全文HTML
--> --> -->气相沉积是指含有成膜元素的气态物质经过物理或化学过程析出在固态衬底上, 从而成膜, 相应地可以分为两大类: 一类是物理气相沉积(physical vapor deposition, PVD), 另一类是化学气相沉积(chemical vapor deposition, CVD). 二者的本质区别在于沉积的薄膜组成材料与所蒸发的材料是否一致. PVD中蒸发和沉积只发生物理过程, 在沉积前后, 材料仅从蒸发源通过气相转移到基底上去, 并没有发生化学性质的变化. 而CVD则是把含有构成元素薄膜的气态反应物质或者经过气化的固体、液体物质引入反应室, 在气相中或衬底表面发生化学反应形成薄膜的过程[12].
本文基于原子制造在当今微电子领域的重要性, 介绍几种具有原子精度构筑低维材料的气相沉积技术, 从原理上理解其原子制造能力, 结合具体实例探讨其在纳米器件制备与加工领域的应用与发展.
MBE技术是在20世纪70年代发展起来的一种高质量薄膜生长技术, 是利用真空中加热蒸发材料, 在衬底沉积原子或分子, 该技术是由贝尔实验室的Cho和Arthur[20]发明. 由于在高真空中气压很低(P ≤ 10–3 Pa), 原子分子数目很少, 其平均自由程(mean free path)长达数米, 因此蒸发出来的原子或分子是径直喷射到衬底表面, 经过在晶体表面上的吸附、扩散、成膜等过程实现外延生长. 但在实际的MBE生长系统中, 往往需要更高真空度的超高真空环境(P ≤ 10–7 Pa)以保证材料生长过程中不受外来物质污染, 生长出纯度极高的材料.
MBE系统中通常的材料源为元素单质蒸发源, 而不是目标化合物材料直接蒸发. 例如, 生长GaAs薄膜, 通常需要独立的单质Ga源和As源, 而不是直接用GaAs源, 因此MBE方法被形象地称为“原子喷涂技术” (atomic spray painting). 因为大多数化合物在加热蒸发的时候, 组成化合物的不同元素原子不会同时被蒸发出来, 这与通常所认识到的有机分子蒸发情况有所不同. 对于直接蒸发GaAs源制备GaAs薄膜, As原子会率先被蒸发出来, 即在刚开始基本上都是As原子, 而到后来As原子快消耗完时, Ga原子才会大量的被蒸出, 这显然不利于制备高质量的GaAs薄膜.
在MBE生长过程中, 衬底温度也是决定薄膜质量的重要因素. 低温时, 衬底表面原子的动能不足以克服不同吸附位置间的扩散势垒, 遂将随机无序地稳定吸附在表面, 导致晶体薄膜质量下降; 而在较高温度的衬底表面上, 原子吸附在表面后将会脱附或者解离, 从而导致生长速度变慢或者薄膜质量下降; 只有在衬底温度处于适中范围时, 原子将具有合适动能, 可以在表面进行扩散重新排列, 形成高质量有序结构.
MBE系统的结构如图1所示. 为了实时观测MBE的薄膜生长质量和厚度, 通常配备反射式高能电子衍射(reflection high energy electron diffraction, RHEED)[30,31], 其工作原理如图2所示, 一束10—30 keV高能电子以1°—2°小角度掠入射到衬底表面, 经过表面晶格的衍射后, 在荧光屏上产生衍射条纹. MBE的先驱者Wood[32]在MBE生长过程中发现, 薄膜的RHEED衍射图样的强度, 尤其是镜面反射点的强度随着生长过程周期波动(oscillation), 每个亮暗周期对应着一个单原子层的生长.
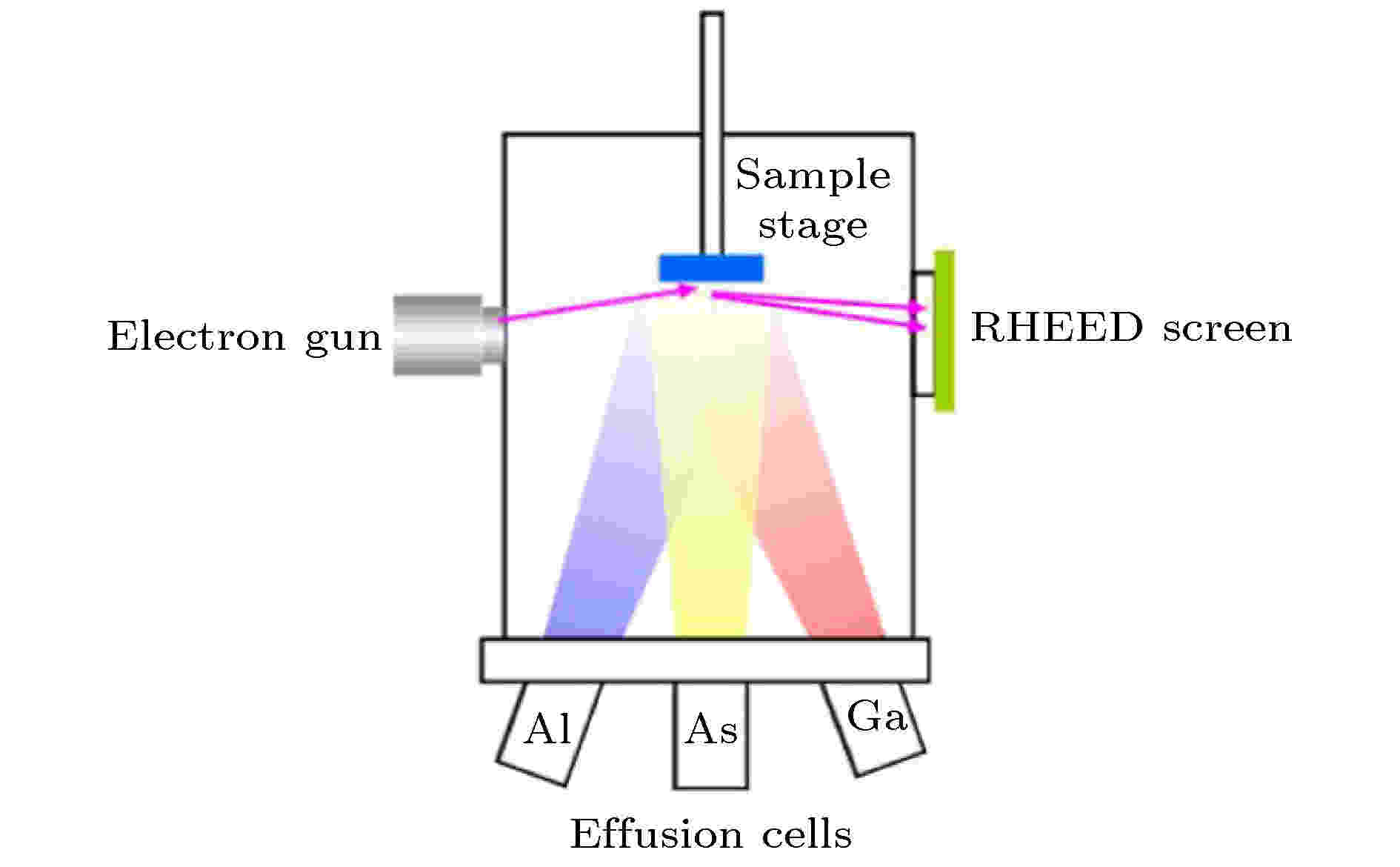
Figure1. Schematic diagram of MBE system.
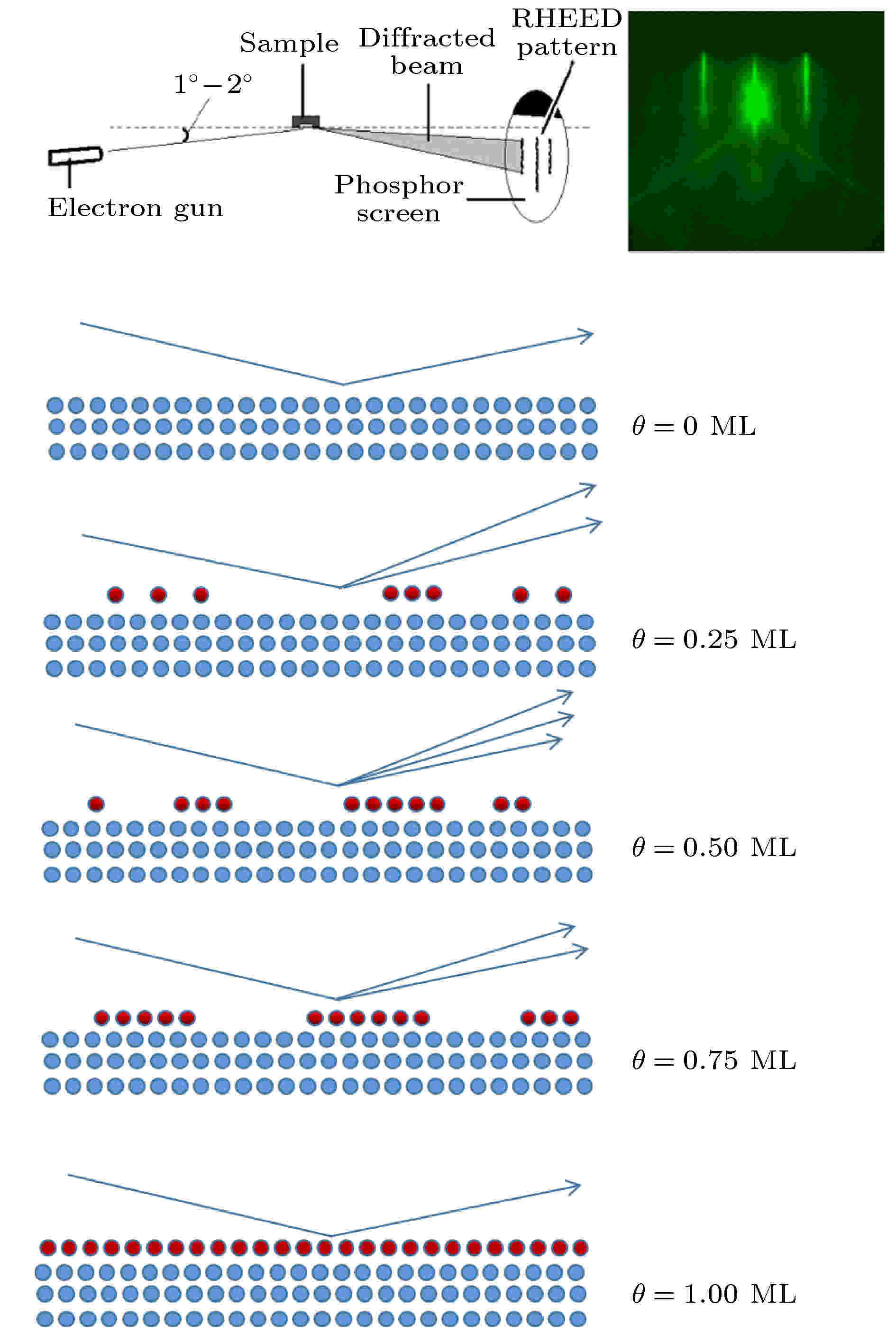
Figure2. Schematic diagram of RHEED, and the relationship between diffuse reflection and film coverage during growth.
单原子层从成核到长大过程中(如图2所示), 由于表面粗糙度的变化, 使得表面漫反射增强, 而镜面反射强度变小, 在单层膜长大到一半覆盖度的时候, 粗糙度最大, 漫反射最大, 而随后随着原子层继续长大镜面反射逐渐增强, 从而形成周期波动的现象, 而波动周期对应着是单原子层沉积, 可用来实时监测MBE生长过程, 实现单原子层的精确控制.
MBE技术由于生长环境干净, 可以制备出纯度高、缺陷少、结晶度高、组分可控的薄膜, 而配合RHEED又可以实现单原子层的生长和实时监控. 正是利用MBE这些优势, 研究人员在SrTiO3(111)表面MBE生长了Cr0.15(Bi0.1Sb0.9)1.85Te3薄膜, 该薄膜在极低温下(30 mK)呈现出显著的量子反常霍尔现象[33], 电荷输运测量显示出反常霍尔电阻为25800Ω, 与理论预测值h/e2一致(h为普朗克常数, e为电子电量). 量子霍尔效应是利用电子结构的拓扑性质使其边缘电子态可在宏观尺度保持其量子力学特性, 尤其是无能耗的性质. 而该工作中实现的量子反常霍尔效应巧妙利用材料自身磁场, 使其可以在不需要外加磁场的状态下就可以实现量子霍尔效应, 为现代工业中微纳器件的功耗问题提供了非常有前景的解决方案[33-35].
MBE生长最关键的要素就是沉积速率很低, 使得表面吸附原子后能够充分扩散成核形成高质量外延薄膜, 因此MBE还被广泛应用于生长单层或少层超薄膜, 比如制备各种低维材料和超薄电荷屏蔽层等[36-41].
锗烯是非常重要的类石墨烯材料, 理论预测锗烯具有比石墨烯和硅烯更强的自旋轨道耦合能隙, 理论研究表明锗烯具有量子自旋霍尔效应[42]和高温超导[43]等非常重要的电子学特性. 研究人员首次成功利用MBE方法在Pt(111)衬底上制备出高质量的二维原子晶体-锗烯[37], 如图3所示. 扫描隧道显微镜(STM)结合低能电子衍射(LEED)结果研究发现锗原子在Pt(111)表面形成

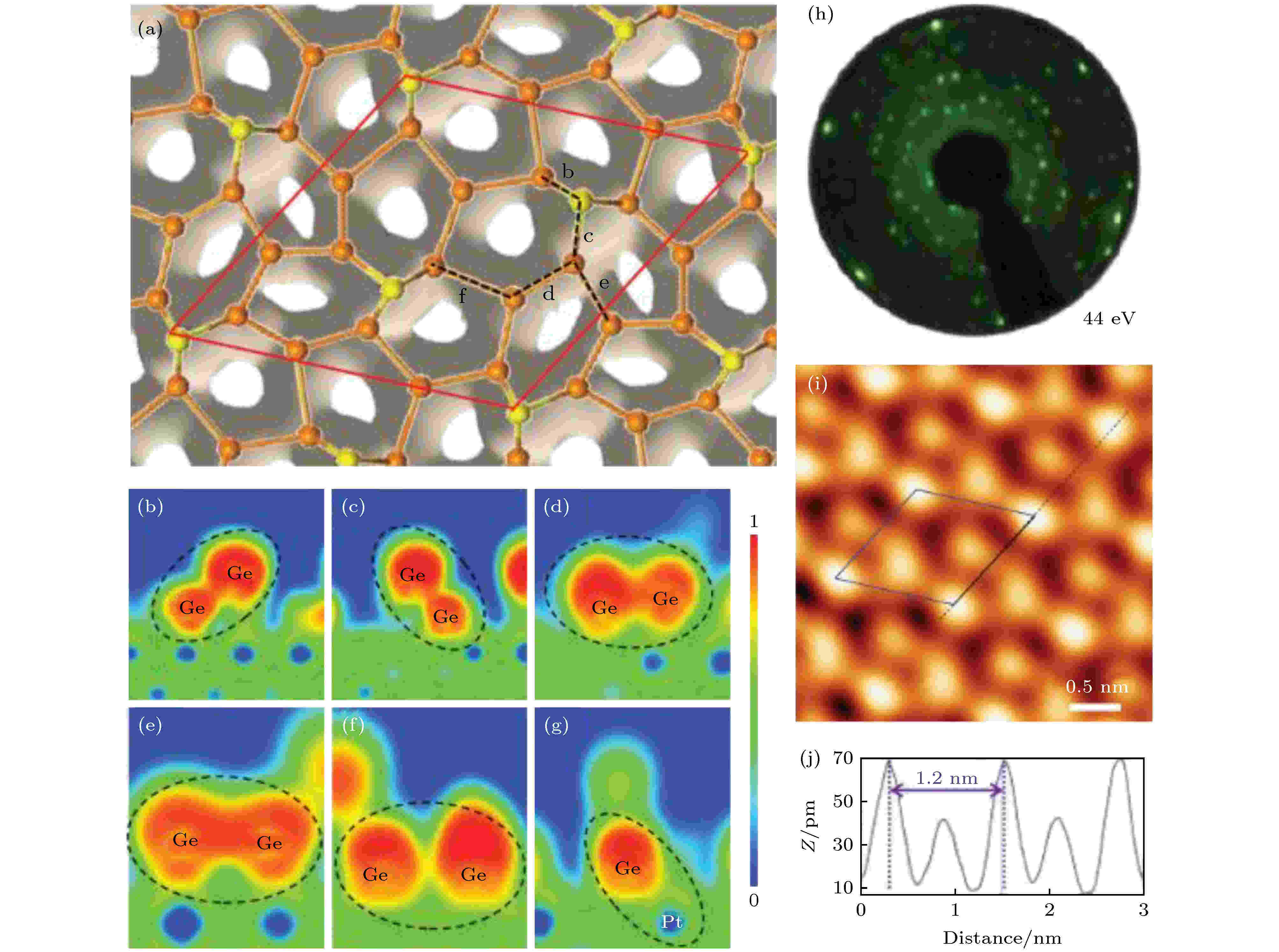
Figure3. (a) Theoretical model of germanene on Pt (111) surface, and the electron localization functions of the cross-sections between the germanium pairs (b)?(f) and between one germanium atom and its nearest Pt neighbor (g). (h)?(j) The experimental results of LEED pattern, STM image and the apparent height along the indicated line in the STM image, respectively[37].
近期, 研究人员在Cu(111)表面用MBE方法也成功可控制备出单层和双层(Bernal-stacked)锗烯[38], 如图4所示. 相对于Pt(111)表面, Cu(111)的晶格常数与锗烯晶格匹配度更好, 因此制备出的锗烯更为规整, 单层锗烯展现出蜂窝结构. 密度泛函理论模拟计算证实, 双层锗烯的蜂窝结构由两层锗烯堆垛组成, 是一种翘曲结构, 这与Ag(111)表面的硅烯十分类似[44]. 理论和实验结果都证实, 单层和双层锗烯在电子结构上具有很大区别, 单层锗烯由于只有一层原子且直接与金属基底相接触, 因此它们之间具有较强相互作用, 而双层锗烯中的上层锗烯则被下层锗烯屏蔽了来自铜基底的电子散射作用, 展现出近自由锗烯具备的狄拉克电子态, 因此, 在扫描隧道显微谱(STS)中呈现出费米能级附近的“V”形对称电子结构, 而在实验中锗烯的单双层数可以通过调节MBE的生长条件即可实现精确控制.
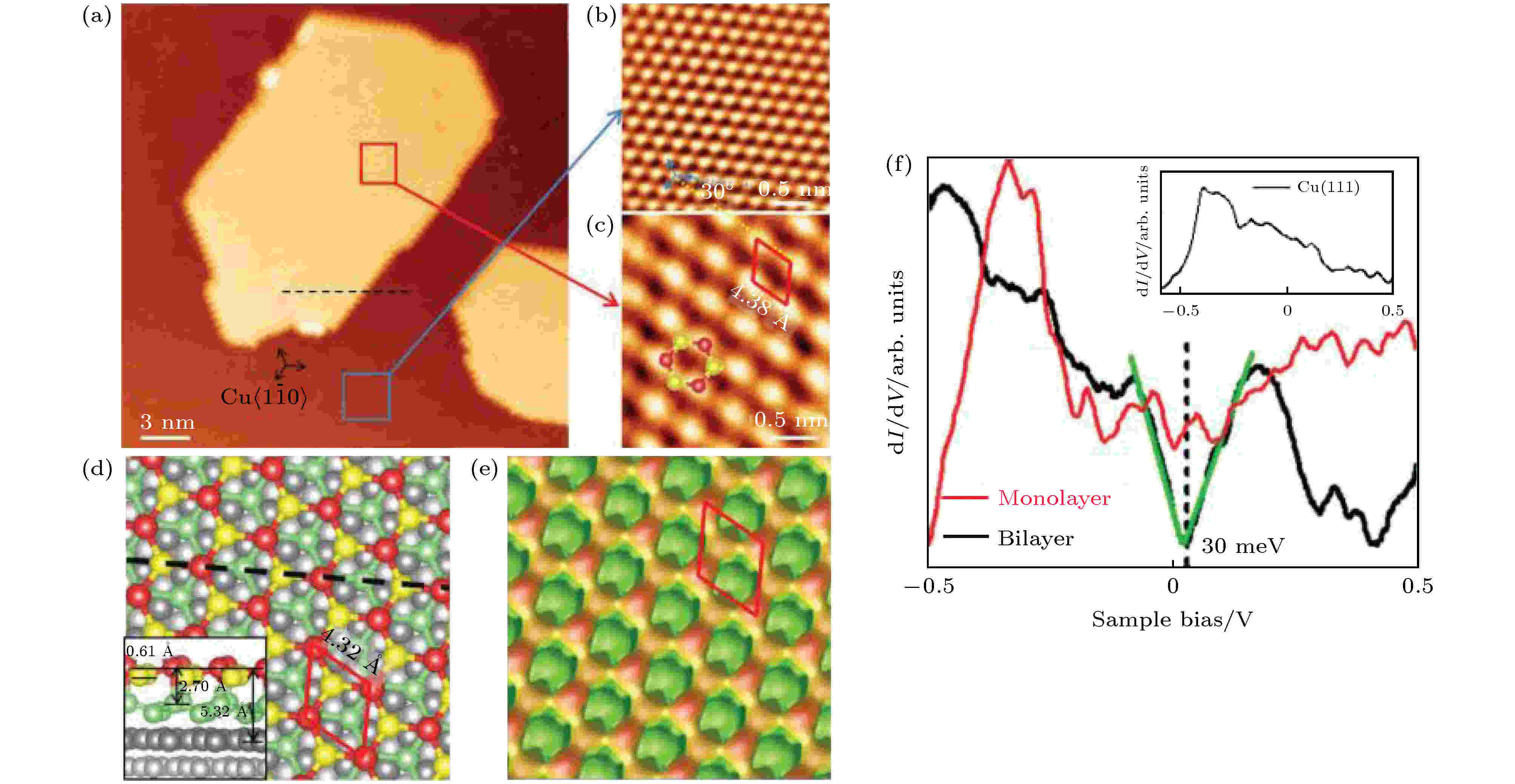
Figure4. (a) STM image of germanene on Cu(111); (b), (c) the atomic-resolved STM images of Cu (111) substrate and germanene, respectively; (d) the adsorption model of bilayer germanene; (e) the simulated STM image with the features fitting very well with the experimental observations; (f) the STS of monolayer (red) and bilayer (black) germanene, and inset is STS taken on the bare Cu(111) to verify the condition of the tip[38].
锗烯还在其他衬底表面成功实现MBE生长制备, 包括Au(111)[45]和Al(111)[46]等. 类石墨烯二维材料, 除锗烯外, 硅烯[44,47]、硼烯[48-50]、铪烯[51]、锡烯[39]等都被用MBE方法成功制备.
与石墨烯类材料类似的, 同样具有蜂窝状原子结构的过渡金属硫族化合物(transition metal dichalcogenide, TMD)是一类非常重要的二维材料. 近期, 通过加热蒸发硒原子, 研究人员在Cu(111)衬底上用MBE方法沉积了高度有序的蜂窝状结构(图5)[52]. 在该结构中, 硒原子在表面形成相对于Cu(111)衬底的


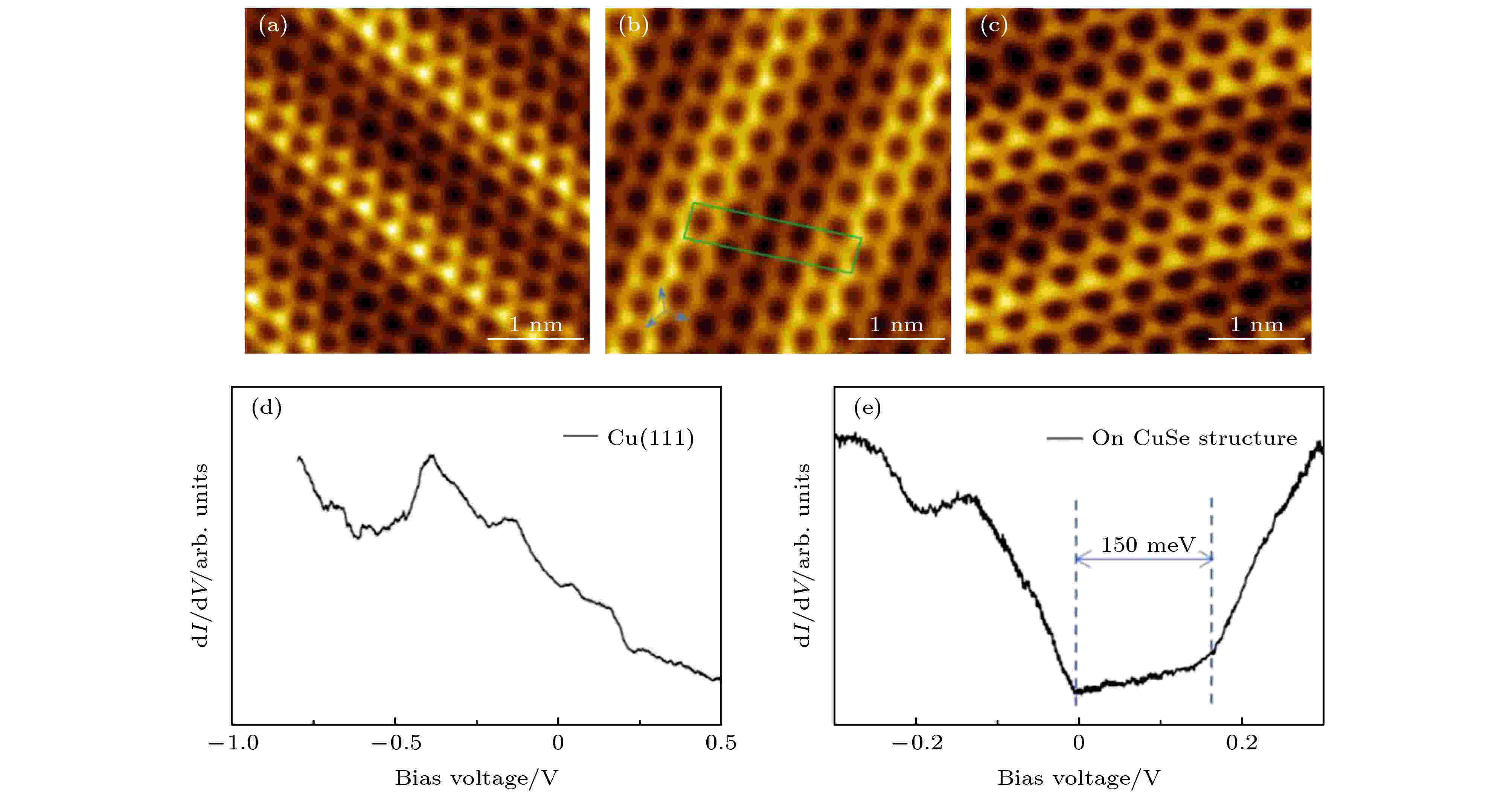
Figure5. (a)?(c) The STM images of honeycomb structures with equivalent orientations on Cu(111) by means of MBE growth; (d) the standard STS of Cu(111) for checking tip status; (e) electronic structure (STS) of CuSe structures[52].
在众多影响微纳电子器件性能的因素当中, 沟道材料本征性质毫无疑问起着决定性作用, 因此添加电荷脱耦层来研究器件材料本征性质非常重要. 氯化钠作为一种绝缘离子型化合物, 带宽可以达到6—8 eV, 且非常惰性, 其超薄薄膜可以用作屏蔽表面分子与基底相互作用的脱耦层. 如图6所示, 研究人员利用MBE方法在Cu(100)表面外延生长了2—3个原子层厚度的氯化钠超薄膜[36], 这种厚度的NaCl层在扫描隧道显微镜下不会影响正常隧穿成像, 同时还可以有效屏蔽分子与金属基底间的较强相互作用. 通过对其上吸附的有机单分子CoPc的电子性质研究表明, 分子显现出近自由分子的状态[53], 说明超薄NaCl层已经足够屏蔽来自衬底的电子散射作用, 可以用于研究微电子器件材料的本征性质. 为了与传统硅基半导体工业结合, 法国研究组[54]在Si(111)表面成功MBE外延生长了超薄氯化钾薄膜. 这些工作将为发展二维材料器件、筛选材料性能提供重要的实验基础.
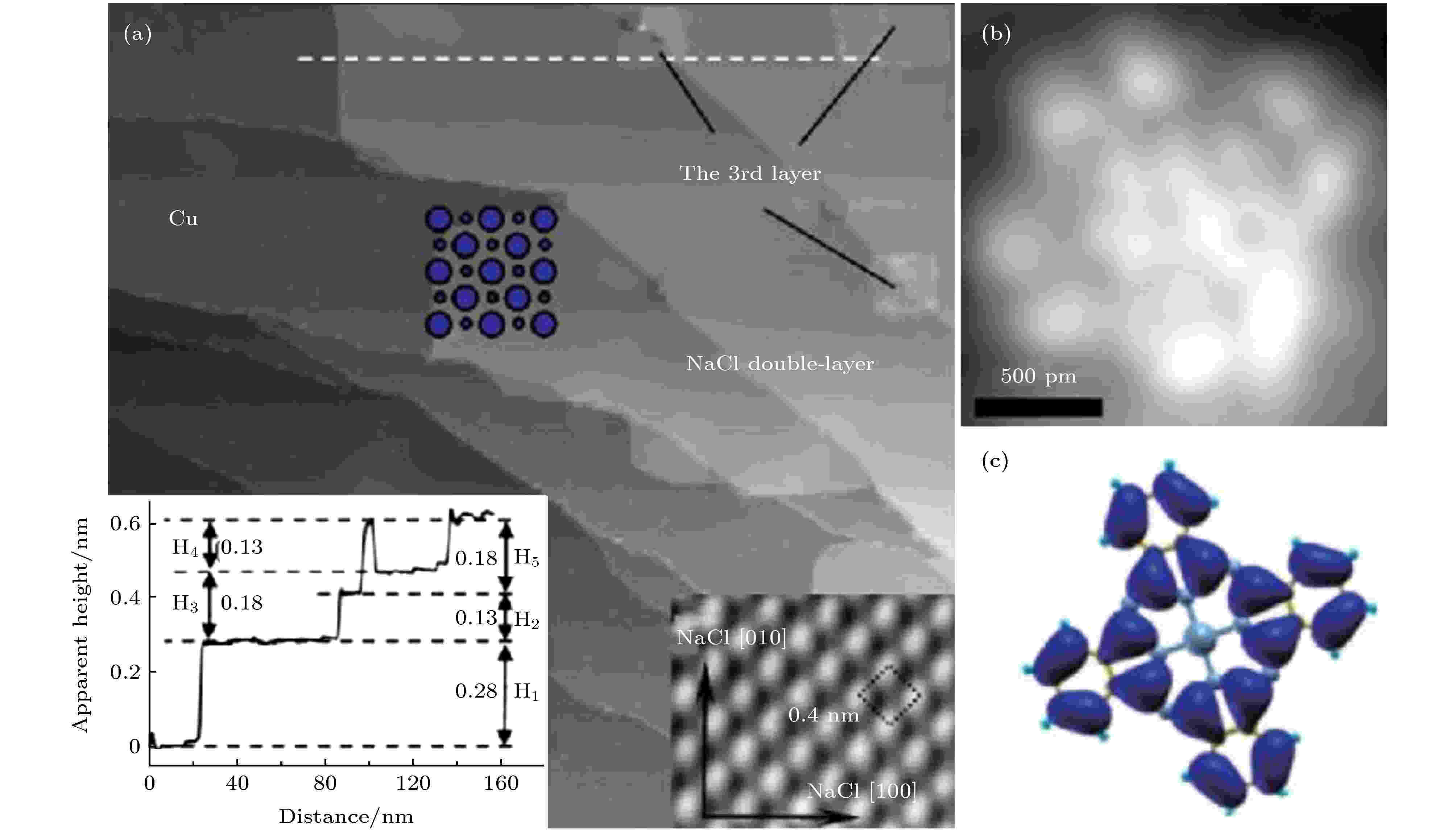
Figure6. (a) MBE growth of NaCl layers on Cu(100)[36], on top of which the quasi-free molecular orbital of adsorbed CoPc can be observed. (b) and (c) are the STM image and theoretical simulation of molecular orbital, respectively[53].
CVD法生长石墨烯的机理主要有两种[75], 如图7所示, 一种是偏析机制, 以Ni箔基底为代表, 在生长过程中, 含碳有机化合物前驱体(如CH4等)会溶解到Ni箔体相中去形成含碳固溶体, 然后该固溶体表面偏析出碳原子形成石墨烯; 另一种是表面反应机制, 以Cu箔基底为代表, 前驱体分子在CVD过程中首先吸附在表面, 然后发生表面化学反应分解前驱体, 使碳原子留在表面形成石墨烯.
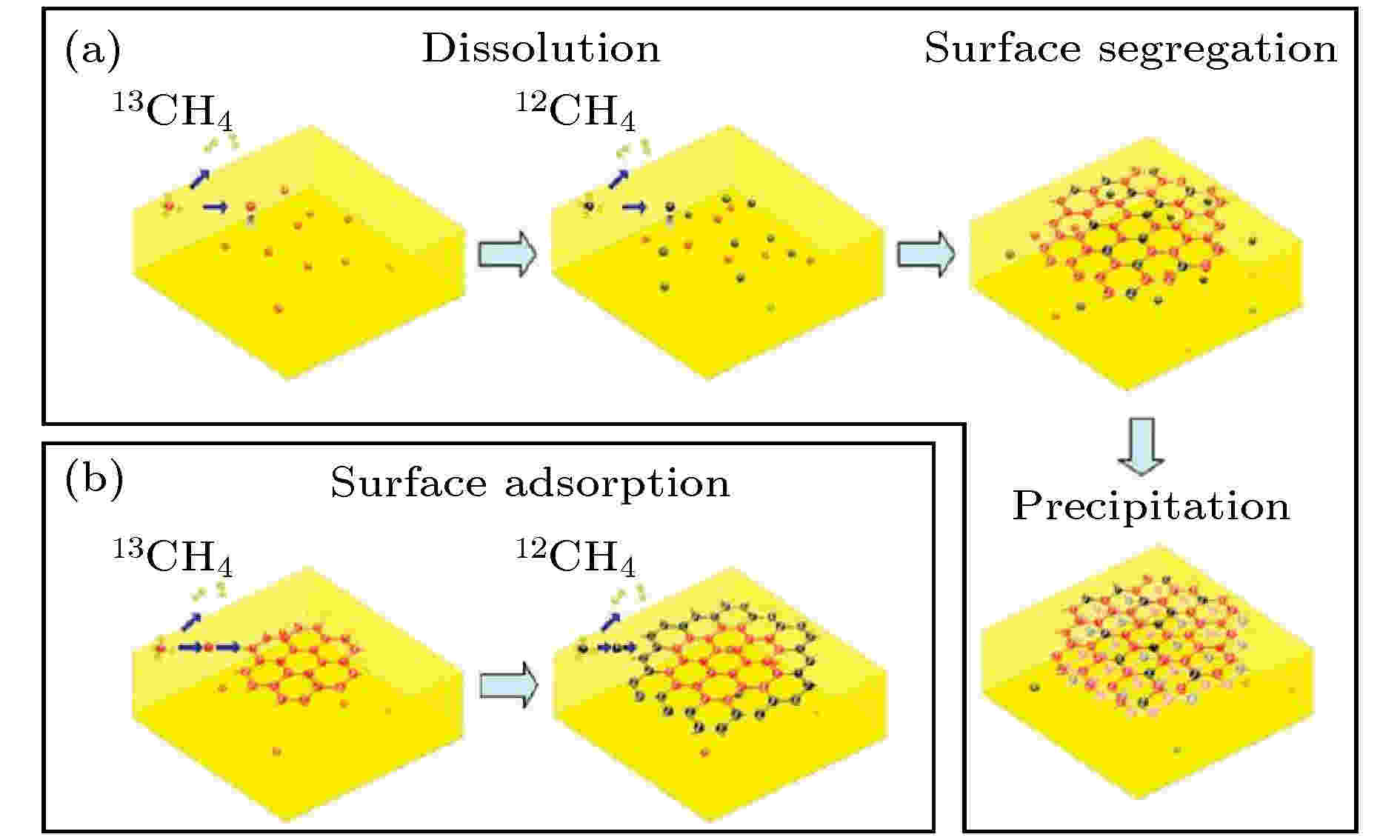
Figure7. Two main mechanisms of CVD growth of graphene[75] (a) Segregation mechanism; (b) surface reaction mechanism.
2
3.1.原子层沉积
随着化学气相沉积技术的发展, 衍生出一种特殊的化学气相沉积技术—原子层沉积(atomic layer deposition, ALD), 亦称原子层外延(atomic layer epitaxy)技术. 与传统化学气相沉积的连续过程不同, 它是利用不同气相前驱体脉冲交替通入反应腔室, 周期性间歇式地在表面沉积材料的一种方法. 它的主要机理是通过特殊的具有自限性(self-limiting)和自饱和性(saturated)的表面化学反应实现对生长过程的自动控制[76]. ALD最早是由苏联[77,78]和芬兰[79]的科学家在六七十年代分别独立提出的, 经过不断的发展, 已成为一种强有力的薄膜制备手段[18,61,76,80-99]. 目前在微电子工业[82,87,100-102]、能源催化[86,103-105]、光学[106]、纳米技术[107]和生物医学[108,109]方面发挥着重要作用. 例如, 在2007年, Intel公司率先将ALD制备所得的超薄铪基氧化物作为栅极介质层引入到45 nm节点的半导体工艺中, 获得了功率更低, 速度更快的酷睿微处理器.典型的原子层沉积系统如图8所示, 通常由前驱体源、气路系统、电子控制系统和真空系统构成. 随着原子层沉积在实验中的不断优化, 逐步衍生出了各式各样的原子层沉积系统, 包括等离子体增强原子层沉积(PEALD)[110,111]、分子层沉积(MLD)[81,112,113]、空间ALD[114-117]、电化学ALD[118,119]等. 其中PEALD属于能量增强型ALD, 能够生长传统ALD难以生长的一些材料, 如金属、氮化物等; 空间ALD则突破传统ALD技术的时间和空间限制, 可以实现薄膜的连续式快速增长进而大批量制造. 这些方法的核心是利用表面自限制反应来达到逐个原子层生长的目的, 通过控制半反应过程次数可以很精确地在原子尺度控制原子层数. 举例说来, 晶体管器件中常用的Al2O3层是以有机金属化合物三甲基铝(TMA)作为金属铝源, 水蒸汽为氧源进行ALD沉积, 总反应过程由以下反应式来表述: Al(CH3)3+ H2O→Al2O3+CH4. 制备过程中, 每个制备循环包含两个半反应和两次吹扫过程, 如图9所示.
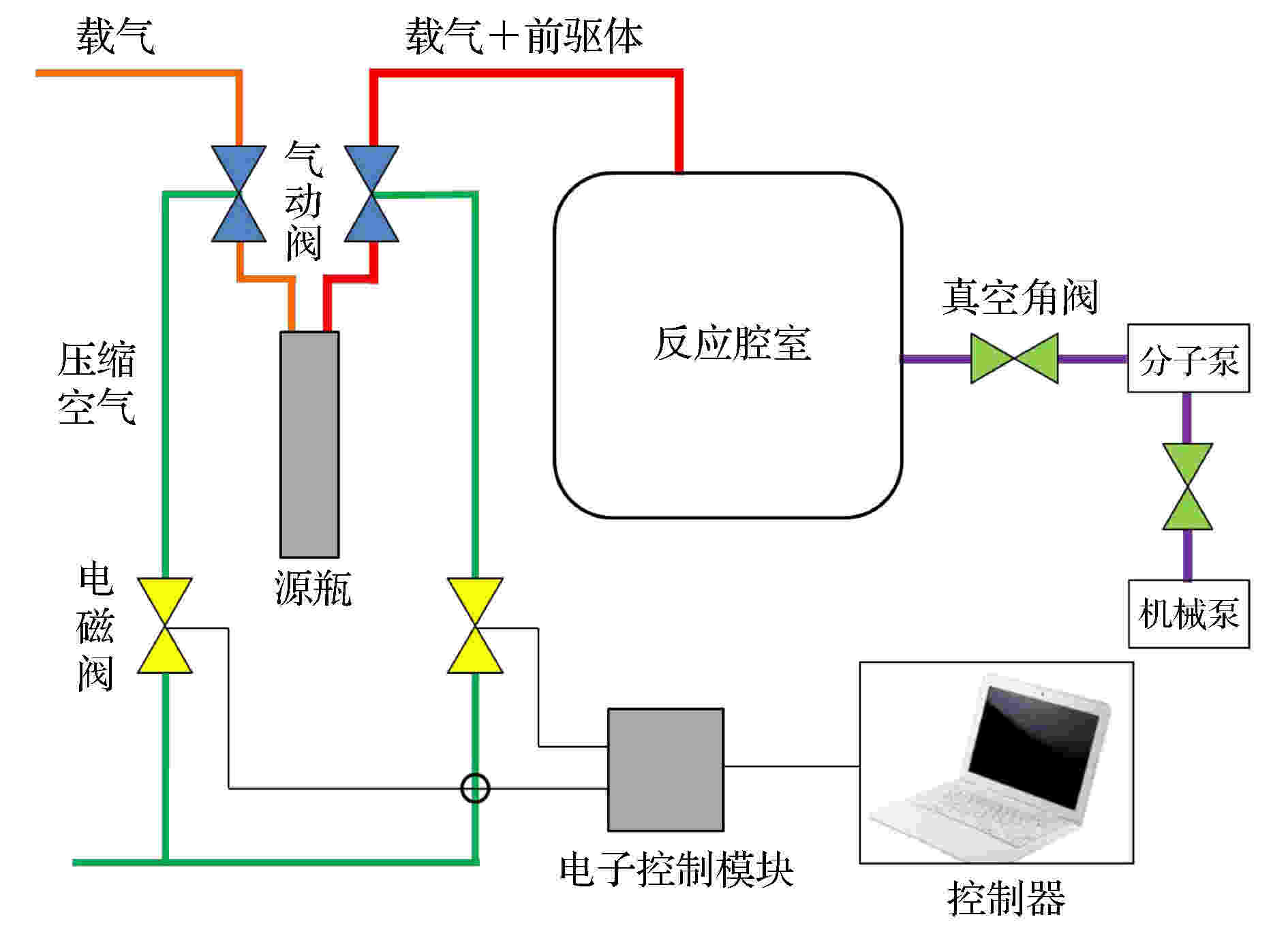
Figure8. Schematic diagram of atomic layer deposition system.
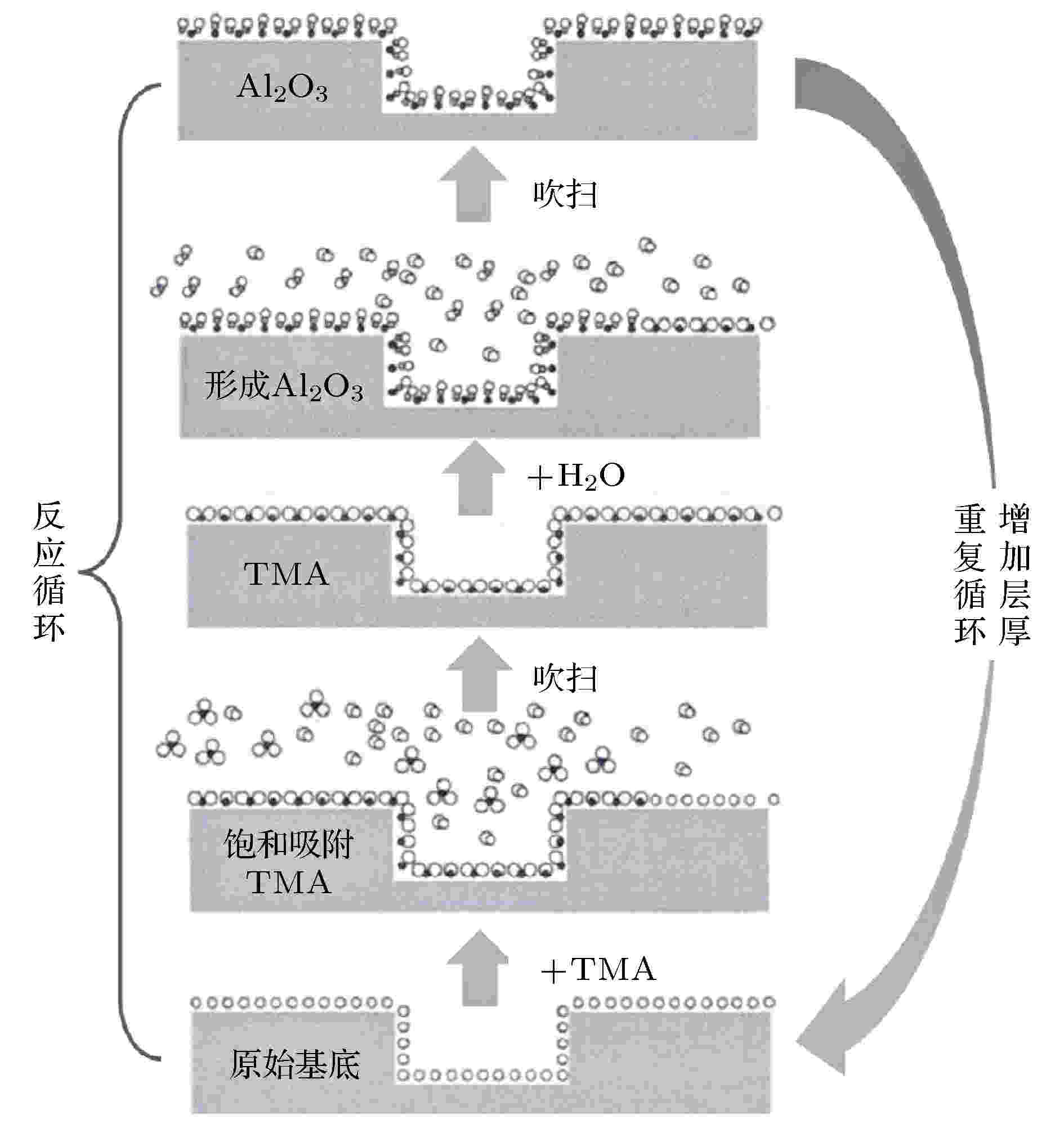
Figure9. The ALD process of Al2O3[76].
相比其他沉积方式(如图10(a)所示), 例如传统化学气相沉积、物理气相沉积、溶胶凝胶(sol-gel)等薄膜沉积技术, ALD具有优异的三维贴合性(conformality)和大面积均匀性(uniformity), 特别适合复杂表面形貌及高深宽(high aspect ratio)结构的填隙生长. 在具有窄纳米深槽的硅基底进行Cu2S薄膜生长实验[120], 结果表明薄膜具有近100%的覆盖率和良好的贴合性(图10(b)), 保形性是ALD最突出的优点, 可很好地解决目前功能器件中的缺陷和均匀性的问题.
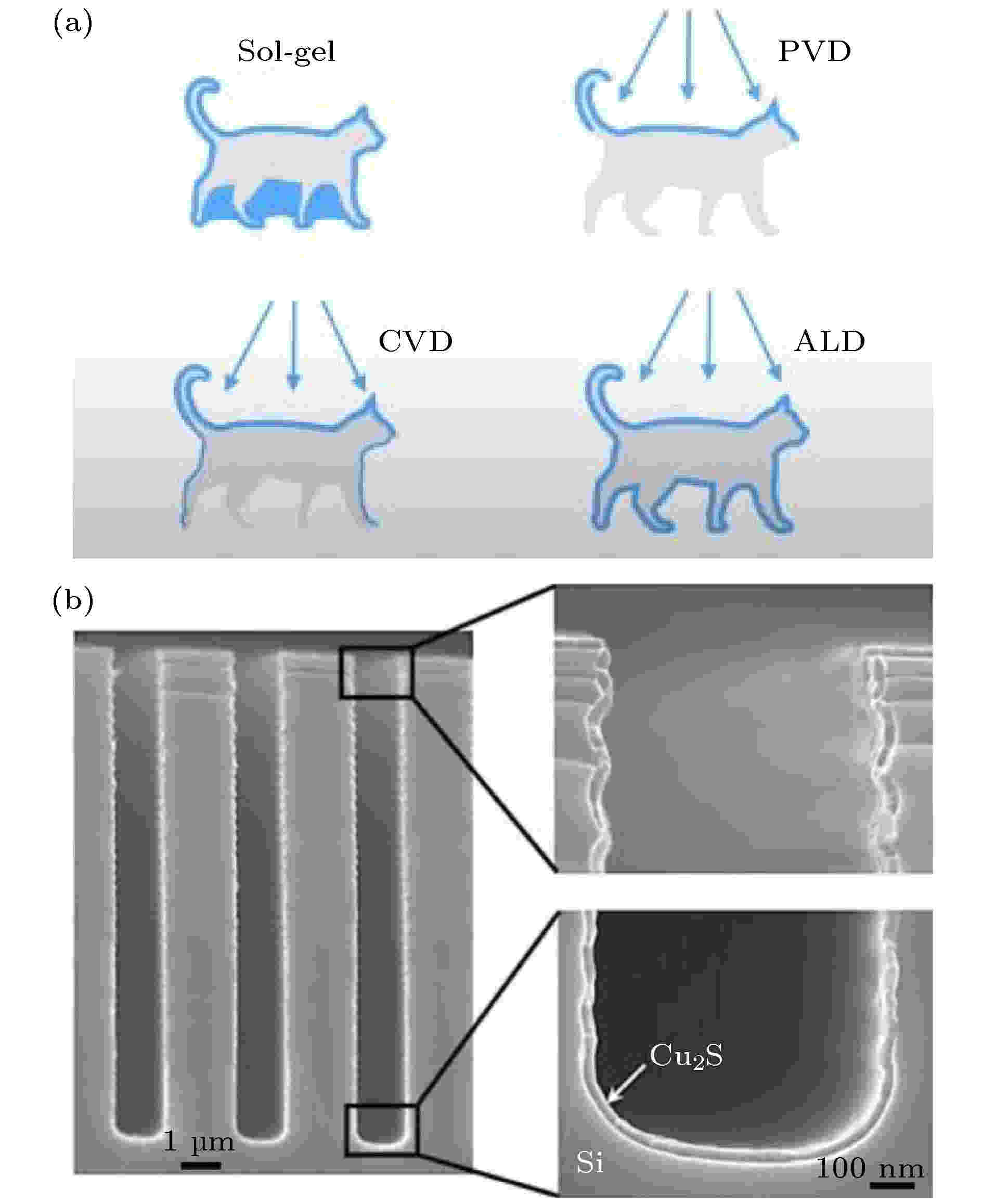
Figure10. (a) The coating effects of ALD and other methods; (b) cross-sectional SEM images of ALD Cu2S film on silicon trench structure[120].
在沉积过程中, 影响原子层沉积速率的因素包括气体暴露量和沉积温度. 对大多数前驱体而言, ALD过程中完全占据基底表面的过程大约会在2 s内完成, 延长吸附时间只会造成前驱体浪费, 并不能提高吸附量; 而吹扫过程一般会在10 s左右, 否则会造成上一个前驱体残留于下一个前驱体在到达表面前就发生反应, 影响表面共构型和均一性.
化学吸附是一个热力学过程, 会受反应温度的影响. 如图11所示, 一般来说原子层沉积速率有一个温度窗口, 若低于窗口温度, 前驱体会产生物理冷凝吸附; 而温度过高的话, 前驱体会受热分解, 甚至已经沉积好的原子层会解吸附. ALD过程中需要通过控制使整个基板不同区域的温度处于原子层沉积温度窗口, 沉积速率接近恒定值.
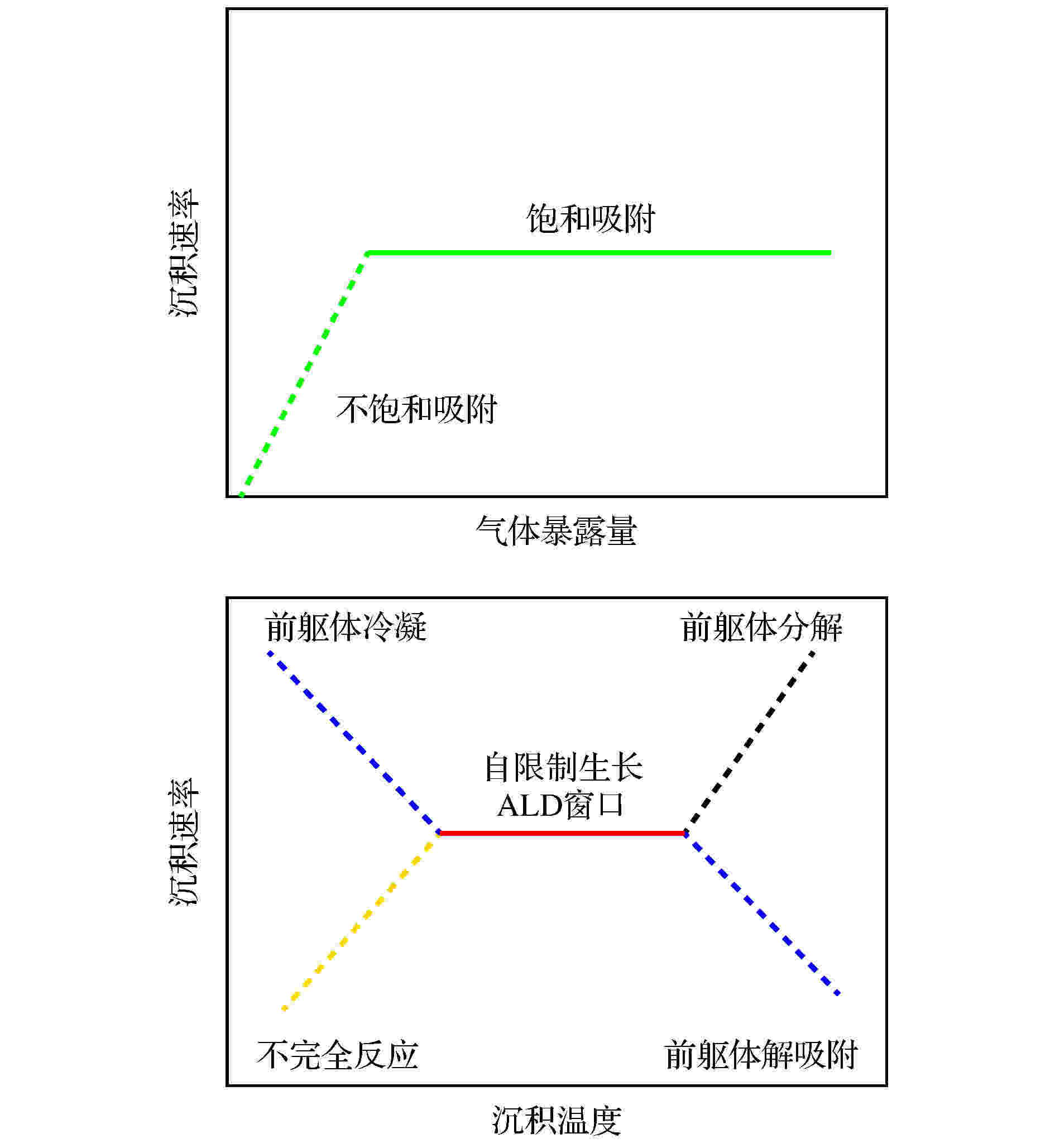
Figure11. Effects of gaseous precursor exposure and deposition temperature on deposition rate of atomic layers.
除此之外, ALD生长过程中对沉积的表面也非常敏感. 例如, 生长SiO2时, 表面具有亲水性的—OH基团的区域就容易成核, 而在具有疏水性—H的表面则几乎不成核[100,121]. 目前精密微纳加工中迫切需要实现薄膜自对准生长, 基于原子层沉积的方法, 发展了选区原子层沉积(area-selective ALD, AS-ALD)即特定区域选择性沉积. 目前在已经实现的AS-ALD主要有两种方式: 自组装单层膜法(SAMs)和聚合物法[122]. 这两种方法的原理都是利用有机分子SAMs或者聚合物去钝化或者活化特定区域, 然后使得前驱体与这些区域有不同于原表面的反应活性. 一般来说, 钝化的情况更为常见, 即SAMs层或聚合物层阻碍反应进行, 使得薄膜不能在该区域生长[101,121,123-125].
选区ALD中常用的SAMs薄膜通常是由长链型分子通过自组装形成, 两端的功能基团一方面可以选择性吸附在亲/疏水区域, 另一方面可以根据实际需求阻止或者允许后续的ALD过程的进行. 斯坦福大学Chen等[121]利用AS-ALD方法, 精确将高介电常数(high-k)氧化铪薄膜沉积到用SiO2/H-Si模板衬底上, 如图12所示, 制备出芯片的基本单元—金属氧化物半导体场效应管(MOSFETs)的结构模型. 他们首先将十八烷基三氯硅烷(ODTS)通过自组装的方式选择性沉积到表面有亲水的-OH基团的SiO2薄膜上, 然后用Hf[N(CH3)2]4和去离子水作ALD反应前驱体制备HfO2薄膜. 由于ODTS分子的SAMs层钝化作用[100,121], 该薄膜会选择性的沉积到Si-H区域, 最后再用溶剂清洗去除物理吸附的SAMs钝化层, 就形成了MOSFETs的结构模型. ZnO, Al2O3, ZrO2甚至金属Pt薄膜都可用SAMs方式的AS-ALD制备出来[101,126-128].
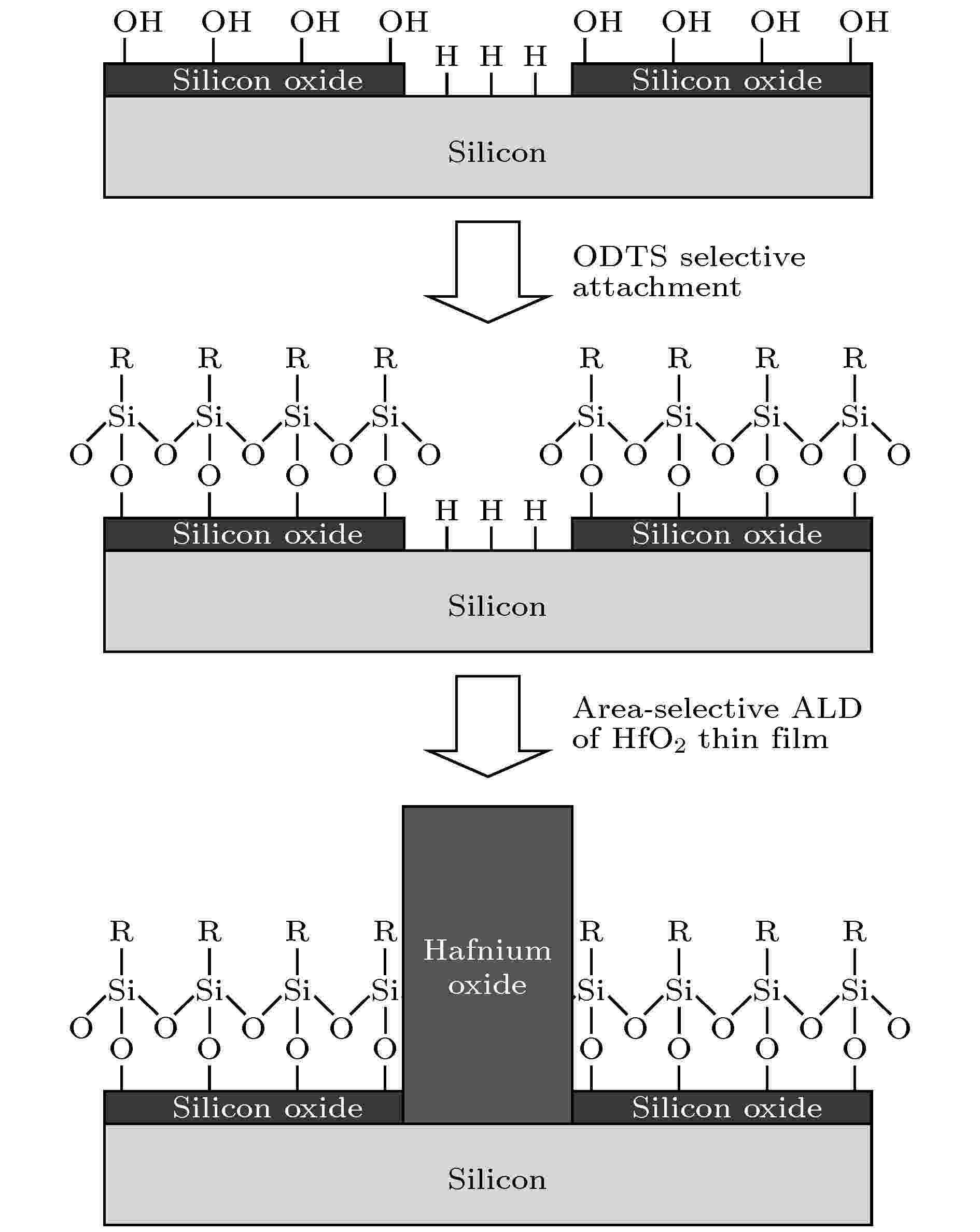
Figure12. The area-selective ALD of high-k hafnium oxide on SiO2/H-Si surface to fabricate MOSFET prototype.[121]
在AS-ALD过程中, SAMs薄膜靠长链分子间的范德瓦耳斯力自组装成单层膜, 这种物理吸附作用力较弱, 聚集过程中容易出现分子卷曲导致SAMs膜出现针眼(pinhole)等缺陷, 而这些缺陷位置又在ALD过程中具有一定活性, 会导致在本该是阻挡层的材料表面出现ALD沉积材料, 并出现如图13(a)所示的沉积材料向阻挡层方向溢出的情况, 严重影响纳米器件性能. 为了解决该问题, Wojtecki等[101]通过对组成SAMs薄膜的分子进行官能团修饰, 例如, 引入增强分子氢键和π-π相互作用的基团(如图13(a)右图所示), 通过自组装形成SMAs膜(图13(b)), 而实验证实最有效的手段是在长链分子中引入可以发生光聚合反应的二炔基基团. 在光照条件下, SAMs膜中分子进一步交联聚合(图13(c)), 有效地抑制缺陷的形成, 保证对后续ALD反应的惰性. 用这种方法获得的结构最窄宽度约为15 nm(图13(d)), 理论上AS-ALD制备微纳结构的精度可以达到原子尺度.
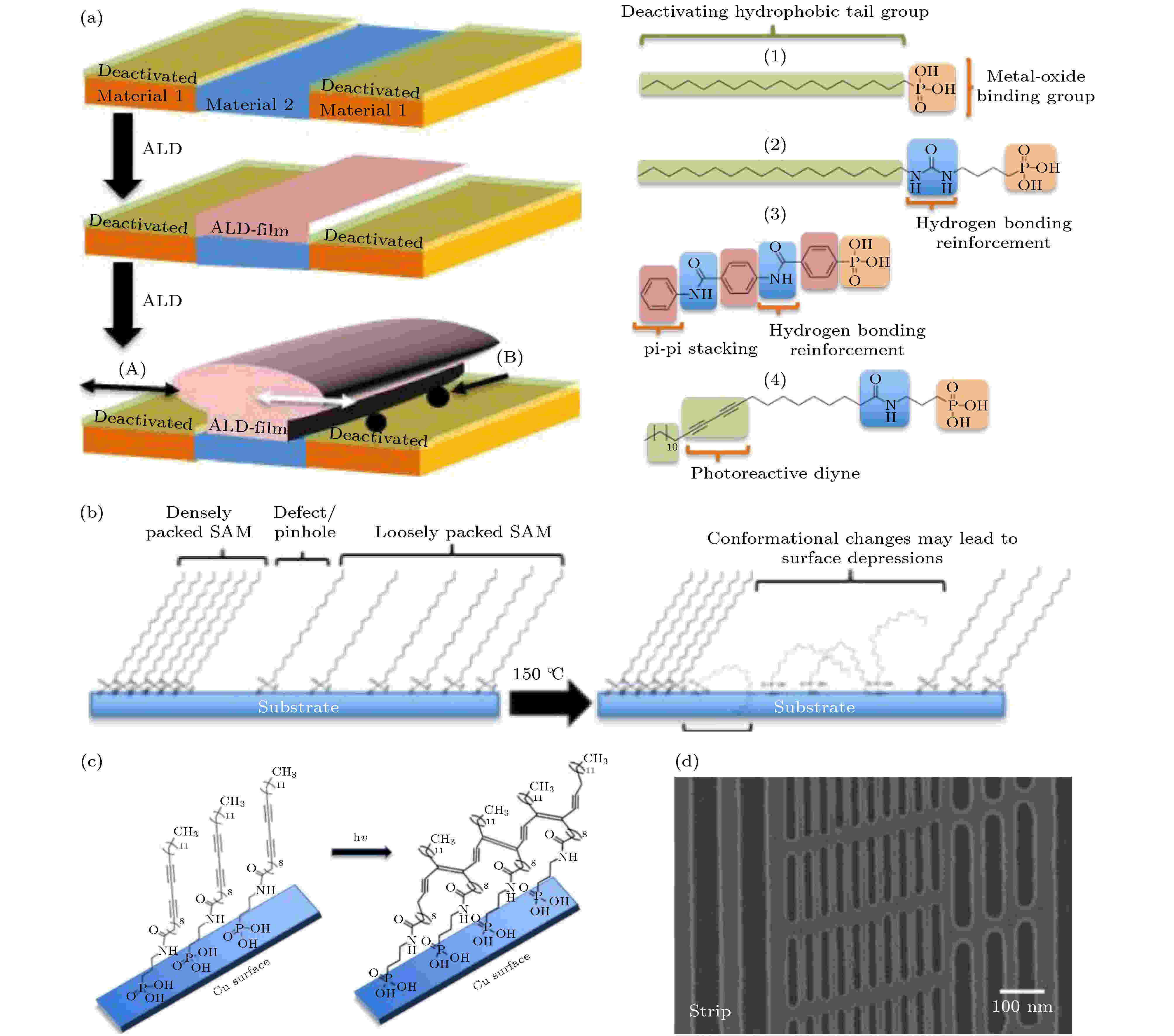
Figure13. (a) Schematic diagram of area-selective ALD growth (left), and the monomer molecular structures forming inactive SAMs (right); (b) the pinhole defect affects the selectivity of ALD deposition; (c) photopolymeric functional groups (diacetylenyl) of SAMs can effectively inhibit defect formation in terms of photo-induced polymerization; (d) SEM micrograph of the microstructure obtained by etching with ZnO mask of area-selective ALD, and the width of narrowest structure reaches 15 nm[101].
2
3.2.原子层刻蚀
上面所介绍的技术都是在基底上沉积材料, 但是在复杂的微纳电子器件制造过程中, 去除特定材料和厚度的薄膜, 即刻蚀过程, 同样非常重要. 成熟的芯片刻蚀工艺包括干法刻蚀和湿法刻蚀. 干法刻蚀利用气体等离子体在真空腔中去除表面层材料, 是一种物理方法; 而湿法刻蚀就是利用化学腐蚀溶液的方法去除表面层的原子. 相对于干法腐蚀, 湿法腐蚀精度低, 通常用于中低端芯片的生产, 而最先进的芯片制造都使用干法腐蚀技术. 值得一提的是, 我国中微半导体设备(上海)有限公司自主研发的5 nm等离子体刻蚀机已经经过权威芯片公司台积电的生产验证, 性能优良, 但是这么高精度的芯片中晶体管的几何尺寸已经非常接近物理极限, 因此需要发展新型的原子精度的刻蚀技术—原子层刻蚀技术(atomic layer etching, ALE)[129-141].由于是以原子精度去除材料, 早期ALE技术被认为效率过低不能应用于实际生产[129,130]. 目前, 器件尺寸已经发展到纳米量级, 这种劣势已经逆转成为独特的优势; 而且ALE可以看作ALD的逆过程[131,132], 同样具有自限性和自饱和性的特点, 因此无论结构的深宽比如何, 每个循环都只去除一层材料的原子, 相比传统的等离子刻蚀工艺, ALE具有更为精密的原子级精度控制, 极有可能成为下一代芯片制造的主打工艺.
ALE过程可分为两个半反应, 如图14所示, 第一个反应主要是自限性吸附前驱反应物, 使其与表面形成覆盖全表面的单层改性薄膜, 这层薄膜的形成导致原先第一层原子与内层体相原子结合能减弱, 然后第二步通入等离子体使得表面改性的分子脱附, 露出新的一层材料原子, 完成单层原子刻蚀, 这样周而复始, 以原子精度刻蚀材料. 从整个过程可以看出, 要完成完美的单层刻蚀, 第二步的等离子体能量至关重要, 能量太弱不足以完全去除改性层分子, 能量太强则会把底层原子一同去除, 但只要离子能量位于改性分子脱附能与第二层原子脱附能之间的能量窗口, 就可以实现可靠的ALE刻蚀过程.
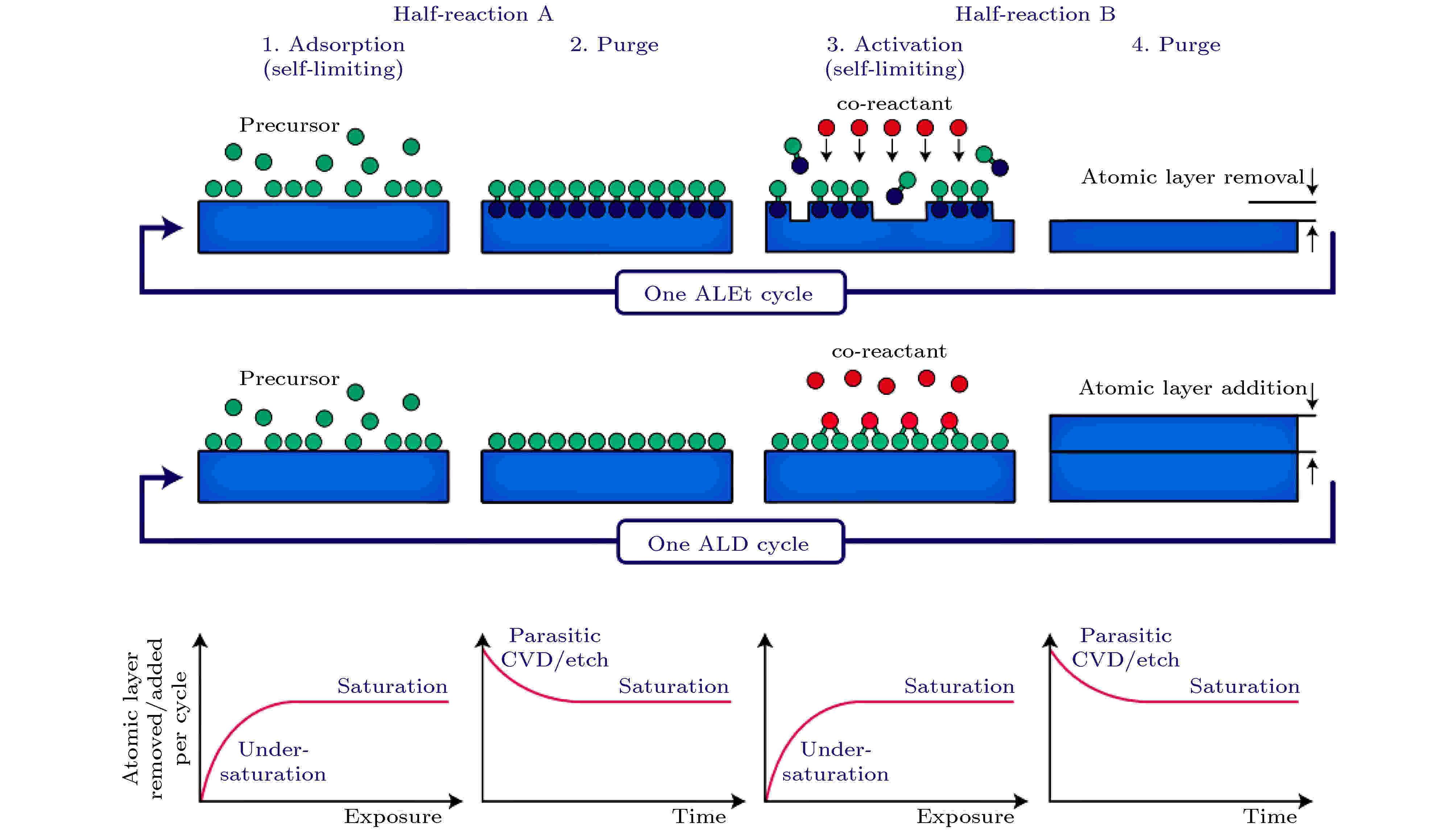
Figure14. Schematic diagram of ALE process compared with ALD[132].
石墨烯等二维材料由于具有高载流子迁移率且有大开关比等优异的电子特性, 理论上非常适用于制造更快更节能的纳米电子器件, 因此, 对于它的原子精度加工尤为重要. 近年来, 很多研究人员致力于石墨烯材料的纳米形貌和电子性质调控[66-74,131], 而ALE技术则是非常有效的原子级精度加工手段. 2017年, 韩国Kim等[136]将铜箔衬底上CVD生长的单层和双层石墨烯, 经PMMA转移到SiO2/Si基底上, 然后通过ALE技术对该石墨烯实现了可控的原子级刻蚀.
图15(a)和图15(b)为双层石墨烯刻蚀前后的光学显微图像, 仅通过一个循环的ALE过程, 就实现了单层石墨烯的均匀刻蚀. 为了确认刻蚀效果, 对该样品进行了原子力显微镜(AFM)成像, 如图15(c)和图15(d)所示, 图中黑色线是石墨烯层厚的高度轮廓线, 刻蚀后石墨烯厚度由1.45 nm变为0.72 nm, 而在石墨烯薄膜的不同区域(见图15(a)和图15(b)中点1—12)测得的拉曼光谱(图15(e))可以看出, 石墨烯在这些位置已经由双层变为单层, 说明已经被均匀的刻蚀掉一个单原子层. 而对于单层石墨烯, 拉曼光谱数据表明一个ALE刻蚀循环就可以实现石墨烯的完全去除, 如图16所示, 所有单层石墨烯的特征峰都在一个ALE循环后消失.
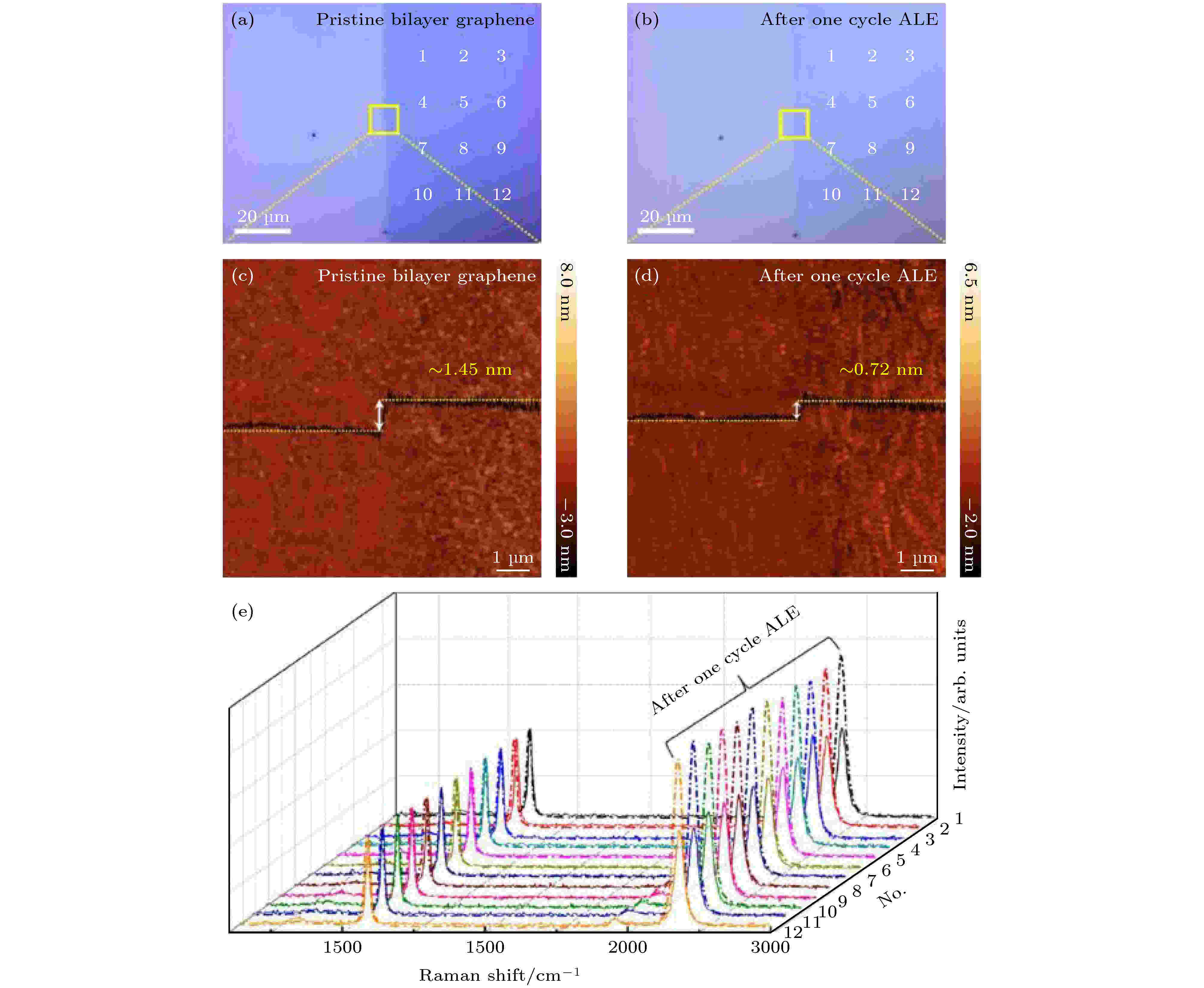
Figure15. Optical microscopic images (a), (b) and AFM images (c), (d) of bilayer graphene before and after one cycle of ALE etching. (e) Raman spectrum of graphene taken at twelve points indicated in (a), (b) before and after etching[136].
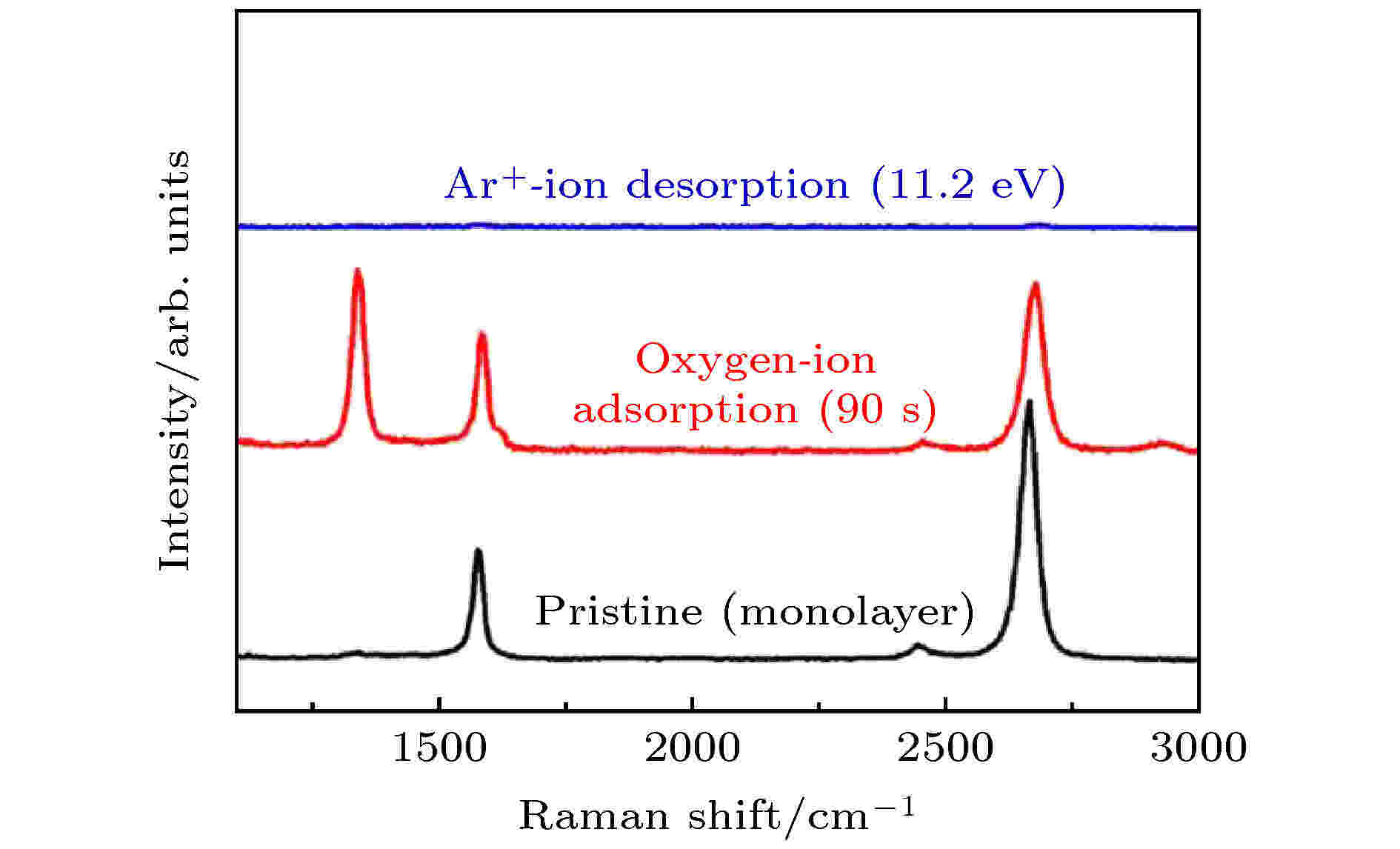
Figure16. Raman spectrum of monolayer graphene before and after one cycle of ALE[136].
当然, 只是无差别的去除整层石墨烯是无法实现对纳米器件制备的, 需要结合纳米掩膜技术. 研究人员利用聚苯乙烯(PS)纳米球自组装密排列结构作为掩膜, 通过CF4和O2的等离子体进行ALE, 成功在SiO2表面制备了石墨烯纳米掩膜[142], 如图17所示. 研究人员先在SiO2/Cu箔表面自组装PS纳米球, 它们会自发排列成六角密堆结构, 然后通过CF4等离子体刻蚀掉没有覆盖PS纳米球的区域, 然后溶剂清洗去除PS纳米球, 露出图形化的SiO2区域, 再通过CVD沉积石墨烯, 因为CVD过程中铜箔具有催化作用, 而SiO2没有催化性, 所以成型的石墨烯具有孔洞形状, 最后用具有选择性的ALE对表面SiO2区域进行刻蚀, 最后得到在铜箔表面的具有纳米模板的石墨烯(图17(g)).
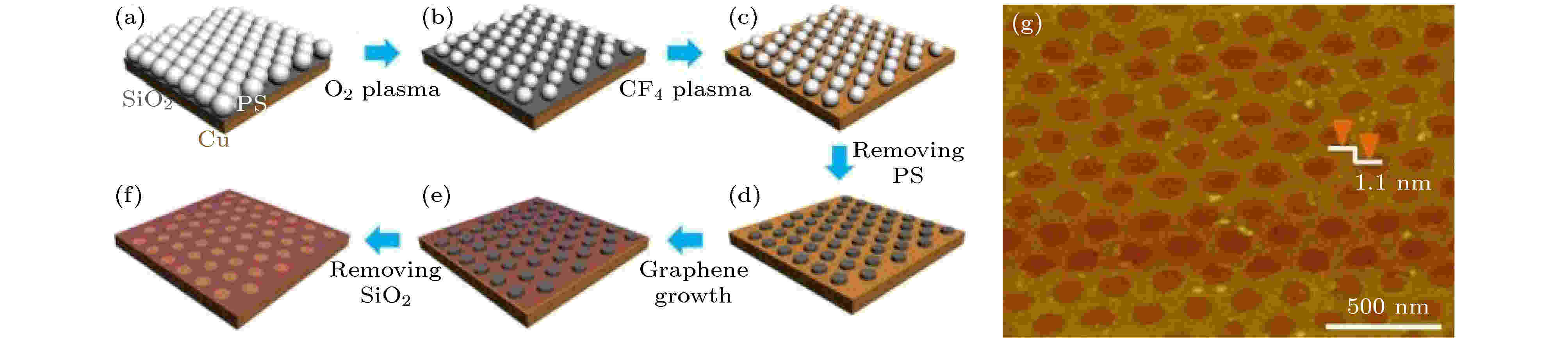
Figure17. (a)?(f) The growth and etching processes of graphene via PS nanoparticle mask; (g) the nano-patterned template graphene on Cu foil[142].