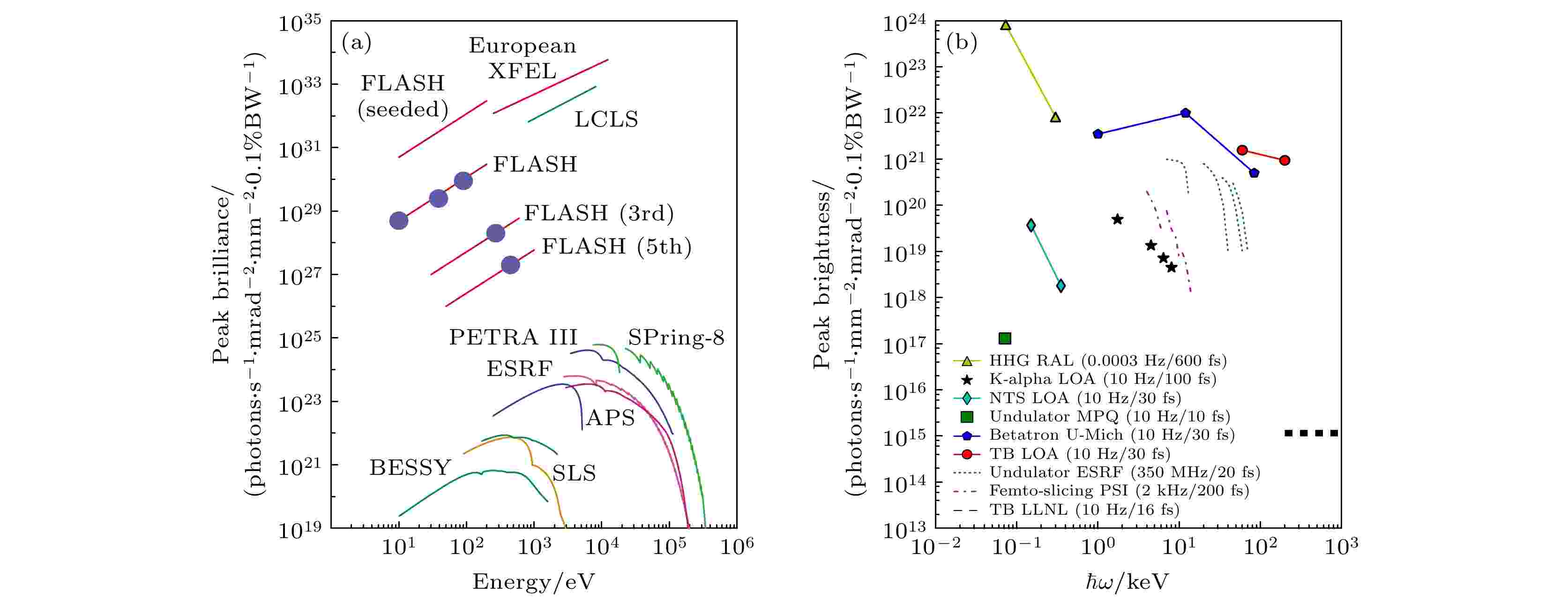
Figure2. Peak brilliance for different types of X-ray radiation sources from the third-generation synchrotron-radiation sources and XFELs[47] (a) and laser-plasma-based radiation sources[8] (b).
Betatron[48] | Compton[35] | Bremsstrahlung[49] | |
能量范围/MeV | ~0.1 | 0.3—2.0 | 0.1—30.0 |
带宽/% | ~100 | 33—60 | ~100 |
光子数 | 108—109 | 107—108 | 108—109 |
峰值亮度/ (photons·s–1· mm–2·mrad–2· 0.1%BW–1) | ~1023 | ~1022 | ~1017 |
尺寸/μm | ~5 | ~4 | ~100 |
脉宽/fs | ~10 | ~10 | ~104 |
发散角/mrad | ~5 | ~4 | ~40 |
表1当前实验中不同物理机制下激光驱动的X射线源和伽马射线源的性能比较
Table1.Comparison of the performance of laser-driven X-ray and gamma-ray sources under different physical mechanisms in current experiments.
2
2.2.基于极强激光场驱动超亮伽马辐射源的研究进展与挑战
另一方面, 基于正在建设的数十拍瓦高功率极强激光装置, 研究人员从理论和数值模拟方面做出了大量的研究, 提出了多种产生超亮伽马辐射源的方案. 例如, 超强激光与临界密度等离子体靶相互作用[50-52]、极强激光场激发的辐射阻尼效应[53,54]、超强激光与固体靶相互作用[55,56]、强场激光与高能电子对撞[57,58]、电磁级联效应[59,60]等. 然而, 这些方案存在着无法避免的物理局限性. 比如极强激光场与电子直接作用时会导致非常大的发散角, 难以产生峰值亮度可达自由电子激光水平的伽马射线脉冲. 获得的高能辐射源在光子能量、辐射功率和峰值亮度等方面的可调性也是有限的. 此外, 这些方案一般需要光场强度达到1023 W/cm2以上, 以产生GeV量级的伽马光子辐射. 这比目前实验中观测到的最高激光强度(5.5 × 1022 W/cm2)高了约1个数量级[61]. 当激光强度降低至目前实验室中可用的光强(约1021 W/cm2)范围时, 上述方案将变得无效或难以产生超亮高能辐射源. 这给实验探索和方案验证带来了极大的挑战, 限制了这些方案的实用性. 迄今为止, 尚未有合适的方法来实现峰值亮度可达X射线自由电子激光水平的极高亮度伽马射线辐射源.2
3.1.超强拍瓦激光驱动丝靶产生超亮伽马射线源的物理方案
由上文介绍可知, 高能伽马射线源在广泛的科学领域中具有重要的作用, 但想要获得能量在1 MeV以上的超亮伽马射线脉冲仍是一项巨大的挑战. 为了解决这一难题, 进一步将辐射光子的能量提高到GeV量级, 王伟民等[80]提出了一种利用拍瓦强激光脉冲与细丝靶作用的新方案, 如图3所示.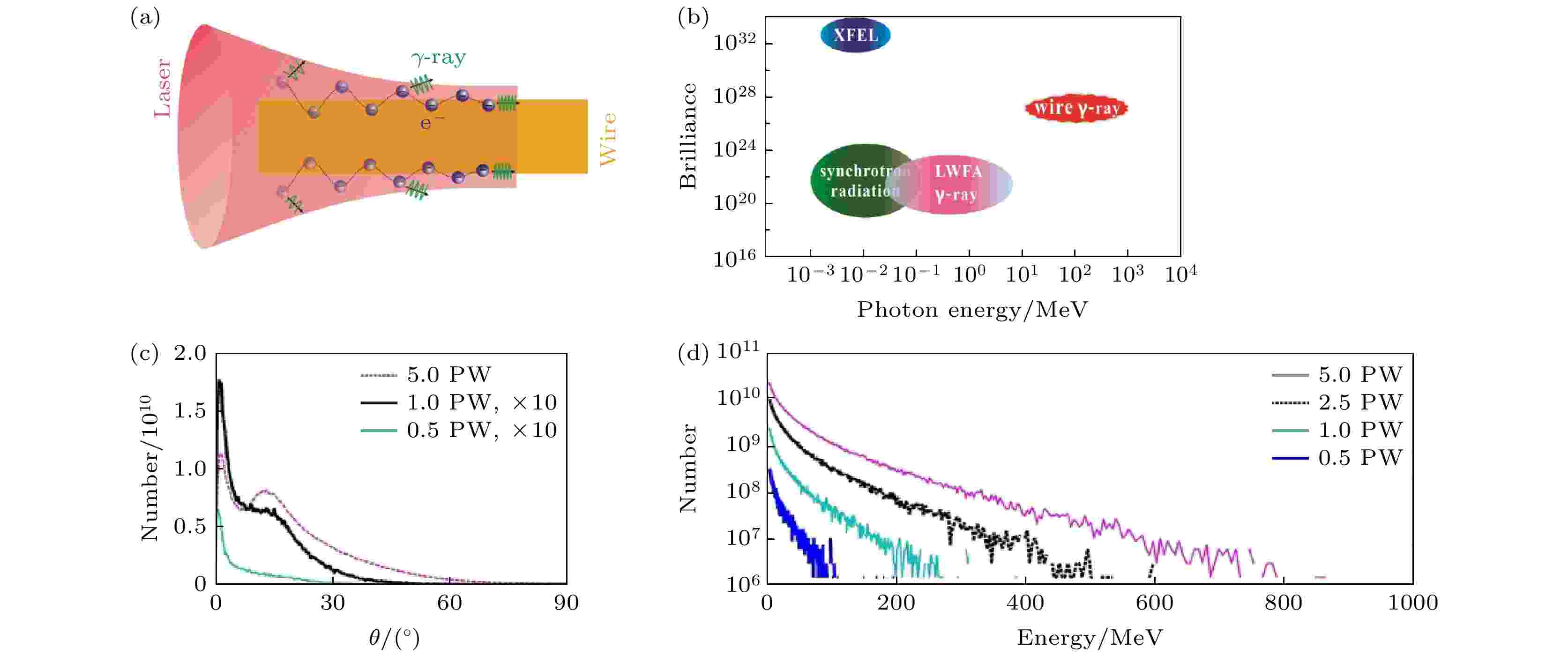
Figure3. (a) Schematic diagram of the wire scheme; (b) chart of photon energy and brilliance of gamma-rays generated from our wire scheme, XFEL, synchrotron radiation facilities, and betatron radiation and Compton scattering based on LWFA; the angular distributions (c) and energy spectra (d) of the generated gamma-rays under different laser powers, where “ ×10” in the legend indicates the photon number multiplied by a factor of 10[80].
利用强激光与横向尺度在激光波长量级的固体细丝靶作用, 发现当采用拍瓦量级功率的驱动激光时, 可以产生显著的QED效应. 当强激光场与细丝靶作用时, 一方面在细丝靶表面将靶电子加速至GeV量级, 另一方面在靶表面激发出很强的准静态横向电磁场, 后者在丝靶表面驱动高能电子作强烈的横向振荡, 从而激发超强类同步辐射, 并产生大量的高能伽马光子. 值得一提的是, 高密度固体细丝靶(或微通道等离子体靶[81])可以引导激光脉冲沿靶表面向前传播并加速电子向前运动, 产生大电量的准直GeV电子束, 获得的电子束总电量可达10 nC量级. 相比较而言, 基于气体靶尾场加速器的电子束电量一般仅有100 pC量级. 与此同时, 强激光场与固体细丝靶作用所激发的准静态横向电磁场可达1014 V/m量级. 在能量为GeV量级的电子静止坐标系下, 激发的振荡场将接近于Schwinger临界场强Esh = 1.32 × 1018 V/m(此场强意味着可以在真空中直接激发产生正负电子对)[82]. 由此引起的高能同步辐射将进入到QED范畴, 从而能够高效地产生高能量的强伽马射线脉冲, 并且靶表面形成的静电场和静磁场可以使得产生的高能电子和伽马光子具有很好的准直性. 王伟民等[80]利用他们自主开发的三维粒子模拟程序KLAPS进行模拟研究, 结果表明, 可以产生峰值亮度仅次于X射线自由电子激光装置水平的伽马射线脉冲, 且获得的光子能量可达几十MeV到几百MeV, 比XFEL的光子能量高3个数量级. 最终, 从激光脉冲到伽马射线源的能量转换效率可达10%左右. 与先前强激光与固体靶作用产生高能伽马射线的方案相比, 此方案不仅大大降低了对驱动激光功率的要求, 而且大幅提高伽马光束的准直性、大幅降低伽马光束的横向尺寸, 因此使得伽马射线源的峰值亮度得到了极大的提高, 可以达到1027 photons/(s·mm2·mrad2·0.1%BW).
2
3.2.利用激光等离子体加速器产生极高亮度GeV伽马辐射源的物理方案
接下来介绍基于激光等离子体加速器产生的超亮GeV伽马射线辐射源的最新研究进展. 众所周知, 电子失相长度是制约激光尾波场加速器的关键因素之一, 它与背景等离子体密度成反比(
为了突破这一困境, 近期上海交通大学朱兴龙等[66]提出了一种基于两级激光等离子体加速器的新物理方案, 通过利用适度低密度等离子体加速产生大电量高能稠密电子束、并结合相对高密度等离子体来辐射超强伽马射线脉冲, 如图4所示. 在该方案中, 首先将一束聚焦光强约为1021 W/cm2的拍瓦激光脉冲入射到密度约为1020 cm–3的等离子体中, 由此激发产生一个高度非线性的等离子体尾波场. 为了能够有效地引导激光脉冲在等离子体中长距离地传输, 这里采用了一个横向密度分布为抛物线型的等离子体通道靶. 在第一级中, 大量的背景等离子体电子被俘获到拍瓦激光驱动的高强度非线性尾波场中并被加速, 从而产生密度高达临界等离子体密度(1021 cm–3)、几十nC电量、低发散角的GeV电子束. 通过这一级高效的等离子体尾波场加速, 从激光脉冲转换为GeV电子束的能量转换效率可以达到40%左右. 处于能谱中准单能峰范围的电子能量转换效率约为22%, 与文献[83]中理论预测结果(约20%)是一致的. 随后, 聚焦的强激光场驱动加速电子束共同进入一个密度更高的第二级等离子体中. 在此过程中, 等离子体空泡尺寸发生剧烈的收缩, 从而进一步压缩俘获电子束并增强其束密度. 另一方面, 在高密度等离子体中, 由于尾波相速度的减小引起额外的电子注入, 使得俘获电子束电量进一步增加至40 nC. 最终, 获得的GeV电子束能量转换效率可提高至50%以上. 由此激发产生更强的准静态自生电磁场, 进而触发量子辐射效应, 产生光子能量可达GeV量级的超亮伽马射线脉冲. 该方案的独特之处在于所获得的电子加速效率和高能光子辐射效率都非常高. 产生的GeV电子束总能量可超过100 J, 对应的能量转换效率约为50%. 产生光子能量在1 MeV以上的伽马射线辐射效率高达10%以上. 因此, 与之前基于百太瓦级激光驱动的单级激光尾波场加速器产生的Betatron辐射或Compton散射源方案相比, 利用这种超强拍瓦激光驱动的两级等离子体加速器方案有望能够将伽马辐射源的光子数目、能量转换效率、峰值亮度和辐射功率提高3—4个数量级.
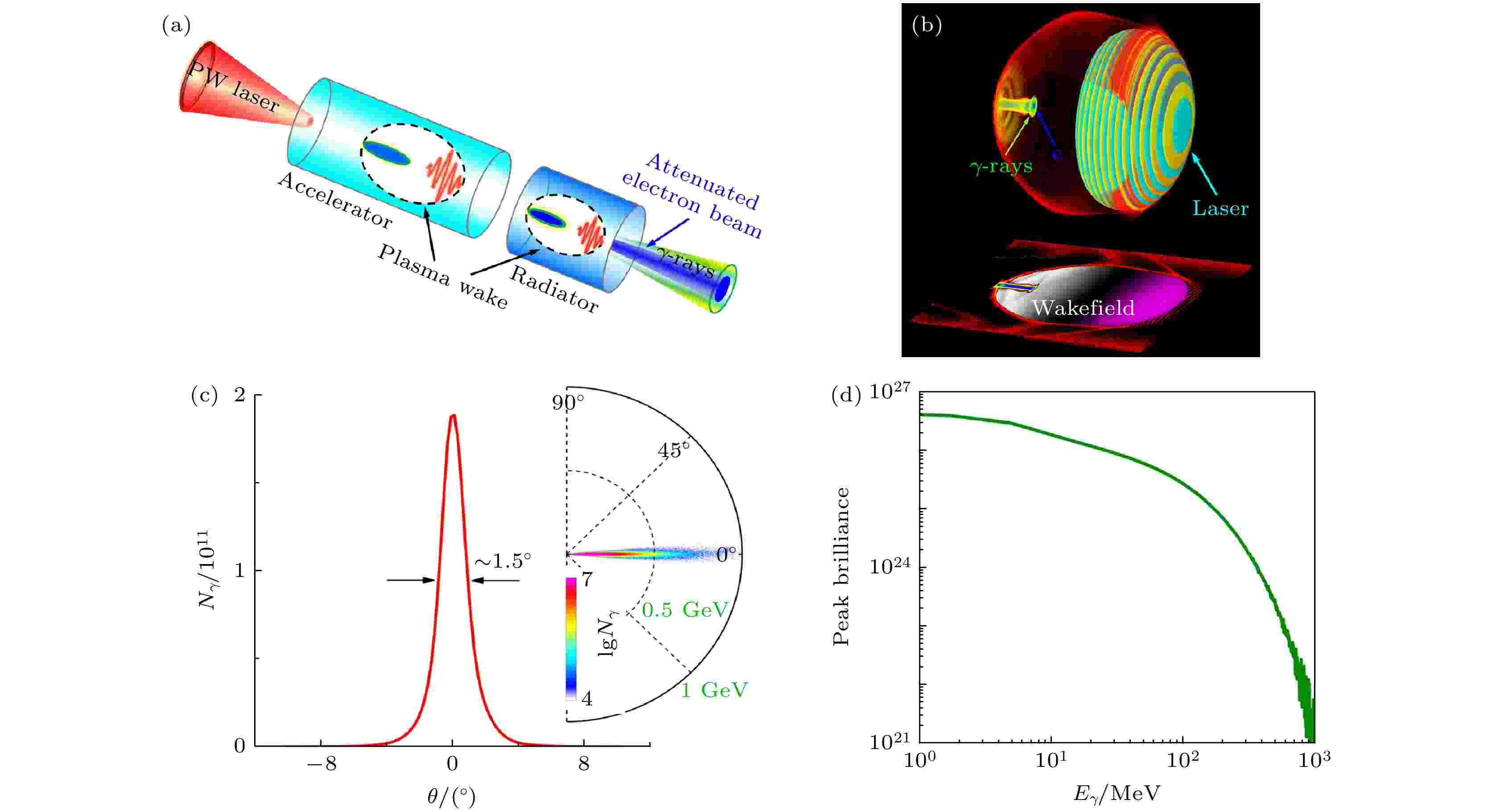
Figure4. (a) Concept of extremely brilliant γ-rays from a two-stage laser-plasma accelerator; (b) 3D simulation results of collimated γ-rays radiation in the two-stage LWFA scheme; (c) the angular-spectrum and angular distribution of the emitted gamma-rays; (d) the gamma-ray peak brilliance (photons/(s·mm2·mrad2·0.1%BW)) as a function of the radiated photon energy[66].
2
3.3.超亮阿秒伽马射线脉冲的研究进展
众所周知, 高次谐波辐射为产生阿秒光脉冲提供了一种有效的途径. 但是, 一般通过高次谐波辐射只能获得极紫外波段的光源, 光子能量限制在keV能量范围内. 对于核物理和核光子学等研究而言, 还亟需伽马射线波段的高能强辐射源, 例如研究核内动力学、核结构、核共振荧光反应等. 虽然通过非线性Thomson或Compton散射可以产生光子能量高达数十MeV的伽马射线脉冲, 但是所获得的脉冲宽度至少为数飞秒以上. 因此, 利用现有的方法难以产生脉宽短至阿秒尺度的伽马射线源. 另一方面, 携带角动量的光子束将会提供新的自由度, 为探索光与粒子之间角动量转移过程、操控粒子动力学等研究提供可能. 目前为止, 尚未有合适的方法产生角动量可调的超亮阿秒伽马射线脉冲.为此, 朱兴龙等[64]提出利用一束圆偏振拉盖尔高斯激光脉冲与锥-固体复合靶作用来产生角动量可调的阿秒MeV伽马射线脉冲的新方案, 如图5所示. 该方案为实现激光聚焦、电子加速和超亮伽马射线辐射提供了一种全光学、高效的三合一方法. 当入射的驱动激光脉冲穿过锥靶时, 其横向分布将被显著地聚焦, 聚焦后的光场强度可提高近一个数量级, 如图5(b),(c)所示. 在激光场与锥靶(或微通道靶[81])相互作用过程中, 大量背景电子将从锥壁中被周期性地拉出, 形成一串稠密的阿秒电子束列. 由此产生一串具有环状结构和百阿秒尺度的超短电子束列. 这主要是由圆偏振拉盖尔高斯激光场的特殊结构所造成的, 该光场在径向和方位角方向上具有独特的电场分量, 呈现出空间周期性的环形分布. 这种独特的电场结构导致电子动力学和运动特性与常规的圆偏振高斯激光场与等离子体相互作用情况有着显著的区别. 在这种结构的激光场驱动下, 电子沿着纵向可以被有效地加速, 并逐渐形成间隔约为一个激光波长的环形电子束列. 之后, 加速的高能电子束与被固体靶反射的聚焦强激光场对撞, 从而触发非线性Compton散射辐射, 产生一串高亮度的阿秒伽马射线脉冲, 并获得与高能电子束相同的环状结构, 如图5(e)所示. 在该相互作用过程中, 由能量和动量守恒可知, 辐射的伽马光子束将从高能电子束中获得相应的能量和角动量. 通过改变驱动激光参数和等离子体靶参数可以对加速电子束角动量和能量以及产生的伽马光子束参数进行调节. 此外, 利用激光条纹技术还有望实现对阿秒电子动力学过程进行实时测量和直接探测[84].
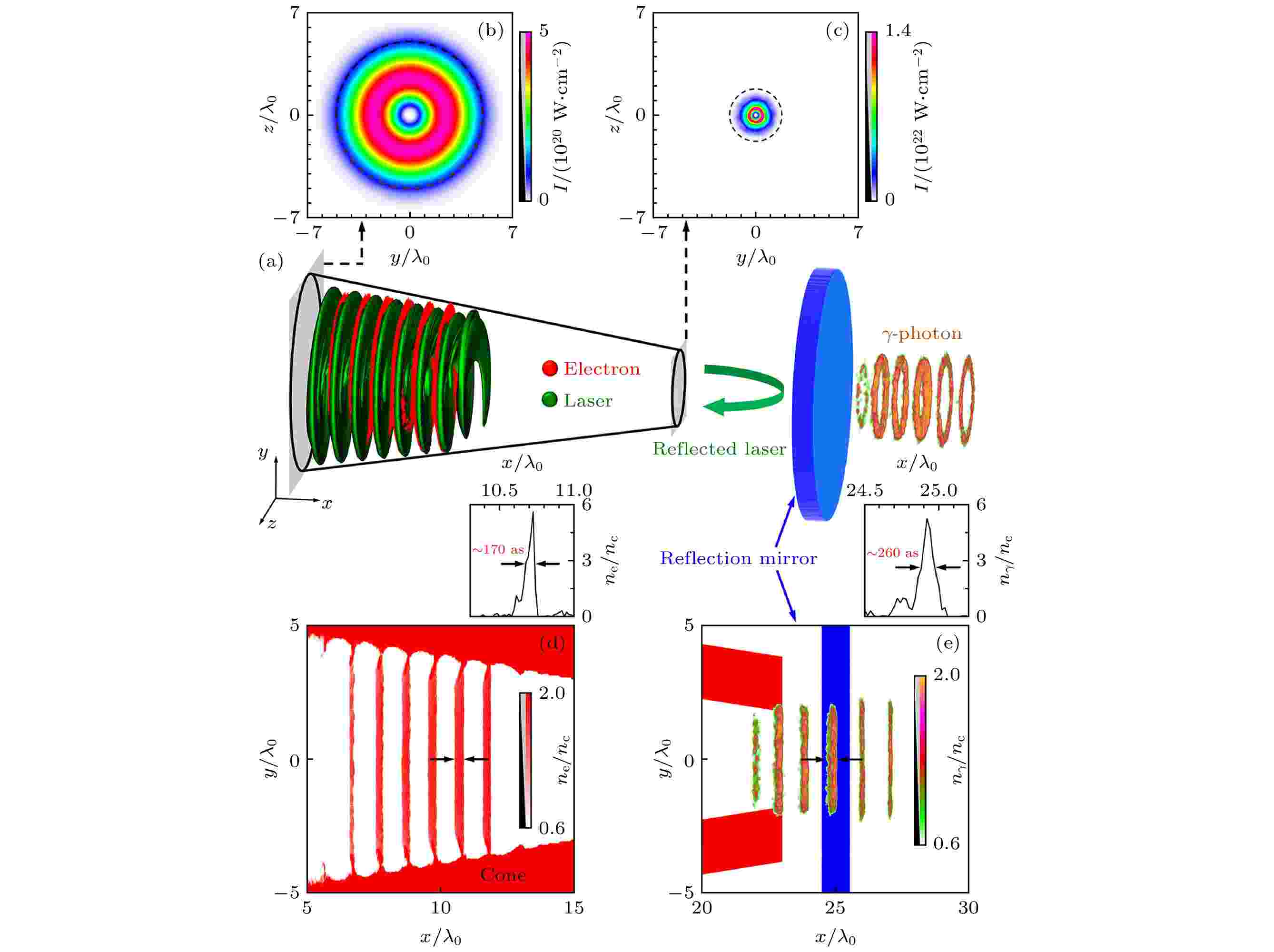
Figure5. (a) Schematic diagram of attosecond γ-ray pulse generation from a circularly-polarized Laguerre-Gaussian laser-driven cone-foil target. Electrons (red rings) are extracted from the cone walls and accelerated by the focusing laser. Then, the focusing laser pulse is reflected by a plasma mirror/foil (blue plate) and collides head-on with the dense energetic attosecond electron bunches, resulting in efficient emission of bright multi-MeV attosecond γ-ray pulses. The spatial distributions of the laser intensity for the incident pulse (b) and in-cone pulse (c). Density distributions of electrons (d) and γ-photons (e)[64].
新一代十拍瓦级激光装置有望使得聚焦光强达到1023 W/cm2, 导致光与物质相互作用进入到QED主导的机制范畴, 从而触发高能伽马光子辐射和稠密正负电子对产生. 理论和数值模拟研究表明, 当驱动光强达到1023 W/cm2以上时, 极端强激光场驱动的非线性多光子Breit-Wheeler过程[96]将为产生高能稠密正电子源提供一种有效的途径[97,98]. 但是, 在相对低强度的驱动光场(约1022 W/cm2)情况下, 如何能够高效地获得准直的稠密GeV正负电子对源, 并具有准中性的束流特性和可控的束结构, 是一项巨大的挑战. 另一方面, 携带角动量的高能粒子束具有额外的自由度特性和独特的能力, 将为基础物理研究、粒子属性探测、涡旋光产生等方面研究提供一种极具潜力的新手段. 在20世纪30年代, 研究人员就已发现了圆偏振光束具有光力矩的特性[99], 可以将光子自旋角动量转移给带电粒子[100,101], 从而可以用来操控粒子的运动特性. 随着激光强度的显著增加, 例如在强激光驱动电子加速[102,103]和高能光子辐射[64,104,105]等研究中, 这种现象变得高度非线性、并进入到相对论区域.
针对上述难题, 2016年朱兴龙等[106]首次提出一种基于双束拍瓦强激光与充满近临界密度等离子体的锥靶作用来实现激光对撞机的全新物理方案, 理论预测了高达数GeV能量的稠密正负电子对束流和强伽马射线脉冲的产生. 为了进一步实现对伽马光束和正电子束流的操控, 2018年朱兴龙等[65]研究并提出利用两束偏振态可调的激光脉冲与临界密度等离子体作用可以有效地产生角动量可调的超亮伽马光子束和稠密GeV正负电子对束流. 在该机制中, 等离子体充当着“转换介质”的作用. 首先, 被俘获的高能电子从驱动激光场中获得能量和角动量, 然后通过非线性Compton 散射产生伽马光子, 辐射的伽马光子将从电子束中获得相应的角动量. 随后, 通过高能光子与超强散射激光场对撞, 触发多光子Breit-Wheeler过程来产生高能稠密的正负电子对束流. 与此同时, 产生的正负电子对将从湮灭的伽马光子中获得相应的能量和角动量. 另一方面, 为了实现阿秒尺度的正电子束流, 2019年我们在前面工作的基础上提出了一种可以产生脉宽短至400 as的超短GeV正电子束流的方案[107]. 该方案采用一束十拍瓦级的线偏振激光脉冲与临界密度等离子体通道靶作用, 在辐射俘获效应和自生强磁场的共同作用下, 聚焦的强激光场驱动等离子体产生阿秒量级的俘获电子束, 并辐射产生阿秒伽马射线脉冲. 随后, 加速的高能电子束和增强的阿秒伽马射线脉冲与另一束相向传播的十拍瓦级激光场进一步作用产生准直的阿秒GeV正电子束流. 此外, 通过改变等离子体靶长度和横向空间分布以及激光等参数可以进一步提高正电子束流和强伽马射线辐射.
但是, 想要作为未来正负电子对撞机的候选粒子束源, 还必须解决正电子加速、能散、发射度等关键性的问题. 此外, 正电子的极化也是一个至关重要的属性, 它将提高正负电子对撞机的研究能力[87]. 为此, 近年来研究人员付出了大量的努力, 例如基于斯坦福直线加速器产生的20 GeV正电子束通过其在等离子体中自激发的尾波场[108]或激光驱动的中空等离子体通道中[109]可以获得数GeV的能量增益; 葡萄牙Vieira 和Mendon?a[110]提出利用相对论拉盖尔高斯激光驱动类“甜甜圈”结构的非线性尾场来加速和聚焦外注入的正电子束; 德国Keitel研究组[111]提出利用十拍瓦级超强双色场与高能GeV电子束对撞产生极化正电子束; 沈百飞课题组[112]提出在外加数十特斯拉磁场作用下利用纳库级电子束激发的相干渡越辐射场可以加速获得数百MeV的正电子束; 西安交通大学李彦霏等[75]提出利用极化电子与超强激光场作用产生高度极化的正电子束等. 虽然这些方案都取得了一些重要进展, 但是仍未解决如何实现正电子产生、加速和极化这一整体连贯的物理过程. 值得一提的是最近上海交通大学与西安交通大学联合团队提出利用中空电子束驱动非线性尾波场来俘获、加速和聚焦自产生的极化正电子束[113], 有望为这一难题提供新的思路. 不过, 目前仍有大量难题亟待解决, 例如如何实现单能正电子加速、提高正电子能量增益、操控正电子极化度和方向等, 以及未来在实验室中如何进行相关的实验研究和应用.